Abstract
Increased detrimental impacts of biological invasions have triggered the development of risk screening tools to streamline the identification of the invasive potential of organisms. These tools assess the biological and historical characteristics of non-native species and predict their invasiveness in a given geographical area. However, challenges for identifying such invasive potential often require a holistic approach, if more accurate predictions are needed. Here, a case study is provided that combines molecular analysis (mtDNA barcoding) with a risk screening (Aquatic Species Invasiveness Screening Kit: AS-ISK) and impact assessment scheme (Environmental Impact Classification for Alien Taxa: EICAT) to address the origin, distribution and potential impact of non-native ruffe Gymnocephalus cernua in Turkey. Recently, several populations of this species have been recorded from the Turkish region of Thrace (the European part of Turkey), which represents the species’ southernmost geographic range of expansion in Europe, where it is non-native. Molecular data suggested the inclusion of populations from Thrace in a haplogroup widespread in eastern and western Europe from where they might have been introduced, as well as natural spread of the species into Thrace through connected river systems rather than by intentional transport. The AS-ISK scores, also accounting for the effects of climate change, indicated that G. cernua is likely to pose a high risk of being invasive in Thrace but a medium risk in Anatolia (the Asian part of Turkey), and the EICAT scores indicated “major” impact. This study suggests that, in the near future, G. cernua has a high potential to invade all suitable habitats in Thrace and establish viable populations in several Turkish freshwater ecosystems, including those in Anatolia.
1. Introduction
Identifying the invasive potential of non-native species (NNS) is a complex process that has become necessary for the prevention, control and management of biological invasions. Most of the toolkits developed for the risk screening of NNS rely on expert opinion and use a scoring system for risk categorisation (Pheloung et al. Citation1999; Copp et al. Citation2005, Citation2016). These tools provide a rapid decision support to policy makers (Copp et al., Citation2005; Copp et al. Citation2021) and address the initial step of a more comprehensive risk analysis (Hill et al. Citation2020). Whilst there are numerous risk screening applications (see Vilizzi et al. Citation2019, Citation2021), studies dealing with follow-up risk assessment are relatively scarce. A full risk assessment examines in detail the risks of introduction, establishment, dispersal and impacts, and is generally limited to those species screened as high risk due to the major economic commitment involved in the process (Copp et al. Citation2005, Citation2016). Yet, the need for such studies has become even more compelling as a result of climate change and increased human-induced introductions of NNS, which require accurate predictions on their potential dispersal routes and pathways of introduction, range of expansion and, ultimately, impact. In a similar way to climate-niche modelling, which has been recently used to achieve robust predictions of the future range of expansion of NNS (Yoğurtçuoğlu et al. Citation2021), recent advancements in molecular techniques have helped to uncover their potential pathways, dispersal routes and invasion history (Estoup & Guillemaud Citation2010; Cristescu Citation2015). Within this context, the integration of molecular techniques to risk assessment in studies on biological invasions is still at an inchoate stage. The present study aims to fill this research gap by investigating, as a case study, the origin and invasion history of an aquatic NNS, namely the ruffe Gymnocephalus cernua.
Gymnocephalus cernua is a freshwater fish native to the Caspian, Aral, Black, Baltic and North Sea basins as well as to water bodies of Siberia (Kottelat & Freyhof Citation2007). Since the 1950s, the species’ range has largely expanded to France (Matthey Citation1966), the UK (Winfield et al. Citation1996) and the Great Lakes region of North America (Pratt Citation1988), mainly as a result of accidental introductions. Gymnocephalus cernua was also reported from the River Isonzo in Italy in the 1980s (Chiara Citation1986), and later in the year 2000 from the Hutovo Blato wetland in Bosnia and Herzegovina (Dulčić et al. Citation2005). One of the most recent records of this species has been from an artificial pond in northeast Algeria, which represents the southernmost point of the species’ range of expansion (Arab et al. Citation2020). Whereas in Europe, the southernmost non-native range of expansion of G. cernua was first reported (Kuru Citation2004) and later confirmed (Çiçek et al. Citation2021) from the Thrace region of Turkey, and specifically from the delta of the River Maritza – a transboundary river between Turkey, Greece and Bulgaria, with the occurrence of G. cernua previously reported from the latter two countries (Popova et al. Citation1998; Petriki et al. Citation2014). This finding is noteworthy, as Thrace is known to be one of the main routes for the introduction and establishment of several non-native freshwater fish species into Anatolia – the Asian part of Turkey (Tarkan et al. Citation2015). However, none of the records of G. cernua in Anatolia has so far been confirmed, and especially so by molecular analysis, nor has any study investigated the genomic origin of non-native species populations in this region except for the invasion history of topmouth gudgeon Pseudorasbora parva (Baltazar‐Soares et al. Citation2020).
Similar to gibel carp Carassius gibelio, pumpkinseed Lepomis gibbosus and Pseudorasbora parva (Tarkan et al. Citation2015), G. cernua is known to be an invasive species that, once established, can disrupt interactions amongst native organisms (Savino & Kolar Citation1996), and ultimately cause adverse impacts (Selgeby Citation1998). This potential for exerting ecological impacts is due to the species’ biological and ecological plasticity, which make it a sturdy and successful invader (e.g. Souchon & Tissot Citation2012). Indeed, G. cernua utilises a wide range of habitats including lakes, pools, estuaries, lagoons, and the margins of streams. Although this species is mainly found in waters up to 10 m depth with sand and gravel substrata, it is known to adapt to a wide range of depths (i.e. 0.25–85 m) in both mountainous and sub-mountainous zones as well as in oligotrophic and eutrophic water bodies (Bergman & Greenberg Citation1994). Gymnocephalus cernua is a successful invader also due to its remarkable reproductive potential (e.g. Kováč Citation1998; Ogle Citation1998), with relatively early sexual maturity (first year of life) and high egg production (up to 200,000 eggs in the first batch). The species’ invasiveness is also a result of its ability to cope with a wide range of environmental conditions including salinity, temperature, turbidity and eutrophication (Rösch et al. Citation1996; Souchon & Tissot Citation2012). The species’ opportunistic feeding behaviour, which includes the ability to feed at night or in highly turbid waters, gives it an inter-specific competitive advantage (Janssen Citation1997; Schleuter & Eckmann Citation2006). Also, several studies have suggested that G. cernua may consume fish eggs in both its native and non-native range (e.g. Adams & Tippett Citation1991; Hölker & Thiel Citation1998; Ogle Citation1998; Selgeby Citation1998) as well as prey upon young-of-the-year and small fishes (Kozlova & Panasenko Citation1977; Hölker & Thiel Citation1998; Popova et al. Citation1998). Globally, G. cernua has been reported to be the second most costly (i.e. US$53 billion) invasive species with remarkable impacts on the abundance of economically valuable fish species (Cuthbert et al. Citation2021).
Preliminary risk screening of G. cernua using the Freshwater Fish Invasiveness Screening Kit (FISK: Copp et al. Citation2009) showed this species to pose a high risk of invasiveness in the Balkans region, the Iberian Peninsula, and Scotland (Vilizzi et al. Citation2019). Using the FISK’s decision-support tool improvement represented by the Aquatic Species Invasiveness Screening Kit (AS-ISK: Copp et al. Citation2016; Vilizzi et al. Citation2021), G. cernua attracted a medium-risk score of being invasive for Turkey (Tarkan et al. Citation2017b), but a high-risk score for the risk assessment areas represented by the River Neretva catchment in Bosnia-Herzegovina and Croatia (Glamuzina et al. Citation2017), Great Britain (Dodd et al. Citation2019), and Croatia and Slovenia (Radočaj et al. Citation2021). Of note, the risk screening for Turkey was made in the event that G. cernua would be introduced into the country, where the presence of the species had not yet been confirmed (see Çiçek et al. Citation2021).
The objective of this study was to identify the populations of G. cernua so far recorded from the Thrace region of Turkey by a molecular approach based on mtDNA barcode sequence analysis coupled with a risk screen and follow-up assessment. It was hypothesised that these populations either originated from a neighbouring geographical range, hence suggesting natural dispersal, or belong to a geographically remote lineage – an indicator of either intentional or unintentional introduction. Based on the premise that Thrace acts as a primary route for non-native freshwater fish introductions into Anatolia, it was predicted that the introduction of G. cernua into this region would be highly likely. For this reason, the invasiveness potential of this species in Thrace and Anatolia was evaluated through a risk screen followed by a risk assessment of its potential impacts. The management implications of the findings are discussed especially in view of the potentially rapid proliferation and possible related impacts of G. cernua on the native ecosystems of the region. Notably, this study exemplifies the use of an integrative and comprehensive (cf. holistic) approach to address the origin, distribution and potential impact of non-native species by complementing a DNA meta-barcoding method with risk analysis.
2. Materials & methods
Specimens of G. cernua were collected from a wetland area in the delta of the River Maritza consisting of the three interconnected shallow lakes Gala, Pamuklu and Sığırcı (40.78°E 26.28°N, 40.77°E 26.20°N and 40.85°E 26.32°N, respectively). Fish were caught with the help of fishers using gill nets of various mesh size (8–70 mm). After capture, all specimens were euthanised with an overdose of 2-phenoxyethanol, stored in ice or directly fixed in 99% ethanol, and transported to the laboratory for later processing.
2.1. Molecular analysis
Total DNA of G. cernua specimens was extracted from alcohol-fixed fin tissues according to the DNeasy Blood & Tissue Kit protocol (Qiagen, Hilden, Germany). The vertebrate mitochondrial DNA barcode region of the cytochrome c oxidase subunit I (COI) gene was amplified using forward primer FishF1 5’-TCAACCAACCACAAAGACATTGGCAC-3’ and reverse primer FishR1 5’- TAGACTTCTGGGTGGCCAAAGAATCA-3’ (Ward et al. Citation2005). Polymerase chain reaction (PCR) was carried out as 50 μL total volume containing 5 μl of 10× reaction buffer, 5 μl MgCl2 (25 mM), 6 μl dNTPmix (10 mM), 1 μl forward primer (10 pmol), 1 μl reverse primer (10 pmol), 0.2 μl Taq DNA polymerase (1 U), 2 μl of DNA template (50 ng/μl), and 29.8 μl sterilised pure water. The PCR was performed using a gradient thermal cycler Biorad T100TM (Bio-Rad, Hercules), with thermal cycling conditions as follows: one cycle at 95°C for 3 min followed by denaturation with 35 cycles at 95°C for 45 seconds, annealing at 60°C for 30 seconds, extension at 72°C for one minute, and one cycle at 72°C for five minutes. The PCR products were visualised on a UV illuminator system after electrophoresis in 1.2% agarose gel containing ethidium bromide (0.5 mg/mL) in Tris-Acetate-EDTA buffer and then purified using the QIAquick PCR Purification Kit (Qiagen) according to manufacturer’s instructions. Bidirectional sequencing of the PCR products was performed with an ABI PRISM 3730 × 1 Genetic Analyser (Applied Biosystem; www.appliedbiosystems.com) using a BigDye Terminator 3.1 cycle sequencing ready reaction kit (Applied Biosystem) at Macrogen Europe (www.macrogen.com).
Mitochondrial COI barcode sequences of G. cernua were analysed via Basic Local Alignment Search Tool searches at the National Center for Biotechnology Information (https://www.ncbi.nlm.nih.gov/) and BOLDSYSTEMS Identification (https://www.boldsystems.org/index.php/IDS_OpenIdEngine) websites. The sequences were aligned with the Clustal W method (Thompson et al. Citation1994) implemented in Bioedit 7.2.5 (Hall Citation1999). Sequences were then trimmed down to 606 bp and converted to Fasta and Nexus formatted files. All positions with less than 98% site coverage were eliminated (i.e. fewer than 2% alignment gaps), and missing data and ambiguous bases were allowed at any position (cf. partial deletion option). Haplotypes were defined using DnaSP v5 (Librado & Rozas Citation2009). Mean genetic distances were calculated using the Kimura 2-parameter (K2P: Kimura Citation1980) distance model in MEGA X software (Kumar et al. Citation2018). Phylogenetic relationships among Gymnocephalus species/populations were estimated using neighbour-joining (NJ) and maximum likelihood (ML) algorithms in MEGA X software. The NJ tree was generated according to the K2P model that provided the best-fit evolution model for nucleotide substitution. The ML tree was generated according to the HKY+G model (Hasegawa et al. Citation1985), which is the most appropriate evolution model selected with the lowest Akaike Information Criterion (AIC) score in jModeltest 0.1.1 (Posada Citation2008). The NJ and ML trees were generated using 1000 bootstrap replicates to explore phylogenetic affinities of the mitochondrial lineages.
For phylogenetic analysis, five newly generated DNA barcodes of G. cernua from the Turkish Thrace region were used in addition to 31 already published sequences from the NCBI GenBank (). DNA barcodes of one individual each of Danube ruffe Gymnocephalus baloni, Gymnocephalus ambriaelacus and schraetzer (striped ruffe) Gymnocephalus schraetser (GenBank accession numbers: HM902262, KM286679, HM902260, respectively) were also included along with that of pike-perch Sander lucioperca (GenBank accession number: HQ960460) as an outgroup. The haplotype network was constructed with the Median-Joining network algorithm (Bandelt et al. Citation1999) implemented in PopART version 1.7.2 (Leigh & Bryant Citation2015). The COI barcode region sequenced from the five specimens of G. cernua and the corresponding sequences were deposited into GenBank accession numbers MW857189–MW857193.
Table I. Origin (country and location), GenBank or BOLD accession numbers and haplotype of the Gymnocephalus specimens analysed for molecular data
2.2. Risk analysis
2.2.1. Risk screening
The AS-ISK decision-support tool was used to quantify the risk of invasiveness of G. cernua in both Thrace and Anatolia (hereafter, also referred to as the RA areas), with screenings carried out independently by AST and BY, hence resulting in four screenings in total. The AS-ISK is fully compliant with the “minimum standards” (Roy et al. Citation2018) for the assessment of non-native species according to the European Commission Regulation on the prevention and management of the introduction and spread of invasive alien species and has been used successfully to screen with a high level of accuracy potentially invasive non-native aquatic organisms in several RA areas worldwide (see Vilizzi et al. Citation2021).
The AS-ISK screening protocol consists of 55 questions (Copp et al. Citation2016). The first 49 questions comprise the Basic Risk Assessment (BRA) and are concerned with the biogeographical and biological aspects of the species being screened. The last six questions address the Climate Change Assessment (CCA) and require the assessor to evaluate how future predicted climate conditions are likely to affect the BRA with respect to risks of introduction, establishment, dispersal and impact. To achieve a valid screening, for each question the assessor must provide a response, a level of confidence in the response, and a justification. Upon completion of the screening, the species receives both a BRA score and a BRA+CCA (composite) score (ranging from −20 to 68 and from −32 to 80, respectively). Scores <1 suggest that the species is unlikely to become invasive and is therefore classified as “low risk” (Pheloung et al. Citation1999). Higher scores classify the species as posing either a “medium risk” or a “high risk” of becoming invasive. Distinction between medium-risk and high-risk levels depends upon setting a “threshold” value, which in this study was based on the calibrated BRA score = 27.75 for Turkey (Tarkan et al. Citation2017b) – noting that no calibrated BRA+CCA score was provided in that study. The ranked levels of confidence (1 = low; 2 = medium; 3 = high; 4 = very high) associated with each question-related response in the AS-ISK mirror the confidence rankings recommended by the International Programme on Climate Change (IPCC Citation2005). Based on the confidence level (CL) allocated to each response, the CLTotal (i.e. all 55 questions), CLBRA and CLCCA are computed.
2.2.2. Environmental impact classification
Recently, the International Union for Conservation of Nature (IUCN) Species Survival Commission in conjunction with the Invasive Species Specialist Group described a classification system known as Environmental Impact Classification for Alien Taxa (EICAT: www.iucn.org/theme/species/our-work/invasive-species/eicat). The EICAT was developed for classifying non-native species in terms of magnitude of their detrimental impact on the environment in recipient areas (Blackburn et al. Citation2014). Implementation of the EICAT has been described in detail and framed into a guideline (Hawkins et al. Citation2015), and ultimately adapted as an IUCN’s impact scheme standard for the classification of non-native species impacts on the environment (IUCN Citation2020). The EICAT is based on the Generic Impact Scoring System of Nentwig et al. (Citation2010).
Using five semi-quantitative categories (i.e. Minimal, Minor, Moderate, Major, Massive), the EICAT ranks the magnitude of a species’ environmental impacts and classifies such impacts from Minimal (species unlikely to cause deleterious impacts on the native biota or abiotic environment) to Massive (species likely to lead to the replacement and local extinction of native species and to produce irreversible changes in the structure of communities and the abiotic/biotic composition of ecosystems). This method also uses 12 classes of impact mechanisms (i.e. competition, predation, hybridisation, transmission of diseases, parasitism, poisoning/toxicity, biofouling, grazing/herbivory/browsing, chemical, physical, and structural impact on ecosystem and interaction with other alien species) associated with a confidence level allocated as follows: high, medium and low (Blackburn et al. Citation2014). Species are assigned to an impact category based on the largest impact value recorded, whereas the overall confidence is taken from the impact category to which the highest confidence rank is attributed (Blackburn et al. Citation2014). In the case of G. cernua, although the impacts of its invasive populations have been well documented throughout its non-native range, there has been no previous attempt to propose an EICAT categorisation for this species. In this study, an EICAT-based risk categorisation was therefore proposed for G. cernua in Europe and North America, where its non-native populations are well established. The EICAT-based assessment followed the risk screening process to evaluate more comprehensively the potentially adverse impacts of G. cernua.
3. Results
In total, 10 haplotypes were identified from the 36 specimens of G. cernua examined (). Phylogenetic and network trees indicated the existence of two haplogroups with a mean genetic distance of 2.03% (). Populations of G. cernua from the Thrace region were nested within Haplogroup 1, which is widespread in central Europe (mainly, Germany and Czechia), with a similarity ratio >99%; whereas populations from Russia and China were nested within Haplogroup 2 (). Molecular data also suggest that non-native G. cernua in Lake Superior (USA) belongs to Haplogroup 1.
Figure 1. Neighbour-Joining (NJ) tree based on cytochrome c oxidase subunit I (COI) barcode gene sequences of ruffe Gymnocephalus cernua, Danube ruffe Gymnocephalus baloni, Gymnocephalus ambriaelacus and schraetzer (striped ruffe) Gymnocephalus schraetser, plus pike-perch Sander lucioperca as an outgroup. Bootstrap values (maximum likelihood-NJ) are shown above nodes if 50% or higher in value.
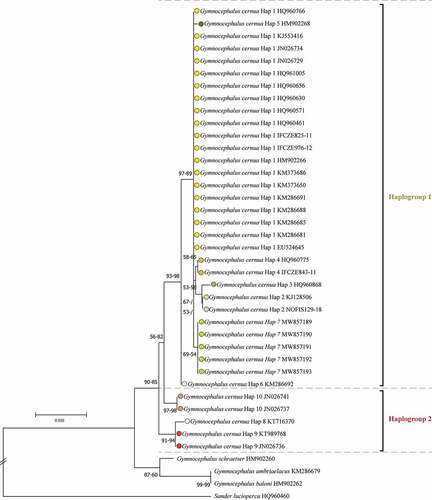
Based on the calibrated threshold of 27.75, the BRA score for G. cernua fell within the high-risk category for Anatolia (28.5) and especially for Thrace (36.0) (). After accounting for the potential effects of climate change, the BRA+CCA score for Anatolia decreased to 21.5, but it did not change for Thrace, hence reflecting a higher risk for the species being invasive in this RA area under predicted climate change conditions (). The factors and traits that contributed to the increase in the BRA score included a history of being invasive elsewhere, high climatic matching, parental care, relatively small size at maturity, opportunistic feeding behaviour, and an elevated likelihood of being illegally stocked; those that reduced the overall score included no likelihood of hybridisation with native species and low risk posed to native threatened or protected taxa. The mean CL for the responses to the questions contributing to the BRA, CCA and BRA+CCA were: CLBRA+CCA = 2.45 ± 0.14 SE, CLBRA = 2.51 ± 0.14 and CLCCA = 1.92 ± 0.08, for G. cernua in Thrace; CLBRA+CCA = 2.44 ± 0.16 SE, CLBRA = 2.52 ± 0.15 and CLCCA = 1.75 ± 0.25, for G. cernua in Anatolia. These values indicated medium-to-high confidence for the BRA but low-to-medium confidence for the CCA.
Table II. Scoring output (mean values from two assessors) from the Aquatic Species Invasiveness Screening Kit for ruffe Gymnocephalus cernua in the risk assessment areas of Thrace and Anatolia (Turkey)
For both Europe and North America, the EICAT impact assessments resulted in “Major impacts”, with a high and medium confidence level, respectively (). The paucity of information on the impacts of introduced populations in North America was a major contributor to the lower confidence ranking. Based on available information, competition and predation received the highest impact score for Europe, whereas for North America this was only true for predation.
Table III. Outcomes of the environmental impact classification of Alien Taxa (EICAT: Blackburn et al. Citation2014) applied to G. cernua in its introduced ranges of Europe and North America
4. Discussion
The phylogenetic analysis of this study has supported the hypothesis that G. cernua may have entered Turkey through its invasive range in Europe. This was indicated by the haplotype identified for the G. cernua populations in the Turkish region of Thrace, which is quite similar to the haplotype for central European populations. Molecular data also showed that non-native G. cernua in Lake Superior (USA) shared the same haplotype (Hap 1) with the one widespread in Germany and Czechia. Based on these results and given the discordance between haplotype similarity and geographic distance in the available dataset, the most likely explanation for the non-native dispersion of G. cernua within Turkey’s Thrace appears to be natural spread through connected river systems (i.e. River Maritza). It is therefore unlikely that G. cernua was introduced by anglers or local fishers, as in the case of some other commonly translocated freshwater fishes in Turkish waters (e.g. perch Perca fluviatilis), because of the species’ relatively small size and likely damage to fishing nets (Edirne İpsala, Yenikarpuzlu Fishing Cooperative in Lake Gala: pers. comm.). Clearly, the current findings for the species’ partial invasion history would be supported by further research based on more DNA sequences being made available from the Balkan region.
Literature information on G. cernua was overall more widely available for the species’ non-native range in Europe (https://www.cabi.org/isc/datasheet/80729), where it has proven to be a powerful invader (Souchon & Tissot Citation2012). However, there have been only a few screening studies evaluating the species’ risk of invasiveness at the national (Tarkan et al. Citation2017b; Vilizzi et al. Citation2019), regional (e.g. river or lake basin: Glamuzina et al. Citation2017; Tarkan et al. Citation2017a) or climo-geographic level (Dodd et al. Citation2019), hence regardless of the species’ status as non-native or translocated. Also, and of note, the calibrated BRA threshold of 27.75 for Turkey (Tarkan et al. Citation2017b) used in the present study was based on a large sample of freshwater fish species (n = 64) screened for their invasiveness. This makes the threshold statistically highly accurate and robust even after accounting for the later confirmed record of G. cernua in Turkey (i.e. a re-screen of the entire dataset after accounting for the presence of G. cernua would not have changed the species’ outcome score in such a way to modify the threshold values). In the above risk screenings, the most influential factor for the invasiveness success of G. cernua was climate suitability, and especially the temperature regimes affecting the species’ successful dispersal. Although the BRA score in this study indicated a high risk of invasiveness in both risk assessment areas of Turkey (i.e. Thrace and Anatolia), when taken together with the CCA, G. cernua was classified as carrying a high risk of invasiveness only for Thrace, whereas for Anatolia, its risk level dropped to medium (). The reason for this discrepancy is that, despite the species’ wide range of temperature tolerances (i.e. Köppen-Geiger climates Cf, Cs, Cw, Df, Ds, Dw: CABI, 2021), the Anatolian part of Turkey has a high variety of climatic regimes (i.e. including the arid types Bskand Bsh), whereas Thrace has a more homogeneous climate that provides suitable temperatures for the successful establishment and dispersal of G. cernua (Öztürk et al. Citation2017). Collectively, Thrace and Anatolia are very large geographic areas, and especially the latter should be evaluated with caution given its several climate types. In this regard, proper basin-level assessments (e.g. Dodd et al. Citation2019; Yoğurtçuoğlu et al. Citation2021) could provide for a better evaluation of the level of risk of this species throughout this RA area. This was clearly reflected in the relatively higher confidence levels for the BRA compared to the CCA, as future climate change scenarios predicting an overall increase in temperature (Collins et al. Citation2013) will likely not facilitate the dispersal and establishment of G. cernua in Anatolia.
Both risk screenings and impact assessments for the two RA areas highlighted some knowledge gaps in the environmental biology and impact mechanisms of G. cernua. These gaps appeared to be slightly more pronounced for the populations of North America (). However, available literature data for the species clearly indicated its potential for invasiveness due to its high reproductive capacity with early maturity (e.g. Kováč Citation1998; Ogle Citation1998), tolerance of a wide range of environmental conditions (Rösch et al. Citation1996), and impacts mainly as a result of its opportunistic feeding behaviour (Schleuter & Eckmann Citation2006), with predation on fish eggs (Adams & Tippett Citation1991) and small fishes (Popova et al. Citation1998). Among these impact mechanisms by G. cernua reported for Europe and North America, predation on benthic species, insects and macroinvertebrates (e.g. Kováč Citation1998) could reduce the rich native biodiversity of both Thrace and Anatolia. This reduction could be more severe with high densities of G. cernua, particularly when predating on young age groups of native organisms (e.g. Hölker & Thiel Citation1998). Also, competition for food and behavioural interference may contribute to the decrease in the abundance of native fish (Bergman Citation1991). More importantly, endemic species that are already endangered may be further threatened by G. cernua as a result of its opportunistic feeding behaviour. Considering the rich and unique ichthyofauna of Anatolia, the potential introduction of G. cernua should therefore be monitored with great caution. On the other hand, this species is known to co-exist not only with native species but also with some other non-native species (i.e. Sander lucioperca, Perca fluviatilis, Lepomis gibbosus, Carassius gibelio and Pseudorasbora parva) as observed in water bodies of Thrace where G. cernua has established populations (Çiçek et al. Citation2021). Yet, it is unclear how G. cernua may interact with these non-native fishes, which are also commonly found in Anatolian water bodies (Tarkan et al. Citation2015). Observations and anecdotal evidence from fishers in Lake Gala revealed that Perca fluviatilis outnumbered G. cernua, though further investigation is required on whether the co-occurrence of these non-native fish species in the same habitat would increase their likelihood of survival, hence ecological impacts and related magnitude – a phenomenon known as “invasion meltdown” (Montgomery et al. Citation2012).
In conclusion, the present findings based on coupling molecular and risk analysis represent a major step forward in understanding the origin, distribution and potential impacts of G. cernua in Turkey. Yet, further research is needed to better understand the species’ potential invasiveness and promote adequate management plans. In this respect, it is unknown whether predation pressure by native species would be efficient enough to control the abundance of G. cernua. Thus, contradictory results have been reported in which predation was unable to prevent the increase in the abundance of this species (Mayo et al. Citation1998), most probably due to selective predation by native species and their avoidance of G. cernua. Regardless, physical control by overfishing can be a partially effective option when employed on a regular basis, even though intense trawling on G. cernua in Lake Vortsjarv (southern Estonia) was reported to fail in contributing to the decrease in the abundance of this species (Pihu & Maemets Citation1982). This was attributed to the species’ adaptive capacity to compensate mortality by rapid growth, early maturity and multiple spawning with high fecundity (Kováč Citation1998; Ogle Citation1998). Overall, the best management option for G. cernua, at least for Anatolia where the species has not yet been reported, would be by prevention or improvement of early detection mechanisms. These would consist of comprehensive and detailed background information such as habitat vulnerability and inter-connectivity, propagule pressure as well as impacts and biology of G. cernua (Hill & Sowards Citation2015). Increased public awareness of the detrimental impacts of G. cernua would further contribute to supportive policy.
Disclosure statement
No potential conflict of interest was reported by the author(s).
References
- Adams CE, Tippett R. 1991. Powan, Coregonus lavaretus (L.), ova predation by newly introduced ruffe, Gymnocephalus cernuus (L.), in Loch Lomond, Scotland. Aquaculture Research 22(2):239–246. DOI: 10.1111/j.1365-2109.1991.tb00513.x.
- April J, Mayden RL, Hanner RH, Bernatchez L. 2011. Genetic calibration of species diversity among North America’s freshwater fishes. Proceedings of the National Academy of Sciences 108(26):10602–10607. DOI: 10.1073/pnas.1016437108.
- Arab IY, Arab A, Kara HM. 2020. Range expansion of the ruffe (Gymnocephalus cernuus) to the southern Mediterranean: First record in Koudiat Medouar reservoir, Algeria. Journal of Applied Ichthyology 36(5):705–708. DOI: 10.1111/jai.14070.
- Baltazar‐Soares M, Blanchet S, Cote J. 2020. Genomic footprints of a biological invasion: Introduction from Asia and dispersal in Europe of the topmouth gudgeon (Pseudorasbora parva). Molecular Ecology 29(1):71–85. DOI: 10.1111/mec.15313.
- Bandelt HJ, Forster P, Röhl A. 1999. Median-joining networks for inferring intraspecific phylogenies. Molecular Biology and Evolution 16(1):37–48. DOI: 10.1093/oxfordjournals.molbev.a026036.
- Bergman E. 1991. Changes in abundance of two percids, Perca fluviatilis and Gymnocephalus cernuus, along a productivity gradient: Relations to feeding strategies and competitive abilities. Canadian Journal of Fisheries and Aquatic Sciences 48:536–545. DOI: 10.1139/f91-068.
- Bergman E, Greenberg LA. 1994. Competition between a planktivore, a benthivore, and a species with ontogenetic diet shifts. Ecology 75(5):1233–1245. DOI: 10.2307/1937449.
- Blackburn TM, Essl F, Evans T, Hulme PE, Jeschke JM, Kühn I, Kumschick S, Marková Z, Marková Z, Nentwig W, Pergl J, Pyšek P, Rabitsch W, Ricciardi A, Richardson DM, Sendek A, Vilà M, Wilson JRU, Winter M, Genovesi P, Bacher S. 2014. A unified classification of alien species based on the magnitude of their environmental impacts. PLoS Biology 12(5):article e1001850. DOI: 10.1371/journal.pbio.1001850.
- Brabrand A. 2016. University of Oslo, Natural History Museum, BOLD Systems. Available: http://boldsystems.org/index.php/Public_RecordView?processid=NOFIS129-18.
- Chiara G. 1986. First record of Acerina (Gymnocephalus) cernua L. (Osteichthyes Perciformes) in Italy. In: Quaderni Ente Tutela PAtrimonio Ittico, Udine, Italy. pp. 59–60.
- Çiçek E, Emiroğlu Ö, Seçer B, Aksu S, Sungur S, Başkurt S, Bahçeci H. 2021. Confirmed record of Gymnocephalus cernua (Linnaeus, 1758) (Perciformes: Percidae) as a new exotic species for Turkey. Acta Biologica Turcica 34:46–53.
- Collins M, Knutti R, Arblaster J, Dufresne JL, Fichefet T, Friedlingstein P, Shongwe M. 2013. Long-term climate change: Projections, commitments and irreversibility. In: Stocker TF, Qin D, Plattner G-K, Tignor M, Allen SK, Boschung J, Nauels A, Xia Y, Bex V, Midgley PM editors, Climate Change 2013: The Physical Science Basis. Contribution of Working Group I to the Fifth Assessment Report of the Intergovernmental Panel on Climate Change. Cambridge, United Kingdom and New York, NY: Cambridge University Press. pp. 1029–1136.
- Copp GH, Garthwaite R, Gozlan RE. 2005. Risk identification and assessment of non-native freshwater fishes: Concepts and perspectives on protocols for the UK. Cefas Science Technical Report. Lowestoft, UK: Cefas. Available: www.cefas.co.uk/publications/techrep/tech129.pdf.
- Copp GH, Vilizzi L, Mumford J, Fenwick GV, Godard MJ, Gozlan RE. 2009. Calibration of FISK, an invasiveness screening tool for non-native freshwater fishes. Risk Analysis 29(3):457–467. DOI: 10.1111/j.1539-6924.2008.01159.x.
- Copp GH, Vilizzi L, Tidbury H, Stebbing PD, Tarkan AS, Moissec L, Goulletquer P. 2016. Development of a generic decision-support tool for identifying potentially invasive aquatic taxa: AS-ISK. Management of Biological Invasions 7(4):343–350. DOI: 10.3391/mbi.2016.7.4.04.
- Copp GH, et al. 2021. Environmental Modelling & Software, 135 article 104900. https://doi.org/10.1016/j.envsoft.2020.104900
- Cristescu ME. 2015. Genetic reconstructions of invasion history. Molecular Ecology 24(9):2212–2225. DOI: 10.1111/mec.13117.
- Cuthbert RN, Pattison Z, Taylor NG, Verbrugge L, Diagne C, Ahmed DA, Leroy B, Angulo E, Briski E, Capinha C, Catford CA, Dalu T, Essl F, Gozlan RE, Haubrock PJ, Kourantidou M, Kramer AM, Renault D, Wasserman RJ, Courchamp F. 2021. Global economic costs of aquatic invasive alien species. Science of the Total Environment 775:article 145238. DOI: 10.1016/j.scitotenv.2021.145238.
- Dodd JA, Vilizzi L, Bean CW, Davison PI, Copp GH. 2019. At what spatial scale should risk screenings of translocated freshwater fishes be undertaken—river basin district or climo-geographic designation? Biological Conservation 230:122–130. DOI: 10.1016/j.biocon.2018.12.002.
- Dulčić J, Glamuzina B, Tutman P. 2005. First record of ruffe Gymnocephalus cernuus (Percidae) in the Hutovo Blato wetland, Adriatic drainage system of Bosnia And Herzegovina. Cybium 29:205–206.
- Estoup A, Guillemaud T. 2010. Reconstructing routes of invasion using genetic data: Why, how and so what? Molecular Ecology 19(19):4113–4130. DOI: 10.1111/j.1365-294X.2010.04773.x.
- Glamuzina B, Tutman P, Nikolić V, Vidović Z, Pavličević J, Vilizzi L, Copp GH, Simonović P. 2017. Comparison of taxon-specific and taxon-generic risk screening tools for identifying potentially invasive non-native fishes in the River Neretva catchment (Bosnia & Herzegovina and Croatia). River Research and Applications 33(5):670–679. DOI: 10.1002/rra.3124.
- Halacka K, Vetesnik L, Sanda R. 2010. National Museum of natural history, Prague. GenBank. Available: https://www.ncbi.nlm.nih.gov/nuccore/HQ961005.
- Halacka K, Vetesnik L, Sanda R. 2011. National Museum of natural history. Prague: BOLD Systems. Available: http://boldsystems.org/index.php/Public_RecordView?processid=IFCZE976-12.
- Hall TA. 1999. BioEdit: A user–friendly biological sequence alignment editor and analysis program for Windows 95/98/NT. Nucleic Acids Symposium Series 41:95–98.
- Hasegawa M, Kishino H, Yano T. 1985. Dating the human-ape splitting by a molecular clock of mitochondrial DNA. Journal of Molecular Evolution 22(2):160–174. DOI: 10.1007/BF02101694.
- Hawkins CL, Bacher S, Essl F, Hulme PE, Jeschke JM, Kühn I, Kumschick S, Nentwig W, Pergl J, Pyšek P, Rabitsch W, Richardson DM, Vilà M, Wilson JRU, Genovesi P, Blackburn TM. 2015. Framework and guidelines for implementing the proposed IUCN Environmental Impact Classification for Alien Taxa (EICAT). Diversity and Distributions 21(11):1360–1363. DOI: 10.1111/ddi.12379.
- Hill J, Sowards J. 2015. Successful eradication of the non-native loricariid catfish Pterygoplichthys disjunctivus from the Rainbow River, Florida. Management of Biological Invasions 6(3):311–317. DOI: 10.3391/mbi.2015.6.3.11.
- Hill JE, Hardin S, Lawson KM, Lawson Jr LL, Tuckett QM, Vilizzi L, Watson CA, Copp GH. 2020. Comparing apples to oranges and other misrepresentations of the Invasiveness Screening Kit family of risk screens: A rebuttal of Marcot et al. (2019). Management of Biological Invasions 11(2):325–341. DOI: 10.3391/mbi.2020.11.2.10.
- Hölker F, Thiel R. 1998. Biology of Ruffe (Gymnocephalus cernuus (L.))-A review of selected aspects from European literature. Journal of Great Lakes Research 24(2):186–204. DOI: 10.1016/S0380-1330(98)70812-3.
- Hubert N, Hanner R, Holm E, Mandrak NE, Taylor E, Burridge M, Watkinson D, Dumont P, Curry A, Bentzen P, Zhang J, April J, Bernatchez L. 2008. Identifying Canadian freshwater fishes through DNA barcodes. PLOS ONE 3(6):article e2490. DOI: 10.1371/journal.pone.0002490.
- IPCC. 2005. Guidance notes for lead authors of the IPCC fourth assessment report on addressing uncertainties. Intergovernmental Panel on Climate Change, WMO & UNEP. Available: www.ipcc.ch/pdf/assessment-report/ar4/wg1/ar4-uncertaintyguidancenote.pdf.
- IUCN. 2020. IUCN EICAT categories and criteria. The Environmental Impact Classification for Alien Taxa (EICAT). First ed. Gland, Switzerland and Cambridge, UK: IUCN.
- Janssen J. 1997. Comparison of response distance to prey via the lateral line in the ruffe and yellow perch. Journal of Fish Biology 51(5):921–930. DOI: 10.1111/j.1095-8649.1997.tb01531.x.
- Karabanov D, Borovikova E. 2016. DNA-barcoding of fishes of Plescheevo Lake (Yaroslavl reg., Russia). GenBank. Available: https://www.ncbi.nlm.nih.gov/nuccore/KT989768.
- Kimura M. 1980. A simple method for estimating evolutionary rates of base substitutions through comparative studies of nucleotide sequences. Journal of Molecular Evolution 16(2):111–120. DOI: 10.1007/BF01731581.
- Knebelsberger T, Dunz AR, Neumann D, Geiger MF. 2015. Molecular diversity of Germany’s freshwater fishes and lampreys assessed by DNA barcoding. Molecular Ecology Resources 15(3):562–572. DOI: 10.1111/1755-0998.12322.
- Kottelat M, Freyhof J. 2007. Handbook of European freshwater fishes. Vol. 13. Switzerland: Publications Kottelat Cornol.
- Kováč V. 1998. Biology of Eurasian ruffe from Slovakia and adjacent central European countries. Journal of Great Lakes Research 24(2):205–216. DOI: 10.1016/S0380-1330(98)70813-5.
- Kozlova MF, Panasenko VA. 1977. Annual food consumption of bream (Abramis brama) and ruffe (Acerina cernua) populations in the Courland Lagoon (Kurshskiy Zaliv). Journal of Ichthyology 17:382–388.
- Kumar S, Stecher G, Li M, Knyaz C, Tamura K. 2018. MEGA X: Molecular evolutionary genetics analysis across computing platforms. Molecular Biology and Evolution 35(6):1547–1549. DOI: 10.1093/molbev/msy096.
- Kuru M. 2004. Recent systematic status of inland water fishes of Turkey. Journal of Education Faculty of Gazi 24:1–21.
- Leigh JW, Bryant D. 2015. Data from: PopART: Full‐feature software for haplotype network construction. Methods in Ecology and Evolution 6(9):1110–1116. DOI: 10.1111/2041-210X.12410.
- Librado P, Rozas J. 2009. DnaSP v5: A software for comprehensive analysis of DNA polymorphism data. Bioinformatics 25(11):1451–1452. DOI: 10.1093/bioinformatics/btp187.
- Matthey G. 1966. Two species new to the fauna of the lake Geneva: Dreissena polymorpha Pallas (Mollusca, Dreissenidae), Acerina cernua (L) (Pisces, Percidae). Bulletin De La Société Vaudoise De Sciences Naturelles 69:229–232.
- Mayo KR, Selgeby JH, McDonald ME. 1998. A bioenergetic modelling evaluation of top-down control of ruffe in the St. Luise River, western Lake Superior. Journal of Great Lakes Research 24(2):329–342. DOI: 10.1016/S0380-1330(98)70824-X.
- Montgomery I, Lundy M, Neil R. 2012. ‘Invasional meltdown’: Evidence for unexpected consequences and cumulative impacts of multispecies invasions. Biological Invasions 14(6):1111–1125. DOI: 10.1007/s10530-011-0142-4.
- Nentwig W, Kuhnel E, Bacher S. 2010. A generic impact scoring system applied to alien mammals in Europe. Conservation Biology 24(1):302–311. DOI: 10.1111/j.1523-1739.2009.01289.x.
- Ogle DH. 1998. A synopsis of the biology and life history of ruffe. Journal of Great Lakes Research 24(2):170–185. DOI: 10.1016/S0380-1330(98)70811-1.
- Öztürk MZ, Çetinkaya G, Aydın S. 2017. Köppen-Geiger iklim sınıflandırmasına göre Türkiye’nin iklim tipleri [Climate types of Turkey according to Köppen-Geiger climate classification]. Istanbul University Journal of Geography 35:17–27. [In Turkish ith English abstract.
- Petriki O, Naziridis T, Apostolou A, Koutrakis E, Bobori DC. 2014. The spread of the introduced Gymnocephalus cernua Linnaeus, 1758 (Perciformes: Percidae) along the transboundary Strymonas (Struma) River basin: First report in Kerkini Dam Lake (Greece). Acta Zoologica Bulgarica 66:563–566.
- Pheloung PC, Williams PA, Halloy SR. 1999. A weed risk assessment model for use as a biosecurity tool evaluating plant introductions. Journal of Environmental Management 57(4):239–251. DOI: 10.1006/jema.1999.0297.
- Pihu ER, Maemets A. 1982. The management of fisheries in Lake Vortsjarv. Hydrobiologia 86(1–2):207–210. DOI: 10.1007/BF00005812.
- Popova OA, Reshetnikov YS, Kiyashko VI, Dgebuadze YY, Mikheev VN. 1998. Ruffe from the former USSR: Variability within the largest part of its natural range. Journal of Great Lakes Research 24(2):263–284. DOI: 10.1016/S0380-1330(98)70818-4.
- Posada D. 2008. jModelTest: Phylogenetic model averaging. Molecular Biology and Evolution 25(7):1253–1256. DOI: 10.1093/molbev/msn083.
- Pratt D. 1988. Distribution and population status of the ruffe (Gymnocephalus cernua) in the St. Louis estuary and Lake Superior. Great Lakes Fishery Commission, Research Completion Report. 12 pp.
- Radočaj T, Špelić I, Vilizzi L, Povž M, Piria M. 2021. Identifying threats from introduced and translocated non-native freshwater fishes in Croatia and Slovenia under current and future climatic conditions. Global Ecology and Conservation 27:article e01520. DOI: 10.1016/j.gecco.2021.e01520.
- Rösch R, Kangur A, Kangur K, Krämer A, Ráb P. 1996. Ruffe (Gymnocephalus cernuus). Annales Zoologici Fennici, Helsinki: Suomen Biologian Seura Vanamo 33:305–308.
- Roy HE, et al. 2018. Developing a framework of minimum standards for the risk assessment of alien species. Journal of Applied Ecology 55(2):526–538. DOI: 10.1111/1365-2664.13025.
- Savino JF, Kolar CS. 1996. Competition between nonindigenous ruffe and native yellow perch (Perca flavescens) in laboratory studies. Transactions of the American Fisheries Society 125(4):562–571. DOI: 10.1577/1548-8659(1996)125<0562:CBNRAN>2.3.CO;2.
- Schleuter D, Eckmann R. 2006. Competition between perch (Perca fluviatilis) and ruffe (Gymnocephalus cernuus): The advantage of turning night into day. Freshwater Biology 51(2):287–297. DOI: 10.1111/j.1365-2427.2005.01495.x.
- Selgeby J. 1998. Predation by ruffe (Gymnocephalus cernuus) on fish eggs in Lake Superior. Journal of Great Lakes Research 24(2):304–308. DOI: 10.1016/S0380-1330(98)70821-4.
- Souchon Y, Tissot L. 2012. Synthesis of thermal tolerances of the common freshwater fish species in large Western Europe rivers. Knowledge and Management of Aquatic Ecosystems 405:article 03.
- Tarkan AS, Marr SM, Ekmekçi FG. 2015. Non-native and translocated freshwater fish species in Turkey. FISHMED Fishes in Mediterranean Environments 2015(3):28.
- Tarkan AS, Sarı HM, İlhan A, Kurtul I, Vilizzi L. 2017a. Risk screening of nonnative and translocated freshwater fish species in a Mediterranean-type shallow lake: Lake Marmara (West Anatolia). Zoology in the Middle East 63(1):48–57. DOI: 10.1080/09397140.2017.1269398.
- Tarkan AS, Vilizzi L, Top N, Ekmekçi FG, Stebbing PD, Copp GH. 2017b. Identification of potentially invasive freshwater fishes, including translocated species, in Turkey using the Aquatic Species Invasiveness Screening Kit (AS-ISK). International Review of Hydrobiology 102(1–2):47–56. DOI: 10.1002/iroh.201601877.
- Thompson JD, Higgins DG, Gibson TJ. 1994. CLUSTAL W: Improving the sensitivity of progressive multiple sequence alignment through sequence weighting, position-specific gap penalties and weight matrix choice. Nucleic Acids Research 22(22):4673–4680. DOI: 10.1093/nar/22.22.4673.
- Vilizzi L, et al. 2019. A global review and meta-analysis of applications of the freshwater Fish Invasiveness Screening Kit. Reviews in Fish Biology and Fisheries 29(3):529–568. DOI: 10.1007/s11160-019-09562-2.
- Vilizzi L, et al. 2021. A global-scale screening of non-native aquatic organisms to identify potentially invasive species under current and future climate conditions. Science of the Total Environment 788:article 147868. DOI: 10.1016/j.scitotenv.2021.147868.
- Ward RD, Zemlak TS, Innes BH, Last PR, Hebert PDN. 2005. DNA barcoding Australia’s fish species. Philosophical Transactions of the Royal Society B 360(1462):1847–1857. DOI: 10.1098/rstb.2005.1716.
- Winfield IJ, Adams CE, Fletcher JM. 1996. Recent introductions of the ruffe (Gymnocephalus cernuus) to three United Kingdom lakes containing Coregonus species. Annales Zoologici Fennici 33:459–466.
- Yang T, Meng W, Zhang R, Gao T, Cai L, Hai S, Zhou Q. 2016. DNA barcoding of fishes in Irtysh River China. Russian Journal of Genetics 52(9):969–976. DOI: 10.1134/S1022795416090167.
- Yoğurtçuoğlu B, Bucak T, Ekmekçi FG, Kaya C, Tarkan AS. 2021. Mapping the establishment and invasiveness potential of Rainbow trout (Oncorhynchus mykiss) in Turkey: With special emphasis on the conservation of native salmonids. Frontiers in Ecology and Evolution 8:article 599881. DOI: 10.3389/fevo.2020.599881.