Abstract
In late 2019, a novel strain of coronavirus (2019-nCoV) named as severe respiratory syndrome coronavirus 2 (SARS-CoV-2) emerged in Wuhan, a city in China, through some zoonotic reservoir, most probably a bat, and spread throughout the world. There have been around 22,213,869 reported cases of COVID-19 and almost 781,677 deaths worldwide according to the data updated by World Health Organization till 20 August 2020. It transmitted via droplets from an infected person to a healthy person in a very short duration of time. After the completion of the incubation period, which ranges from 2 to 14 days, the person experiences pneumonia-like symptoms such as fever, sore throat, cough, breathlessness, malaise, fatigue, and multi-organ dysfunction, etc. The main receptor of SARS-CoV-2 is angiotensin-converting enzyme 2 which binds with the spike (S) protein of the virus and helps it in attachment and entry to the host cells. COVID-19 is diagnosed by molecular testing of respiratory secretions and CT scan analysis. Because of the absence of any approved treatment options for COVID-19, a number of research studies are being carried out to find out any combination of already approved drugs or new lead compounds using in silico docking and screening strategies.
1. Introduction
Viral diseases have a history of the emergence and causing serious issues to mankind. According to the data of the World Health Organization (WHO), several viral epidemics have occurred in the last twenty years, such as severe acute respiratory syndrome SARS (2002–2003), H1N1 influenza (2009), and the Middle East respiratory syndrome (MERS) (2012). Both SARS and MERS were associated with the viruses of the same family, Coronoviridae (Cascella, Rajnik, Cuomo, Dulebohn, & Di Napoli, Citation2020). Coronavirus majorly targets the respiratory tract. Recently, in late December 2019, a new strain of coronavirus has been reported from the specimens of patients infected with the severe pneumonia-like disease (Bogoch et al., Citation2020). This strain was first provisionally named as 2019 novel coronavirus (2019 n-CoV), but now it is termed as severe acute respiratory distress syndrome coronavirus 2 (SARS-CoV-2) after being declared as a successor of SARS-CoV. COVID-19, viral pneumonia caused by coronavirus belongs to the coronaviridae family Orthocoronavirinae subfamily including α, β, γ, and delta-coronavirus. SARS-CoV-2 has a high value of transmissibility factor Ro which means it can spread very fast from one person to another person. It might have originated from Bats because the genome sequence of SARS-CoV-2 shares 79.5% similarity with SARS-CoV of bats while 96% similarity with SARS-CoV, the causative agent of 2003 coronavirus outbreak. Actually, SARS-CoV has fourteen amino acids that are involved in binding to angiotensin-converting enzyme-2 (ACE2) of human lungs out of these fourteen amino acids, eight are present in SARS-CoV-2 which clearly shows that there is a unique resemblance between these two viruses (Fehr & Perlman, Citation2015). SARS-CoV-2 basically infects the lower respiratory tract by entering the alveolar cells through the ACE2 receptor, replicate here, release mature virions through exocytosis, and ultimately activates the immune system which causes the secretion of cytokines (Jiang et al., Citation2020). Clinical features of COVID-19 include fever, dry cough, diarrhea, myalgia, and vomiting but sometimes it may lead towards severe lung infection, kidney failure, and eventually death. According to the data collected by the WHO, till 20 August 2020, the total confirmed cases and total deaths due to COVID-19 are 22,213,869 and 781,677 respectively . Due to this worst situation and lack of any specific medication, a number of research projects are being carried out worldwide to find a proper treatment for COVID-19. One of the best strategies is in silico drug designing or screening of pharmacologically active compounds that can act as inhibitors of SARS-CoV-2 target proteins. This article covers the major aspects of SARS-CoV-2 structure, pathogenesis, and transmission along with the application of molecular docking strategies for drug designing against SARS-CoV-2 ().
Table 1. Molecular docking to find lead compounds as candidates of antiCOVID-19 drug.
2. Historical background of pandemic
In December 2019, the local hospitals of Wuhan, the capital of Hubei province in China, started reporting the cases of severe pneumonia with unknown etiology (Bogoch et al., Citation2020). Many of the patients were found to be directly associated somehow to the exposure to Huanan wholesale seafood market which was popular for its live animal trading. When these increasing cases attracted attention, the respiratory samples of patients were checked in labs for the etiological investigation of causative agents (Lu, Stratton, & Tang, Citation2020). Although, the surveillance system put this infection under the category of SARS outbreak. On 31 December 2019, this outbreak was streamed in the consideration of the WHO, and the seafood market was banned as a first step to prevent the spread of infection (Lu et al., Citation2020). On 7 January, the causative agent was identified as coronavirus having more than 95% homology with bat coronavirus and more than 70% similarity with SARS-CoV. Hence, it was named as SARS-CoV-2. Samples from the seafood market also tested positive for SARS-CoV-2 due to which it was declared as the site of origin of this novel pandemic. The number of cases keeps on increasing even in the people having no direct contact or exposure to the seafood market which indicated that transmission was occurring person to person. At the time of New Year, the massive migration of people from China fueled the spread of a deadly pandemic worldwide (Cascella et al., Citation2020).
The first case of COVID-19, outside of China, was first reported on 13 January 2020, in Thailand and after a few days in the United States and Europe. On 30 January 2020, WHO declared it as a Global Health Emergency. On 19 February 2020, the first six COVID-19 cases were reported in Iran which kept on increasing day by day with the highest reported death number (226 deaths) on 31 July 2020. On 11 March 2020, COVID-19 was characterized as a pandemic, by WHO, affecting several countries worldwide. Italy is also one of the highly affected countries from this pandemic with cumulative positive cases and mortalities as 255,278 and 35,412, receptively. On 26 March, the total number of positive cases was reported more than 500,000 and till 2 April, the United States had the highest number of cases worldwide followed by Italy and Spain. The total number of COVID-19 cases is still highest in the United States and then in Brazil and India. shows the top ten global regions showing the highest number of cumulative cases of infections and mortality.
3. Structure of SARS-CoV-2
Coronaviruses were placed into family coronaviridae because of the crown-like structure of this virus. This crown-shaped appearance was due to glycoproteins studded envelop which is surrounded by core having matrix proteins in it. In that matrix proteins, there is a single-stranded positive-sense RNA that is linked to other nucleoproteins. The glycoproteins present on the envelope are involved in attachment to host cells (Tyrrell & Myint, Citation1996). Size of SARS-CoV-2 ranges from 60 to 140 nm having 4 common serotypes i.e. NL63, HKU1, OC43, and 229E which are circulating in humans and causing mild infection (Richman, Whitley, & Hayden, Citation2016). The genome of coronavirus is considered as the largest genome, among the known RNA virus, whose G + C content ranges from 32% to 43%. A typical coronavirus genome can contain at least six open reading frames (ORFs). The conserved genes of ORF1ab, envelope, spike, nucleo-capsid, and membrane contain many small ORFs among them. SARS-CoV-2 contains positive sense RNA with two main ORFs i.e. ORF1a and ORF1b which encode genes for certain enzymes responsible for the proper functioning of the central dogma of virus replication cycle. Rest of the genome codes for the structural proteins i.e. spike protein (S-protein), an envelope protein (E-protein), membrane protein (M-protein), and nucleocapsid protein (N-protein) (Mousavizadeh & Ghasemi, Citation2020) as shown in . A 3D model of SARS-CoV-2 has also been developed by assembling the 3D components of coronaviruses already available in the database. These components included S-protein, E-protein, and M-protein by recruiting their structures from protein data bank using PDB code 6CRV, 5 × 29, and 3I6G, respectively (Victoria, Citation2020). shows the illustration of SARD-COV-2 virion (Sohrabi et al., Citation2020).
Figure 3. Structure of SARS-CoV-2. (a) 2D anatomical demonstration of SARS-CoV-2 (Mousavizadeh & Ghasemi, Citation2020). (b) 3D structure of SARS-CoV-2 (Sohrabi et al., Citation2020). (c) Positive sense RNA genome of SARS-CoV-2 with two main ORFs. ORF1a codes for two enzymes (Papain like proteases and 3CL-proteases) responsible for the cleavage of polyproteins to activate them and ORF1b codes for other two enzymes (RNA-dependent RNA polymerase and Helicase) which carry out replication. The remaining portion of RNA codes for structurel proteins i.e., spike (S) protein, envelope (E) protein, membrane (M) protein and nucleocapsid (N) protein (Alanagreh, Alzoughool, & Atoum, Citation2020).
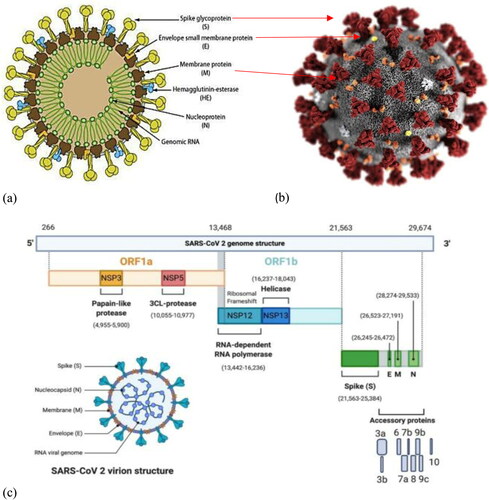
4. Pathogenesis
Pathogenesis of SARS-CoV-2 is still in mystery but it can be correlated with the pathogenesis of SARS-CoV and MERS-CoV. Studies reveal that entry of coronavirus depends on the binding of its S-proteins with suitable receptors (Walls et al., Citation2020) and host proteases which carry out the priming of S protein. Recently, several studies have confirmed that ACE2 acts as the potential receptor of SARS-CoV-2 (Zhou et al., Citation2020) and serine protease TMPRSS2 as a major candidate for S protein priming. SARS-CoV-2 either enters the cell via endocytosis, by packing itself into the endosome, or via plasma membrane fusion, by injecting its genome into the host cell. In the case of endosome mediated entry, ACE2 acts as a receptor and Cathepsin L carries out protein priming by activating S protein. Further, the lowering of pH facilitates the release of the virus genome into the host cell. In the case of plasma membrane fusion entry of genome, TMPRSS2 carries out protein priming. S-protein of coronavirus attaches to the membrane ACE2 which is followed by the S-protein priming through TMPRSS2. This event promotes the fusion of viral and host cell membranes resulting in the entry of the virus in the cell. Then viral and host plasma membrane fusion occurs which is mediated by proteolytic cleavage at S2 (Belouzard, Chu, & Whittaker, Citation2009). The second phenomenon is more suitable for the virus because it is less likely to provoke the host cell immune response. After the virus has entered into the cell cytoplasm it releases the genome into the host cell. Analysis of Coronaviridae genome suggests that most of them encode two vital polyproteins i.e. pp1a and pp1ab which are converted into functional non-structural proteins (NSPs) after cleavage. These NSPs play a major role in regulating the central dogma of the virus during the period of infection. The cleavage of pp1a and pp1ab is the responsibility of two proteases, encoded by open reading frame 1, viral 3-chymotrypsin-like cysteine protease (3Clpro), and papain-like protease (PLpro) respectively as shown in (Thiel et al., Citation2003). This results in the expression of replicase proteins which start the replication of positive-sense RNA viral genome first into a negative-sense strand and then again replicate the negative strand into positive-sense strand (Perlman & Netland, Citation2009). The viral RNA then expresses into structural proteins (S proteins, M proteins, E proteins, and N proteins) and two polyproteins. The structural proteins are translated by ribosomes present on rough endoplasmic reticulum (RER) which are then transported to Golgi complex, whereas nucleoproteins combine with viral RNA to form its genome. The viral particles then assembled into ER Golgi inter compartment, fused with the plasma membrane of the host and released to infect other cells (de Wit, van Doremalen, Falzarano, & Munster, Citation2016) as shown in .
Figure 4. SARS-CoV-2 proteases A SARS-CoV-2 3CL protease (3CL pro) in complex with a novel inhibitor (PDB code 6M2N). B The crystal structure of COVID-19 main protease (Mpro) in complex with an inhibitor N3 (PDB code: 6LU7) (PDB databse).
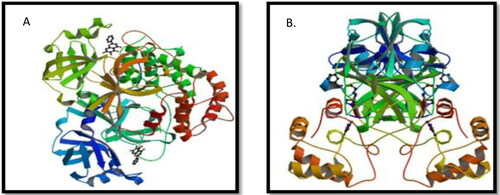
Figure 5. Life cycle of SARS-CoV-2 (Alanagreh et al., Citation2020). SARS-CoV-2, via its spike protein (S-protein), attaches to its receptor ACE2 on the alveolar cell surface and enters the cell either by forming endosome or by injecting its positive sense RNA strand into the host. The ORF1a and ORF1ab of viral genome is translated into pp1a and pp1b polyproteins which on cleavage produce viral replicase protein RdRp. RdRp carry out RNA replication of positive sense RNA strand in such a way that at first it replicate + RNA into a -RNA and then finally into a + RNA strand which is further translatedinto proteins. Viral structural proteins are translated on Rough Endoplasmic Reticulum (RER) and are transported to golgi complex where the assembly of stracturel protein and nucleocapsid takes place. The mature virion is then released out of the cell via exocytosis.
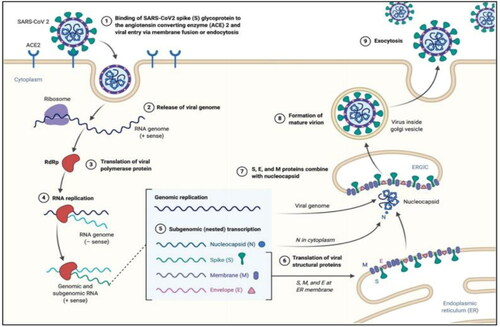
Viral antigens of SARS-CoV-2 are presented by antigen-presenting cells present in the host. The viral antigenic peptides are presented by major histocompatibility complex or human leukocyte antigen which results in the production and release of virus-specific cytotoxic Helper T lymphocytes. MHC1 is mainly involved in the presentation of antigen (Liu et al., Citation2010). Due to this, both types of immunity in humans are activated i.e. humoral and cell-mediated. Due to humoral immunity B-cells produce a large number of IgM and IgG antibodies. IgG antibodies are mainly involved in coping with SARS-CoV-2 and it can bind to S and N proteins. In cellular immunity, it is clearly observed that patients with SARS-CoV infection have a lesser number of CD4 and CD8 T cells. However these memory cells can remain in host blood for approximately four years and in many cases, it has seen that these memory cells can respond to the invasion of SARS-CoV in the host by recognizing S-proteins even after six years of recovery (Tang et al., Citation2011). A cytokine storm similar to the infection of SARS-CoV and MERS-CoV occurs in the cases of severe SARS-CoV-2 infection as well. Acute respiratory distress syndrome (ARDS) occurs during immune pathological events of SARS-CoV-2. Mainly in ARDS, the main mechanism which may occur is cytokine and chemokine storm. In this, a large number of inflammatory agents have released mainly cytokines including interferons (alpha and gamma), interleukins, and tumor necrosis factor-alpha and beta. Other than this a large number of chemokines are also released. This aggressive response by the immune system causes the body to become violent which may result in ARDS and organ failure and ultimately to death (Channappanavar & Perlman, Citation2017).
5. Transmission
A large number of infected people were first reported to be associated with exposure to animal market the Huanan Seafood Wholesale Market of Wuhan. Hence, the disease was suggested to be zoonotic in nature. As the number of cases increased, it became clear that contact or exposure to animals is not necessary for this infection rather it can be spread, by close contact, from person to person (Cascella et al., Citation2020). Search for the intermediate host of novel coronavirus is continue, but no authentic host has been identified yet. Some reports identified snakes as the possible intermediate host while some other findings cues toward the mammals and bats to be the possible reservoirs of COVID-19 (Rothan & Byrareddy, Citation2020). SARS-CoV-2 can spread through air droplets by the sneeze of an infected person. The symptomatic person can spread droplets more frequently and considered as a source of further spread as compared to the asymptomatic carriers, but they cannot be neglected as well. However, the virus is not transmitted from pregnant mother to her child, but might be transferred to the child during cesarean or through vaginal birth. So it’s the utmost necessity to take preventive care during cesarean birth or normal birth to save a child from a deadly virus because pregnant women are highly susceptible to respiratory disease-causing agents. In our normal routine life, preventive measures should be taken to avoid viral spread (Phan et al., Citation2020). Surface viability of SARS-CoV-2 on different surfaces is different i.e. depending upon either the surface is living or non-living which is a major factor in this regard. A patient suffering from COVID-19 when coughs in any environment, the aerosols produced due to the coughing remains stranded in the air in which SARS-CoV-2 remains viable for up to 3 hours. Viability time for other surfaces is even more than 3 hours ranging from 4 to 72 hours and even more.
The maximum time for viability was shown on plastic surfaces while for other surfaces like steel and cardboard, the viability time is much less than the plastic surfaces. The stability and viability of SARS-CoV-2 in aerosols and other surfaces can be reduced by sterilization and using detergent.
6. Clinical features
The clinical manifestations of SARS-CoV-2 are reported differently in different studies which include some common signs and symptoms and some different features as shown in . Its symptoms range from asymptomatic to severe respiratory distress and dysfunction of several body organs which ultimately causes the death of patients (Singhal, Citation2020). The incubation period of SARS-CoV-2 is approximately 5.2 days while the time period from the appearance of symptoms and the death of patients ranges from 6 to 41 days. This time period depends upon the age and health status of the patient as the patients of age greater than 70 died earlier than the patients of age less than 70 years (Rothan & Byrareddy, Citation2020). Studies conducted in Wuhan during 16 December 2019, to 3 February 2020, reported the sign and symptoms of more than 600 patients which revealed some common signs and symptoms including fever, cough, sore throat, runny nose, headache, sputum production, myalgia, fatigue, shortness of breath and diarrhea. While some other symptoms such as confusion, rhinorrhea, anorexia, and hemoptysis have also been reported (Song et al., Citation2020). Comparing the findings of various studies, it became clear that COVID-19 results in respiratory as well as systemic disorders. Respiratory disorders cause complications in the respiratory tract i.e. pneumonia, sneezing, ground-glass opacities, RNAanemia, and ARDS while systemic disorders include fever, hypoxemia, cardiac distress, lymphopenia and diarrhea (Rothan & Byrareddy, Citation2020).
Figure 6. Reapiratory and systemic disorders caused by SARS-CoV 2 adaptive from (Rothan & Byrareddy, Citation2020).
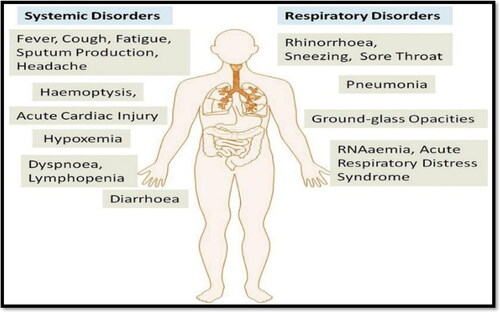
7. Diagnosis
Specific molecular tests are carried out on the respiratory samples of COVID-19 patients for the diagnosis purpose. WHO has recommended taking samples from both i.e. upper as well as from lower respiratory tract. Samples of the upper respiratory tract are taken from throat and nasopharynx using swab while samples of the lower respiratory tract can be collected by expectorating sputum, endotracheal fluid, and Bronchoalveolar lavage. Virus can also be found in stool samples and blood samples (in severe cases) of patients. Nasal and throat swabs show 30%–60% positive results. Moreover, viral RNA can be easily detected in a nasophyrangeal specimen. RT PCR can be used for the detection of infection in patient’s samples. As the infection rate of SARS-CoV-2 is rapid therefore, it is an urgent need to detect the causative agent in the person’s body as soon as possible. Samples obtained from the respiratory tract are analyzed for the detection of SARS-CoV-2 structural spikes, RNA dependent RNA polymerase genes, and nucleocapsid genes that can be used as a target for the detection purpose. CT value i.e. cycle threshold level of SARS-CoV-2 determines either the test is positive or negative; the CT level of 37 shows a positive result while 40 or above shows negative results. According to the current research and evidence, WHO has recommended using immunodiagnostic tests for the diagnosis of COVID-19. For serological testing of humans for SARS-CoV-2 infection IgA, IgM, and total antibodies count is considered. Simple diagnostic kits are available which can detect either the viral proteins in respiratory samples of the patient or the antibodies generated by the patient’s immune system. If the patient's serum has antibodies against specific antigens of SARS-CoV-2, it refers to the indication of a positive result while the absence of any reaction in pateint serum and viral antigens, the results are considered as negative. Some other factors are also being used for diagnosis of COVID-19 such as low count of WBCs, lymphopenia, and low count of platelets. Ct scan imaging of the chest is more specific as it shows sub-segmental consolidations and ground-glass opacities.
8. Drug discovery approaches for SARS-CoV-2
The lack of any specific therapeutic approach and effective ways to treat viral pneumonia outbreak has led the researchers to discover some lead compounds or already approved drugs which can prove as effective treatment options, using the approaches of structure-based drug design, molecular docking and virtual screening of available libraries of compounds.
8.1. Structure-based discovery for new lead compounds
Structure-based drug discovery has long been used to discover new lead compounds that can act as drug candidates (Anderson, Citation2003). The first step of drug designing is the selection of drug targets. These targets could either be viral enzymes, structural proteins, or even the receptors on host cells. For this purpose, differential targets should be selected whose inhibition effects the virus negatively and not the host. After the selection of targets, a library of compounds is formed for docking them against the targets to check their inhibition potential. These compounds could be the already approved drugs, their analogs, a combination of these drugs, and even phytochemicals from medicinal plants. After docking, the lead hits are screened out on the basis of different criteria such as docking score, binding energy, number of interactions and absorption, digestion, metabolism, excretion, and toxicity ADMET properties of these compounds. For SARS-CoV-2, two proteases (3CLpro and Mpro) that play a vital role in the formation of active replication enzymes are considered as the best targets to find antiviral drug candidates for COVID-19.
8.1.1. Chymotrypsin-like cysteine protease (3Clpro)
The latest findings on the structure of 3CLpro of SARS-COV (PDB code 1UK4) and the 3CLpro of SARS-COV2 (PDB code 6LU7) revealed that proteases of both viruses are different in the arrangement of only 12 amino acids where α C-atom reside 1 nm far from the active site of 3CLpro of SARS-COV2. On the basis of these findings, non-covalent inhibitors of SARS-COV 3CLpro could also have an inhibitory effect on 3CLpro of a novel strain of coronavirus (Macchiagodena, Pagliai, & Procacci, Citation2020; Yang et al., Citation2003). Macchiagodena and his colleagues designed a library of ligands and then docked them with 3CLpro of SARS-COV and SARS-COV2 to identify the best lead compound. Their results showed a similar binding energy calculation for proteases of both viruses for each ligand used. The best lead compound (ligand 27) shared a common binding pattern for both viruses. This study strengthens the idea that inhibitors of SARS-COV 3CLpro should be taken under consideration which can prove the best candidates for the novel coronavirus infection (Macchiagodena et al., Citation2020). Another study was also carried out on 3CLpro in which a library of 32,297 phytochemicals and Chinese medicinal compounds was screened via docking with the 3D homology model of 3CLpro. Their findings revealed that the top nine hits or the compounds could serve as drug candidates for SARS-CoV-2, but further optimization of compounds is still required (ul Qamar, Alqahtani, Alamri, & Chen, Citation2020). shows some lead hit compounds for 3CLpro.
Figure 7. Molecular docking findings of SARS-CoV-2 protease, 3Clpro A. Cartoon representation of the 3CLpro monomer model of SARS-CoV-2 B. Docking of 5,7,3′,4′-tetrahydroxy-2’-(3,3-dimethylallyl) isoflavone inside the receptor-binding site of SARS-CoV-2 3CLpro (ul Qamar et al., Citation2020). C. Ligand 27 from the compounds library D. ligand 27 in the binding pocket of 3CLpro of SARS-CoV-2 (Macchiagodena et al., Citation2020).
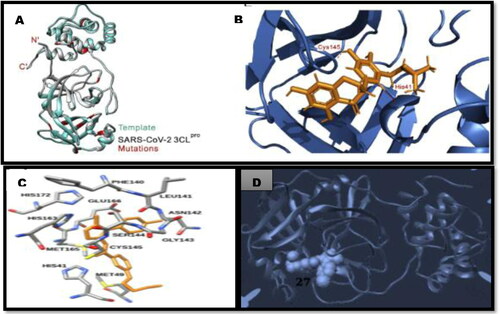
8.1.2. COVID-19 main protease (Mpro)
A viral protein named COVID-19 main protease (Mpro) is considered as a vital target for drug discovery as this enzyme regulates the process of replication and transcription in Coronavirus (Anand, Ziebuhr, Wadhwani, Mesters, & Hilgenfeld, Citation2003). A group of Chinese researchers, Liu and his colleagues, has also identified the crystalline structure of Mpro by using N3 (a mechanism-based inhibitor of Mpro) through in silico drug designing approaches (Liu et al., Citation2020). Then a library of 10,000 compounds that contained approved drugs, a number of pharmacologically active compounds and even drug candidates under clinical trials was assayed through structure-based high-throughput screening to identify the best inhibitor of Mpro. Seven compounds among this library proved the best inhibitors of Mpro in silico while the cell-based assays revealed Ebselen, N3, and Thiadiazolidinone as strong antiviral agents (Jin et al., Citation2020). This study demonstrated the efficiency of in silico screening strategy for rapid drug discovery. After this, a number of similar studies have also been carried out in the search of lead inhibitors of viral proteins. Another molecular docking study was also carried out at Mpro by screening a number of bioactive phytochemicals from approved medicinal plants against it. Nelfinavir and lopinavir were included in the compound's library as a standard for comparison. Based on the binding energy data of all the ligands docked with Mpro, luteolin-7-glucoside, apigenin-7-glucoside, demethoxycurcumin, oleuropein, catechin, curcumin, and epicatechin-gallate appeared to have best drug potential, but it still needs further research to investigate any ill effect (Khaerunnisa, Kurniawan, Awaluddin, Suhartati, & Soetjipto, Citation2020). A library of 80 flavonoid compounds from medicinal plants was also screened via docking against COVID-19 Mpro and binding energy data was used to evaluate the best inhibitor. The phytochemicals such as hesperidin, diosmin, rutin, diacetylcurcumin, and apiin appeared more effective than nelfinavir according to the comparison of their binding energies. These studies have provided a valid option to carry out advanced research for the discovery of the best drug candidates (Adem, Eyupoglu, Sarfraz, Rasul, & Ali, Citation2020). Ginkgolide A, a terpenoid present in many medicinal plants has also shown high affinity and strong binding with COVID-19 proteases after docking (Shaghaghi, Citation2020).
8.2. Drug repurposing of approved drugs
Drug repurposing is a suitable strategy for fast and rapid discovery of drugs and in the present age of COVID-19 pandemic, its demand has been increased to a great extent (Aly, Citation2020). shows the drugs being considered for drug repurposing for the cure of COVID-19. In order to find the best antiviral drugs for COVID-19, it is essential to best know the structural proteins of viruses and their interactions with human receptors. Wenzhong and Hualan tried to identify the biological roles of certain structural proteins of novel coronavirus by analyzing conserved domains of proteins through molecular modeling and molecular docking of viral proteins with hemoglobin structure. Their findings hypothesized that surface glycoproteins of novel coronavirus have the ability to bind with porphyrin of hemoglobin while other proteins such as ORF1ab, ORF3a, and ORF10 have roles in dissociating iron from 1-beta chain of hemoglobin which results in the decreasing oxygen and carbon dioxide binding ability of hemoglobin and ultimately extreme poisoning and inflammation in lung cells. The validation analysis of these findings revealed that chloroquine could prevent the binding of these surface proteins to heme porphyrin and beta-chain and can be used to relieve the symptoms of infection. Another drug Favipiravir was also considered as an inhibitor of ORF7a protein of coronavirus. The hypothesis provided in this research was further analyzed by other researchers as well (Wenzhong & Hualan, Citation2020). On contrary to this (Read, Citation2020) claimed that the interpretations based on accepted standards do not support this hypothesis as there is no evidence of shared functional domains between hemoglobin and viral proteins. Moreover, it was commented that in the previous hypothesis, the highest binding energies were taken under consideration while the appropriate and standard way is to consider the lowest binding energies because it ensures the most stable complex (Read, Citation2020). Anyhow, more research is required in this scenario.
Table 2. Re-purposing of already approved drugs and their effect on selected targets.
In another study, an HIV protease inhibitor and a nucleoside analog i.e. indinavir and remdisiver, respectively were docked with SARS-CoV-2 proteins to check their efficacy against coronavirus infection. Findings suggest that Indinavir does not dock with any binding pocket of coronavirus protease while the active form of Remdesivir (CHEMBL2016761) showed perfect docking in the nucleoside triphosphate motif region of protease. Hence, Remdesivir could be suggested as a potential drug candidate against COVID-19, but at the same time, further clinical trials must be done for the confirmation of the efficacy of this drug (Chang et al., Citation2020). Muralidharan and his colleagues have suggested that combinations of drugs can prove fruitful instead of using single drugs in therapy. They tested the combination of oseltamivir, lopinavir, and ritonavir, using computational docking methods, against SARS-CoV-2 proteases. Their root mean square deviation and root mean square fluctuation results revealed that a stable complex of the combined drugs and protease binding site formed in a range of 3 Å that is in a quite favorable range. Hence, the combination of oseltamivir, lopinavir, and ritonavir must be further explored to confirm their efficacy against COVID-19 (Muralidharan, Sakthivel, Velmurugan, & Gromiha, Citation2020). In another study, Mpro and TMPRSS2 receptors were considered for docking study to screen out a library of available drugs and some novel drug-like compounds. They reported the best docking score of Mpro for Talampicillin and Lurasidone, the two available drugs. Moreover, Rubitecan and Loprazolam drugs along with two novel compounds (ZINC000015988935 and ZINC000103558522) showed the best docking with TMPRSS2 receptors. ADMET properties of all these compounds are also suitable to be used as drugs (Elmezayen, Al-Obaidi, Şahin, & Yelekçi, Citation2020). Rolitetracycline and a combination of metamizole with darunavir have also been checked for their docking with protease enzymes of COVID-19 showed promising results, but further studies are still required (Aly, Citation2020). Farag and his colleagues carried out the screening of almost 2000 FDA approved drugs against Mpro and reported darunavir, saquinavir, and nelfinavir as top hits. These drugs are being tested on Corona patients as well (Farag, Wang, Ahmed, & Sadek, Citation2020).
8.3. Receptor based drug discovery
Mode of receptor recognition and transmission of COVID-19 into the host cells could also be considered as a target to search for the best therapeutic agent. ACE2 is a membrane-associated carboxypeptidase that is present in several organs of the body such as the heart, kidney, lungs, and small intestine. In the lungs, ACE2 is expressed mostly in type II alveolar cells, epithelial cells of bronchi, trachea, and in macrophages. Angiotensin II is degraded and converted into angiotensin 1–7, by ACE2, which activates oncogene receptors. As a result of this, the actions of angiotensin II type 1 receptor (AT1R) are regulated negatively. Therefore ACE2 is considered as the best target (Hamming et al., Citation2004). ACE2 cannot be considered as the only receptor of SARS-CoV-2 in the host cells because there is certain evidence of other receptors as well. A C-type lectin glycoprotein CD2089L is also identified as an alternative receptor in the in vitro cell culture of hamster ovary (Jeffers et al., Citation2004). Glucose regulated protein 78 (GRP78), another cell surface receptor, was also checked by docking it against S protein of SARS-CoV-2. Studies revealed that the substrate binding domain β (SBDβ) of GRP78 showed favorable binding and affinity with III and IV regions of s protein with a value of 9.8 kcal/mol. Hence, these binding pockets of GRP78 could also be considered in the development of therapeutics against SARS-CoV-2 (Ibrahim, Abdelmalek, Elshahat, & Elfiky, Citation2020).
9. Possible drugs and treatment for COVID-19
Currently, the only treatment for COVID-19 is self-isolation or social distancing. However, scientists are trying their best to find the treatment. The reverse genetic system can be used to make coronavirus so that more research experiments can be done and treatment can be found as soon as possible (Cockrell, Beall, Yount, & Baric, Citation2017). NSPs, involved in replication and pathogenesis of virus, are highly conserved among SARS-CoV-2, MERS-CoV, and SARS-CoV and hence, they can be used as a target to combat COVID-19 pandemic. So, by inhibiting the mechanism of replication or pathogenesis the number of viruses can be decreased. Nsps are essentially involved in the replication of viral genome by the help of RNA dependent RNA polymerase, moreover, some proteases like papain-like proteases (PLPro) and 3C like proteases (3CLPro) these proteases are involved in the proteolytic cleavage of NSPs of virus to perform specific functions so these all are being used as a target to make specific antiviral drugs for SARS-CoV 2 (Totura & Bavari, Citation2019). enlists some drugs that are being tested on COVID-19 patients and have positive results.
Table 3. Antiviral drugs, route of administration and duration of treatment for COVID-19.
Drugs that can inhibit the function of proteases can be used to decrease the viral load. Therefore, certain protease inhibitors can be used such as lopinavir and ritonavir which were used as protease inhibitors for HIV-1(Wu et al., Citation2004). This combination along with ribavirin was used to treat SARS-CoV and MERS-CoV infection in the past to decrease the severity of the infection and to save many lives (Chu et al., Citation2004; de Wilde et al., Citation2014). Ribavirin a guanosine analog can be used as a treatment for COVID-19 because it inhibits the formation of RNA by RdRp but researches have shown that the efficacy of ribavirin alone is very low and can also increase the signs of disease. Therefore, either it shouldn't be taken or it should be given in combination with interferons (Falzarano et al., Citation2013; Ferron et al., Citation2018; Hart et al., Citation2014).
Monoclonal antibodies can play a vital role in the treatment of COVID-19, but the need is to prepare MAbs against structural proteins of viruses such as S proteins, M proteins, and E proteins. MAbs however is not a useful treatment for the COVID-19 because it can modify its structure by mutations as seen already in many cases earlier (Sui et al., Citation2014). Remdesivir which is a nucleoside analog and can be used to treat COVID-19. It is also suggested that Remdesivir can be used at very low concentrations to treat SARS-CoV-2. Moreover, experiments on mice showed that reduced viral loads in the lungs tissue of mice (Sheahan et al., Citation2020). Holshue et al. also suggested that remdesivir can also be used as an efficient treatment for COVID-19 in the United States (Holshue et al., Citation2020). Phase 3 clinical trials have been started since 5 February 2020, and these trials would be finalized at the end of April. Patients have given an initial dose of 200 milligrams and 100 milligrams for 9 days subsequently intravenously (Al-Tawfiq, Al-Homoud, & Memish, Citation2020; Grein et al., Citation2020).
Other drugs such as darunavir which is an HIV1 second-generation drug can also be used as a protease inhibitor for the treatment of COVID-19. A study has been initiated for the clinical trials of darunavir on February 4 (China News Network, Citation2020). SARS-CoV-2 uses ACE2 and type 2 transmembrane serine protease (TMSPSS) to enter into the host cell hence, TMSPSS inhibitors can be used to treat COVID-19 by blocking the entry. Imatinib which stops the fusion of viral particles with endosomal membrane can also be used as a potential drug against COVID-19 (Coleman et al., Citation2016).
Currently, vaccine development is going on to target S-protein and whole virion including RNA vaccine, DNA vaccine to target S proteins, while different recombinant protein vaccine and viral vector-based proteins are also under the consideration of research. To target, the whole virion live attenuated vaccines and inactivated vaccines are being in process for development (Alsuliman, Sulaiman, Ismail, Srour, & Alrstom, Citation2020). According to the draft of candidate vaccines, updated by WHO till 24 July 2020, there are almost 25 candidate vaccines for COVID-19 which are under trial. The inactivated vaccine candidates such as NCT04456595, ChiCTR2000034780, and ChiCTR2000034780 are still in phase 3. There are other vaccine candidates as well such as non-viral candidate vaccines; ISRCTN89951424 and ChiCTR2000031781 which are in phase 3 and phase 2, respectively. Clinical trials for nucleotide analogs, S proteins, and protein inhibitors are under research currently in many countries. However, there is no any declared vaccine for COVID-19 yet. There are a number of factors which are responsible for this delay i.e. development of animal model for COVID-19 vaccine testing, expression of virus in wild type animal such as a wild type mice and other such as extremely efficient and up-to-date information, speciality and laboratory practices etc (Alsuliman et al., Citation2020).
10. Preventions
In the recent age where no specific cure of COVID-19 has been approved yet, preventive measures are the only way to limit the spread of this infection. It is evident from the previous studies that an epidemic keeps on increasing if its R0 value remains greater than 1 and this value is still 2.2 for COVID-19. Preventive measures and self-isolation is the only way to reduce the value of R0. As the infection spreads person to person, it is necessary to maintain an appropriate distance from the patient and any material that has remained in direct or indirect contact with the patient . WHO has issued some important precautions that should be adopted for better care. According to WHO, hands should be washed properly or sanitized using an alcohol-based sanitizer. A distance of about 3 feet should be maintained between two people. Crowded places should be avoided as there is a high chance of spread from person to person. Before going out, the face should be covered with N95 or FFp3 masks and eye protection goggles. The proper measures of hygiene should be practiced and immunity should be increased by a healthy diet.
11. Discussion
Coronaviruses are historically important in causing various human and animal diseases. In late 2019, a new strain of coronavirus SARS-CoV-2 has emerged in China which causes severe pneumonia-like disease. At present no appropriate drug is available for the cure of this disease and hence, there is a need to discover some potential cure. Structure-based drug designing, via molecular docking and screening of compounds, is one of the best methods to find potential drug candidates. Complete information about the replication cycle of SARS-CoV-2 provides a variety of targets i.e. proteases (CLpro, Mpro) replication enzyme (Helicase, RdRp) or host cell receptors (ACE2, TMPRSS2 etc) that are being exploited for drug discovery. After target selection, pharmacologically active compounds are screened against these targets for the best suitable hits. Here the repurposing of already approved drugs is the best choice as one antiviral-drug can act as an inhibitor of various viral enzymes because of the similarity in their function in several metabolic pathways. For example, an influenza virus drug and hepatitis C virus drug i.e. favipiravir and sofosbuvir respectively were repurposed against Ebola and Zika virus (Mercorelli, Palù, & Loregian, Citation2018). As the genomic analysis of SARS-CoV and SARS-CoV-2 indicates that CL protease of both has high similarity, therefore, drugs of SARS-CoV (nelfinavir, ritonavir, and lopinavir, etc) are being widely investigated for SARS-CoV-2. Moreover, phytochemicals from medicinal plants are also the best option because medicinal plants usually form secondary metabolites such as flavonoids that have drug-like activities. Therefore, several molecular docking studies are also investigating the libraries of phytochemicals to identify potential drug candidates.
12. Conclusion
SARS-CoV-2, an RNA virus enters the human cells via its S protein. Identification of its structural proteins and its pattern of pathogenesis is vital for the search of specific treatment. COVID-19 disease has become a pandemic that is affecting many lives worldwide. Its clinical manifestations include fever, cough, sneezing, severe respiratory distress which can spread through droplets. Research works are being carried out different aspects of drug repurposing, the discovery of new antiviral compounds, and receptor inhibitors via docking and screening strategies. The lead compounds are actually screened out by considering several parameters such as binding energies, the total number of interactions of the compound with the binding pocket of protein, and also the ADMET properties. However, only the best docking score of the compound is not sufficient to make it a drug candidate because, besides docking, the other most important property of drugs is their best suitable pharmacodynamic and pharmacokinetic properties. Hence, the lead hit compounds are further analyzed to have the best suitable candidate. Moreover, after in silico screening of compounds, there are several other steps that must be carried out such as lead compound optimization, in vitro, and in vivo studies to check the response of host cells for the specific compound used.
Disclosure statement
No potential conflict of interest was reported by the authors.
References
- Adem, S., Eyupoglu, V., Sarfraz, I., Rasul, A., & Ali, M. (2020). Identification of potent COVID-19 main protease (Mpro) inhibitors from natural polyphenols: An in silico strategy unveils a hope against CORONA. Preprints. doi:https://doi.org/10.20944/preprints202003.0333.v1
- Alanagreh, L. A., Alzoughool, F., & Atoum, M. (2020). The human coronavirus disease COVID-19: Its origin, characteristics, and insights into potential drugs and its mechanisms. Pathogens, 9(5), 331. doi:https://doi.org/10.3390/pathogens9050331
- Alsuliman, T., Sulaiman, R., Ismail, S., Srour, M., & Alrstom, A. (2020). COVID-19 paraclinical diagnostic tools: Updates and future trends. Current Research in Translational Medicine, 68(3), 83–91. doi:https://doi.org/10.1016/j.retram.2020.06.001
- Al-Tawfiq, J. A., Al-Homoud, A. H., & Memish, Z. A. (2020). Remdesivir as a possible therapeutic option for the COVID-19. Travel Medicine and Infectious Disease, 34, 101615. doi:https://doi.org/10.1016/j.tmaid.2020.101615
- Aly, O. M. (2020). Molecular docking reveals the potential of aliskiren, dipyridamole, mopidamol, rosuvastatin, rolitetracycline and metamizole to inhibit COVID-19 virus main protease. ChemRxiv, Preprint. doi:https://doi.org/10.26434/chemrxiv.12061302.v1
- Anand, K., Ziebuhr, J., Wadhwani, P., Mesters, J. R., & Hilgenfeld, R. (2003). Coronavirus main proteinase (3CLpro) structure: Basis for design of anti-SARS drugs. Science (New York, N.Y.), 300(5626), 1763–1767. doi:https://doi.org/10.1126/science.1085658
- Anderson, A. C. (2003). The process of structure-based drug design. Chemistry & Biology, 10(9), 787–797. doi:https://doi.org/10.1016/j.chembiol.2003.09.002
- Belouzard, S., Chu, V. C., & Whittaker, G. R. (2009). Activation of the SARS coronavirus spike protein via sequential proteolytic cleavage at two distinct sites. Proceedings of the National Academy of Sciences of the United States of America, 106(14), 5871–5876. doi:https://doi.org/10.1073/pnas.0809524106
- Bogoch, I. I., Watts, A., Thomas-Bachli, A., Huber, C., Kraemer, M. U., & Khan, K. (2020). Pneumonia of unknown etiology in Wuhan, China: Potential for international spread via commercial air travel. Journal of Travel Medicine, 27(2), taaa008. doi:https://doi.org/10.1093/jtm/taaa008
- Cao, B., Wang, Y., Wen, D., Liu, W., Wang, J., Fan, G., … Li, X. (2020). A trial of lopinavir–ritonavir in adults hospitalized with severe COVID-19. New England Journal of Medicine, 382(19), 1787–1799. doi:https://doi.org/10.1056/NEJMoa2001282
- Cascella, M., Rajnik, M., Cuomo, A., Dulebohn, S. C., & Di Napoli, R. (2020). Features, evaluation and treatment coronavirus (COVID-19). In Statpearls [internet]. Treasure Island, FL: StatPearls Publishing.
- Chang, Y. C., Tung, Y. A., Lee, K. H., Chen, T. F., Hsiao, Y. C., Chang, H. C., … Shih, S. S. (2020). Potential therapeutic agents for COVID-19 based on the analysis of protease and RNA polymerase docking. Preprints. doi:https://doi.org/10.20944/preprints202002.0242.v1
- Channappanavar, R., & Perlman, S. (2017). Pathogenic human coronavirus infections: Causes and consequences of cytokine storm and immunopathology. In Seminars in immunopathology (Vol. 39, No. 5, pp. 529–539). Berlin, Heidelberg: Springer. doi:https://doi.org/10.1007/s00281-017-0629-x
- China News Network. (2020, February 5). The team of Li Lanjuan: Abidor and Darunavir can effectively inhibit coronavirus. Retrieved from http://www.sd.chinanews.com/2/2020/0205/70145.html
- Chu, C. M., Cheng, V. C. C., Hung, I. F. N., Wong, M. M. L., Chan, K. H., Chan, K. S., … Peiris, J. S. M. (2004). Role of lopinavir/ritonavir in the treatment of SARS: Initial virological and clinical findings. Thorax, 59(3), 252–256. doi:https://doi.org/10.1136/thorax.2003.012658
- Cockrell, A. S., Beall, A., Yount, B., & Baric, R. (2017). Efficient reverse genetic systems for rapid genetic manipulation of emergent and preemergent infectious coronaviruses. In Reverse genetics of RNA viruses (pp. 59–81). New York, NY: Humana Press.
- Coleman, C. M., Sisk, J. M., Mingo, R. M., Nelson, E. A., White, J. M., & Frieman, M. B. (2016). Abelson kinase inhibitors are potent inhibitors of severe acute respiratory syndrome coronavirus and Middle East respiratory syndrome coronavirus fusion. Journal of Virology, 90(19), 8924–8933. doi:https://doi.org/10.1128/JVI.01429-16
- de Wilde, A. H., Jochmans, D., Posthuma, C. C., Zevenhoven-Dobbe, J. C., van Nieuwkoop, S., Bestebroer, T. M., … Snijder, E. J. (2014). Screening of an FDA-approved compound library identifies four small-molecule inhibitors of Middle East respiratory syndrome coronavirus replication in cell culture. Antimicrobial Agents and Chemotherapy, 58(8), 4875–4884. doi:https://doi.org/10.1128/AAC.03011-14
- de Wit, E., van Doremalen, N., Falzarano, D., & Munster, V. J. (2016). SARS and MERS: Recent insights into emerging coronaviruses. Nature Reviews. Microbiology, 14(8), 523–534. doi:https://doi.org/10.1038/nrmicro.2016.81
- Dorward, J., & Gbinigie, K. (2020). Lopinavir/ritonavir: A rapid review of effectiveness in COVID-19.
- Elmezayen, A. D., Al-Obaidi, A., Şahin, A. T., & Yelekçi, K. (2020). Drug repurposing for coronavirus (COVID-19): In silico screening of known drugs against coronavirus 3CL hydrolase and protease enzymes. Journal of Biomolecular Structure and Dynamics. doi:https://doi.org/10.1080/07391102.2020.1758791
- Falzarano, D., De Wit, E., Martellaro, C., Callison, J., Munster, V. J., & Feldmann, H. (2013). Inhibition of novel β coronavirus replication by a combination of interferon-α2b and ribavirin. Scientific Reports, 3, 1686. doi:https://doi.org/10.1038/srep01686
- Farag, A., Wang, P., Ahmed, M., & Sadek, H. (2020). Identification of FDA approved drugs targeting COVID-19 virus by structure-based drug repositioning. ChemRxiv, Preprint. doi:https://doi.org/10.26434/chemrxiv.12003930.v1
- Fehr, A. R., & Perlman, S. (2015). Coronaviruses: An overview of their replication and pathogenesis. In Coronaviruses (pp. 1–23). New York, NY: Humana Press.
- Ferron, F., Subissi, L., De Morais, A. T. S., Le, N. T. T., Sevajol, M., Gluais, L., … Imbert, I. (2018). Structural and molecular basis of mismatch correction and ribavirin excision from coronavirus RNA. Proceedings of the National Academy of Sciences of the United States of America, 115(2), E162–E171. doi:https://doi.org/10.1073/pnas.1718806115
- Gendrot, M., Javelle, E., Le Dault, E., Clerc, A., Savini, H., & Pradines, B. (2020). Chloroquine as prophylactic agent against COVID-19? International Journal of Antimicrobial Agents, 55(6), 105980. doi:https://doi.org/10.1016/j.ijantimicag.2020.105980
- Grein, J., Ohmagari, N., Shin, D., Diaz, G., Asperges, E., Castagna, A., … Nicastri, E. (2020). Compassionate use of remdesivir for patients with severe COVID-19. New England Journal of Medicine, 382(24), 2327–2336. doi:https://doi.org/10.1056/NEJMoa2007016
- Hamming, I., Timens, W., Bulthuis, M. L. C., Lely, A. T., Navis, G. J., & van Goor, H. (2004). Tissue distribution of ACE2 protein, the functional receptor for SARS coronavirus. A first step in understanding SARS pathogenesis. The Journal of Pathology, 203(2), 631–637. doi:https://doi.org/10.1002/path.1570
- Hart, B. J., Dyall, J., Postnikova, E., Zhou, H., Kindrachuk, J., Johnson, R. F., … Hensley, L. (2014). Interferon-β and mycophenolic acid are potent inhibitors of Middle East respiratory syndrome coronavirus in cell-based assays. The Journal of General Virology, 95(Pt 3), 571–577. doi:https://doi.org/10.1099/vir.0.061911-0
- Holshue, M. L., DeBolt, C., Lindquist, S., Lofy, K. H., Wiesman, J., Bruce, H., … Diaz, G. (2020). First case of 2019 novel coronavirus in the United States. New England Journal of Medicine, 382(10), 929–936.
- Ibrahim, I. M., Abdelmalek, D. H., Elshahat, M. E., & Elfiky, A. A. (2020). COVID-19 spike-host cell receptor GRP78 binding site prediction. Journal of Infection, 80(5), 554–562. doi:https://doi.org/10.1016/j.jinf.2020.02.026
- Jeffers, S. A., Tusell, S. M., Gillim-Ross, L., Hemmila, E. M., Achenbach, J. E., Babcock, G. J., … Ambrosino, D. M. (2004). CD209L (L-SIGN) is a receptor for severe acute respiratory syndrome coronavirus. Proceedings of the National Academy of Sciences of the United States of America, 101(44), 15748–15753. doi:https://doi.org/10.1073/pnas.0403812101
- Jiang, F., Deng, L., Zhang, L., Cai, Y., Cheung, C. W., & Xia, Z. (2020). Review of the clinical characteristics of coronavirus disease 2019 (COVID-19). Journal of General Internal Medicine, 35(5), 1545–1545. doi:https://doi.org/10.1007/s11606-020-05762-w
- Jin, Z., Du, X., Xu, Y., Deng, Y., Liu, M., Zhao, Y., … Duan, Y. (2020). Structure of Mpro from COVID-19 virus and discovery of its inhibitors. bioRxiv, Preprint. doi:https://doi.org/10.1101/2020.02.26.964882
- Khaerunnisa, S., Kurniawan, H., Awaluddin, R., Suhartati, S., & Soetjipto, S. (2020). Potential inhibitor of COVID-19 main protease (Mpro) from several medicinal plant compounds by molecular docking study. Preprint. doi:https://doi.org/10.20944/preprints202003.0226.v1
- Khalili, J. S., Zhu, H., Mak, N. S. A., Yan, Y., & Zhu, Y. (2020). Novel coronavirus treatment with ribavirin: Groundwork for an evaluation concerning COVID‐19. Journal of Medical Virology, 92(7), 740–746. doi:https://doi.org/10.1002/jmv.25798
- Li, X., Geng, M., Peng, Y., Meng, L., & Lu, S. (2020). Molecular immune pathogenesis and diagnosis of COVID-19. Journal of Pharmaceutical Analysis, 10(2), 102–108. doi:https://doi.org/10.1016/j.jpha.2020.03.001
- Liu, J., Wu, P., Gao, F., Qi, J., Kawana-Tachikawa, A., Xie, J., … Gao, G. F. (2010). Novel immunodominant peptide presentation strategy: A featured HLA-A*2402-restricted cytotoxic T-lymphocyte epitope stabilized by intrachain hydrogen bonds from severe acute respiratory syndrome coronavirus nucleocapsid protein. Journal of Virology, 84(22), 11849–11857. doi:https://doi.org/10.1128/JVI.01464-10
- Liu, M., Jin, Z., Du, X., Xu, Y., Deng, Y., Zhao, Y., … Yu, J. (2020). Structure-based drug design, virtual screening and high-throughput screening rapidly identify antiviral leads targeting COVID-19. bioRxiv, Preprint.
- Lu, H., Stratton, C. W., & Tang, Y. W. (2020). Outbreak of pneumonia of unknown etiology in Wuhan China: The mystery and the miracle. Journal of Medical Virology, 92(4), 401–402. doi:https://doi.org/10.1002/jmv.25678
- Macchiagodena, M., Pagliai, M., & Procacci, P. (2020). Identification of potential binders of the main protease 3CLpro of the COVID-19 via structure-based ligand design and molecular modeling. Chemical Physics Letters, 750, 137489. doi:https://doi.org/10.1016/j.cplett.2020.137489
- Mercorelli, B., Palù, G., & Loregian, A. (2018). Drug repurposing for viral infectious diseases: how far are we? Trends in Microbiology, 26(10), 865–876. doi:https://doi.org/10.1016/j.tim.2018.04.004
- Mousavizadeh, L., & Ghasemi, S. (2020). Genotype and phenotype of COVID-19: Their roles in pathogenesis. Journal of Microbiology, Immunology and Infection. doi:https://doi.org/10.1016/j.jmii.2020.03.022
- Muralidharan, N., Sakthivel, R., Velmurugan, D., & Gromiha, M. M. (2020). Computational studies of drug repurposing and synergism of lopinavir, oseltamivir and ritonavir binding with SARS-CoV-2 Protease against COVID-19. Journal of Biomolecular Structure and Dynamics. doi:https://doi.org/10.1080/07391102.2020.1752802
- Perlman, S., & Netland, J. (2009). Coronaviruses post-SARS: Update on replication and pathogenesis. Nature Reviews. Microbiology, 7(6), 439–450. doi:https://doi.org/10.1038/nrmicro2147
- Phan, L. T., Nguyen, T. V., Luong, Q. C., Nguyen, T. V., Nguyen, H. T., Le, H. Q., … Pham, Q. D. (2020). Importation and human-to-human transmission of a novel coronavirus in Vietnam. The New England Journal of Medicine, 382(9), 872–874. doi:https://doi.org/10.1056/NEJMc2001272
- Read, R. (2020). Flawed methods in “COVID-19: Attacks the 1-beta chain of hemoglobin and captures the porphyrin to inhibit human heme metabolism.” ChemRxiv, Preprint. doi:https://doi.org/10.26434/chemrxiv.12120912.v2
- Richman, D. D., Whitley, R. J. and Hayden, F. G. (Eds.). (2016). Clinical virology. Hoboken, NJ: John Wiley & Sons.
- Rothan, H. A., & Byrareddy, S. N. (2020). The epidemiology and pathogenesis of coronavirus disease (COVID-19) outbreak. Journal of Autoimmunity, 109, 102433. doi:https://doi.org/10.1016/j.jaut.2020.102433
- Sallard, E., Lescure, F. X., Yazdanpanah, Y., Mentre, F., Peiffer-Smadja, N., Florence, A. D. E. R., … Bouadma, L. (2020). Type 1 interferons as a potential treatment against COVID-19. Antiviral Research, 178, 104791. doi:https://doi.org/10.1016/j.antiviral.2020.104791
- Shaghaghi, N. (2020). Molecular docking study of novel COVID-19 protease with low risk terpenoides compounds of plants. ChemRxiv, Preprint. doi:https://doi.org/10.26434/chemrxiv.11935722.v1
- Sheahan, T. P., Sims, A. C., Leist, S. R., Schäfer, A., Won, J., Brown, A. J., … Spahn, J. E. (2020). Comparative therapeutic efficacy of remdesivir and combination lopinavir, ritonavir, and interferon beta against MERS-CoV. Nature Communications, 11(1), 1–14. doi:https://doi.org/10.1038/s41467-019-13940-6
- Singhal, T. (2020). A review of coronavirus disease-2019 (COVID-19). Indian Journal of Pediatrics, 87(4), 281–286. doi:https://doi.org/10.1007/s12098-020-03263-6
- Sohrabi, C., Alsafi, Z., O'Neill, N., Khan, M., Kerwan, A., Al-Jabir, A., … Agha, R. (2020). World Health Organization declares global emergency: A review of the 2019 novel coronavirus (COVID-19). International Journal of Surgery, 76, 71–76. doi:https://doi.org/10.1016/j.ijsu.2020.02.034
- Song, F., Shi, N., Shan, F., Zhang, Z., Shen, J., Lu, H., … Shi, Y. (2020). Emerging 2019 novel coronavirus (2019-nCoV) pneumonia. Radiology, 295(1), 210–217. doi:https://doi.org/10.1148/radiol.2020200274
- Sui, J., Deming, M., Rockx, B., Liddington, R. C., Zhu, Q. K., Baric, R. S., & Marasco, W. A. (2014). Effects of human anti-spike protein receptor binding domain antibodies on severe acute respiratory syndrome coronavirus neutralization escape and fitness. Journal of Virology, 88(23), 13769–13780. doi:https://doi.org/10.1128/JVI.02232-14
- Tang, F., Quan, Y., Xin, Z. T., Wrammert, J., Ma, M. J., Lv, H., … Cao, W. C. (2011). Lack of peripheral memory B cell responses in recovered patients with severe acute respiratory syndrome: A six-year follow-up study. Journal of Immunology, 186(12), 7264–7268. doi:https://doi.org/10.4049/jimmunol.0903490
- Thiel, V., Ivanov, K. A., Putics, A., Hertzig, T., Schelle, B., Bayer, S., … Gorbalenya, A. E. (2003). Mechanisms and enzymes involved in SARS coronavirus genome expression. The Journal of General Virology, 84(Pt 9), 2305–2315. doi:https://doi.org/10.1099/vir.0.19424-0
- Totura, A. L., & Bavari, S. (2019). Broad-spectrum coronavirus antiviral drug discovery. Expert Opinion on Drug Discovery, 14(4), 397–412. doi:https://doi.org/10.1080/17460441.2019.1581171
- Tyrrell, D. A., & Myint, S. H. (1996). Coronaviruses. In Medical Microbiology (4th ed.). Galveston, TX: University of Texas Medical Branch at Galveston.
- ul Qamar, M. T., Alqahtani, S. M., Alamri, M. A., & Chen, L. L. (2020). Structural basis of SARS-CoV-2 3CLpro and anti-COVID-19 drug discovery from medicinal plants. Journal of Pharmaceutical Analysis, 10(4), 313–319.
- Victoria, R. (2020). 3D visualization of COVID-19 surface released for researchers. Drug Target Reviews.
- Walls, A. C., Park, Y. J., Tortorici, M. A., Wall, A., McGuire, A. T., & Veesler, D. (2020). Structure, function, and antigenicity of the SARS-CoV-2 spike glycoprotein. Cell, 181(2), 281–292.e6. doi:https://doi.org/10.1016/j.cell.2020.02.058
- Wenzhong, L., & Hualan, L. (2020). COVID-19: Attacks the 1-beta chain of hemoglobin and captures the porphyrin to inhibit human heme metabolism. ChemRxiv, Preprint. doi:https://doi.org/10.26434/chemrxiv.11938173.v6
- Wu, C. Y., Jan, J. T., Ma, S. H., Kuo, C. J., Juan, H. F., Cheng, Y. S. E., … Liang, F. S. (2004). Small molecules targeting severe acute respiratory syndrome human coronavirus. Proceedings of the National Academy of Sciences of the United States of America, 101(27), 10012–10017. doi:https://doi.org/10.1073/pnas.0403596101
- Yang, H., Yang, M., Ding, Y., Liu, Y., Lou, Z., Zhou, Z., … Gao, G. F. (2003). The crystal structures of severe acute respiratory syndrome virus main protease and its complex with an inhibitor. Proceedings of the National Academy of Sciences of the United States of America, 100(23), 13190–13195. doi:https://doi.org/10.1073/pnas.1835675100
- Zhou, P., Yang, X. L., Wang, X. G., Hu, B., Zhang, L., Zhang, W., … Chen, H. D. (2020). A pneumonia outbreak associated with a new coronavirus of probable bat origin. Nature, 579(7798), 270–273. doi:https://doi.org/10.1038/s41586-020-2012-7