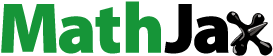
ABSTRACT
Physiological responses in seaweeds are influenced by environmental conditions such as UV radiation among other abiotic factors, concerns that must be taken into account for bioprospecting purposes. The production of UV-absorbing compounds is a well-known strategy for protecting organisms against UV radiation stress as the compounds absorb and dissipate short wavelengths. Besides their natural photoprotective sunscreen value, there is strong evidence of potential use as antioxidant, anti-inflammatory, antibacterial, antifungal, antimalarial, antiproliferative, immunomodulatory and anticancer agents. Here, we studied the relationship of potential cell damage to antioxidant activity and bioactive properties of brown seaweed Sargassum filipendula under the effect of radiation treatments: PAR (control), PAR+UVA and PAR+UVB after 10 days of exposure. Cell damage was assessed as DNA impairment by the quantification of cyclobutane pyrimidine dimers (CPDs). Antioxidant potential, tested by ABTS, DPPH, FRAP and chelating activity assays and quantification of total phenolic compounds were performed using methanolic crude extracts at different concentrations. Antiviral activity was assessed by the ability of different concentrations of methanolic and aqueous extracts to inhibit retroviral reverse transcriptase (RT) activity. PAR+UVB treatment negatively affected DNA integrity, and more CDP-DNA complexes were observed. Higher antioxidant activity was observed under PAR treatment than UVA and UVB radiation treatments. There was 25–90% inhibition of the RT enzyme for methanolic extracts, while aqueous extracts showed nearly 100% inhibition for all treatments and tested concentrations. UV-absorbing compounds in S. filipendula provide adaptive advantages against UV radiation exposure. Additionally, the species can be considered as a potential biotechnology source of natural bioactive compounds, and isolation of these compounds or obtaining bioactively rich extracts will potentially be of great benefit for the development of functional bioproducts.
Introduction
Marine organisms like marine seaweeds are constantly exposed to combined stressors such as high irradiance and UV radiation. Changes in irradiance and light quality can either promote or inhibit many of their biological processes if radiation becomes excessive, or if short wavelength radiations with high energy content such as UVB radiation are absorbed by biomolecules (Vass, Szilard, & Sicora, Citation1997). Consequently, important components of seaweed metabolism such as proteins, lipids and nucleic acids (especially cyclobutyl pyrimidine dimers and pyrimidine (6–4) pyrimidone photoproducts) are particularly affected. It has been reported for these organisms that conformation changes in the reaction centre protein (D1) of photosystem II and the CO2 fixing enzyme in the Calvin cycle lead to negative effects such as the inhibition of photosynthesis and subsequent decrease in productivity (Bischof et al., Citation2007). Two major classes of mutagenic DNA lesions induced by UV radiation are cyclobutyl pyrimidine dimers (CPDs) and 6–4 photoproducts (6–4PPs, which are pyrimidine adducts). CPDs and 6–4PPs make up around 75 and 25%, respectively, of the UV-induced DNA damage products and both classes of lesions distort the DNA helix (Britt & May, Citation2003), causing inhibition in DNA replication and transcription, with consequent disruption of cell metabolism and division (Buma, Van Oijen, Van De Poll, Veldhuis, & Gieskes, Citation2000) that could directly impair growth and survival.
To counteract the lethal effects of DNA lesions, efficient DNA repair mechanisms have been developed by the organisms, in which specialized repair proteins continuously scan the genome for the presence of DNA lesions. DNA damage by the accumulation of CPDs has been reported for all seaweed groups (Bischof, Citation2002; Roleda, Van De Poll, Hanelt, & Wiencke, Citation2004; Roleda, Wiencke, Hanelt, & Bischof, Citation2007a; Van de Poll, Eggert, Buma, & Breeman, Citation2001). For brown seaweeds, significant CPD accumulation in Saccharina latissima (as Laminaria saccharina; van de Poll, Hanelt, Hoyer, Buma, & Breeman, Citation2002), Ascoseira mirabilis (Roleda, Zacher, Wulff, Hanelt, & Wiencke, Citation2007b) and Laminaria digitata (Roleda et al., Citation2010) has been observed after exposure to UV radiation. Effective DNA repair through a repair mechanism has been reported. Physiological changes in algae in response to alteration of environmental light, temperature and nutritional conditions directly influence their chemical composition. Under non-stressing metabolic conditions, algae produce homoeostatic amounts of reactive species (free radical and non-radical species) as byproducts of the electron transport system in chloroplasts and mitochondria and other biological reactions (Trevisan et al., Citation2001). Environmental drivers, such as high sunlight or elevated exposure to UV radiation, can increase the overproduction of reactive species and overwhelm antioxidative defences of the organism, which lead to oxidative modification of biological macromolecules (lipids, proteins, DNA, among others), as well as modifications in cellular organization and ultrastructure (Holzinger & Lütz, Citation2006; Polo et al., Citation2014), cell/tissue injuries and cellular death acceleration (Trevisan et al., Citation2001).
Thus, efficient antioxidant mechanisms are essential to withstand photooxidation (Okamoto, Pinto, Latorre, Bechara, & Colepicolo, Citation2001). In the case of brown seaweeds, it is known that they are rich in natural antioxidants such as phenolic compounds (phlorotannins), carotenoids (mainly fucoxanthin) and isoprenoids. Among antioxidants, phenolic compounds, which are characterized by the presence of large multiple phenol units and widely produced among brown algae, are secondary metabolites that act as an oxidative defence mechanism in this group (Li, Wijesekara, Li, & Kim, Citation2011). The interaction of polyphenols binding to sulphated polysaccharides, another secondary metabolite with biological activity, has been investigated due to the linkage into the cell wall as an integrated mechanism of environmental defence. As reported by Salgado, Tomazetto, Cinelli, Farina, & Amado Filho (Citation2007), the strong relationship between phenolic compounds and alginates in Padina gymnospora would preserve the absorption capacity of UV radiation by phenolic compounds over time. Antioxidant substances are probably the most relevant bioactive compounds found in algae (Munir, Sharif, Naz, & Farkhanda, Citation2013) and have attracted great interest because their mechanisms of action go far beyond the modulation of oxidative stress. There is strong evidence that demonstrates the contribution of these compounds to the prevention of cardiovascular diseases and neurodegenerative diseases (Oueslati et al., Citation2012; Urquiaga & Leighton, Citation2003), with potential as anti-ageing, anti-inflammatory, antibacterial, antifungal, cytotoxic, antimalarial, antiproliferative and anticancer agents (Cornish & Garbary, Citation2010; Fujimura, Tsukahara, Moriwaki, Kitahara, & Takema, Citation2000; Thomas & Kim, Citation2011).
Besides the bioactivities mentioned above, a variety of bioactive compounds from brown seaweeds have demonstrated antiviral properties when tested against the Human Immunodeficiency Virus (HIV). These viruses are grouped into two types: HIV-type 1 (HIV-1) and HIV-type 2 (HIV-2) the former being the main agent of Acquired Immune Deficiency Syndrome (AIDS), which by 2019 had affected 38 million of people around the world (Joint United Nations Programme on HIV/AIDS; UNAIDS, Citation2019). Despite significant advances in rational drug design against HIV-1, issues such as drug resistance, side effects and the need for long-term antiviral treatment urge the development of new anti-HIV agents, targets and therapies (Worm et al., Citation2010). In this regard, marine resources can be an invaluable basis for the discovery of new bioactive compounds for the treatment of diseases like AIDS, since many have been reported to exhibit significant anti-HIV activity and are able to inhibit almost every stage of the viral infection cycle (Jiang et al., Citation2010). These compounds, which have been successfully extracted from seaweeds, include phlorotannins, lectins, steroids, sulfoglycolipids and polysaccharides; within this group, natural and synthetic sulphated polysaccharides have been the focus of most research. In the particular case of antiviral activity, inhibition of HIV-1 RT by sulphated polysaccharides from brown seaweeds has been shown to be efficient in disrupting the viral peptide attachments, which are supposed to be highly preserved in the drug-resistance mutation process (Karadeniz, Karagozlu, & Kim, Citation2015). Among sulphated polysaccharides, fucans from brown seaweed species, for example Dictyota mertensii, Lobophora variegata, Spatoglossum schroederi and Fucus vesiculosus, have been shown to inhibit successfully up to 99% of HIV RT activity (Queiroz et al., Citation2008). Knowing that stressors such as UV radiation can lead to oxidative stress in marine algae, that the presence of antioxidant substances in seaweeds acts as a defence system and that these compounds can also play a key role as bioactive ingredients and a vital role in human health, the aim of the present study is to investigate potential DNA cell damage and its relationship to antioxidant activity and bioactive properties of Sargassum filipendula C.Agardh under the radiation treatments PAR (control), PAR+UVA and PAR+UVB.
Material and methods
Algal material and acclimation conditions
Specimens of Sargassum filipendula were collected at Cigarras Beach (24°43ʹ55.74”S and 45°23ʹ54.48”W) in São Sebastião on the north coast of São Paulo State, Brazil, during the summer season (February–March 2016). The material was transported in cooler boxes to the laboratory, cleaned of macroepiphytes and washed with abundant filtered seawater. Cleaned apical portions (± 8 cm) were acclimated for one week under laboratory conditions in sterilized seawater at 32 psu enriched with von Stosch solution diluted to 50% (VS 50%) (Ursi & Plastino, Citation2001) with a PAR (photosynthetically active radiation) of 60 ± 5 μmol photons m–2 s–1, 25 ± 1°C, a photoperiod of 14 h and intermittent aeration every 30 min. The culture ratio was 3 g of alga per 1 l of culture medium.
Experimental setup
After the acclimation period, the material was exposed to three different radiation treatments: a) PAR (control treatment), b) PAR+UVA and c) PAR+UVB under the same laboratory culture conditions described above, using five biological replicates for each treatment. The experiments were performed under 3 h exposure to UV radiation per day in the middle of the light phase for 10 days. During this exposure, aeration was increased in all treatments to encourage greater movement of the algal fragments to promote a homogeneous exposure for all apical portions, and culture medium was added at the beginning of the experiment and then on the seventh day of exposure to avoid nutrient limitation. UVB (312 nm; 2.04 W m–2; 21.71 kJ m–2 day–1) and UVA (365 nm; 7.04 W m–2; 76.32 kJ m–2 day–1) radiation was provided by Philips lamps models TL 20 W/12 (Germany) and Actinic BL TL-K 40 W/10-R (Germany), respectively. The total radiation spectrum emitted by radiation sources (PAR and UV) was obtained using the SphereOptics SMS-500 (Spectral Measurements System, USA) spectroradiometer.
Oxidative DNA damage evaluation
The potential DNA damage caused by UV radiation was evaluated by the OxiSelect™ UV-Induced DNA Damage ELISA kit (CPD Quantitation; Cell Biolabs Inc., USA), according to the manufacturer’s specifications. Algal samples of 20 mg fresh weight (FW) exposed to different radiation treatments for 10 days were ground in liquid nitrogen until a fine powder was obtained. DNA extraction was done using the NucleoSpin Plant II kit (Macherey-Nagel, Germany), following the manufacturer’s instructions. The DNA samples (2 μg ml–1) were first heat-denatured before being adsorbed onto a 96-well DNA high-binding microplate. The CPDs in the sample or standard were probed with an anti-CPD antibody, followed by an HRP (Horse Radish Peroxidase) conjugated secondary antibody. Absorbance of the samples and controls was read at 450 nm using a UV–visible microplate spectrophotometer (Epoch Biotek, USA). The CPD content in the samples was determined by comparing with a standard curve prepared from predetermined CPD-DNA standards and expressed as CPD-DNA ng.
Antioxidant potential and total phenolic content
The algal extracts were prepared from samples after 10 days exposure to PAR and UV radiation. Samples of 200 mg FW were ground in liquid nitrogen, suspended in 1 ml of methanol and extracted for 3 h at room temperature, protected from light and temperature to avoid photo- and thermo-oxidation. The samples were centrifuged at 14 000 rpm for 15 min at room temperature and the supernatants were recovered. Antioxidant activity was evaluated using four different assays: a) ABTS (2,2’-azino-bis(3-ethylbenzothiazoline-6-sulphonic acid) free radical scavenging activity; b) DPPH (2,2-diphenyl-1-picrylhydrazyl) free radical scavenging activity; c) Ferric Reducing Antioxidant Power (FRAP); and d) ferrous ion-chelating ability. The total phenolic content was determined according to the Folin–Ciocalteu method. Each assay was analysed in six concentrations of the algal methanolic extract (2, 4, 6, 8, 10 and 12 mg ml–1). The half-maximal effective concentration (EC50), which is the concentration of the sample to reach 50% of antioxidant activity, was calculated using dose–response curves with the software GraphPad Prism v. 6.0 and hyperbolic dose–response adjustment model. Since the analysis was performed by using extracts from fresh biomass, data were transformed to mg GAE g–1 of dry algal extracts based on the extract yield of Sargassum species, which corresponds to 12% (Khaled, Hiba, & Asma, Citation2012). The standard equivalent was calculated from the calibration curves of gallic acid and Trolox and expressed as gallic acid equivalent (GAE) based on the dry mass of the extract (mg GAE g−1) ().
Table 1. Summary of the parameters of the respective standard curves for the different antioxidant assays, specifying the standard concentration range (μg ml–1), linear function (y = axe + b), regression coefficient (R2) and conversion factor (CF) of the standard in relation to gallic acid.
ABTS free radical scavenging activity
The antioxidant assay by ABTS free radical capture was performed according to Torres, Pires, Santos, and Chow (Citation2017), modified by Rufino et al. (Citation2007) and Yang et al. (Citation2011). The reaction assay was prepared by mixing 280 μl of ABTS+ solution and 20 μl of algal extract; after incubation for 20 min at room temperature and light-protected, the absorbance was read at 734 nm.
DPPH free radical scavenging activity
The antioxidant assay by DPPH-free radical scavenging activity was determined according to Pires, Torres, Dos Santos, & Chow (Citation2017a), modified by Brand-Williams, Cuvelier, & Berset (Citation1995). The reaction mixture was prepared with 280 μl of DPPH solution and 20 μl of algal extract; after incubation for 30 min at room temperature and light-protected, the absorbance was read at 517 nm.
Ferric Reducing Antioxidant Power (FRAP)
The antioxidant activity through the FRAP assay was evaluated following the method described in Urrea-victoria, Pires, Torres, & Santos (Citation2016), modified by Rufino, Alves, Brito, & Morais (Citation2006). For the reaction mixture, the FRAP solution (265 μl) was mixed with 20 μl of algal extract and 15 μl of ultrapure water, incubated for 30 min at 37°C and light-protected, and then, the absorbance was read at 595 nm.
Ferrous ion-chelating ability
The antioxidant activity through the ferrous ion-chelating ability was evaluated according to Harb, Torres, Pires, Santos, and Chow (Citation2016), modified by Min, McClung, and Chen (Citation2011). The reaction mixture was prepared with 250 μl of ammonium acetate solution 10%, 15 μl of ammonium sulphate solution (2 mM) and 20 μl of algal extract and then incubated for 5 min at room temperature and light-protected. After incubation, 15 μl of the ferrozine solution (6.1 mM) was added and incubated for additional 10 min with shaking at 100 rpm and the absorbance was read at 562 nm.
Total phenolic compounds
This procedure was performed as in Pires, Torres, Santos, & Chow (Citation2017b), modified by Waterman & Mole (Citation1994). The reaction mixture was prepared by mixing 200 μl of ultrapure water, 20 μl of algal extract, 20 μl of Folin–Ciocalteu reagent 2 N and 60 μl of Na2CO3 at 10 mg ml–1, incubated for 30 min at room temperature and light-protected, and then, the absorbance was read at 760 nm.
Overall Antioxidant Potency Composite Index (OAPCI)
This index was calculated based on Seeram et al. (Citation2008) by using the obtained values of antioxidant assays for the EC50. An overall antioxidant potency composite index was determined by assigning all assays an equal weight, fixing an index value of 1 to the lowest value of EC50 for each assay and then calculating an index score for all other samples within the test as follows:
The OAPCI was calculated for each assay taking into account all treatments and for each type of radiation treatment considering an estimation for all assays. The data were expressed as 1/EC50, meaning that a higher value represents a better index.
Antiviral activity
Antiviral activity of S. filipendula under UV radiation treatments for 10 days was assessed by the ability to inhibit the activity of the enzyme HIV-1 reverse transcriptase (HIV-1 RT) using the colorimetric kit for the RT assay (Roche, Germany) and based on Woradulayapinij, Soonthornchareonnon, & Wiwat (Citation2005). Two kinds of algal extracts were tested: a) Methanolic extract: samples of 200 mg FW were grounded in liquid nitrogen, suspended in 1.2 ml of methanol and extracted for 3 h at room temperature. The samples were centrifuged at 8 000 rpm for 5 min at room temperature and the supernatant was recovered into a new microtube. This procedure was repeated twice and the final methanolic extract was concentrated at 40°C. b) Aqueous extract: the precipitated material obtained after methanolic extraction was back-extracted with 2.4 ml of ultrapure water for 3 h at 80°C and the supernatant was recovered into a new microtube. This procedure was repeated twice and the final aqueous extract was concentrated by freeze-drying for three days. Samples were diluted in 10% DMSO and ultrapure DEPEC-treated water added to reach the desired concentrations. Inhibition of the enzyme HIV-1 RT was tested at concentrations of 200, 400, 600 and 800 μg ml–1 for the methanolic extract and 50, 100, 150 and 200 μg ml–1 for the aqueous extract. The reaction mixture was obtained by adding 20 μl of samples or standards, 19 μl of lysis buffer, 20 μl oligo (dT)15 + polyA and 1 μl of the HIV-1 RT enzyme, in a 200 μl microtube. 10% DMSO without the enzyme was used as the blank and 10% DMSO with addition of the enzyme as the negative control. After incubation for 1 h at 37°C, the solution was transferred to the reaction modules provided by the kit and remained for another 1 h at 37°C. After this incubation period, the modules were washed with wash buffer. The mixture prepared with buffer and antibody (200 μl) was added to the wells and incubated for 1 h at 37°C. After incubation, the modules were washed with wash buffer and 200 μl of ABTS solution added. Absorbance at 405 and 490 nm was read with an UV–visible microplate spectrophotometer (Epoch Biotek, USA). Two controls were used: (a) 10% DMSO without sample (negative control) and (b) Foscarnet with the addition of the RT enzyme (positive control). A calibration curve was made with Foscarnet, a positive control, at final concentrations of 0.0625, 0.125, 0.25, 0.5, 1, 2 and 4 μg ml−1. The results of the positive control and extracts were as the half inhibitory concentration (IC50) which was calculated using dose–response curves with software GraphPad Prism v. 6.0 and hyperbolic dose–response adjustment model.
Data analysis
Statistical analysis of the data set was performed with the STATISTICA software (version 10.0). Five replicates were used for all studied parameters. Data were checked for normality (Kolmogorov–Smirnov test) and homoscedasticity (Bartlett test) and then submitted to unifactorial or multifactorial (ANOVA), followed by the Newman–Keuls post hoc test to verify the significance of the differences (p < 0.05). Additionally, Pearson’s correlation was performed for the antioxidant activity and total phenolic content to evaluate the interactions between both parameters. Correlation grade was established according to Callegari-Jacques (Citation2003) as follows: 0: null; 0–0.3: weak; 0.3–0.6: regular; 0.6–0.9: strong; 0.9–1: very strong; 1: plain.
Results
Oxidative DNA damage evaluation
The oxidative DNA damage was assessed by the quantification of cyclobutane pyrimidine dimers (CPDs). presents the CPD-DNA levels (ng) of S. filipendula after 10 days of exposure to PAR, PAR+UVA and PAR+UVB radiations. No changes were observed when comparing the PAR and PAR+UVA treatments, with CPD values of 0.034 ± 0.010 ng and 0.088 ± 0.030 ng, respectively. Nevertheless, the samples treated with PAR+UVB showed differences, with a notable increase in the CDP-DNA content (2.11 ± 0.82 ng).
Antioxidant potential and total phenolic content
Antioxidant activities from S. filipendula extracts using diverse antioxidant assays (except for ferrous ion-chelating ability) after UV radiation exposure are presented in , expressed as EC50 (mg ml–1) and total phenolic content as mg GAE g–1. Dose-dependency was observed for all assays, where the lowest (2 mg ml–1) and highest (12 mg ml–1) concentrations of crude extracts had the lowest and the highest antioxidant activity, respectively. The PAR treatment showed the lowest EC50 values, which represents the major efficiency of antioxidant potential, and the highest total phenolic content (TPC), followed by PAR+UVA and PAR+UVB treatments (). EC50 data for ABTS assay was 0.62 ± 0.03 mg ml–1, followed by PAR+UVA with 0.73 ± 0.03 mg ml–1 and PAR+UVB with 0.83 ± 0.04 mg ml–1. A similar trend was obtained for DPPH assays with EC50 values of 0.76 ± 0.02 mg ml–1 (PAR), 0.89 ± 0.02 mg ml–1 (PAR+UVA) and 1.33 ± 0.07 mg ml–1 (PAR+UVB), representing the following radical scavenging order: PAR > PAR+UVA > PAR+UVB (). EC50 values for the FRAP assay also showed differences between radiation treatments, where PAR had the best antioxidant efficiency (0.61 ± 0.07 mg ml–1), followed by PAR+UVA (0.68 ± 0.05 mg ml–1) and then by PAR+UVB (1.09 ± 0.09 mg ml–1) (). Finally, no differences were observed between radiation treatments for the ferrous ion-chelating ability assay, and since the extracts did not reach 50% of antioxidant activity by this assay, EC50 values were not calculated. Conversely, none of the extracts showed comparable activity to the gallic acid standard for ABTS (0.0010 mg ml−1), DPPH (0.0016 mg ml−1) and FRAP (0.0025 mg ml−1). Differences in total phenolic content were observed between radiation treatments, where PAR showed the highest values (10.00 ± 0.11 mg GAE g–1), followed by PAR+UVA (8.16 ± 0.10 mg GAE g–1) and PAR+UVB (7.16 ± 0.09 mg GAE g–1).
Table 2. Antioxidant activity and total phenolic content (TPC) for Sargassum filipendula after 10 days of UV radiation exposure. The values are expressed in mean ± SD (n = 5) based on EC50 value (half-effective concentration) for ABTS, DPPH and FRAP assay or gallic acid equivalent (GAE) on algal extract for chelating assay and TPC. Letters indicate differences between the means according to the unifactorial ANOVA, for each assay separately, and Newman–Keuls post hoc test (p < 0.05). n.c.: not calculated.
Pearson’s correlations between the antioxidant potential and total phenolic content in S. filipendula extracts are shown in for PAR ), PAR+UVA ) and PAR+UVB ). By comparing the TPC for the PAR treatment with all the assays, it was possible to observe a strong correlation between them, showing the highest values for ABTS (R = 0.89), followed by FRAP (R = 0.86), metal chelating activity (R = 0.83) and DPPH (R = 0.77) ). A strong correlation between TPC and antioxidant activity was also observed for PAR+UVA, except for the DDPH assay, which had a normal correlation value (R = 0.41) ). PAR+UVB samples showed a similar correlation trend to PAR+UVA treatment for antioxidant activity and TPC; however, for the DPPH assay, a negative correlation was observed for this treatment (R = −0.56) ).
Figure 2. Pearson correlation matrix (R; p < 0.05) between total phenolic content (TPC) and antioxidant activity assays (mg GAE g−1) of Sargassum filipendula after 10 days of UV radiation exposure. a) PAR treatment; b) PAR+UVA treatment; and c) PAR+UVB treatment.
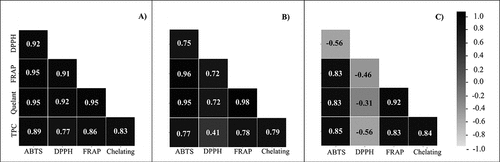
Additionally, total phenolic compounds in S. filipendula under PAR treatment were compared with literature values for other Sargassum species where similar results were found (). As complementary data, the OAPCI (overall antioxidant potency composite index) was calculated for each antioxidant assay and radiation treatment (). The OAPCI values were calculated based on the EC50 for ABTS, DPPH and FRAP assays and as (mg GAE g–1) for chelating and TPC. Values were expressed as 1/EC50, therefore a lower index means lower potency for the assay. The lowest index per radiation was registered for PAR+UVB treatment (index 0.72), followed by PAR+UVA (0.79) and PAR (1.00). In relation to the antioxidant assays, chelating was the most sensitive assay (index = 0.91) followed by ABTS (0.87), TPC (0.84), FRAP (0.82) and finally DPPH (0.81).
Table 3. Total phenolic content of Sargassum filipendula after 10 days of UV radiation exposure and a summary obtained from other authors for Sargassum species. Data are expressed in mean ± SD (n = 5) as gallic acid equivalent and mass of dry algal extract (mg GAE g−1).
Table 4. Overall antioxidant potency composite index based on EC50 values of Sargassum filipendula after 10 days of UV radiation exposure. The index was calculated for each antioxidant assay and then used to calculate the Index for assay (as the mean of columns) and the Index for radiation (as the mean of lines).
Inhibition of HIV-1 reverse transcriptase
After 10 days of three different radiation treatments (PAR, PAR+UVA and PAR+UVB), extracts from S. filipendula showed inhibitory effects against HIV-1 RT by using different concentrations of methanolic and aqueous extracts. The methanolic extracts showed differences between concentrations of algal crude extracts when analysing each radiation treatment independently, presenting a dose-dependent response (data not shown). No stimulation of the antiviral activity was observed for samples treated with UV (). For the methanolic extracts, higher efficiency was observed for the PAR treatment, which had an IC50 of 264.77 ± 15.07 µg ml–1 and among UV extracts, PAR+UVB had better efficiency than PAR+UVA, showing values of 417.40 ± 6.50 µg ml–1 and 589.70 ± 56.14 µg ml–1, respectively (PAR+UVA > PAR+UVB > PAR) (), recalling that lower IC50 values mean better efficiency of the extracts.
Table 5. IC50 (half-inhibitory concentration; μg ml−1) for crude methanolic extracts of Sargassum filipendula after 10 days of exposure to different laboratory radiation treatments against the activity of HIV-1 reverse transcriptase (HIV-1 RT) enzyme. Values are expressed in mean ± SD (n = 3). Letters indicate differences according to one-way ANOVA and Newman–Keuls post-hoc test (p < 0.05).
A high antiviral activity for the aqueous extracts was observed for all the tested samples, including those treated with UV radiation. Even with the lowest extract concentration (50 μg ml−1), all the extracts had close to 100% efficiency so the IC50 was not calculated.
Discussion
Sargassum species are found in the lower intertidal zone and the subtidal zone and constitute an important ecosystem engineer for diverse marine communities. As many species of Sargassum inhabit the intertidal, they are commonly exposed to fluctuating adverse air-water stressors. UV radiation is expected to increase with human population growth and development and global climate changes. Because of the highly deleterious effect of UV radiation, it is particularly relevant for these important seaweeds in tropical and subtropical regions to understand the biological mechanisms underlying acclimation, sensitivity, tolerance and repair.
Oxidative DNA damage evaluation
Cyclobutane pyrimidine dimer (CPD) formation is a DNA mutagenic lesion typically reported after UV radiation exposure of several brown seaweeds, responsible for the inhibition in replication of DNA and transcription and consequent disruption of cell metabolism and division, affecting growth and survival. In the present study, after 10 days, the moderate UVB radiation dose used here increased CPD levels in S. filipendula by 35 times in relation to PAR and PAR+UVA treatments, meaning that the DNA was negatively affected by this factor. The same trend has been reported for the early stages (gametes and zoospores) of the brown seaweeds Saccharina latissima, Laminaria digitata, L. hyperborea and Ascoseira mirabilis which have a greater susceptibility to UV exposure than adult stages (Roleda et al., Citation2010, Roleda, Wiencke, Hanelt, Van De Poll, & Gruber, Citation2005; Roleda et al., Citation2007b; van de Poll et al., Citation2002). Mechanisms of DNA repair, like the enzyme photolyase, are essential dynamic tools for life and for evolution. Specialized repair proteins trigger efficient DNA repair processes, which restore genetic information, reverse the damage and remove the DNA lesions caused by UV radiation (Essen & Klar, Citation2006). Our results did not evaluate a post-exposure recovery period; however, since S. filipendula was exposed to a moderate UV dose, we would expect gradual recovery by the action of lesion recognition proteins.
Antioxidant potential and total phenolic content
Excess UV radiation has been reported to increase the production of reactive species. Complexes I and II of the respiratory chain favour the generation of mitochondrial reactive oxygen species (ROS) under exposure to this factor (Turrens, Citation1997; Turrens & Boveris, Citation1980) leading to oxidative damage. As a photoprotective mechanism to prevent and ameliorate oxidative damage caused by UV radiation, seaweeds improve the production of UV-absorbing compounds, serving as sunscreen (Ruhland, Fogal, Buyarski, & Krna, Citation2007).
High antioxidant activity was observed in the crude extracts of S. filipendula, results that are in agreement with diverse literature, in which Sargassum species tend to have high antioxidant potential and are expected to develop a very effective antioxidant defence system due to strong UV radiation (Budhiyanti, Raharjo, Marseno, & Lelana, Citation2012; Demirel, Yilmaz-Koz, Karabay-Yavasoglu, Ozdemir, & Sukatar, Citation2009; Lim, Cheung, Ooi, & Ang, Citation2002; Santos et al., Citation2019; Zubia et al., Citation2009; Zubia, Robledo, & Freile-Pelegrin, Citation2007). The capability of S. filipendula to synthesize antioxidant compounds enhances its tolerance, which enables the maintenance of all metabolic processes and survival in the presence of UV radiation. The EC50 values for S. filipendula showed a better index for PAR treatment in all antioxidant assays, evidencing the active action of the antioxidant system in the species under non-stressing conditions and could be attributed to phenolic compounds. However, when S. filipendula was submitted to UV radiation, EC50 was higher for the samples exposed to this stress. Nevertheless, we must take into account that besides the accumulation of phenolic compounds to counteract the effects of UV radiation, the species could also exude these compounds into the surrounding medium to create an UV-absorbing microenvironment (Roleda et al., Citation2010). This behaviour can be an additional photoprotective mechanism, which could explain the lower efficiency of these extracts since this phenomenon reduces the content of these compounds inside the cells. Comparing data for total phenolic compounds (mg GAE g−1) in several Sargassum species, our results were similar to those for other species such as S. vulgare (Santos et al., Citation2019),S. furcatum (Vasconcelos et al., Citation2018), S. plagiophyllum (Chakraborty, Maneesh, & Makkar, Citation2017), S. linifolium (Ismail, Citation2017) and S. marginatum (Vinayak, Sabu, & Chatterji, Citation2011), respectively 10.84 ± 1.24 mg GAE g−1, 17.00 ± 3.00 mg GAE g−1, 7.48 ± 0.02 mg GAE g−1, 10.35 ± 0.92 mg GAE g−1 and 13.19 ± 0.41 mg GAE g−1. Other species as S. linearifolium (Dang, Bowyer, Van Altena, & Scarlett, Citation2018), S. muticum (Lee & Kim, Citation2015) and S. siliquastrum had higher phenolic contents than S. filipendula, 47.06 ± 0.65 mg GAE.g−1, 37.98 ± 0.53 mg GAE.g−1 and 127.37 mg GAE.g−1, respectively. Phenolic content can vary depending on the species and several biotic factors (reproductive stages, age, herbivory) and abiotic factors (sun exposure, depth, salinity), modulating the accumulation of phlorotannins (Connan, Delisle, Deslandes, & Ar Gall, Citation2006). When comparing the EC50 values obtained by ABTS and DPPH assays (methods based on radical scavenging activity), differences were observed between other Sargassum species. Here, we reported EC50 values of ~0.68 mg ml–1, ~0.8 mg ml–1 and ~1.0 mg ml–1 for PAR, PAR+UVA and PAR+UVB, respectively, lower than those in Vinayak et al. (Citation2011), where EC50 from methanolic extracts was 2.87 mg ml–1 for S. marginatum. Higher EC50 values were also reported by Zubia et al. (Citation2007) for ethanolic extracts from S. ramifolium and S. pteropleuron, 6.64 mg ml–1 and 7.14 mg ml–1, respectively. These findings demonstrate that even under UV radiation treatments, S. filipendula extracts displayed characteristics of an efficient antioxidant. Additionally, ABTS+ and DPPH activities might relate to the presence of compounds with multiple -OH groups and/or centres of unsaturation, which enable them to donate a proton to a free radical by hydrogen atom transfer (HAT) to deactivate the free radicals, as discussed by Maneesh, Chakraborty, & Makkar (Citation2017) when analysing antioxidant activity in methanolic/ethyl acetate extracts in S. wightii. Further analysis showed a strong correlation between phenolic content and the DPPH radical scavenging activity for PAR (R = 0.77, p < 0.05) suggesting the presence of phenolic compounds with antioxidant action. Our data are in accordance with those of other authors who have shown that a high total polyphenol content increases antioxidant function and that there is a linear correlation between polyphenol content and antioxidant function (Gorinstein et al., Citation2003). For PAR+UVA treatment, this correlation was lower (R = 0.41, p < 0.05), indicating that S. filipendula contains complex mixtures of various types of bioactive compounds such as the terpenoid-quinones sargaquinoic acid and sargachromenol, and the steroid fucosterol, capable of deactivating DPPH and ABTS+ radicals, as reported for other Sargassum species (Matanjun, Mohamed, Mustapha, Muhammad, & Ming, Citation2008; Wang, Jónsdóttir, & Ólafsdóttir, Citation2009). Moreover, extracts from the PAR+UVB treatment showed a negative correlation (R = – 0.56, p < 0.05), between total phenolic content and the DPPH assay, which could be related to the exudation of phenolic compounds into the water as previously reported in brown seaweed species (Celis-Plá et al., Citation2018; Polo et al., Citation2014; Polo & Chow, Citation2020; Roleda et al., Citation2010). A significant correlation between the DPPH radical-scavenging activity and the reducing power assays was also observed for PAR (R = 0.91, p < 0.05) and PAR+UVA (R = 0.72, p < 0.05) treatments, which is explained by the fact that both assays rely on a mechanism of electron/hydrogen donation (Zubia et al., Citation2009). Potential Fe2+ ion chelating ability has been reported for brown seaweeds and attributed to phenolic compounds such as phlorotannins (Karawita et al., Citation2005). Despite low activity reported by S. filipendula in all treatments when testing ferrous ion-chelating ability, a positive correlation with TPC was also observed within the treatments, probably related to phenolic moieties which bond with Fe2+, diminishing the redox potential and balancing out the oxidized type of Fe2+ (Maneesh et al., Citation2017). When analysing all assays for the antioxidant activity of extracts from S. filipendula, it was observed that chelating and ABTS methods based on radical scavenging activity presented the highest OAPCI index. In the case of TPC, a lower index was obtained. Differences could be related to the pH reactivity condition of the sample compounds; it has been reported that the ABTS index showed higher sensitivity at neutral pH, compared to the assays with acidic (FRAP) or alkaline (Folin–Ciocalteu) reaction pHs (Apak, Özyürek, Güçlü, & Çapanoʇlu, Citation2016). The OAPCI index is a useful parameter to assess the total antioxidant property of a complex sample with diverse metabolites since it integrates the activity detected from different assays. As secondary metabolites have multiphase antioxidant action, it is recommended to use different assays with diverse chemical reactivity. In the present study, four different antioxidant methods were tested. Various assays have been developed due to the complexity and diversity of this research area, none of which has gained universal acceptance. Thus, a major challenge in this kind of investigation is to determine which method is best suited for a particular future application, knowing that antioxidants may exert their effect through various mechanisms such as scavenging radicals, sequestering transition metal ions, decomposing hydrogen peroxide or hydroperoxides, quenching active prooxidants and repairing biological damage (Niki & Noguchi, Citation2000).
Inhibition of HIV-1 reverse transcriptase
Another highlight in relation to the effect of UV radiation is the activation or suppression of secondary metabolites with different biological activity, such as antiviral activity. Methanolic extracts of S. filipendula showed values of RT inhibition ranging from 25% to 90%. Similar results were reported by Ahn et al. (Citation2004) for some species of Sargassum, when testing the same extraction solvent. Nevertheless, the efficiency of methanolic extracts decreased when samples were treated with UV radiation. Currently, there is no evidence that confirms the effects of UV radiation on the antiviral activity of seaweed. However, the low inhibition observed in S. filipendula extracts after exposure to UV could be related to the exudation and migration through the cell wall to the seawater of phenolic compounds, more specifically phlorotannins, in response to the stimuli caused by this type of radiation (Polo & Chow, Citation2020; Polo et al., Citation2014), reducing these compounds inside the algae. Despite the lower inhibition reached by methanolic extracts of samples exposed to UV radiation, we suggest that phenolic compounds could be responsible for this activity, since it has been reported that phlorotannins can inhibit the polymerase and ribonuclease activities of HIV-1 RT (Ahn et al., Citation2006, Citation2004). Some researchers have been focused on the isolation of these compounds from brown algae in order to study the potential to inhibit HIV-1 RT. Ahn et al. (Citation2004) isolated two phlorotannins from Ecklonia cava: 8,8’-bieckol and 8,4”’-dieckol that are dimers of eckol. Both compounds presented inhibition against HIV-1 RT with IC50 values of 0.0005 μg ml−–1 and 0.0053 μg ml–1, respectively. Since the 8,8’-bieckol showed higher activity, it has been suggested that it might be employed as a drug candidate for the development of new generation therapeutic agents against HIV. Another phlorotannin isolated from the same species is 6,6’-bieckol, which has presented inhibition of HIV-1 RT with an IC50 value of 0.00107 μg ml–1 and reduction of cytopathic effects; thus, having potential as a safe therapeutic agent (Artan et al., Citation2008). In relation to these studies, S. filipendula had IC50 values of 238.97, 644.01 and 372.94 μg ml–1 for PAR, PAR+UVA and PAR+UVB, respectively. However, the inhibition of HIV-1 RT tested here was by crude extracts, without any fractioning, pre-extraction or purification processes that could cleanse or concentrate the most active metabolites, and even so, it was possible to reach a high level of inhibition. For aqueous extracts of S. filipendula, HIV-1 RT inhibition reached almost 100% for all samples at all tested extract concentrations. It has been reported that aqueous extracts and fractions of seaweed can be activated through various phases of HIV replication, such as in the case of the Carraguard® microbicide gel obtained from red algae (Cutler & Justman, Citation2008) and aqueous fractions of S. swartzii (Dinesh et al., Citation2016). This activity could be attributed to brown seaweed’s sulphated polysaccharides, e.g., fucoidan, alginate and laminaran, compounds containing ester sulphate groups in their sugar residues which are rich constituents of the seaweed cell wall that represents more than 50% of dry weight (Wang, Wang, & Guan, Citation2012). Several authors have reported that antiviral activity of macroalgae aqueous extracts is associated with the presence of these compounds (Kremb et al., Citation2014; Thuy et al., Citation2015; Zaid, Abdel-Wahab, & Abed, Citation2017), acting as virus adsorption blockers in the host cell (De Clercq, Citation2000), and as competitive inhibitors, preventing the connection between the reverse transcriptase enzyme and the reverse transcription initiator (Viennois, Chen, Laroui, Baker, & Merlin, Citation2013). Additionally, sulphated polysaccharides have attracted attention due to many beneficial biological activities such as anticoagulant (Mao et al., Citation2009), anticancer (Synytsya et al., Citation2010; Zandi et al., Citation2010), anti-inflammatory (Na et al., Citation2010) and antiviral activity (Schaeffer & Krylov, Citation2000). Isolated sulphated polysaccharides from Sargassum patens (Zhu, Chiu, Ooi, Chan, & Ang, Citation2004), S. tenerrimum (Sinha, Astani, Ghosh, Schnitzler, & Ray, Citation2010) and S. trichophyllum (Lee, Takeshita, Hayashi, & Hayashi, Citation2011) have been tested for antiviral activity, showing promising results against retroviruses. Thus, they are a very important resource for the development of novel drugs. Despite the promising advances obtained in various in vitro and in vivo assays, clinical trials with these organisms regarding a human scenario are still required in order to complete the research cycle (Brown et al., Citation2014). Our results showed UV radiation can induce the production of CPDs in S. filipendula; however, further studies need to test repair mechanisms that could reverse DNA damage, together with the measurement of antioxidant enzymes that may give a fuller panorama of the adaptation to high solar radiation. The phenolic compounds within the extracts are important characteristics responsible for the evolutionary success of the group, playing a vital role in scavenging free radicals and assuring protection from photoxidation. This research will be important in understanding the ecological implications under a scenario of climate change due to stratospheric ozone depletion and global warming. Even without stimulation of antiviral activity by UV radiation, efficient inhibition against HIV-1 reverse transcriptase was observed for methanolic and aqueous extracts at different concentrations, confirming the elevated bioactivity of S. filipendula. Thus, we can suggest that the species could be a potential rich source of a natural antiviral and further isolation of compounds will help us to understand the mechanisms behind the antiviral activity observed. This type of initial screening of potential compounds suitable for the development of nutraceutical, cosmeceutical and pharmaceutical products is invaluable.
Impact statements
The present manuscript is framed within the United Nations Sustainable Development Goal 14. Life below water: “Conserve and sustainably use the oceans, seas and marine resources for sustainable development”. Nowadays the importance of UV radiation effects is recognized for determining the distribution and abundance of organisms such as seaweeds in aquatic ecosystems. This feature is of great importance for species within the genus Sargassum, which are important community structuring organisms in tropical and subtropical marine habitats. In their natural distribution, Sargassum species are exposed to high radiation levels with possible consequences such as oxidative stress, resulting in several vital metabolic changes. Moreover, species of this genus are rich in bioactive compounds, indicating their potential as an important source of products that can contribute to consumer well-being, as a part of new functional foods and a resource for the development of novel drugs.
Acknowledgments
L.K. Polo thanks CAPES (Coordination for the Improvement of Higher Education Personnel, Brazil; 2014/2326859) and FAPESP (São Paulo Research Foundation, Brazil; 2016/03095-0) for the PhD fellowships. F. Chow acknowledges the FAPESP research grant (Biota/FAPESP 2013/50731-1) and the CNP research productivity grant (National Council for Scientific and Technological Development, Brazil; 303937/2015-7).
Disclosure statement
No potential conflict of interest was reported by the author(s).
Additional information
Funding
References
- Ahn, M. J., Yoon, K. D., Kim, C. Y., Kim, J. H., Shin, C. G., & Kim, J. (2006). Inhibitory activity on HIV-1 reverse transcriptase and integrase of a carmalol derivative from a brown Alga,Ishige okamurae. Phytotherapy Research, 20, 711–713.
- Ahn, M.-J., Yoon, K.-D., Min, S.-Y., Lee, J. S., Kim, J. H., Kim, T. G., … Kim, J. (2004). Inhibition of HIV-1 reverse transcriptase and protease by phlorotannins from the brown alga Ecklonia cava. Biological and Pharmaceutical Bulletin, 27, 544–547.
- Apak, R., Özyürek, M., Güçlü, K., & Çapanoʇlu, E. (2016). Antioxidant activity/capacity measurement. 1. Classification, physicochemical principles, mechanisms, and electron transfer (ET)-based assays. Journal of Agricultural and Food Chemistry, 64, 997–1027.
- Artan, M., Li, Y., Karadeniz, F., Lee, S. H., Kim, M. M., & Kim, S. K. (2008). Anti-HIV-1 activity of phloroglucinol derivative, 6,6′-bieckol, from Ecklonia cava. Bioorganic & Medicinal Chemistry, 16, 7921–7926.
- Bischof, K. (2002). Effects of solar UV-B radiation on canopy structure of Ulva communities from southern Spain. Journal of Experimental Botany, 53, 2411–2421.
- Bischof, K., Gómez, I., Molis, M., Hanelt, D., Karsten, U., Lüder, U., … Wiencke, C. (2007). Ultraviolet radiation shapes seaweed communities. Life in Extreme Environments, 2, 187–212.
- Brand-Williams, W., Cuvelier, M. E., & Berset, C. (1995). Use of a free radical method to evaluate antioxidant activity. LWT - Food Science and Technology, 28, 25–30.
- Britt, A. B., & May, G. D. (2003). Re-engineering plant gene targeting. Trends in Plant Science, 8, 90–95.
- Brown, E. M., Allsopp, P. J., Magee, P. J., Gill, C. I., Nitecki, S., Strain, C. R., & Mcsorley, E. M. (2014). Seaweed and human health. Nutrition Reviews, 72, 205–216.
- Budhiyanti, S. A., Raharjo, S., Marseno, D. W., & Lelana, I. Y. B. (2012). Antioxidant activity of brown algae Sargassum species extracts from the coastline of Java island. American Journal of Agricultural and Biological Sciences, 7, 337–346.
- Buma, A. G. J., Van Oijen, T., Van de Poll, W., Veldhuis, M. J. W., & Gieskes, W. W. C. (2000). The sensitivity of Emiliania huxleyi (Prymnesiophyceae) to ultraviolet-b radiation. Journal of Phycology, 36, 296–303.
- Callegari-Jacques, S. M. (2003). Bioestatística: Princípios e aplicações (First ed.). Artmed, Porto Alegre.
- Celis-Plá, P. S. M., Brown, M. T., Santillán-Sarmiento, A., Korbee, N., Sáez, C. A., & Figueroa, F. L. (2018). Ecophysiological and metabolic responses to interactive exposure to nutrients and copper excess in the brown macroalga Cystoseira tamariscifolia. Marine Pollution Bulletin, 128, 214–222.
- Chakraborty, K., Maneesh, A., & Makkar, F. (2017). Antioxidant activity of brown seaweeds. Journal of Aquatic Food Product Technology, 26, 406–419.
- Cho, S., Kang, S., Cho, J., Kim, A., Park, S., Hong, Y., & Ahn, D. (2007). The antioxidant properties of brown seaweed (Sargassum siliquastrum) extracts. Journal of Medicinal Food, 10, 479–485.
- Connan, S., Delisle, F., Deslandes, E., & Ar Gall, E. (2006, Mar 49). Intra-thallus phlorotannin content and antioxidant activity in Phaeophyceae of temperate waters. Botanica Marina, 49. doi:10.1515/BOT.2006.005
- Cornish, M. L., & Garbary, D. J. (2010). Antioxidants from macroalgae: Potential applications in human health and nutrition. Algae, 25, 155–171.
- Cutler, B., & Justman, J. (2008). Vaginal microbicides and the prevention of HIV transmission. The Lancet Infectious Diseases, 8, 1–23.
- Dang, T. T., Bowyer, M. C., Van Altena, I. A., & Scarlett, C. J. (2018). Comparison of chemical profile and antioxidant properties of the brown algae. International Journal of Food Science & Technology, 53, 174–181.
- De Clercq, E. (2000). Current lead natural products for the chemotherapy of human immunodeficiency virus (HIV) infection. Medicinal Research Reviews, 20, 323–349.
- Demirel, Z., Yilmaz-Koz, F., Karabay-Yavasoglu, U., Ozdemir, G., & Sukatar, A. (2009). Antimicrobial and antioxidant activity of brown algae from the Aegean sea. Journal of the Serbian Chemical Society, 74, 619–628.
- Dinesh, S., Menon, T., Hanna, L. E., Suresh, V., Sathuvan, M., & Manikannan, M. (2016). In vitro anti-HIV-1 activity of fucoidan from Sargassum swartzii. International Journal of Biological Macromolecules, 82, 83–88.
- Essen, L. O., & Klar, T. (2006). Light-driven DNA repair by photolyases. Cellular and Molecular Life Sciences, 63, 1266–1277.
- Fujimura, T., Tsukahara, K., Moriwaki, S., Kitahara, T., & Takema, Y. (2000). Effects of natural products extracts on contraction and mechanical properties of fibroblast populated collagen gel. Biological and Pharmaceutical Bulletin, 23, 291–297.
- Gorinstein, S., Martin-Belloso, O., Katrich, E., Lojek, A., Číž, M., Gligelmo-Miguel, N., … Trakhtenberg, S. (2003). Comparison of the contents of the main biochemical compounds and the antioxidant activity of some Spanish olive oils as determined by four different radical scavenging tests. The Journal of Nutritional Biochemistry, 14, 154–159.
- Harb, T. B., Torres, P. B., Pires, J. S., Santos, D. Y. A. C., & Chow, F. (2016).Ensaio em microplaca do potencial antioxidante através do sistema quelante de metais para extratos de algas. Instituto de Biociências. Universidade de São Paulo, 1–5. 978-85-85658-63-2.
- Holzinger, A., & Lütz, C. (2006). Algae and UV irradiation: Effects on ultrastructure and related metabolic functions. Micron, 37, 190–207.
- Ismail, G. A. (2017). Biochemical composition of some Egyptian seaweeds with potent nutritive and antioxidant properties. Food Science and Technology, 37, 294–302.
- Jiang, Y., Ng, T. B., Wang, C. R., Zhang, D., Cheng, Z. H., Liu, Z. K., … Liu, F. (2010). Inhibitors from natural products to HIV-1 reverse transcriptase, protease and integrase. Mini-reviews in Medicinal Chemistry, 10, 1331–1344.
- Karadeniz, F., Karagozlu, M. Z., & Kim, S. (2015). Antiviral activities of marine algal extracts. In S.-K. Kim & K. Chojnacka (Eds.), Marine Algae extracts: Processes, products, and applications (pp. 371–379). Weinheim: Wiley-VCH Verlag GmbH & Co. KGaA.
- Karawita, R., Siriwardhana, N., Lee, K. W., Heo, M. S., Yeo, I. K., Lee, Y. D., & Jeon, Y. J. (2005). Reactive oxygen species scavenging, metal chelation, reducing power and lipid peroxidation inhibition properties of different solvent fractions from Hizikia fusiformis. European Food Research and Technology, 220, 363–371.
- Khaled, N., Hiba, M., & Asma, C. (2012). Antioxidant and antifungal activities of Padina pavonica and Sargassum vulgare from the Lebanese Mediterranean coast. Advances in Environmental Biology, 6, 42–48.
- Kremb, S., Helfer, M., Kraus, B., Wolff, H., Wild, C., Schneider, M., … Brack-Werner, R. (2014). Aqueous extracts of the marine brown alga Lobophora variegata inhibit HIV-1 infection at the level of virus entry into cells. PLoS One, 9, 1–12.
- Lee, J. H., & Kim, G. H. (2015). Evaluation of antioxidant activity of marine algae-extracts from Korea. Journal of Aquatic Food Product Technology, 24, 227–240.
- Lee, J. B., Takeshita, A., Hayashi, K., & Hayashi, T. (2011). Structures and antiviral activities of polysaccharides from Sargassum trichophyllum. Carbohydrate Polymers, 86, 995–999.
- Li, Y. X., Wijesekara, I., Li, Y., & Kim, S. K. (2011). Phlorotannins as bioactive agents from brown algae. Process Biochemistry, 46, 2219–2224.
- Lim, S. N., Cheung, P. C. K., Ooi, V. E. C., & Ang, P. O. (2002). Evaluation of antioxidative activity of extracts from a brown seaweed, Sargassum siliquastrum. Journal of Agricultural and Food Chemistry, 50, 3862–3866.
- Maneesh, A., Chakraborty, K., & Makkar, F. (2017). Pharmacological activities of brown seaweed Sargassum wightii (Family Sargassaceae) using different in vitro models. International Journal of Food Properties, 20, 931–945.
- Mao, W., Li, H., Li, Y., Zhang, H., Qi, X., Sun, H., … Guo, S. (2009). Chemical characteristic and anticoagulant activity of the sulfated polysaccharide isolated from Monostroma latissimum (Chlorophyta). International Journal of Biological Macromolecules, 44, 70–74.
- Matanjun, P., Mohamed, S., Mustapha, N. M., Muhammad, K., & Ming, C. H. (2008). Antioxidant activities and phenolics content of eight species of seaweeds from north Borneo. Journal of Applied Phycology, 20, 367–373.
- Min, B., McClung, A. M., & Chen, M.-H. (2011). Phytochemicals and antioxidant capacities in rice brans of different color. Journal of Food Science, 76, 117–126.
- Movahedinia, A., & Heydari, M. (2014). Antioxidant activity and total phenolic content in two alga species from the Persian Gulf in Bushehr Province, Iran. International Journal of Science and Research, 3, 954–958.
- Munir, N., Sharif, N., Naz, S., & Farkhanda, M. (2013). Algae: A potent antioxidant source. Sky Journal of Microbiology Research, 1, 22–31.
- Na, Y. S., Kim, W. J., Kim, S. M., Park, J. K., Lee, S. M., Kim, S. O., … Park, Y. I. (2010). Purification, characterization and immunostimulating activity of water-soluble polysaccharide isolated from Capsosiphon fulvescens. International Immunopharmacology, 10, 364–370.
- Niki, E., & Noguchi, N. (2000). Evaluation of antioxidant capacity. What capacity is being measured by which method?. IUBMB Life, 50, 323–329.
- Okamoto, O. K., Pinto, E., Latorre, L. R., Bechara, E. J. H., & Colepicolo, P. (2001). Antioxidant modulation in response to metal-induced oxidative stress in algal chloroplasts. Archives of Environmental Contamination and Toxicology, 40, 18–24.
- Oueslati, S., Ksouri, R., Falleh, H., Pichette, A., Abdelly, C., & Legault, J. (2012). Phenolic content, antioxidant, anti-inflammatory and anticancer activities of the edible halophyte Suaeda fruticosa Forssk. Food Chemistry, 132, 943–947.
- Pires, J., Torres, P. B., Dos Santos, D. Y. A. C., & Chow, F. (2017a). Ensaio em microplaca do potencial antioxidante através do método de sequestro do radical livre DPPH para extratos de algas. Instituto de Biociências. Universidade de São Paulo, 1–7.
- Pires, J. S., Torres, P. B., Santos, D. Y. A. C., & Chow, F. (2017b). Ensaio em microplaca de substâncias redutoras pelo método do Folin-Ciocalteu para extratos de algas. Instituto de Biociências. Universidade de São Paulo, 1–5.
- Polo, L. K., & Chow, F. (2020). Physiological performance by growth rate, pigment and protein content of the brown seaweed Sargassum filipendula (Ochrophyta: Fucales) induced by moderate UV radiation exposure in the laboratory. Scientia Marina, 84, 59–70.
- Polo, L. K., de L Felix, M. R., Kreusch, M., Pereira, D. T., Costa, G. B., Simioni, C., … Schmidt, E. C. (2014). Photoacclimation responses of the brown macroalga Sargassum cymosum to the combined influence of UV radiation and salinity: Cytochemical and ultrastructural organization and photosynthetic performance. Photochemistry and Photobiology, 90, 560–573.
- Queiroz, K. C. S., Medeiros, V. P., Queiroz, L. S., Abreu, L. R. D., Rocha, H. A. O., Ferreira, C. V., … Leite, E. L. (2008). Inhibition of reverse transcriptase activity of HIV by polysaccharides of brown algae. Biomedicine & Pharmacotherapy, 62, 303–307.
- Roleda, M. Y., Lüder, U. H., & Wiencke, C. (2010). UV-susceptibility of zoospores of the brown macroalga Laminaria digitata from Spitsbergen. Polar Biology, 33, 577–588.
- Roleda, M. Y., Van De Poll, W. H., Hanelt, D., & Wiencke, C. (2004). PAR and UVBR effects on photosynthesis, viability, growth and DNA in different life stages of two coexisting Gigartinales: Implications for recruitment and zonation pattern. Marine Ecology Progress Series, 281, 37–50.
- Roleda, M. Y., Wiencke, C., Hanelt, D., & Bischof, K. (2007a). Sensitivity of the early life stages of macroalgae from the northern hemisphere to ultraviolet radiation. Photochemistry and Photobiology, 83, 851–862.
- Roleda, M. Y., Wiencke, C., Hanelt, D., Van De Poll, W. H., & Gruber, A. (2005). Sensitivity of laminariales zoospores from Helgoland (North Sea) to ultraviolet and photosynthetically active radiation: Implications for depth distribution and seasonal reproduction. Plant, Cell and Environment, 28, 466–479.
- Roleda, M. Y., Zacher, K., Wulff, A., Hanelt, D., & Wiencke, C. (2007b). Photosynthetic performance, DNA damage and repair in gametes of the endemic Antarctic brown alga Ascoseira mirabilis exposed to ultraviolet radiation. Austral Ecology, 32, 917–926.
- Rufino, M. D. S., Alves, R., Brito, E., & Morais, S. (2006). Metodologia científica: Determinação da atividade antioxidante total em frutas pelo métodos de redução do ferro (FRAP. Embrapa Agroindústria Tropical Comunicado Técnico, 3–6.
- Rufino, M. D. S. M., Alves, R. E., de Brito, E. S., de Morais, S. M., Sampaio, C. D. G., Pérez-Jimenez, J., & Saura-Calixto, F. D. (2007). Metodologia científica: Determinação da atividade antioxidante total em frutas pela captura do radical livre ABTSo+ Embrapa Agroindústria Tropical . In Embrapa Agroindústria Tropical. Comunicado técnico Vol. 128 (pp. 1–3). Fortaleza: Embrapa Agroindústria Tropical.
- Ruhland, C. T., Fogal, M. J., Buyarski, C. R., & Krna, M. A. (2007). Solar ultraviolet-B radiation increases phenolic content and ferric reducing antioxidant power in Avena sativa. Molecules, 12, 1220–1232.
- Salgado, L. T., Tomazetto, R., Cinelli, L. P., Farina, M., & Amado Filho, G. M. (2007). The influence of brown algae alginates on phenolic compounds capability of ultraviolet radiation absorption in vitro. Brazilian Journal of Oceanography, 55, 145–154.
- Santos, J. P., Torres, P. B., Dos Santos, D. Y. A. C., Motta, L. B., & Chow, F. (2019). Seasonal effects on antioxidant and anti-HIV activities of Brazilian seaweeds. Journal of Applied Phycology, 31, 1333–1341.
- Schaeffer, D. J., & Krylov, V. S. (2000). Anti-HIV Activity of extracts and compounds from algae and cyanobacteria. Ecotoxicology and Environmental Safety, 45, 208–227.
- Seeram, N. P., Aviram, M., Zhang, Y., Henning, S. M., Feng, L., Dreher, M., & Heber, D. (2008). Comparison of antioxidant potency of commonly consumed polyphenol-rich beverages in the United States. Journal of Agricultural and Food Chemistry, 56, 1415–1422.
- Sinha, S., Astani, A., Ghosh, T., Schnitzler, P., & Ray, B. (2010). Polysaccharides from Sargassum tenerrimum: Structural features, chemical modification and anti-viral activity. Phytochemistry, 71, 235–242.
- Sinjal, C. A., Rompas, R. M., Sumilat, D. A., & Suryanto, E. (2018). Antioxidant and photoprotective activity of brown seaweed from North Sulawesi Coast. International Journal of ChemTech Research, 11, 121–133.
- Synytsya, A., Kim, W. J., Kim, S. M., Pohl, R., Synytsya, A., Kvasnička, F., … Il Park, Y. (2010). Structure and antitumour activity of fucoidan isolated from sporophyll of Korean brown seaweed Undaria pinnatifida. Carbohydrate Polymers, 81, 41–48.
- Thomas, N. V., & Kim, S. K. (2011). Potential pharmacological applications of polyphenolic derivatives from marine brown algae. Environmental Toxicology and Pharmacology, 32, 325–335.
- Thuy, T. T. T., Ly, B. M., Van, T. T. T., Van Quang, N., Tu, H. C., Zheng, Y., … Ai, U. (2015). Anti-HIV activity of fucoidans from three brown seaweed species. Carbohydrate Polymers, 115, 122–128.
- Torres, P. B., Pires, J. S., Santos, D. Y. A. C., & Chow, F. (2017). Ensaio do potencial antioxidante de extratos de algas através do sequestro do ABTS•+ em microplaca. 1–4.
- Trevisan, M., Browne, R., Ram, M., Muti, P., Freudenheim, J., Carosella, A. M., & Armstrong, D. (2001). Correlates of markers of oxidative status in the general population. American Journal of Epidemiology, 154, 348–356.
- Turrens, J. F. (1997). Superoxide production by the mitochondrial respiratory chain. Bioscience Reports, 17, 3–8.
- Turrens, J. F., & Boveris, A. (1980). Generation of superoxide anion by the NADH dehydrogenase of bovine heart mitochondria. Biochemical Journal, 191, 421–427.
- Urquiaga, I., & Leighton, F. (2003). Plant polyphenol antioxidants and oxidative stress. Biological Research, 33, 55–64.
- Urrea-Victoria, V., Pires, J., Torres, P. B., & Santos, D. Y. A. C. (2016). Ensaio antioxidante em microplaca do poder de redução do ferro (FRAP) para extratos de algas. Universidade de São Paulo, 1–6.
- Ursi, S., & Plastino, E. M. (2001). Crescimento in vitro de linhagens de coloração vermelha e verde clara de Gracilaria birdiae (Gracilariales, Rhodophyta) em dois meios de cultura: Análise de diferentes estádios reprodutivos. Revista Brasileira de Botanica, 24, 587–594.
- Van de Poll, W. H., Eggert, A., Buma, A. G. J., & Breeman, A. M. (2001). Effects of UV-B-induced DNA damage and photoinhibition on growth of temperate marine red macrophytes: Habitat-related differences in UV-B tolerance. Journal of Phycology, 37, 30–37.
- van de Poll, W. H., Hanelt, D., Hoyer, K., Buma, A. G. J., & Breeman, A. M. (2002). Ultraviolet-B-induced cyclobutane-pyrimidine dimer formation and repair in Arctic marine macrophytes. Photochemistry and Photobiology, 76, 493–500.
- Vasconcelos, J. B., de Vasconcelos, E. R. T. P. P., Urrea-Victoria, V., Bezerra, P. S., Reis, T. N. V., Cocentino, A. L. M., Fujii, M. T. (2018). Antioxidant activity of three seaweeds from tropical reefs of Brazil: Potential sources for bioprospecting. Journal of Applied Phycology, 31, 835–846.
- Vass, I., Szilard, A., & Sicora, C. (1997). Adverse effects of UV-B light on the structure and function of the photosynthetic apparatus. Handbook of Photosynthesis, 931–949. doi:10.1201/9781420027877.ch43
- Viennois, E., Chen, F., Laroui, H., Baker, M. T., & Merlin, D. (2013). Dextran sodium sulfate inhibits the activities of both polymerase and reverse transcriptase: Lithium chloride purification, a rapid and efficient technique to purify RNA. BMC Research Notes, 6. doi:10.1186/1756-0500-6-360
- Vinayak, R. C., Sabu, A. S., & Chatterji, A. (2011). Bio-prospecting of a few brown seaweeds for their ctotoxic and antioxidant activities. Evidence-Based Complementary and Alternative Medicine, 2011, 1–9.
- Wang, T., Jónsdóttir, R., & Ólafsdóttir, G. (2009). Total phenolic compounds, radical scavenging and metal chelation of extracts from Icelandic seaweeds. Food Chemistry, 116, 240–248.
- Wang, W., Wang, S. X., & Guan, H. S. (2012). The antiviral activities and mechanisms of marine polysaccharides: An overview. Marine Drugs, 10, 2795–2816.
- Waterman, P. G., & Mole, S. (1994). Analysis of phenolic plant metabolites (First edit. ed.). Oxford: Phytochemistry. Blackwell Scientific.
- Woradulayapinij, W., Soonthornchareonnon, N., & Wiwat, C. (2005). In vitro HIV type 1 reverse transcriptase inhibitory activities of Thai medicinal plants and Canna indica L. rhizomes. Journal of Ethnopharmacology, 101, 84–89.
- Worm, S. W., Sabin, C., Weber, R., Reiss, P., El‐Sadr, W., Dabis, F., … Lundgren, J. (2010). Risk of myocardial infarction in patients with HIV infection exposed to specific individual antiretroviral drugs from the 3 major drug classes: The data collection on adverse events of anti‐HIV drugs (D:A:D) study. The Journal of Infectious Diseases, 201, 318–330.
- Yang, H., Dong, Y., Du, H., Shi, H., Peng, Y., & Li, X. (2011). Antioxidant compounds from propolis collected in Anhui, China. Molecules, 16, 3444–3455.
- Zaid, S. A. A.-L., Abdel-Wahab, K. S. E.-D., & Abed, N. N. (2017). Screening for antiviral activities of aqueous extracts of some Egyptian seaweeds. The Egyptian Journal of Hospital Medicine, 64, 430–435.
- Zandi, K., Ahmadzadeh, S., Tajbakhsh, S., Rastian, Z., Yousefi, F., Farshadpour, F., & Sartavi, K. (2010). Anticancer activity of Sargassum oligocystum water extract against human cancer cell lines. European Review for Medical and Pharmacological Sciences, 14, 669–673.
- Zhu, W., Chiu, L. C. M., Ooi, V. E. C., Chan, P. K. S., & Ang, P. O. (2004). Antiviral property and mode of action of a sulphated polysaccharide from Sargassum patens against Herpes simplex virus type 2. International Journal of Antimicrobial Agents, 24, 81–85.
- Zubia, M., Fabre, M. S., Kerjean, V., Lann, K. L., Stiger-Pouvreau, V., Fauchon, M., & Deslandes, E. (2009). Antioxidant and antitumoural activities of some Phaeophyta from Brittany coasts. Food Chemistry, 116, 693–701.
- Zubia, M., Robledo, D., & Freile-Pelegrin, Y. (2007). Antioxidant activities in tropical marine macroalgae from the Yucatan Peninsula, Mexico. Journal of Applied Phycology, 19, 449–458.