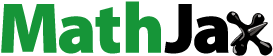
ABSTRACT
Wild pollinators play a crucial role in maintaining healthy ecosystems and sustaining agricultural productivity. However, their survival is at risk due to habitat loss and land use changes, exacerbating the ongoing biodiversity crisis, and jeopardising global food security. We assessed the contribution of native vegetation cover to pollination and avocado crop yield by modelling the abundance of native bees and the pollination services they provide in agroecosystems of the Aconcagua River basin in Central Chile. This region stands as a critical biodiversity hotspot, currently confronted with the challenge of land use change, especially due to the expansion of avocado (Persea americana Mill.) monocultures. Our findings revealed a clear relationship between native bee abundance, pollination services, and avocado yield as a function of natural vegetation cover surrounding the crops. Areas with a higher proportion of native vegetation exhibited the most abundant supply of pollination services, while within avocado crops show lower levels of pollination support. A spatial mismatch between supply and demand for pollination revealed by our results indicates that agricultural expansion threatens native pollinators and agricultural yield production in this region. The development of pollination maps has been proposed as a strategic tool to guide land-use planning decisions within agricultural landscapes. Prioritizing the conservation of native vegetation or adopting biodiversity-friendly practices can help mitigate the effects of agricultural expansion on wild pollinators. These strategies are vital for safeguarding biodiversity, ensuring food security, and mitigating the far-reaching impacts of the current biodiversity crisis.
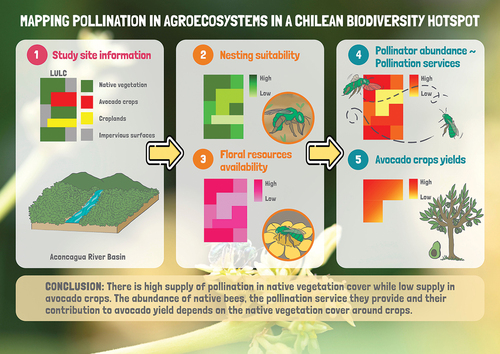
Key policy highlights
The rising demand for avocado, sought after as a superfood, has led to a rapid expansion of its cultivation areas in Chile and consequent loss of the native vegetation that serves as habitat for pollinators.
Considering that production of avocado is highly dependent on pollinators, we used models to obtain pollination maps, which revealed a positive relationship between native bee abundance, pollination services, and avocado yield as a function of natural vegetation cover surrounding the crops.
The expansion of avocado plantations with the consequent loss of native vegetation puts pollination services at risk, compromising their own productivity and long term sustainability
Conserving and restoring native vegetation in agricultural landscapes is essential to safeguard key ecosystems services, such as the ones provided by wild pollinators.
Pollination maps are strategic tools to guide land use planning decisions in order to balance agricultural activity and ecological sustainability in central Chile production landscapes.
EDITED BY:
1. Introduction
Wild pollinators, such as native bees, play a critical role in facilitating the reproductive processes of many flowering plants (Ollerton et al. Citation2011), including crops essential for global food security (Klein et al. Citation2007). With 75% of food crops relying on animal-mediated pollination (Klein et al. Citation2007; Aizen and Harder Citation2009), pollinators provide vital ecosystem services that sustain around 35% of the world’s food crop production (IPBES Citation2016). Despite their significance, anthropogenic activities are threatening the sustainability of these essential ecosystem services, which have been linked to a decline in both wild and domesticated pollinator populations (Potts et al. Citation2010; Grab et al. Citation2019; IPBES et al. Citation2019). Pollinator conservation research has become an urgent global issue (IPBES Citation2016; CBD Citation2018) as the effects of pollinator reduction go beyond ecological concerns and directly affect agricultural productivity and food security (Aizen et al. Citation2008; IPBES et al. Citation2019).
Landscapes characterised by greater complexity in their configuration, composition, and heterogeneity are known to support higher levels of biodiversity (Estrada-Carmona et al. Citation2022) and play a critical role in sustaining wild pollinator populations (Kennedy et al. Citation2013). The loss and fragmentation of natural habitats, driven by land use change and agricultural demand, pose significant challenges to native pollinators (IPBES Citation2016). Particularly detrimental is the transformation of natural habitats adjacent to croplands into less diverse areas, often through the clearing of native vegetation or its replacement with monoculture plantations (Ricketts et al. Citation2008). This process significantly reduces the availability of foraging and nesting resources that are essential for the maintenance of wild pollinators (Steffan-Dewenter et al. Citation2002; Kremen et al. Citation2002; Ricketts et al. Citation2008; Garibaldi et al. Citation2011).
Wild bees stand out as a significant pollinator group due to their abundance, efficiency in pollination, and widespread distribution (Westrich and International Bee Research, A., and Linnean Society of, L. Citation1996; Winfree et al. Citation2008, Citation2011; Grab et al. Citation2019). They have shown to complement and even replace pollination services provided by managed bees, enhancing the stability and resilience of agroecosystems (Kremen et al. Citation2002; Garibaldi et al. Citation2011, Citation2013; Wentling et al. Citation2021). The dependency of wild bees on floral resources and nesting substrates for their survival makes them particularly sensitive to land use changes (Bukovinszky et al. Citation2017). Isolation and greater distances from natural habitat patches are known to negatively impact bee populations, leading to reduced richness, visitation rates, and ultimately, lower fruit set and production in agricultural areas (Ricketts et al. Citation2008; Garibaldi et al. Citation2011). Conversely, an increase in the proportion of natural habitats correlates positively with greater bee richness and enhancement of their pollination services (Rahimi et al. Citation2021).
The Mediterranean-type ecosystem in central Chile holds a high proportion of endemism and is considered a global biodiversity hotspot (Myers et al. Citation2000). This ecosystem is characterised by sclerophyllous and xeric shrubland vegetation that have recently been identified as hotspots of bee species richness (Orr et al. Citation2021). Land use and land cover change is one of the most important pressures on ecosystems in Chile (Schulz et al. Citation2010). In central Chile, especially in the Aconcagua river basin located in the Valparaíso region, there has been a strong intensification and expansion of avocado (Persea americana Mill) monocultures, generating extensive losses of native vegetation cover (Armesto et al. Citation2010; Soto Bäuerle et al. Citation2011; Magrach et al. Citation2020). Chile is a major avocado producer and exporter worldwide (Guevara et al. Citation2021), with the Valparaíso region producing the largest amount of this fruit (Gardiazabal Citation2001). An estimate of 40–60% of avocado yield depends on animal-mediated pollination (Klein et al. Citation2007; Dymond et al. Citation2021). Avocado pollination is managed in Chile by incorporating hives of Apis mellifera into the orchards in densities of 6 to10 hives/ha (Monzón et al. Citation2020). A progressive decrease in avocado production has been observed in Chile (Guevara et al. Citation2021), which may be related to water and bee hive offer shortage (Basualdo et al. Citation2022). Recent studies suggest that wild pollinators increase avocado productivity (Dymond et al. Citation2021; García et al. Citation2021; Celis-Diez et al. Citation2023). Native bees comprise about one-third of the abundance of avocado floral visitors (Dymond et al. Citation2021), highlighting the importance of conserving their habitat in order to promote their presence in agricultural and natural landscapes.
Considering the threat posed by the accelerated rates of natural habitat loss in Central Chile it becomes urgent to assess the extent to which native vegetation cover influences the abundance of wild pollinators and the pollination services they provide in agroecosystems. This study focuses on the crucial role of native vegetation in supporting avocado pollination and yield within the Aconcagua river basin. Utilising the InVEST crop pollination model, we bridged the knowledge gap regarding native bee abundance in Chile by generating spatially explicit data in the form of maps that detail native bee abundance and their pollination services and contribution to avocado crop yields. Through this modelling effort we seek to investigate the relationship between native bee populations and avocado crop yields in relation to landscape composition, specifically examining the area, proportion, and number of types of native vegetation formations proximate to agricultural areas. We hypothesize that areas in which the expansion of avocado cultivation have degraded native vegetation the most will show the lowest supply of pollination services.
Our work provides important insights into the conservation and restoration of native vegetation as a strategy to safeguard biodiversity and food security by elucidating the relationships between wild pollinators and agricultural practices. The spatially explicit data generated, along with insights into how pollinator communities are impacted by changes in land use, can inform policymakers, land managers, and stakeholders to develop effective strategies that balance agricultural expansion and ecological sustainability in central Chile production landscapes.
2. Materials and methods
The InVEST crop pollination model makes predictions about the abundance of wild bees based on the capacity of the landscape and the different land cover types to provide the main requirements of wild bees to remain in a landscape, (i.e. floral resources and nesting sites) (Sharp et al. Citation2020). We used the InVEST crop pollination model to spatially analyse the pollination services provided by native bees in the Aconcagua river basin as a representative area of the Chilean Mediterranean-type ecosystem. We specifically analysed: i) the landscape coverages that provide habitat for native bees; ii) the areas with the greater abundance of native bees providing pollination services; iii) the contribution of native bees to avocado crop yield in the Aconcagua river basin; iv) and the relationship between their contribution to crop yield and landscape composition in terms of native vegetation formations. We hypothesise that the abundance of native bees and, consequently, the pollination service they provide and their contribution to avocado yield increases with increasing area, types and proportion of native vegetation formations.
2.1. Study site
The study site corresponds to the Aconcagua river basin, located in the Valparaíso region, in central Chile, which comprises an area of 7,334 km2 (Flores Citation2020). The study site has a temperate Mediterranean-type climate with a prolonged dry season, except for the area of the Andes Mountains 3,000 metres above sea level, which has a cold high altitude climate (DGA Citation2004). The area has a great diversity of flora and fauna, with 14 types of vegetation formations (Soto Bäuerle et al. Citation2011). Through remote sensing and field sampling during 2019 we determined that an area of 410 km2 of the Basin is covered by avocado crops (, see supplementary materials for methods).
2.2. Crop pollination model
The InVEST crop pollination model delivers a map of wild bees abundance in the landscape as an indicator of pollination services. For this, it first calculates a pollinator supply index , which corresponds to an index of abundance of bees nesting in each cell of the landscape (30 × 30 m), expressed as suitability indexes (from 0 to 1) for each cover, where higher values indicate a greater source of pollinators, generating a pollinator supply map for each bee species. The calculations use estimates of nesting sites in each cell and the availability of floral resources in surrounding cells within the flight and foraging ranges of the species, where floral resources in cells close to nesting sites receive more weight than those at distant cells. Thus, the index of pollinator supply
in the pixel
for the species β is defined as:
Where, is the nesting suitability for each cover
,
corresponds to the floral resources generated in the cover
,
is the Euclidean distance between the nesting cells (
) and the floral resource cell (m) and α the foraging distance of the species β.
The model then calculates the abundance of pollinators in each agricultural cell for each bee species, i.e. the number of bees visiting each cell for each bee species, indicating in which parts of the landscape they are actively providing pollination services, and delivering a map of pollinator abundance as an approximation of the pollination services they provide. This is calculated based on the floral resources that attract pollinators in and around each cell, and the supply of pollinators that can access these resources, considering the feeding activity and flight range of the species. The abundance of pollinators
of the species β travelling from a cell
to an agricultural cell
corresponds to:
Where, is the supply of pollinators of the species β in the cell
,
is the distance between the source cell
and the agricultural cell
, and α the typical foraging distance of the species. For more details see Tallis et al. (Citation2013).
Finally, the model calculates a simple index of the contribution of native bees to crop yield, based on the abundance of bees and the dependence of the crop on pollinators. The total yield achieved by the crop will depend on the crop’s dependence on pollination and the proportion in which pollinators meet those pollination needs. For more details see Sharp et al. (Citation2020). From this, we obtained a map of pollinator abundance in avocado crops, and a map of total yield of avocado crops for the Aconcagua River basin.
2.3. Model requirements
To feed the model we generated four base resources that reflect the characteristics of the case study: a Land Use Land Cover (LULC) map of the Aconcagua river basin, a table with information on the bee species, a table of biophysical information of the landscape that contains values of nesting suitability and availability of floral resources for each cover of the LULC map and a vector file with information on avocado crops. With these resources, the model generates as intermediate results a nesting suitability map and a floral resource availability map for the study site, in our case the Aconcagua river basin. Then, using these maps as input, the model delivers as final result a map of native bee abundance as a proxy of pollination services in the basin, a map of pollinator abundance and pollination services delivered specifically in avocado orchards, and a map of avocado crop yield. This process is illustrated in . The methodology for the creation of the four input resources are detailed below:
Figure 2. Inputs and outputs generated by the InVEST crop pollination model: From the 4 base resources required by the model, the model delivers 2 maps as intermediate outputs (floral resource availability map and nesting suitability map for native bees) and 3 maps as final outputs (landscape pollinator abundance map, crop yield map, and crop pollinator abundance map).
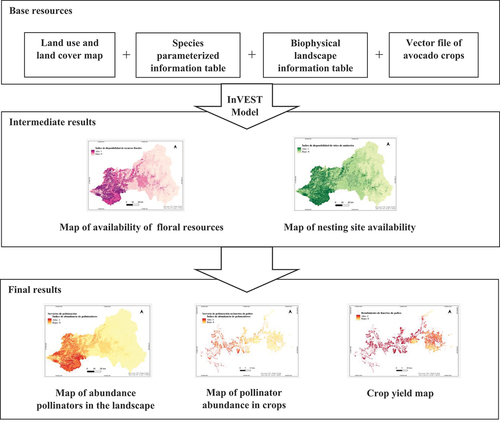
2.3.1. Raster map of land use and land cover
A map was constructed based on the land cover map of Chile generated by Zhao et al. (Citation2016), which was elaborated with data from 2014 with a resolution of 30 × 30 m. This map was updated with 2019 data, maintaining the original resolution to ensure consistency in our spatial analysis. This update was achieved by integrating remote sensing data with ground-truthing, focusing on changes in avocado cultivation areas and the status of native vegetation. The remote sensing data came from satellite imagery with spectral and resolution capabilities similar to those employed by Zhao et al. (Citation2016). This guaranteed the accuracy and consistency of our modified LULC map with the original map by Zhao et al. (Citation2016) (see supplementary materials for methods). In this way, we added the location and land use and land cover category corresponding to avocado crops (), and the native vegetation formations present in the Aconcagua river basin. The native vegetation formations obtained were classified according to the vegetational belts defined by MMA (Citation2020). When comparing the extent of native vegetation cover for 2019 with the 2014 data from the work of Zhao et al. (Citation2016), areas were observed that no longer corresponded to native vegetation. These areas were reclassified as areas of ‘degraded vegetation’ informed by a combination of spectral analysis and field observations. Specifically, areas previously identified as vegetation or agricultural land in the work by Zhao et al. (Citation2016) but showing significant changes in their spectral signatures by 2019 were closely examined. Upon field verification, it was found that many of these areas had suffered degradation, primarily due to water scarcity and increased demand for irrigation water (Centro de Cambio Global UC Citation2019). This degradation often resulted in a loss of original vegetation cover, rendering these lands unsuitable for classification as active agricultural areas or native shrublands. The updated LULC map was generated using QGIS 3.22 software (QGIS Development Team Citation2022) thus obtaining a LULC map of the Aconcagua River basin with 23 classes relevant for running the model, corresponding to 14 types of native vegetation formations, avocado crops, and 8 classes derived from Zhao’s classification ().
2.3.2. Species parameterized information table
Nine native bee species recorded as floral visitors of avocado flowers were considered in this study (Monzón et al. Citation2020; Martínez-Harms, J., personal communication; Citation2020) (Table S1). A parameterized table was constructed that includes values for suitability of nesting substrate, activity season, typical foraging distance and relative abundance for each species (Table S5). The availability and suitability of nesting sites for each bee species will depend on which substrate they use, so it is necessary to know the nesting substrate used by native bees. Depending on the availability of the nesting substrate in each land cover, the nesting suitability index for each substrate was estimated. According to the nesting substrate used by the bees, the nesting suitability index for each land cover was estimated and included in the biophysical landscape information table, the details of its calculation are explained in section 2.3.2. Each species was assigned a nesting suitability value for the nesting substrates they use in a range from 0 to 1, where 0 means that they do not use the substrate at all and 1 means that they use it completely. All bee species considered in our study use soil as their solely nesting substrate and were assigned a value of 1 for soil nesting suitability. We considered the whole year as a single season (Tallis et al. Citation2013), given that the native bee species considered are all present during avocado flowering. Based on this, a seasonal activity index value of 1 was used for each species.
The typical foraging distance of each species was obtained using the BeeIT package in R developed by Cariveau et al. (Citation2016), based on regression formulas developed by Greenleaf et al. (Citation2007) that allometrically relate measures of the inter-tegular width to their foraging distance (Table S4). It was not possible to obtain the inter-tegular width measurement for all bee species due to the lack of access to specimens. For this reason, based on the available data, an average value foraging distance was calculated, corresponding to 1845 metres. The versatility of the InVEST model, which allows the use of generalised ‘proto pollinator’ profiles in situations when species-specific data are missing, served as the basis for this methodological decision (Sharp et al. Citation2020).
The model allows incorporating relative abundance values for each bee species considered in order to weight the contribution of each bee to the total abundance of pollinators. Since there is no data on the abundance of native bees in the study area, it was decided to weight all the species equally, assigning a value of 1 to each species. This simplification is recommended when data are not available, and allows us to identify general patterns in the pollination services provided by native bees (Sharp et al. Citation2020). A life history trait table for the native bee species is shown in supplementary material (Table S1).
2.3.3. Biophysical landscape information table
A table with biophysical information about floral and nesting resources availability for the different land cover classes was constructed (Table S6). Nest site availability values were assigned for the substrate type ‘soil’ (since all bee species considered nest in soil), ranging from 0 (not suitable) to 1 (maximum suitability), depending on the area of soil available in the land cover and the level of soil disturbance. For this, a value of 1 was first assigned to the cover with the highest substrate availability and least disturbance, and then the other covers were assigned values in proportion to that maximum value (Tallis et al. Citation2013; Sharp et al. Citation2020). Similarly, for floral resources availability values between 0 and 1 were assigned for each land cover (Table S6). Floral resource availability values were calculated considering the floristic composition of each land cover (MMA Citation2020) and the floristic association with native bees (Table S2 and S3). The floral resource availability value for native vegetation cover types was calculated as the number of floral species used by native bee species divided by the total number of species present on a given vegetational belt (RF= n° floral species used/n° floral species on vegetational belt). The suitability values for the 14 native vegetation coverages were grouped into six ranges, 0.5 - 0.6 - 0.7 - 0.8 - 0.9 -1.0, corresponding to the highest values among the classes. The remaining nine classes (those not corresponding to native vegetation coverages) were assigned their floral resource availability index through expert opinion and literature, considering the criteria of attractiveness and availability of floral resources for bee species present in the coverages and in relation to the maximum values calculated for native vegetation cover types (Tallis et al. Citation2013; Monzón et al. Citation2020; Rodríguez et al. Citation2022)
2.3.4. Vector file of avocado crops
Additionally, a vector file of avocado crops was created, which contains the geospatial location of each avocado crop and the following information: 1) degree of avocado dependence on pollinators: avocado has a high dependence on pollinators with a dependence ratio of 0.65 ~ 0.7 (Klein et al. Citation2007); 2) proportion of pollination delivered by managed pollinators (A. mellifera): Estimated from expert opinion and literature (Sharp et al. Citation2020). A value of 0.5 was assigned considering that avocado have a high need for pollination and their flowers are not so attractive to A. mellifera (Afik et al. Citation2006), not meeting the pollination demand, resulting in a pollination deficit (Pérez Giraldo Citation2021). This value is used by the model to calculate the contribution of native bees to crop yield, considering the contribution already being made by managed pollinators; 3) nesting suitability value for the soil substrate; 4) availability of floral resources of the crop; and 5) average crop saturation coefficient (Kc), which represents the abundance of pollinators needed to reach 50% of the pollinator-dependent crop yield, in this case a Kc value of 0.125 was used following the recommendation of Tallis et al. (Citation2013).
2.4. Analysis of results
To evaluate the relationship between the abundance of native bees and the pollination service they provide in each avocado crop, with the number, area and proportion of native vegetation formations, we used the information and output maps obtained by the modelling. Using the ‘Buffer’ tool of the QGIS 3.22 and 3.28 software, zones of influence were defined based on the average flight range of the bee species (1845 m), considered from the edge of each avocado crop patch. An avocado crop patch was defined as an avocado crop polygon of the vector file of avocado crops obtained from field sampling and remote sensing during 2019 (see supplementary material). Using the field calculator and the ‘sum’ function of the QGIS software, the number, area and proportion of native vegetation formations were calculated within each buffer as shown in .
Figure 4. Zone of influence according to flight range of native bee species around avocado orchards.
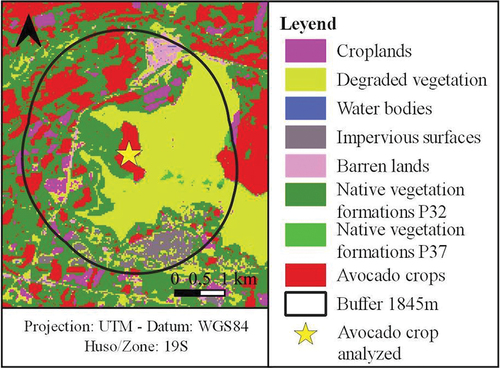
The Lilliefors test was used to evaluate the normality of the data. The relationship between the calculated variables (number, area and proportion of native vegetation formations), with the values provided by the model of native bee abundance and crop yield, was then evaluated using Spearman’s correlations and generalised linear models (GLM). Pollinator abundance as a proxy for pollination services and avocado crop yield were considered as response variables, and the number, area and proportion of native vegetation formations around each avocado crop patch were considered as explanatory variables. For the GLMs, the area of native vegetation variable was not considered since it is highly correlated with the proportion of native vegetation variable. The variables corresponding to proportion of native vegetation, number of native vegetation cover types and their interactions were evaluated. The analysis considered 6615 avocado crop patches that had native vegetation around them within the buffer zone. The best GLMs were selected using the Akaike information criterion (AIC) and deviancy analysis, the latter allowing a pseudo R2. The best GLM for the response variable pollinator abundance followed an inverse Gaussian distribution with identity link function and the predictor variables were fitted with the x2-transformation. The GLM for the response variable crop yield followed a gamma distribution with identity link function. The analyses were performed using RStudio 4.2.1 software (RStudio-Team Citation2020).
3. Results
Through the modelling we obtained maps of availability of floral resources and availability of nesting sites, and thereby maps of pollination services in the Aconcagua River basin ().
Figure 5. Maps obtained from InVEST’s crop pollination model in the Aconcagua river basin: (a) Map of availability of ground nesting sites for native bees, (b) Map of availability of floral resources for native bees, (c) Map of pollination service provided by native bees, (d) Map of pollination services provided by native bees in avocado crops, (e) Yield map of avocado crops.
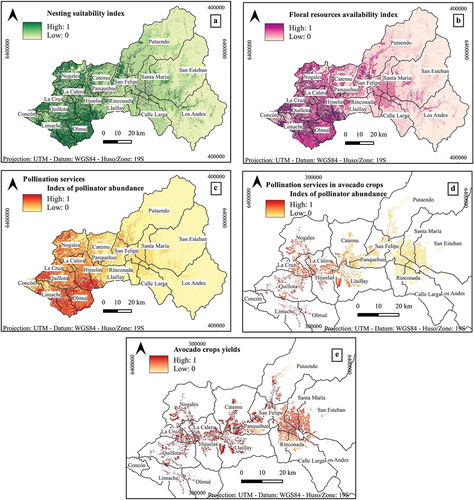
As shown in higher rates of availability of floral resources and nesting sites are found west of the Aconcagua River basin compared to the central and more eastern mountainous zones of the river basin. Coincidentally, the same pattern is observed for the abundance of pollinators and pollination services provided by wild bees (), with a greater abundance of pollinators in the western part of the river basin, in the localities of Hijuelas, Quillota, Calera, and especially in Olmué and Limache, compared to the rest of the river basin.
The map of pollinator abundance in avocado crops revealed a general low abundance of native bees and low supply of pollination services, with higher bee abundance and greater pollination services supply in the crops located at the western part of the river basin (). In terms of crop yield, a higher crop yield was observed towards the western part of the river basin, coinciding with a higher abundance of pollinators. The lowest yields were found in crops located in the central parts of the Aconcagua River basin, in the provinces of San Felipe and Los Andes, specifically in the localities of Panquehue, San Felipe, Calle Larga, Rinconada, Los Andes, San Esteban, Santa María, which are also the areas with higher density of avocado crops (). The results revealed a spatial mismatch in the supply of pollination services and their beneficiaries.
The analysis revealed that pollinator abundance and calculated crop yields were positively and significantly correlated with the surrounding native vegetation areas (rho = 0.611, p-values <2.2e- 16/rho = 0.632, p-values <2.2e-16) and the proportion of surrounding native vegetation areas (rho = 0.619, p-values <2.2e-16/rho = 0.637, p-values <2.2e-16) (Fig. S1A, B; Fig. S2A, B). No correlation was found between the number of vegetation cover types and pollinator abundance and crop yield (rho = 0.054, p-value = 1.3e-5/rho = 0.218, p-values <2.2e-16) (Fig. S1C, S2C). The number of native vegetation cover types within the buffer zones ranged from 0 to 5, and only two crop patches had five cover types around them. The native vegetation cover type with the largest area around avocado crops corresponded to the vegetational belt 40, i.e. Mediterranean coastal sclerophyllous forest of Lithrea caustica - Cryptocarya alba, followed by Mediterranean interior thorny forest of Vachellia caven – Prosopis chilensis (vegetational belt 32) and Mediterranean interior thorny scrub of Trevoa quinquenervia - Colliguaja odorifera (vegetational belt 27). The percentage of area occupied by each land cover type within the buffer zones and along the Aconcagua river basin is shown in Table S7.
The GLMs revealed that the proportion of native vegetation and the interaction between the number of native vegetation covers and the proportion of native vegetation around the crop explains 23% of the variation in native bee abundance in avocado crops and 29% of the variation in avocado crop yield. For both native bee abundance and avocado crop yield, the most important predictor variable was the proportion of native vegetation. The number of native vegetation cover types showed a significant and positive interaction with the proportion of native vegetation for both response variables ().
Table 1. Generalized linear model (GLM) results that explains the variation of the response variable pollinator abundance (p_ab), from the proportion of native vegetation (prop_veg) and the interaction between the variable number of native vegetation covers (n_cob) and the proportion of native vegetation, both variables adjusted with the transformation x2, considering an inverse gaussian distribution with identity link function. (“”: indicates interaction between the variables).
Table 2. Generalized linear model (GLM) results that explains the variation of the response variable avocado crop yield (yield), from the variable of proportion of native vegetation (prop_veg) and the interaction between the variable number of native vegetation covers (n_cob) and the proportion of native vegetation, considering a gamma distribution with identity link function (“”: indicates interaction between the variables).
4. Discussion
4.1. Relationship between native vegetation cover and wild pollinator abundance
Our findings reveal a clear association between the extent of native vegetation cover and the abundance of wild bees in agroecosystems in the Aconcagua river basin. Previous studies have shown that areas with higher proportions of natural habitat exhibit increased diversity and population sizes of native bees (Goulson et al. Citation2015; Winfree et al. Citation2018; Grab et al. Citation2019). Native vegetation provides essential foraging and nesting resources for many native pollinator species (Steffan-Dewenter et al. Citation2002; Ricketts et al. Citation2008). Consequently, the loss of natural habitats adjacent to avocado crops is likely to reduce the availability of such critical resources for wild pollinators, potentially leading to pollinator population declines (Aizen et al. Citation2008).
Plant biodiversity provides important ecosystem services, ensures food security and strengthens the adaptive capacity of agricultural landscapes in the face of global change (Tscharntke et al. Citation2005; Garibaldi et al. Citation2011). Modelling spatial patterns of native pollinators distribution shows that the proportion of native vegetation is the main predictor for the abundance of native bees and avocado crop yield (). This finding fulfils the hypothesis that the abundance of native bees and the pollination services they provide in avocado crops increases as the proportion of native vegetation in the surroundings increases.
Our results are consistent with previous studies showing that the proportion of natural habitat around crops represent a simple but comprehensive measure of crop isolation from natural habitat. The smaller the proportion of natural habitat around the crop, the more isolated the crop becomes, increasing the negative consequences for pollinators (Kremen et al. Citation2004; Ricketts et al. Citation2008; Carré et al. Citation2009; Garibaldi et al. Citation2011). These landscape variables presented a positive relationship, i.e. there is a higher abundance of native bees and higher yields when there is a higher proportion of native vegetation formations around the crop (Kremen et al. Citation2004; Garibaldi et al. Citation2011; Rahimi et al. Citation2021). Ricketts et al. (Citation2008) also found this relationship, however, they found weak evidence that this affected crop yields. In the case of avocado this relationship may be stronger given that avocado flowers seem to be unattractive to honey bees (Afik et al. Citation2006). Wild insects have been shown to contribute to pollination, fruit set and yield of avocado (Dymond et al. Citation2021) and data collected in farms located in the Aconcagua river basin indicate that visits of wild insect positively and significantly explain avocado productivity (García et al. Citation2021; Celis-Diez et al. Citation2023).
In order to assess whether a higher diversity of native vegetation types would benefit bee populations, we evaluated the effect of the number of native vegetation formation types around each crop on the abundance of native bees and crop yield. Although a higher diversity of vegetation cover types may reflect a greater complexity of habitat and diversity of resources (Fahrig et al. Citation2011), no effect was found on pollinator abundance or crop yield. This may be due to the variation in the number of native vegetation cover types within the buffer zones ranging from 0 to 5, with only two crop patches being surrounded by all five cover types. The GLMs analysis revealed that the interaction between the number of cover types with the proportion of native vegetation correlates significantly with the abundance of native bees and crop yield (). This may indicate that a higher proportion of native vegetation areas and a greater diversity of resources are necessary to increase the abundance of wild pollinators, supporting the idea that biodiversity-friendly landscapes are more productive (Tscharntke et al. Citation2021).
4.2. Modelling pollination maps and spatial mismatch of pollination services
Our results indicate that the abundance of native bees, as a proxy of pollination service in the Aconcagua river basin, is strongly linked to the proportion of the native vegetation areas providing their habitat. The area with the greater abundance of native bees towards the western part of the river basin, is also the section with higher proportion of conservation areas (MMA Citation2019). On the other hand, in the central portion of the river basin where the abundance of native bees was the lowest, there are no conservation areas and native vegetation has been strongly degraded due to an increase of agricultural land (Soto Bäuerle et al. Citation2011) and severe drought events (DGA Citation2022). Avocado crops in this area are mostly surrounded by degraded vegetation, which may explain the low abundance of native bees and lower crop yields.
Our spatial analysis revealed a concerning mismatch between the areas supplying pollination services and the locations where these services are needed the most for avocado production. This spatial discrepancy probably arises due to the encroachment of avocado monocultures into areas recently occupied by native vegetation (Kremen et al. Citation2002). As a result, wild pollinators face reduced foraging and nesting resources near agricultural landscapes, and avocado crops may suffer from insufficient pollination support (Klein et al. Citation2007). This mismatch adds to the evidence that agricultural expansion, especially the expansion of avocado monocultures, threatens native pollinators and the long-term viability of agricultural production in the region. These results highlight the urgency of adopting biodiversity-friendly land-use planning practices that prioritise the preservation of native vegetation habitats adjacent to croplands (IPBES et al. Citation2019; Tscharntke et al. Citation2021).
4.3. Limitations of the study
The present study provides relevant evidence to better understand the relationships between agricultural intensity and biodiversity in the Aconcagua river basin and can serve as input for future studies aimed at determining an ‘optimal’ spatial agricultural configuration for the conservation of biodiversity and ecosystem services. However, for direct use in decision making, it must be validated, for example, by comparing its results with field data in representative areas of the study site (Koh et al. Citation2016; Wentling et al. Citation2021). The predictions present limitations, essentially due to the lack of knowledge on native bees ecology, pollinator abundance and floristic composition. Particularly, information about flight ranges of pollinator species is of great relevance, since the effects of isolation from natural areas are expected to be lower for pollinators with larger flight ranges given their greater dispersal capacity (Williams et al. Citation2010; Garibaldi et al. Citation2011). This information can determine at what scale and what type of measures are the most appropriate for different targets and species and therefore relevant for applying crop-specific strategies. An additional limitation is that the crop pollination model does not consider the size of habitat patches when estimating pollinator abundance, so it does not take into account a minimum area required for proper development of bee populations (Tallis et al. Citation2013). Although, small patches tend to support fewer species, bees can survive in very small areas when the habitat is suitable (Kremen et al. Citation2004; Ricketts, Citation2004). Along these lines, Dymond et al. (Citation2021) suggested that to inform sustainable management of avocado pollination service it is necessary to better understand the ecology of insect pollinators and their role in avocado pollination and fruit set. Our modelling approach provides predictions of broad patterns of bee abundance and avocado yields and how they correlate with natural vegetation patches.
It is important to note that the generalised linear models performed explain only 23% of the variation in native bee abundance and 29% of the variation in crop yield. This indicates that there might be other variables influencing pollination services, bee abundance and crop yields in agricultural landscapes. These could include other landscape variables, climatic variables, geographic variables and information on agricultural practices. For example, considering the proportion of other land cover classes in the analysis may be of relevance given that they may differ in their suitability as habitat for pollinators (Kennedy et al. Citation2013). Another important variable to consider is the distance of crops to these different coverages, and especially to native vegetation covers, since it has been shown that greater distances to areas of natural vegetation reduces pollinators visitation rates, fruit set and crop productivity (Ricketts et al. Citation2008; Garibaldi et al. Citation2011). Floristic composition and climatic variables can also be important components behind variation in bee abundance and crop production (De Assis et al. Citation2020; Dymond et al. Citation2021). In particular, climate fluctuation could influence both variables considering the mega drought affecting central Chile and its impact on nectar and honey bee production (Gajardo-Rojas et al. Citation2022). Altitude is also an important variable to consider, especially considering the observation that areas with higher pollination supply were found in mountainous areas. Other variables such as pesticide use or fertiliser application may also affect the presence of pollinators (IPBES Citation2016). Future studies incorporating some of the above mentioned variables could provide additional insights for conservation and management strategies. Finally, it is also important to note that mapping of vegetation cover in Chile (Zhao et al. Citation2016) and the avocado crops has been carried out using remote sensing techniques, which has a degree of uncertainty associated (Richards and Richards Citation2022).
4.4. Implications for land-use planning and sustainable agriculture
This work provides evidence on the dependence relationships between avocado crop productivity, native vegetation and native pollinators, highlighting the importance of conserving and restoring areas of native vegetation near crops, and the urgency of considering these relationships in territorial management and planning to safeguard wild pollinator populations, agricultural productivity and food security.
The modelled pollination maps can inform the adoption of practices for sustainable agriculture and the configuration and composition planning of agricultural landscapes. For example, identifying priority areas for regeneration and restoration (Viana et al. Citation2012), or defining strategic areas for crop establishment that minimise natural habitat loss while maximising adequate provision of ecosystem services to ensure production and yield. Landscape planning is especially relevant in the study area, which is part of a biodiversity hotspot with little protection (Arroyo et al. Citation2008), and that is strongly threatened by the expansion of avocado crops and other agricultural uses. Considering that 70% of Chilean bee species are endemic (Montalva and Ruz Citation2010), and that the study area has a high projected richness of wild bee species (Orr et al. Citation2021), the information generated in this work is relevant for their conservation, as it identifies the key areas where they are most abundant, and where in the landscape the resources for their survival and maintenance are located or lacking. Furthermore, our results indicate the existence of a hotspot of native bee biodiversity in the western part of the Aconcagua river basin.
To ensure the continued provision of pollination services to crops and to maintain the ecological balance, it is essential to implement conservation strategies that support wild pollinators (Aizen and Harder Citation2009). Our study emphasises the significance of preserving native vegetation cover and creating pollinator-friendly landscapes in agricultural regions. Such practices could include the establishment of native vegetation corridors, the adoption of agroforestry systems, and the promotion of diversified farming practices (Steffan-Dewenter et al. Citation2002; Winfree et al. Citation2018). Additionally, targeted educational and awareness programs for farmers and policymakers are necessary to encourage sustainable land-use decisions that integrate both agricultural productivity and ecological conservation. Along these lines, pollination maps are an important and easy-to-use visual tool for dissemination and awareness-raising among stakeholders.
5. Conclusions
Our study provides critical insights about the interdependence of native vegetation cover, wild pollinators, and avocado yield in Central Chile. The modelled pollination maps allows advancing our knowledge about the spatial patterns of distribution of native pollinators and the pollination services they provide. The proportion of native vegetation around avocado crops would represent the main predictor of native bees abundance in crops, positively influencing their yield. The results revealed that the abundance of native bees and the pollination services they provide in the Aconcagua river basin and in avocado crops is strongly linked to the composition of the landscape in terms of native vegetation formations. Our results revealed a spatial mismatch between the areas supplying pollination services and the locations where these services are most needed, i.e. the avocado crop fields. The results emphasise the importance of conserving natural habitats to support pollinator populations and secure food production in agroecosystems. Sustainable land-use planning that considers the requirements of wild pollinators and promotes biodiversity-friendly practices is imperative for the long-term viability of agriculture in the region.
Supplemental Material
Download PDF (326.2 KB)Acknowledgements
We thank Francisca Cárcamo Rojas for her contribution designing the graphical abstract.
Disclosure statement
No potential conflict of interest was reported by the author(s).
Data availability statement
The authors confirm that the data supporting the findings of this study are mostly available within the article and its supplementary materials. More details of the data are available from the corresponding author [UL], upon reasonable request.
Supplementary material
Supplemental data for this article can be accessed online at https://doi.org/10.1080/26395916.2024.2358471
Additional information
Funding
References
- Afik O, Dag A, Shafir S. 2006. The effect of avocado (Persea americana) nectar composition on its attractiveness to honey bees (Apis mellifera). Apidologie. 37(3):317–15. doi: 10.1051/apido:2005064.
- Aizen MA, Garibaldi LA, Cunningham SA, Klein AM. 2008. Long-term global trends in crop yield and production reveal no current pollination shortage but increasing pollinator dependency. Curr Biol. 18(20):1572–1575. doi: 10.1016/j.cub.2008.08.066.
- Aizen MA, Harder LD. 2009. The global stock of domesticated honey bees is growing slower than agricultural demand for pollination. Curr Biol. 19(11):915–918. doi: 10.1016/j.cub.2009.03.071.
- Armesto JJ, Manuschevich D, Mora A, Smith-Ramirez C, Rozzi R, Abarzúa AM, Marquet PA. 2010. From the Holocene to the anthropocene: A historical framework for land cover change in southwestern South America in the past 15,000 years. Land Use Policy. 27(2):148–160. doi: 10.1016/j.landusepol.2009.07.006.
- Arroyo MTK, Marquet P, Marticorena C, Simonetti J, Cavieres L, Squeo F, Simonetti Zambelli, JA, Rozzi, R, Massardo, F. 2008. El hotspot chileno, prioridad mundial para la conservación. In: Rovira J, Ugalde J, Stutzin M, editors. 2nd ed. Biodiversidad de Chile, Patrimonio y Desafíos. Santiago, Chile: Ocho libros editores ltda; p. 90–93.
- Basualdo M, Cavigliasso P, de Avila RS Jr, Aldea-Sánchez P, Correa-Benítez A, Harms JM, Ramos AK, Rojas-Bravo V, Salvarrey S. 2022. Current status and economic value of insect-pollinated dependent crops in Latin America. Ecol Econ. 196:107395. doi: 10.1016/j.ecolecon.2022.107395.
- Bukovinszky T, Verheijen J, Zwerver S, Klop E, Biesmeijer JC, Wäckers FL, Prins HHT, Kleijn D. 2017. Exploring the relationships between landscape complexity, wild bee species richness and reproduction, and pollination services along a complexity gradient in the Netherlands. Biol Conserv. 214:312–319. doi: 10.1016/j.biocon.2017.08.027.
- Cariveau DP, Nayak GK, Bartomeus I, Zientek J, Ascher JS, Gibbs J, Winfree R. 2016. The allometry of bee proboscis length and its uses in ecology. PLOS ONE. 11(3):e0151482. doi: 10.1371/journal.pone.0151482.
- Carré G, Roche P, Chifflet R, Morison N, Bommarco R, Harrison-Cripps J, Krewenka K, Potts SG, Roberts SPM, Rodet G, et al. 2009. Landscape context and habitat type as drivers of bee diversity in European annual crops. Agricult Ecosys Environ. 133(1–2):40–47. doi: 10.1016/j.agee.2009.05.001.
- CBD. 2018. Decision adopted by the conference of the parties to the convention on biological diversity: conservation and sustainable use of pollinators. Montreal, Canada: Secretariat of the Convention on Biological Diversity.
- Celis-Diez JL, García CB, Armesto JJ, Abades S, Garratt MPD, Fontúrbel FE. 2023. Wild floral visitors are more important than honeybees as pollinators of avocado crops. Agronomy. 13(7):1722. doi: 10.3390/agronomy13071722.
- Centro de Cambio Global UC. 2019. Análisis Situacional: Principales problemas en seis cuencas de Chile. In: Documento de trabajo en el marco de la iniciativa EH2030. Santiago, Chile: Centro de Cambio Global UC.
- De Assis JC, Toppa RH, Martines MR, Arena MVN, da Silva Souza M, Carneiro LT, da Silva-Zacarin ECM, da Silva-Zacarin ECM. 2020. The influence of climate and seasonality on bee communities: a complementary method for bee sampling in forest patches of an anthropic matrix. Int J Trop Insect Sci. 41(1):711–723. doi: 10.1007/s42690-020-00261-1.
- DGA. 2004. Diagnóstico y clasificación de los cursos y cuerpos de agua según objetivos de calidad: cuenca el río Aconcagua. Santiago, Chile: Ministerio de Obras Públicas, Gobierno de Chile.
- DGA. 2022. Planilla decretos zonas de escasez hídrica (2008 – 2022). Santiago, Chile: Ministerio de Obras Públicas, Gobierno de Chile.
- Dymond K, Celis-Diez JL, Potts SG, Howlett BG, Willcox BK, Garratt MPD. 2021. The role of insect pollinators in avocado production: A global review. J Appl Entomol. 145(5):369–383. doi: 10.1111/jen.12869.
- Estrada-Carmona N, Sánchez AC, Remans R, Jones SK. 2022. Complex agricultural landscapes host more biodiversity than simple ones: A global meta-analysis. Proc Natl Acad Sci USA. 119(38):e2203385119. doi: 10.1073/pnas.2203385119.
- Fahrig L, Baudry J, Brotons L, Burel FG, Crist TO, Fuller RJ, Sirami C, Siriwardena GM, Martin J-L. 2011. Functional landscape heterogeneity and animal biodiversity in agricultural landscapes. Ecol Lett. 14(2):101–112. doi: 10.1111/j.1461-0248.2010.01559.x.
- Flores C. 2020. Modelación Operacional del Río Aconcagua. Santiago, Chile: Dirección general de aguas, Ministerios de obras públicas, Gobierno de Chile.
- Gajardo-Rojas M, Muñoz AA, Barichivich J, Klock-Barría K, Gayo EM, Fontúrbel FE, Olea M, Lucas CM, Veas C. 2022. Declining honey production and beekeeper adaptation to climate change in Chile. Prog Phys Geogr: Earth Environ. 46(5):737–756. doi: 10.1177/03091333221093757.
- García CB, Chávez-Cárdenas DS, Olmos-Moya N, Neaman A, Celis-Diez JL. 2021. Importancia de insectos silvestres en la polinización del palto cultivado bajo manejo orgánico y convencional en Chile central. Agro Sur. 1(1):13–19%V 49. doi: 10.4206/agrosur.2021.v49n1-03.
- Gardiazabal F. 2001. Historia y desarrollo del palto en Chile. In: California Avocado Society 2001 Yearbook. California, USA: California Avocado Society; p. 93–112.
- Garibaldi LA, Steffan-Dewenter I, Kremen C, Morales JM, Bommarco R, Cunningham SA, Carvalheiro LG, Chacoff NP, Dudenhöffer JH, Greenleaf SS, et al. 2011. Stability of pollination services decreases with isolation from natural areas despite honey bee visits. Ecol Lett. 14(10):1062–1072. doi: 10.1111/j.1461-0248.2011.01669.x.
- Garibaldi LA, Steffan-Dewenter I, Winfree R, Aizen MA, Bommarco R, Cunningham SA, Kremen C, Carvalheiro LG, Harder LD, Afik O, et al. 2013. Wild pollinators enhance fruit set of crops regardless of honey bee abundance. Science. 339(6127):1608–1611. doi: 10.1126/science.1230200.
- Goulson D, Nicholls E, BotíBotíAs C, Rotheray EL. 2015. Bee declines driven by combined stress from parasites, pesticides, and lack of flowers. Science. 347(6229):1255957. doi: 10.1126/science.1255957.
- Grab H, Branstetter MG, Amon N, Urban-Mead KR, Park MG, Gibbs J, Blitzer EJ, Poveda K, Loeb G, Danforth BN, et al. 2019. Agriculturally dominated landscapes reduce bee phylogenetic diversity and pollination services. Science. 363(6424):282–284. doi: 10.1126/science.aat6016.
- Greenleaf SS, Williams NM, Winfree R, Kremen C. 2007. Bee foraging ranges and their relationship to body size. Oecologia. 153(3):589–596. doi: 10.1007/s00442-007-0752-9.
- Guevara W, Hidalgo-Alcázar C, Rojas JL. 2021. Análisis de la agroindustria chilena del aguacate (Palta) en el mercado internacional. Chil J Agric Anim Sci. 37(1):54–64. doi: 10.29393/CHJAAS37-6AAWG30006.
- IPBES. 2016. The assessment report of the intergovernmental science-policy platform on biodiversity and ecosystem services on pollinators, pollination and food production. In: Potts SG, Imperatriz-Fonseca VL & Ngo HT, editors. Bonn, Germany: IPBES secretariat. doi: 10.5281/zenodo.3402856.
- IPBES. 2019. Global assessment report on biodiversity and ecosystem services of the intergovernmental science-policy platform on biodiversity and ecosystem services. E.S. Brondizio, J. Settele, S. Díaz & H.T. Ngo, editors. Bonn, Germany: IPBES secretariat.
- Kennedy CM, Lonsdorf E, Neel MC, Williams NM, Ricketts TH, Winfree R, Bommarco R, Brittain C, Burley AL, Cariveau D, et al. 2013. A global quantitative synthesis of local and landscape effects on wild bee pollinators in agroecosystems. Ecol Lett. 16(5):584–599. doi: 10.1111/ele.12082.
- Klein A-M, Vaissière BE, Cane JH, Steffan-Dewenter I, Cunningham SA, Kremen C, Tscharntke T. 2007. Importance of pollinators in changing landscapes for world crops. Proc R Soc B. 274(1608):303. doi: 10.1098/rspb.2006.3721.
- Koh I, Lonsdorf EV, Williams NM, Brittain C, Isaacs R, Gibbs J, Ricketts TH. 2016. Modeling the status, trends, and impacts of wild bee abundance in the United States. Proc Natl Acad Sci USA. 113(1):140–145. doi: 10.1073/pnas.1517685113.
- Kremen C, Williams NM, Bugg RL, Fay JP, Thorp RW. 2004. The area requirements of an ecosystem service: crop pollination by native bee communities in California. Ecol Lett. 7(11):1109–1119. doi: 10.1111/j.1461-0248.2004.00662.x.
- Kremen C, Williams NM, Thorp RW. 2002. Crop pollination from native bees at risk from agricultural intensification. Proc Natl Acad Sci. 99(26):16812–16816. doi: 10.1073/pnas.262413599.
- Magrach A, Sanz MJ, Harris J. 2020. Environmental and social consequences of the increase in the demand for ‘superfoods’ world-wide. People Nat. 2(2):267–278. doi: 10.1002/pan3.10085.
- MMA. 2019. Áreas de preservación Ecológica. Santiago, Chile: Ministerio de medio ambiente, Gobierno de Chile.
- MMA. 2020. Pisos vegetacionales de Luebert y Pliscoff 2017. Santiago, Chile: Ministerio de medio ambiente, Gobierno de Chile.
- Montalva J, Ruz L. 2010. Actualización sistemática de las abejas chilenas (Hymenoptera: Apoidea). Revista chilena Entomología. 35:15–52.
- Monzón VH, Avendaño-Soto P, Araujo RO, Garrido R, Mesquita-Neto JN. 2020. Avocado crops as a floral resource for native bees of Chile. Rev Chil Hist Nat. 93(1):5. doi: 10.1186/s40693-020-00092-x.
- Myers N, Mittermeier RA, Mittermeier CG, da Fonseca GAB, Kent J. 2000. Biodiversity hotspots for conservation priorities. Nature. 403(6772):853–858. doi: 10.1038/35002501.
- Ollerton J, Winfree R, Tarrant S. 2011. How many flowering plants are pollinated by animals? Oikos. 120(3):321–326. doi: 10.1111/j.1600-0706.2010.18644.x.
- Orr MC, Hughes AC, Chesters D, Pickering J, Zhu C-D, Ascher JS. 2021. Global patterns and drivers of bee distribution. Curr Biol. 31(3):451–458.e4. doi: 10.1016/j.cub.2020.10.053.
- Pérez Giraldo L. 2021. Efectos de las Apoidea nativas sobre la polinización y rendimiento de huertos de palto a escala local y de paisaje en la Región Metropolitana. Chile. https://repositorio.uchile.cl/handle/2250/182956.
- Potts SG, Roberts SPM, Dean R, Marris G, Brown MA, Jones R, Neumann P, Settele J. 2010. Declines of managed honey bees and beekeepers in Europe. J Apic Res. 49(1):15–22. doi: 10.3896/IBRA.1.49.1.02.
- QGIS Development Team. 2022. QGIS geographic information system. Open Source Geospatial Found Proj. http://www.qgis.org.
- Rahimi E, Barghjelveh S, Dong P. 2021. Using the lonsdorf model for estimating habitat loss and fragmentation effects on pollination service. Ecol Processes. 10(1):22. doi: 10.1186/s13717-021-00291-8.
- Richards JA, Richards JA. 2022. Remote sensing digital image analysis Vol. 5. Berlin/Heidelberg, Germany: springer.
- Ricketts TH. 2004. Tropical forest fragments enhance pollinator activity in nearby coffee crops. Biol Conserv. 18(5):1262–1271. doi: 10.1111/j.1523-1739.2004.00227.
- Ricketts TH, Regetz J, Steffan-Dewenter I, Cunningham SA, Kremen C, Bogdanski A, Gemmill‐Herren B, Greenleaf SS, Klein AM, Mayfield MM, et al. 2008. Landscape effects on crop pollination services: are there general patterns? Ecol Lett. 11(5):499–515. doi: 10.1111/j.1461-0248.2008.01157.x.
- Rodríguez S, Acuña I, Escanilla C. 2022. Polinización Sostenible: Adaptación al cambio climático para la producción de fruta en Chile. Valparaíso, Chile: Centro de Biotecnología de Sistemas, Universidad Andrés Bello. https://bibliotecadigital.ciren.cl/handle/20.500.13082/148269.
- RStudio-Team. 2020. RStudio: integrated development environment for R. Boston (MA): RStudio, Inc.
- Schulz JJ, Cayuela L, Echeverria C, Salas J, Rey Benayas JM. 2010. Monitoring land cover change of the dryland forest landscape of Central Chile (1975–2008). Appl Geogr. 30(3):436–447. doi: 10.1016/j.apgeog.2009.12.003.
- Sharp R, Chaplin-Kramer R, Wood S, Guerry A, Tallis H, Ricketts T. 2020. InVEST user’s guide. California (USA): The Natural Capital Project, Stanford University, University of Minnesota, The Nature Conservancy, and World Wildlife Fund.
- Soto Bäuerle MV, Arriagada González J, Castro Correa CP, Maerker M, Rodolfi G. 2011. Relación entre el cambio de uso del suelo en la cuenca del Aconcagua y su litoral arenoso correlativo: Chile central. Rev Geogr Norte Gd. (50):187–202. doi: 10.4067/S0718-34022011000300011.
- Steffan-Dewenter I, Münzenberg U, Bürger C, Thies C, Tscharntke T. 2002. Scale-dependent effects of landscape context on three pollinator guilds. Ecology. 83(5):1421–1432. doi: 10.1890/0012-9658(2002)083[1421:SDEOLC]2.0.CO;2.
- Tallis HT, Ricketts T, Guerry A, Wood SA, Sharp R, Nelson E 2013. InVEST 2.5. 6 user’s guide. California (USA): The Natural Capital Project: Stanford.
- Tscharntke T, Grass I, Wanger TC, Westphal C, Batáry P. 2021. Beyond organic farming – harnessing biodiversity-friendly landscapes. Trends Ecol Evol. 36(10):919–930. doi: 10.1016/j.tree.2021.06.010.
- Tscharntke T, Klein AM, Kruess A, Steffan-Dewenter I, Thies C. 2005. Landscape perspectives on agricultural intensification and biodiversity – ecosystem service management. Ecol Lett. 8(8):857–874. doi: 10.1111/j.1461-0248.2005.00782.x.
- Viana BF, Boscolo D, Mariano Neto EMGC, Lopes L, Lopes A, Fereira P, Pigozzo CM, Primo L. 2012. How well do we understand landscape effects on pollinators and pollination services? J Pollinat Ecol. 7. doi: 10.26786/1920-7603(2012)2.
- Wentling C, Campos FS, David J, Cabral P. 2021. Pollination potential in Portugal: Leveraging an ecosystem service for sustainable agricultural productivity. Land. 10(4):431. doi: 10.3390/land10040431.
- Westrich P, International Bee Research, A. and Linnean Society of, L. 1996. Habitat requirements of central European bees and the problems of partial habitats. In: Symposium, Conservation of bees. London, England: Academic Press; p. 1–16.
- Williams NM, Crone EE, Roulston TAH, Minckley RL, Packer L, Potts SG. 2010. Ecological and life-history traits predict bee species responses to environmental disturbances. Biol Conserv. 143(10):2280–2291. doi: 10.1016/j.biocon.2010.03.024.
- Winfree R, Bartomeus I, Cariveau DP. 2011. Native pollinators in anthropogenic habitats. Annu Rev Ecol Evol Syst. 42(1):1–22. doi: 10.1146/annurev-ecolsys-102710-145042.
- Winfree R, Reilly JR, Bartomeus I, Cariveau DP, Williams NM, Gibbs J. 2018. Species turnover promotes the importance of bee diversity for crop pollination at regional scales. Science. 359(6377):791–793. doi: 10.1126/science.aao2117.
- Winfree R, Williams NM, Gaines H, Ascher JS, Kremen C. 2008. Wild bee pollinators provide the majority of crop visitation across land-use gradients in new jersey and Pennsylvania, USA. J Appl Ecol. 45(3):793–802. doi: 10.1111/j.1365-2664.2007.01418.x.
- Zhao Y, Feng D, Yu L, Wang X, Chen Y, Bai Y, Hernández HJ, Galleguillos M, Estades C, Biging GS, et al. 2016. Detailed dynamic land cover mapping of Chile: accuracy improvement by integrating multi-temporal data. Remote Sens Environ. 183:170–185. doi: 10.1016/j.rse.2016.05.016.