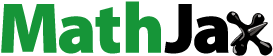
ABSTRACT
Nasal filters are increasingly used in reducing personal haze exposures, but there is no retrievable evidence on its efficiency. In this study, a nasal filter testing system was designed. NaCl aerosols were generated to simulate PM1. Three different sizes of a new model of nasal filter were tested under two flow types (constant vs. cyclic) and three flow rates (15, 30, and 50 L/min). All PM1 penetration values were > 80%. Either enlarging filter size or lowering flow rate significantly reduced PM1 penetration, and PM1 penetration under cyclic flow was significantly higher compared with constant flow. To avoid mouth breathing, nasal filters could only be worn under light work-rates or rest conditions. The quality factor of nasal filters was only hundredths that of the N95 and P100 FFRs. This study reveals that there is a future need to design nasal filter materials with higher efficiency and lower flow resistance.
Introduction
In China, due to rapid urbanization and sharp increase of fossil energy consumption, more than 99% of the top 500 largest cities, at least 30% land areas, and nearly 800 million people are suffering from frequently (>100 days per year) severe haze pollution [Citation1]. Various studies have shown that air pollution may increase respiratory diseases, cardiovascular and cerebrovascular cases, lung cancer deaths, and overall mortality [Citation1–5]. Moreover, it was reported that around 1.2 million premature deaths per year were caused by air pollution in China, accounting for nearly 40% of this kind of global deaths [Citation6]; correspondingly, the health-related economic losses caused by haze pollution were estimated to be as high as 87 billion dollars per year [Citation6]. Air pollution or PM2.5 (particulate matters with an aerodynamic diameter ≤ 2.5 μm) related adverse health effects in other countries such as America were also not optimistic. It was estimated that air pollutants cause 200,000 early deaths per year in the U.S [Citation7].
Faced with such a grim situation, global governments and scientists have devoted themselves in searching for the ‘real killer’ that threatens human health. Long-term epidemiological studies revealed that there is a close relationship between the mortality of urban residents and the size depended atmospheric particulate concentrations; the smaller the particle size the higher the mortality would be [Citation8]. As a subpart of PM2.5, PM1 (particulate matters with an aerodynamic diameter ≤ 1 μm), with the range of size covers the wavelength of visible light (0.4–0.7 μm), played an important role in daylight extinction and may be the key factor in the formation of haze [Citation9]. It was found that the PM1 mass concentration during haze days was several times higher than that of ‘non-haze’ days [Citation10]; meantime, haze pollution was an indicator of the existence of high number concentration of PM1 [Citation4,Citation11]. Compared with PM2.5, PM1 features a larger surface area-to-mass ratio, thus carrying more toxic pollutants (such as heavy metals, PAHs, bacteria, etc.); with a smaller particle size, PM1 could penetrate deeper into human lungs [Citation12]. It has been concluded that the PM1 constituted most of the ambient particulates (both mass and number concentrations) during haze days [Citation10,Citation11], and there is a statistical association between adverse health effects and PM1 [Citation13]. Therefore, the high number concentration of PM1 may be the ‘real killer’ during the occurrence of haze pollution [Citation4].
Since haze pollution affects all population, the concept of respiratory protection has been extended from industrial sectors and healthcare environment to the whole society, everyone needs to choose an effective respiratory protective equipment (RPE) during haze days’ commuting, especially those who work outdoors such as traffic policemen, construction workers, street cleaners, etc. Traditionally, face masks such as filtering facepiece respirators (FFRs) are widely used by people to reduce air pollutant exposure. However, there are several limitations associated with FFRs that may deter common people from wearing them: 1) FFRs are not comfortable to use [Citation14]; 2) face masks may cause fogs on eyeglasses especially in winter days; 3) there is a muffling effect on voice and influence on cosmetic.
Since people breath 90% of air through their nose [Citation15], nasal filters are developed to augment the filtering efficacy of nose by inserting two pieces of miniature filter into the nasal cavity. Compared with traditional face masks, nasal filters feature several advantages: 1) nasal filters are invisible (or less obvious) from outside which makes them more cosmetically appealing; 2) wearing nasal filters could avoid the problem of fogging on glasses; 3) the muffling effect on face-to-face communication can be lessened. In fact, it has been demonstrated that the foreign body sensation caused by nasal filters was mild/minimal; although some of the wearers may experience a nasal twang, the influence generally disappeared within 1–2 days [Citation16]. Therefore, nasal filters may be a practical and comfortable alternative to traditional face masks to prevent against air pollution.
Although it is claimed by the manufactures that nasal filters can effectively protect against air pollutants (filtration efficiency>90%), till now, except for several investigations regarding the effectiveness of nasal filters in reducing pollen allergy and improving life quality of wearers [Citation17,Citation18]; there is no traceable evidence on the efficacy of the nasal filters in protecting against PM1, which is assumed to be the ‘real killer’ during haze days. Therefore, utilizing a self-designed nasal filter testing system, this study aimed at assessing the performance of a new model of nasal filter when challenged with PM1 aerosols.
Materials and methods
Tested nasal filters
One new model of nasal filter designed to protect against air pollutants was tested in this study. As presented in , the nasal filter consists of twin flexible filter holders that are bridged by a thin translucent plastic bar (connector). There is a retainer ring in each frame to secure the filter. The twin filter membranes are made of electret polypropylene fibers to enhance the filtration efficiency. The nasal filter membrane in shape is similar with human nostril, with a thickness of 1 mm. The two filter holders are soft and comfortable that can be inserted into both nostrils. The connector that holds the twin filters in place could prevent the filters from being pushed too far into the nasal vestibule, which also provides a means of insertion and removal of the nasal filter. Since the connector is translucent and located at the bottom of the nose, it is almost virtually invisible. Above stated filter material and design was quite similar for the tested and many other brands of nasal filters. In this study, to accommodate various sizes of nostrils, three different sizes of the tested nasal filter (one pair each: small, 88 mm2 × 2; medium, 110 mm2 × 2; large, 134 mm2 × 2) were purchased from amazon.com, and under each combination of experimental condition, a brand-new nasal filter was tested. Therefore, six specimens of each size and totally 18 nasal filters were tested in this study.
Figure 1. Schematic diagram of the experimental set-up [modified from Citation23]
![Figure 1. Schematic diagram of the experimental set-up [modified from Citation23]](/cms/asset/b56c0fda-88f4-485b-98ee-d38382521e08/tcsb_a_1991847_f0001_oc.jpg)
Challenge aerosols
Sodium chloride (NaCl) aerosols (0.02–1 μm) were used as the challenge surrogate for PM1. The submicron NaCl aerosols were generated by a constant output particle generator (Model 8026, TSI Inc., Shoreview, Minn., USA) using 2% NaCl solution and fed into a nasal filter testing chamber (see ). The testing chamber is 1.2 × 1.2 × 1.2 m3 and constructed by synthetic resin. Four fans were placed at the four corners of the chamber to circle the air to assure the generated aerosols were uniformly distributed. During each test, the particle generator was operated continuously at an output flow of 0.5 L/min to maintain a stable concentration level of 30,000 ± 300 particles/cm3, representing the average PM1 number concentration in the urban area of Beijing [Citation11].
Experimental set-up
The performance of the tested nasal filters was assessed in two aspects: 1) the filtration efficiency, which was determined by the PM1 penetration, and 2) the comfort of the filter, which was represented by the breathing resistance (Rf). National Institute for Occupational Safety and Health (NIOSH)-approved FFRs are certified under 85 L/min constant flow [Citation19]. However, actual human breathing patterns more closely resemble a sinusoidal waveform (cyclic flow) [Citation20]. Furthermore, several studies reported that at the same test flow rates, particle penetration under cyclic flow may be higher than that of constant flow [Citation21–23], which suggests that the cyclic flow may be a more stringent test flow regime. For a FFR, it is typically associated with a higher Rf, leading to the well-known unwillingness of the users to wear [Citation14]. A good model of nasal filter should combine high efficiency with low resistance; however, the resistance and filter efficiency often conflict with each other. Comprehensively considering these two, the overall performance of a filter can be assessed by the quality factor (qf) [Citation24]. Higher qf value corresponds to better overall performance of nasal filter.
Based on the analyses above, a nasal filter testing system was modified from Citation23, as shown in . The tested nasal filters were inserted into two thin copper tubes (simulated as different size human nostrils). The interface between the frame of the filter and the wall of the tubes was fully sealed by silicone. The ends of the two thin copper tubes were connected to a large diameter copper pipe (simulating the upper human respiratory tract). The pipe was then connected to either a vacuum system or a breathing simulator (Series 1101, Hans Rudolph, Inc., Shawnee, KS, USA) to introduce constant or cyclic test flow. Aerosol samples (0.02–1 μm) from outside and inside the tested nasal filter were taken by a P-Trak Ultrafine Particle Counter (Model 8525, TSI Inc., Shoreview, Minn., USA) for PM1 number concentration analysis. Detail specifications for related equipment in this study were presented in .
Table 1. Detail specifications for related equipment in this study
As expressed by EquationEquation (1)(1)
(1) [Citation24], to achieve isokinetic sampling results, based on the sampling flow of the P-Trak (Qsample, 0.1 L/min) and the inner diameter of the sampling tube (Dsample, 6 mm), different inner diameters of copper pipes (Dpipe) 73, 104 and 134 mm were designed and applied to match different test flow rates (Qtest) 15, 30, and 50 L/min.
Test conditions
All three sizes of the nasal filter were tested under the fully sealed condition. To better simulate various human breathing efforts, sinusoidal cyclic flows with mean inspiratory flow (MIF) rates of 15, 30, and 50 L/min (breathing frequency = 15, 20, and 25 breaths/min, respectively) were applied [Citation25,Citation26]. To investigate the effect of the flow type on the PM1 penetration, the above-specified three flow rates were also performed at constant flow regime. A summary of the test conditions was shown in . For each tested nasal filter, the two flow types, three flow rates, and four replicates were fully randomized to minimize the experimental error. The PM1 penetration, Rf, and qf were calculated by the following EquationEquations (2)(2)
(2) -(Equation4
(4)
(4) ) to assess the protection, comfort, and overall performance of the nasal filters, respectively.
Table 2. Summary of the experimental conditions
PM1 penetration (P%)
The PM1 penetration (P%) was determined as the ratio of inside (Cin) to outside PM1 concentration (Cout) [Citation27–29]:
where Cin and Cout were the PM1 number concentration inside and outside of the tested nasal filters, particles/cm3.
Breathing resistance (Rf)
Rf was determined as the difference between the pressure outside (Pout) and inside (Pin) of the nasal filter when constant flow was applied [Citation27–29]. The Rf was obtained by a manometer (AirflowTM PVM100, TSI Inc., Shoreview, Minn., USA).
where Pin and Pout were the air pressure inside and outside of the tested nasal filters, Pa.
Quality factor (qf)
Comprehensively considering PM1 penetration and Rf, the overall performance of a nasal filter was assessed by the qf [Citation24,Citation29]. The higher the qf value, the better the overall performance of a nasal filter:
where qf was the quality factor of the tested nasal filters, Pa−1.
Data analysis
For each combination of the experimental conditions, the mean value and standard deviation of the PM1 penetration, Rf, and qf were calculated from the four replicates. Normal distribution test was performed. Three-way Analysis of Variance (ANOVA) was applied to investigate the effects of filter size, flow type, and flow rate on the PM1 penetration. Two-way ANOVA was used to study the significance of filter size and flow rate for the Rf and qf. Pairwise comparisons were performed by Tukey’s range test. All statistical data analysis was conducted using SAS version 9.3 (SAS Institute Inc., Cary, NC). p-values < 0.05 were considered significant.
Results
PM1 penetration
The mean PM1 penetration values found for all nasal filters under different testing airflows were presented in . Three-way ANOVA revealed that filter size, flow type, and flow rate all had a significant effect on the PM1 penetration (p < 0.0001). As can be seen in , all PM1 penetration values were >80%; for the large size nasal filter (performed the best), the minimum penetration under the 15 L/min constant flow was around 83%, while the corresponding value at 15 L/min cyclic flow was nearly 90%. The reported data suggested that the tested nasal filters had a limited protection efficiency against PM1 aerosols.
Table 3. Mean PM1 penetration for three sizes nasal filters under different testing airflows
The pairwise comparison results for three sizes nasal filters, two flow types, and three flow rates were presented in . As expected, the PM1 penetration values obtained from the three filter sizes were all significantly different from each other (p < 0.05), the larger the filter size the lower the PM1 penetration value, indicating that filters with a bigger surface area provided a lower face velocity, and therefore a higher protection level. In addition, the PM1 penetration values under cyclic flows were found to be significantly higher than that of constant flows (p < 0.05), which was consistent with previous studies [Citation21–23]. This finding suggests that the sinusoidal waveform, which better reflects a real human breathing pattern, is a more stringent and realistic flow regime to test the efficacy of a nasal filter. Meantime, it was found that under either constant or cyclic flow condition, increasing the test flow rate (or face velocity) would result in a significant increase of the PM1 penetration (p < 0.05). A similar conclusion has been drawn by many other scholars when testing the 95 and P100 FFRs or other types of respirators [Citation22,Citation23,Citation27–32].
Table 4. Pairwise multiple comparisons: mean PM1 penetration among three sizes nasal filters under different testing airflows (ANOVA with Tukey’s Range Test)
Flow resistance (Rf)
Rf is an important parameter to characterize the performance of various types of respirators [Citation27–29]. This study was the first of its kind to quantitively evaluate the Rf of a nasal filter. Unlike traditional respirators, for a nasal filter to function properly, wearers must breathe in through the nose only. However, higher Rf may lead the wearers to breathing through their mouth without any respiratory protection. The Rf values of the nasal filters under different constant flow rates are presented in . Two-way ANOVA showed that both filter size and flow rate were statistically significant (p < 0.0001). Pair-wise multiple-comparison analysis revealed that for each specific filter size, as the breathing flow increased, the Rf across the nasal filter increased significantly (p < 0.05). On the other hand, increasing the filter size resulted in a decrease in the face velocity and therefore a significant reduce in the average Rf values for all testing flow rates (p < 0.05).
Table 5. Flow resistance (Rf) of three sizes nasal filters under different constant flow rates
Previous studies [Citation33,Citation34] have demonstrated that when the nasal airway resistance was greater than 3.5 cm H2O/L/s, people began to feel difficulties in nasal breathing; when resistance exceeded 4.5 cm H2O/L/s, a significant number of subjects would inhale through their mouth. As listed in , even at the 15 L/min lowest test flow (corresponding to the minute air volume required by a medium sized person when performing light work activities, as defined in ISO/TS 16976–1:2015) [Citation35], the Rf across the small size nasal filter was > 4.5 cm H2O/L/s, suggesting that wearers may tend to execute mouth breathing. For the medium and large sizes nasal filters, users may feel nasal airflow restriction at the breathing flow of 15 L/min; when inhalation flow increased to 30 L/min (minute volume during moderate workload tasks, as listed in ISO/TS 16976–1:2015) [Citation35], wearers may breathe through their mouth. These findings revealed that the tested nasal filters may not be recommended for moderate or heavier workload conditions.
Quality factor (qf)
Based on the values of PM1 penetration and Rf reported in , qf was calculated to assess the overall performance of the tested nasal filters. The results are presented in . The higher qf value corresponds to a better overall filter performance. Two-way ANOVA showed that both filter size and flow rate significantly affected the qf (p < 0.0001). Specifically, for each pair of the tested nasal filter, the qf value decreased rapidly with increasing the flow rates (p < 0.05), which was consistent with the finding of other scholars reported for FFRs [Citation29–31]. The qf values of the larger size nasal filters were significantly higher at all breathing flow rates (p < 0.05).
Table 6. Quality factor (qf) of three sizes nasal filters and two models of N95 and P100 FFRs under different constant flow rates
To better assess the performance of the tested nasal filters, the qf values obtained in this study were compared to that of two models of N95 and P100 FFRs under the same testing conditions [data acquired from our previous study, please see detail testing information in reference Citation23]. As presented in , the qf values for those four FFRs were hundredfold higher than those of the nasal filters, which means the overall performance of the tested nasal filters was far worse than N95 and P100 FFRs.
Discussion
China has been experiencing frequently severe haze pollution, which threatens the health of an 800 million population. PM1 aerosols constitute most of haze particles [Citation10], which may be the ‘real killer’ during haze days. Assuming that in China, 800 million people wear single-use FFRs 100 days per year, one N95 respirator was one dollar, then the annual expense would be 0.8 billion dollars. The surface areas of FFRs were generally 100–300 cm2 [Citation36], while the sizes of nasal filters were 1–3 cm2, which was only hundredths of the former. Therefore, the expense on filter material would be saved hundreds of times. Since human breathe 90% of the air through the nose [Citation15], innovative nasal filters (worn inside the nose and worked as a miniature air filter) were designed to reduce the haze exposure. Currently, in several major cities of China (such as Beijing, Shanghai, Hangzhou, etc.), the tested nasal filters are increasingly used as a respiratory protection device by traffic policemen and street cleaners, though it is unknow how effective those filters are. The PM1 penetration results obtained in this study revealed that this new model of nasal filter, which was claimed to filter > 90% of PM2.5, failed to provide an adequate prevention against PM1 (penetration > 80%).
Whether nasal filters or traditional FFRs are worn, human body requires the same volume of air during certain work activities, and consequently the face velocity of airflow passing through the nasal filters should be hundredfold higher compared with the FFRs, as presented in . It has been well documented that with the increasing face velocity, the filter efficiency significantly decreases [Citation22,Citation23,Citation27–32], because high face velocity would greatly reduce the residence time for particles passing through the respirator filter, resulting in a lower probability of particles being captured by filtering mechanisms of diffusion and electrostatic attraction [Citation23,Citation27–29,Citation31]. Therefore, to reduce the PM1 penetration, there may be two ways: one is to increase the surface area of the nasal filter through various techniques such as fold methods; another solution may be reengineering the filter membrane with higher filtration efficiency and lower flow resistance.
Table 7. Face velocity of three sizes nasal filters and two models of N95 and P100 FFRs under different constant flow rates
Since the development and usage of nasal filters have just begun in recent years, there is still a lack of filtration testing standards. Current NIOSH certification test flow for FFRs is 85 L/min constant flow [Citation19], which was closely related to the minute volume of breathing under high workloads defined in ISO/TS 16976–1:2015 [Citation35]. The prerequisite of using nasal filters is that the wearer must breathe only through the nose, while under moderate or heavier physical exertions, people may change to mouth breathing (the switching point from nasal to oronasal breathing was 105 W/m2, corresponding to light-moderate work rates) [Citation35,Citation37,Citation38]. In other words, the nasal filters may only be worn under light workloads or rest conditions. Obviously, the 85 L/min test flow exceeds the light work condition, and nasal filters should be tested under a lower airflow standard. As for the test flow type, this and many other studies have demonstrated that at the same flow rate, particle penetration under sinusoidal cyclic flows (close to real human breathing pattern) was higher when compared with the constant flow [Citation21–23]. Therefore, a cyclic flow type may provide a more stringent testing condition for nasal filters.
A limitation of this study is that only one model of nasal filter was tested. In future, more models should be investigated to study if the results will differ from one model to another. In addition, the nasal filters in this study were fully sealed, while there may exist nasal seal leakage under the actual usage condition.
Conclusion
Although nasal filters may have good application prospects in reducing haze exposure. The PM1 penetration results in this study were all > 80% (significantly higher than the penetration <10% claimed by the manufacturer), suggesting that the tested nasal filters failed to offer a sufficient protection against haze particles. In addition, to avoid mouth breathing, the nasal filters may only be used under light work rates or rest conditions. Overall, the findings suggest that more efforts should be done by the manufactures to improve the filtration efficiency and guarantee the protection and comfortability of the wearer.
Disclosure statement
The authors declare that they have no competing interests.
Additional information
Funding
References
- Gao J, Woodward A, Vardoulakis S, et al. Haze, public health and mitigation measures in China: a review of the current evidence for further policy response. Sci Total Environ. 2017;578:148–157.
- Hadei M, Hopke PK, Nazari SSH, et al. Estimation of mortality and hospital admissions attributed to criteria air pollutants in tehran metropolis, iran. Aerosol Air Qual Res. 2017;17(10):2474–2481.
- Prueitt RL, Li W, Edwards L, et al. Systematic review of the association between long-term exposure to fine particulate matter and mortality. Int J Environ Health Res. 2021;1–39. DOI:https://doi.org/10.1080/09603123.2021.1901864
- Stieb DM, Shutt RH, Kauri LM, et al. Associations between air pollution and cardio-respiratory physiological measures in older adults exercising outdoors. Int J Environ Health Res. 2019;1–14. doi:https://doi.org/10.1080/09603123.2019.1699506
- Zhang J, Feng L, Hou C, et al. Interactive effect between temperature and fine particulate matter on chronic disease hospital admissions in the urban area of tianjin. China Int J Environ Health Res. 2021;31(1):75–84.
- Lim SS, Vos T, Flaxman AD, et al. A comparative risk assessment of burden of disease and injury attributable to 67 risk factors and risk factor clusters in 21 regions, 1990–2010: a systematic analysis for the global burden of disease study 2010. Lancet. 2012;380:2224–2260.
- Caiazzo F, Ashok A, Waitz IA, et al. Air pollution and early deaths in the united states. part i: Quantifying the impact of major sectors in 2005. Atmos Environ. 2013;79:198–208.
- Beelen R, Raaschou-Nielsen O, Stafoggia M, et al. Effects of long-term exposure to air pollution on natural-cause mortality: An analysis of 22 european cohorts within the multicentre escape project. Lancet. 2014;383:785–795.
- Li Y, Zhao H, Wu Y. Characteristics of particulate matter during haze and fog (pollution) episodes over northeast China, autumn 2013. Aerosol Air Qual Res. 2015;15(3):853–864.
- Xu P, Zhang J, Ji D, et al. Evaluating the effects of springtime dust storms over beijing and the associated characteristics of sub-micron aerosol. Aerosol Air Qual Res. 2017;17(3):680–692.
- Wu Z, Hu M, Lin P, et al. Particle number size distribution in the urban atmosphere of beijing, China. Atmos Environ. 2008;42(34):7967–7980.
- Gaita SM, Boman J, Gatari MJ, et al. Characterization of size-fractionated particulate matter and deposition fractions in human respiratory system in a typical african city: Nairobi, Kenya. Aerosol Air Qual Res. 2016;16(10):2378–2385.
- Pope III CA. Epidemiological basis for particulate air pollution health standards. Aerosol Sci Technol. 2000;32(1):4–14.
- Davidson CS, Green CF, Gibbs SG, et al. Performance evaluation of selected n95 respirators and surgical masks when challenged with aerosolized endospores and inert particles. J Occup Environ Hyg. 2013;10(9):461–467.
- Camner P, Bakke B. Nose or mouth breathing? Environ Res. 1980;21(2):394–398.
- Pasricha J. Use of nasal filters in naso-bronchial allergy. Indian J Pediatr. 1981;48(1):47–50.
- D’Amato G, D’amato M, Rumi G, et al. Improvement of quality of life in allergic rhinoconjunctivitis patients using nasal filters, a preliminary study. Eur Ann Allergy Clin Immunol. 2013;45:167–175.
- Kenney P, Hilberg O, Sigsgaard T. Clinical application of nasal filters: An obser vational study on the usability of nasal filters in managing seasonal allergic rhinitis. J Allergy Clin Immunol. 2016;4(3):445–452.
- National Institute for Occupational Safety and Health (NIOSH). 42 CFR part 84 respiratory protective devices: Final rules and notice. Fed Regist. 1995;60:30336–30402.
- Cooper E. A comparison of the respiratory work done against an external resistance by man and by a sine-wave pump. Exp Physiol. 1960;45(2):179–191.
- Eshbaugh JP, Gardner PD, Richardson AW, et al. N95 and P100 respirator filter efficiency under high constant and cyclic flow. J Occup Environ Hyg. 2009;6(1):52–61.
- Wang A, Richardson AW, Hofacre KC. The effect of flow pattern on collection efficiency of respirator filters. J Int Soc Respir Prot. 2012;29:41–54.
- Zhu J, He X, Guffey S, et al. Performance comparison of N95 and P100 filtering facepiece respirators with presence of artificial leakage. Ann Work Expo Health. 2020;64(2):202–216.
- Hinds WC. Respiratory Deposition in Aerosol Technology: filtration, Properties, Behaviour, and Measurement of Airborne Particles. 2nd ed., Vol. 11, New York, America: John Wiley & Sons, Inc, Publisher; 1999. p. 182–205.
- Zhi Y, Vena D, Popovic MR, et al. Detecting inspiratory flow limitation with temporal features of nasal airflow. Sleep Med. 2018;48:70–78.
- Zhu J, He X, Bergman MS, et al. A pilot study of minimum operational flow for loose-fitting powered air-purifying respirators used in healthcare cleaning services. J Occup Environ Hyg. 2019;16:440–445.
- Kumar A, Bhattacharjee B, Sangeetha DN, et al. Evaluation of filtration effectiveness of various types of facemasks following with different sterilization methods. J Ind Text. 2021;0:1–36.
- Kumar A, Sangeetha DN, Yuvaraj R, et al. Quantitative performance analysis of respiratory facemasks using atmospheric and laboratory generated aerosols following with gamma sterilization. Aerosol Air Qual Res. 2020;21(1):1–17.
- Kumar A, Subramanian V, Joshi S, et al. Assessment of N95 respirator for reuse after sterilization: Filtration efficacy, breathing resistance, quality factor, chemical structure and surface charge density. medRxiv. [Preprint] May 27, 2021. https://doi.org/https://doi.org/10.1101/2021.05.25.21257801
- Eninger RM, Honda T, Adhikari A, et al. Filter performance of N99 and N95 facepiece respirators against viruses and ultrafine particles. Ann Occup Hyg. 2008;52(5):385–396.
- Huang S, Chen C, Kuo Y, et al. Factors affecting filter penetration and quality factor of particulate respirators. Aerosol Air Qual Res. 2013;13(1):162–171.
- Mukhametzanov IT, Grinshpun SA, Zaripov SK, et al. Assessing the protection provided by facepiece filtering respirator: New model involving spherical porous layer with annular peripheral opening. Aerosol Air Qual Res. 2016;16(10):2428–2437.
- Watson RM, Warren DW, Fischer ND. Nasal resistance, skeletal classification, and mouth breathing in orthodontic patients. Am J Orthod. 1968;54(5):367–379.
- Warren DW, Hinton VA, Pillsbury HC, et al. Effects of size of the na sal airway on nasal airflow rate. Arch Otolaryngol Head Neck Surg. 1987;113(4):405–408.
- International Organization for Standardization (ISO). ISO/TS. 16976-1 respiratory protective devices-human factors. In: Part 1: Metabolic rates and respiratory flow rates. Geneva, Switzerland: ISO Copyright Office; 2015: 1-19.
- Chen S, Wang J, Bahk YK, et al. Carbon nanotube penetration through fiberglass and electret respirator filter and nucleopore filter media: experiments and models. Aerosol Sci Technol. 2014;48(10):997–1008.
- Lizal F, Elcner J, Jedelsky J, et al. The effect of oral and nasal breathing on the deposition of inhaled particles in upper and tracheobronchial airways. J Aerosol Sci. 2020;150:105649.
- Niinimaa V, Cole P, Mintz S, et al. The switching point from nasal to oronasal breathing. Respir Physiol. 1980;42(1):61–71.