Abstract
Current in vitro pancreatic lipase inhibitor screenings are based on previous spectrophotometric lipase assays. Nevertheless, they are with little evaluation of assay conditions. This study focuses on the impacts of experimental factors on enzyme activity in the assay with p-nitrophenyl palmitate as substrate by monitoring their effects on the hydrolysis rates. On the results, experimental conditions for lipase inhibitory assay were proposed. Notably, 5 mM sodium deoxycholate as emulsifier not only maintains the assay homogeneity but also enhances lipase activity. Organic co-solvents to dissolve organic inhibitors including DMSO, EtOH, MeOH, IPA, AcCN [0–30% (v/v)] was found well tolerated by the enzyme. With 10% (v/v) glycerol, lipase solutions can be stored at –20°C for up to one month without significant loss of activity. The results reported here provide researchers the assay condition sets in which most inhibitors can be dissolved, and lipase activity is not severely affected. This could accelerate the rational development of novel lipase inhibitors.
Introduction
According to the World Health Organization (Citation2021), obesity has globally tripled since 1975. If this trend continues, there are estimated over 2.7 billion overweight and 1 billion obese adults by 2025 (Obesity and Overweight [Internet] Citation2021). Obesity not only affects the body weight but also correlates with a higher risk for diet-related disorders, such as cardiovascular diseases, diabetes, cancers, and higher mortality rates (Schrauwen and Westerterp Citation2000). Especially, having obesity elevates the risk of severe illness from COVID-19 (Popkin et al. Citation2020). Most medications used for obesity long-term treatment target the appetite regulation in the central nervous system. Meanwhile, orlistat is the only over-the-counter medication (Daneschvar et al. Citation2016). Its mode of action relies on the inhibitory effect on pancreatic lipase (Daneschvar et al. Citation2016; Bialecka-Florjanczyk et al. Citation2018). Pancreatic lipase hydrolyzes ester linkages of triglycerides during the absorption of dietary fat (Winkler et al. Citation1990). Orlistat, however, possesses adverse and inconvenient side effects (Lerner and Mehler Citation2019). Since the commercialization of orlistat two decades ago, there are no new drugs with a similar mechanism getting approval. Thus, finding new pancreatic lipase inhibitory compounds has withdrawn much attention (Birari and Bhutani Citation2007; Sridhar et al. Citation2019; de la Garza et al. Citation2011; Rajan et al. Citation2020; Abdul Rahman et al. Citation2017; Newman and Cragg Citation2012; Buchholz and Melzig Citation2015; Sridhar et al. Citation2017; Sridhar et al. Citation2017; Mammal and Sankar Citation2019; Chauhan et al. Citation2019).
Drug discovery involves the identification and optimization of lead compounds through a series of structural modifications and quantitative in vitro bioactivity determination. Current in vitro pancreatic lipase inhibitory assays are mostly adopted from the previously developed analytical lipase assays using porcine pancreatic lipase. The native substrates for pancreatic lipase are long-chain triacylglycerols (Gilham and Lehner Citation2005; Lowe Citation1999). Although these compounds mimic well the native substrates, direct analysis of activity is not straightforward. Chromogenic analogs, including p-nitrophenyl acyl esters, allow direct and continuous spectrophotometric assays. Among different p-nitrophenyl acyl esters, p-nitrophenyl palmitate is often used as its long-chain palmityl group can distinguish lipase activity and esterase activity (Pinsirodom and Parkin Citation2001). p-Nitrophenol released upon enzymatic hydrolysis exhibits a yellow color, which can be conveniently monitored at 405–415 nm (Pohanka Citation2019). This method has the advantage of the low cost and feasibility at basic (bio)chemical laboratories. It yet encounters some known and recurrent complications. In particular, the formation of insoluble long-chain fatty acids after the hydrolysis can lead to a turbid solution which in turn prevents accurate spectrophotometric measurements (Gupta et al. Citation2002). Solubility limitation of organic inhibitors in lipase assay’s aqueous medium is another major issue. A common procedure is to prepare the inhibitor solution in an organic co-solvent and introduce it into the aqueous assay (Di and Kerns Citation2006). To ensure that the inhibitor is completely soluble at the highest concentration, a high percentage of organic solvent is often needed. A large amount of organic solvent may, however, interfere with the enzyme activity. Another factor to be considered is that the mechanism of action has not frequently been elucidated at the early stage of drug discovery. The incubation step of the enzyme and inhibitor can therefore affect the bioassay results. In the novel pancreatic lipase inhibitor screening studies so far, modifications of the assay conditions varied on a case-by-case basis (Newman and Cragg Citation2012; Buchholz and Melzig Citation2015; Sridhar et al. Citation2017; Sridhar et al. Citation2017; Emimmal and Sankar Citation2019). The modifications include the use of emulsifier, temperature, and organic co-solvent to introduce the inhibitors into the bioassay mixture. Nevertheless, there is no study on the effect of these modifications on enzyme activity.
In this study, we extensively evaluated the effect of experimental conditions including the use of emulsifier, co-solvents, additives in the reaction, assay temperature, incubation time, and enzyme storage conditions on the outcome of the lipase inhibition assay using p-nitrophenyl palmitate as substrate. Based on the obtained results, ranges of experimental conditions not affecting porcine pancreatic lipase activity and suitable for most basic (bio)chemical laboratories are reported with minimum instrumental requirements. This finding potentially facilitates the search for novel lipase inhibitors in obesity drug development.
Material and methods
Chemicals and reagent solutions
All chemicals were analytically graded and directly used after purchase. Porcine pancreatic lipase type II (L-3126), p-nitrophenyl palmitate (pNPP), sodium deoxycholate (SDC), Triton X-100 (TX-100), Tris·HCl, HEPES, and tricine were purchased from Sigma-Aldrich (USA) and Acros (Belgium). Inorganic salts used to prepare buffers were from Prolabo (France). Organic solvents were from Merck (USA) and Prolabo (France). Orlistat 99.4% was from Kolli Indra (India). Double distilled water was utilized in all experiments. Preparation and storage conditions of the stock solutions used in this study was included in the Supporting Information.
General methods
Lipase activity was evaluated through the conversion of pNPP into p-nitrophenol. Formation of p-nitrophenol was monitored with an iMark™ Microplate Absorbance Reader (Bio-rad, USA) at 415 nm. The assay was performed in a 96-well, flat bottomed microplate (08-772-2C, Fisher Scientific, USA) with 200 µL reaction volume, at either 25°C or 37°C. The initial assay procedure used in this study was based on previously described procedures (Lewis and Liu Citation2012; Winkler and Stuckmann Citation1979). The basic assay reaction contained 1.0 mg/mL lipase and 200 µM pNPP and 50 mM NaP buffer (pH 8.0). The initial hydrolysis rate (v0) was determined as the slope of the linear section of the absorbance profile, recorded every 30 seconds for 5 minutes. The relative hydrolysis rate (%v) was the ratio of the test sample hydrolysis rate and the reference hydrolysis rate. To study the effect of one factor on the enzyme activity, the evaluated factor property was independently varied while other variables remained constant. The optimal condition was the one with the highest and most stable hydrolysis rate during the assay course. In different experiment set, the assay component volumes and concentrations varied to maintain the final concentrations.
Statistics
All experiments were performed in triplicates which were blank subtracted; the results were reported as means and standard deviations of measured values. Statistical analysis was performed via one-way analysis of variance (ANOVA), followed by Tukey’s HSD Post Hoc Test. Data were analysed by using IBM SPSS version 23.0 (IBM, Chicago, IL, USA). p < 0 .05 was used to indicate statistical significance.
Effect of emulsifier on turbidity of assay mixture and lipase activity
The effect of emulsifier on the turbidity of the assay mixture was first visually examined in the test tubes. Each test tube contained 10 mL of an emulsifier solution at evaluated concentration, 1.0 mg/mL lipase and 200 µM pNPP in 50 mM NaP buffer at pH 8.0. The tube without emulsifier was used as control. Turbidity was observed on a black background after 30 min at room temperature. The emulsifier concentrations at which the reaction mixtures were homogeneous were selected for further study to compare the hydrolysis rates. The hydrolysis rates were determined by performing lipase assays in a 96-well plate as described above. In which, buffer solution was included emulsifier at evaluated concentration.
Effect of incubation time and temperature on lipase activity
To examine the stability of lipase at 37°C, the study was performed by varying the incubation time (0, 5, and 10 min) of lipase and buffer solution at 37°C before initiating the hydrolysis reaction. For the comparison of the hydrolysis rates at 25°C and 37°C, identical assays were performed at these two temperatures. The hydrolysis rates were determined as described above.
Effect of buffer and pH on lipase activity
All examined buffers were prepared at the concentration of 50 mM and supplemented with 6.25 mM SDC. The hydrolysis rates of the assay with different buffer solutions were determined as described above.
Effect of organic co-solvent on lipase activity
First, we set to examine the effect of different co-solvents on hydrolysis rates with a fixed amount [10% (v/v)]. From the preliminary result, further analyses were conducted to determine the appropriate concentration range of selected solvents [0–50% (v/v)]. The experiments were carried out as described above with the appopriate volumes of the buffer and the solvent solutions to obtain the final co-solvent concentrations of 0, 5, 10, 20, 30, 40 and 50% (v/v).
Effect of additives on lipase activity
Inorganic salts: All examined inorganic salts were prepared in 50 mM NaP buffer (pH 8.0). The hydrolysis rates of the assays with the addition of inorganic salts were determined as described above.
Glycerol as storage additive: To examine the effect of glycerol as storage additive on the hydrolysis rate, buffer solution with glycerol was made as a mixture of 50 mM NaP buffer (pH 8.0) and glycerol at the concentrations of 0, 1, 5 and 10% (v/v). Obtained buffer solutions were used to prepare corresponding 100 mg/mL lipase stock solutions. Stock solutions were centrifuged (14,000 × g, 5 min, 4°C) to remove insolubles. The supernatants were aliquoted in polypropylene tubes and stored at –20°C until examined. Lipase activity or hydrolysis rate was measured after 1–3 weeks of storage.
Implementation of results for pancreatic lipase inhibitory assay
The hydrolysis rates of samples with different orlistat concentrations were determined as described above at 25°C. Hydrolysis rate values were converted into the inhibition percentage (I%) according to the formula I% = (vinhibitor – vblank)/vblank × 100%. IC50 was interpolated from the curve of I% and logarithm of inhibitor concentrations.
Results and discussion
Effect of emulsifier on turbidity of assay mixture and lipase activity
In lipase assays employing pNPP as substrate, insoluble long-chain fatty acid from the hydrolysis can cause a turbid solution (Gupta et al. Citation2002). Several solutions for this problem have been reported. For example, addition of CaCl2 results in precipitation of the formed fatty acids (Pohanka Citation2019; Plou et al. Citation1998). Alternatively, the addition of emulsifiers (e.g. 2% TX-100 (Gupta et al. Citation2002), 5 mM SDC (Lewis and Liu Citation2012) or 0.01% gum arabic (Gilham and Lehner Citation2005; Kordel et al. Citation1991)) prevents turbidity issues. While the use of CaCl2 results in an additional step to remove the calcium salts (Gupta et al. Citation2002), gum arabic has been found to reduce lipase activity (Mendes et al. Citation2012), TX-100 and SDC showed their potency. The effect of TX-100 or SDC on the hydrolysis rate, however, had not yet been evaluated.
We set out to probe the use of TX-100 and SDC in the lipase assay. First, the turbidity of the assay mixture upon addition of SDC (3–8 mM) (M3–M8) and TX-100 (0.1−2.0%) (T0.1–T2) was visually inspected in test tubes. Without emulsifiers, the assay mixture became cloudy after mixing (C). The addition of emulsifiers 5–8 mM SDC or 0.2–2.0% TX-100 was effective in keeping the assay mixture homogenous (Figure ).
Figure 1. Assay mixture with the addition of sodium deoxycholate (SDC) and Triton X-100 (TX-100).
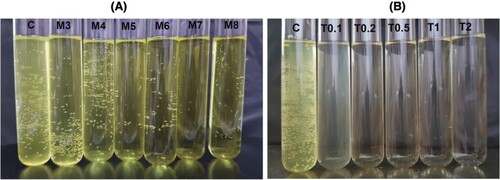
5 mM SDC (M5), 0.5% TX-100 (T0.5), and 2.0% TX-100 (T2) were chosen for further study to investigate their effect on lipase activity (Figure ). This revealed a striking effect of SDC on the rate of hydrolysis. In presence of 5 mM SDC (M5) (%v = 100%), the observed rate of hydrolysis was about one order of magnitude faster when compared with the control sample (C) (%v = 8.0%). In contrast to SDC, TX-100 (T0.5, T2) decreased the rate of hydrolysis significantly. These results suggest that, while TX-100 is detrimental for lipase activity, SDC as emulsifier not only helps to maintain the assay mixture homogeneous, but also improves the hydrolysis rate.
Figure 2. Effect of emulsifiers on the rate of hydrolysis.
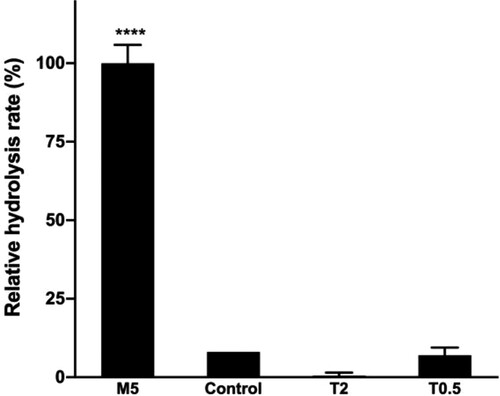
Effect of incubation time and temperature on lipase activity
Enzyme activity is temperature dependent. Some lipase inhibitory assays were performed at physiological temperature (37°C) while other used lower temperatures (Ninomiya et al. Citation2004; Mateos-Díaz et al. Citation2012). Some lipase inhibition assays included an incubation step of the enzyme and inhibitors at different temperature (25°C (Sergent et al. Citation2012), 30°C (Ninomiya et al. Citation2004) or 37°C (Sridhar et al. Citation2017; Kwon et al. Citation2003; Menteşe et al. Citation2016; Jeong et al. Citation2015)), in varying time (1–30 min). We set out to examine the stability of lipase at 37°C, which can well mimic the enzyme’s natural condition, in varying incubation time. Figure shows that the hydrolysis rate at 37°C dramatically decreased as the incubation time prolonged. After 5 min at 37°C, the remaining enzyme activity was only half of that before the incubation, indicating that lipase is not stable at 37°C. In screening of potential lipase inhibitors, incubation should either be avoided or carried out with sufficient controls to ensure the meaningful interpretation of the inhibitory effects.
Figure 3. Effect of incubation time at 37°C on the rate of hydrolysis.
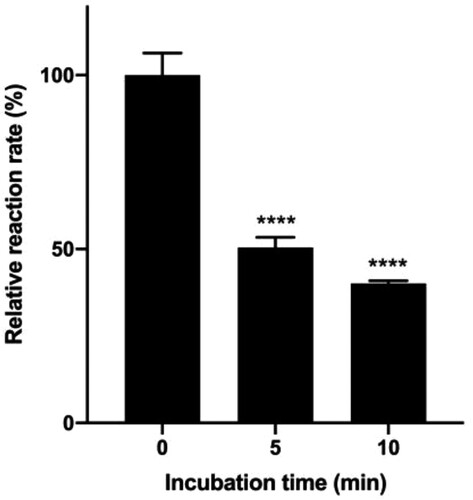
Higher temperatures (e.g. 37°C), can lead to enzyme denaturation, and proper thermostatting is required (Bisswanger Citation2014). Room temperature (25°C) will result in somewhat lower activity, however, it brings the convenience to perform the assay. To investigate the possibility to perform the assay at room temperature, we compared the rate of lipase-catalyzed hydrolysis at 25°C and 37°C. The hydrolysis rate at 25°C was 1.7-fold lower compared with that at 37°C (kobs values were 3.0 × 10−8 s−1 and 5.2 × 10−8 s−1, respectively). Nevertheless, considering the enzyme stability and the ease of the assay practice, researchers can alternatively choose 25°C for the assay.
Effect of buffer and pH on lipase activity
Pancreatic lipase is reported to perform best at pH 7.3−9.0 (Mendes et al. Citation2012), as shown for the hydrolysis of olive oil as substrate (Bagi et al. Citation1997; Bai et al. Citation2006; Zou et al. Citation2010). With triacetin and tributyrin, the optimum pH for the lipase was 7.3 (Vasudevan et al. Citation2004) and for ethyl acetate the optimum was reported to be pH 7.5 (Bautista et al. Citation1998). Nevertheless, to our knowledge, the optimal pH of lipase activity using a p-nitrophenyl ester substrate has not been reported. In this study, the pH dependent activity profile of lipase in different buffers was investigated (Figure ).
Figure 4. Relative hydrolysis rates in different buffers and pH.
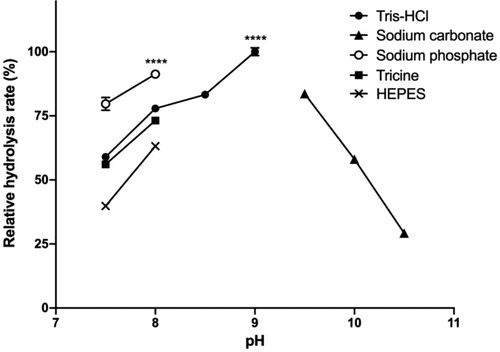
At pH 7, the assay mixture became turbid after 10–15 min upon the addition of SDC, which prohibited reliable activity measurements. At more alkaline pH, the assay mixture remained transparent throughout the performed assay, enabling the measurement of the hydrolysis rate at various pH values. Among the tested buffers, the highest lipase activities were measured at pH 8.0 in NaP buffer and pH 9.0 in Tris·HCl buffer. Activities were still > 50% in the range of pH 7.5–10. Based on these results, either NaP pH 8.0 or Tris·HCl pH 9.0 can be used for highest lipase activity. Yet, at pH 9.0, the alkaline medium itself can cause the spontaneous hydrolysis of p-nitrophenyl esters. Moreover, pH 9.0 is quite different from the physiological pH in which human pancreatic lipase has maximum activity in the small intestine (6.5–7.5) (Carrière et al. Citation2000). Therefore, we recommend the use of sodium phosphate buffer pH 8.0.
Effect of organic co-solvent for dissolving inhibitor on lipase activity
Lipase activity is frequently measured in an aqueous environment, which does not favor the dissolution of organic small molecules. Organic co-solvent, therefore, can be used to introduce the inhibitor into the assay mixture. In this study, various solvents including H2O, MeOH, EtOH, IPA, DMSO, AcCN, DMF, Ac2O, and DCM were evaluated. We observed that 10% (v/v) Ac2O, DMF and DCM generated turbidity upon adding into the assay mixture containing substrate, lipase, and SDC in 50 mM NaP buffer. On the other hand, the use of MeOH, EtOH, IPA, DMSO or AcCN as co-solvent did not induce any turbidity. The hydrolysis rates in the presence of 10% (v/v) MeOH, EtOH, IPA, DMSO and AcCN did not decrease lipase activity (Figure ). Next, we investigated the concentration range of each co-solvent that can be used. Hydrolysis rates were observed for MeOH, EtOH, IPA, AcCN, and DMSO at different concentrations [0–50% (v/v)] (Figure ). The addition of MeOH, EtOH, IPA, AcCN and DMSO up to 10% (v/v) slightly improves the hydrolysis rates. Above 10% (v/v) addition, different organic solvents behave with different manners. IPA and AcCN can be used up to 10% (v/v), EtOH up to 20% (v/v), DMSO and MeOH up to 30% (v/v). Above these limits, the hydrolysis rate dramatically declined.
Figure 5. Effect of organic co-solvent on hydrolysis rates.
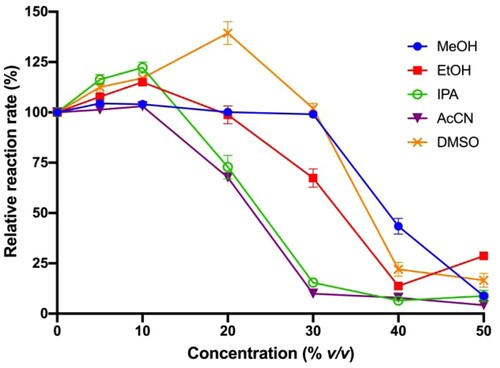
Among the evaluated co-solvents, DMSO stands out for several reasons. DMSO can dissolve most of the organic compounds. It can be added up to 30% (v/v) of assay volume without considerably changing the lipase activity. This is particularly significant for the initial stage of drug discovery. At this stage, many potential lead compounds are with low inhibitory activity. Their physicochemical properties have not been optimized. A high percentage of organic solvent facilitates the introduction of inhibitor at high concentration into the assay mixture. Additionally, DMSO with a high boiling point (189°C) does not evaporate easily, which can prevent the change of the reaction volume or lead to the re-precipitation of the inhibitors in the assay course. Lastly, it is not toxic and easy to handle. Other than DSMO, EtOH, MeOH are also good alternatives.
Effect of additives on lipase activity
NaCl and CaCl2
The influence of metal ions on lipase activity has been previously studied. Sodium and calcium ions were found to have positive effect on lipase activity. The effect, however, much depended on the substrate and the source of enzymes (Lowe Citation1999; Freitas et al. Citation2007; Li et al. Citation2011). The most tested substrates were tributyrin, triolein, or vegetable oil. In this study, the effect of sodium and calcium ion addition at different concentrations was evaluated with p-nitrophenyl palmitate as substrate.
In phosphate buffer, the hydrolysis rate with 5 mM NaCl is slightly higher than the rate without NaCl. As the NaCl concentration increased (10−500 mM), the hydrolysis rate decreased remarkably (Figure (a)). The positive effect of NaCl on lipase was also reported by Naka et al. This effect was also found to be much depended with the enzyme source (Naka and Nakamura Citation1994).
Figure 6. Effects of NaCl (a) or CaCl2 (b) on hydrolysis rates.
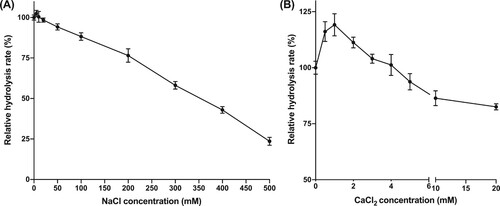
With CaCl2 a similar trend was observed (Figure (b)). The hydrolysis rate was observed to increase in the presence of low concentrations of CaCl2 (0.5−5 mM), reaching the highest activity at 1 mM. At higher concentrations (5−20 mM), the hydrolysis rate decreased. Notably, it was observed that when CaCl2 (1.0 mM) was added in sodium phosphate buffer (50 mM, pH 8.0), the solutions became turbid. As soon as pNPP was added, the assay mixture turned transparent again. The turbidity upon the addition of CaCl2 is due to the formation of insoluble calcium phosphate. Once the substrate was added, the hydrolysis occurred, forming free fatty acids. The combination of fatty acids and calcium ion reversed the formation of calcium phosphate, making the mixture clear again.
Glycerol
For long-storage purposes, enzyme stock solutions are usually stored at −20°C to −80°C, thawed, and diluted (if necessary) before used (Scopes Citation1993). Glycerol is known to have a positive effect on enzyme stability during freeze-thawing and is often used as additive for enzyme storage. In this study, glycerol was tested as additive at different amounts [1, 5, and 10% (v/v)] in the enzyme stock solution (100 mg/mL in NaP buffer pH 8.0). Enzyme mixtures were subsequently stored at −20°C. Lipase activity was measured after 1–3 weeks of storage (Figure ).
Figure 7. Relative hydrolysis rates of lipase solutions stored at –20°C with the addition of different amounts of glycerol as antifreeze.
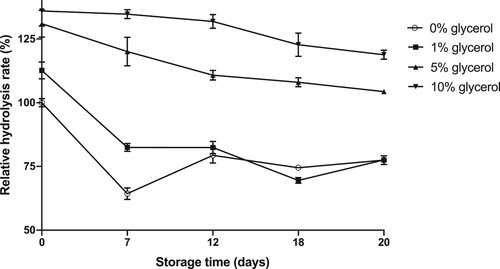
The hydrolysis rate of enzyme frozen and thawed without glycerol dropped significantly after one day of storage. Storage for several days revealed similar activity decays of about 25%. It can be partly explained by enzyme denaturation and/or aggregation, resulting in loss of activity upon freezing and thawing. Precipitation occurring after thawing not only requires an additional centrifuging step, but also leads to the reduction of enzyme concentration. With the highest glycerol concentration tested (10%, v/v), lipase could maintain activity up to 3 weeks of storage as stock solutions. Such additive prevents enzyme precipitation and, hence, is highly convenient for routine uses in the laboratories.
Implementation of results for pancreatic lipase inhibitory assay
In screening for novel pancreatic inhibitors, it would be advantageous to have a universal and standardized bioassay. The results from different studies therefore can be compared. Preferably, the assay could be implemented in laboratories with the basic equipment and at a low cost. The current pancreatic lipase inhibitory assay conditions experimentally varied depending on the inhibitor nature and laboratory conditions. In this study, we disclose the experimental conditions for in vitro pancreatic lipase assays using p-nitrophenyl palmitate substrate (Table ). With these results, researchers would have a preliminary procedure for lipase inhibitory assay to start without bothering which conditions would negatively affect the enzyme activity.
Table 1. Experimental conditions for in vitro lipase inhibitory assay.
As for the implementation of the results, we combined the optimal parameters and tested three condition sets: NaP buffer with 10% DMSO (NaP-10), NaP buffer with 20% DMSO (NaP-20), and Tris·HCl buffer with 20% DMSO (Tris·HCl-20). Proposed conditions were firstly evaluated to confirm that the combination of optimized factors does not much affect the reaction rates (Figure S1). All three condition sets were implemented to determine the IC50 values of orlistat. They all gave IC50 values with excellent goodness-of-fit (R2 > 0.99) (Table ).
Table 2. IC50 values determined by proposed condition sets.
Conclusion
In summary, this study evaluated experimental factors influencing the hydrolysis rate in lipase assay. These factors are emulsifiers, assay temperatures, buffers and pH, organic co-solvents, additives, and enzyme storage conditions. Sodium deoxycholate (5 mM) was found to maintain a homogenous assay solution allowing more accurate spectrophotometric measurement. Tris-HCl (pH 9.0) and sodium phosphate (pH 8.0) were suitable buffers. 5 mM NaCl and 1 mM CaCl2 can be added into the assay to have a better hydrolysis rate. Notably, this study revealed a range of organic co-solvents (DMSO, EtOH, MeOH, and IPA) up to 30% (v/v) concentration can be used to dissolve inhibitors before introducing into the aqueous assay environment. In terms of storage, a concentrated solution of porcine pancreatic lipase in buffer solution added 10% (v/v) glycerol can be stored at –20°C for up to 3 weeks. The enzyme stock solution can be used directly without thawing. Enzyme activity does not much degrade over the storage.
Disclosure statement
No potential conflict of interest was reported by the author(s).
Data availability statement
The data that support the findings of this study are available in figshare [https://figshare.com/] at http://doi.org/10.6084/m9.figshare.16864072.v1.
Additional information
Funding
References
- Abdul Rahman H, Saari N, Abas F, Ismail A, Mumtaz MW, Abdul Hamid A. 2017. Anti-obesity and antioxidant activities of selected medicinal plants and phytochemical profiling of bioactive compounds. Int J Food Prop. 20(11):2616–2629.
- Bagi K, Simon LM, Szajani B. 1997. Immobilization and characterization of porcine pancreas lipase. Enzyme Microb Technol. 20(7):531–535.
- Bai Y-X, Li Y-F, Yang Y, Yi L-X. 2006. Covalent immobilization of triacylglycerol lipase onto functionalized novel mesoporous silica supports. J Biotechnol. 125(4):574–582.
- Bautista FM, Bravo MC, Campelo JM, García A, Luna D, Marinas JM, Romero AA. 1998. Covalent immobilization of porcine pancreatic lipase on amorphous AlPO4 and other inorganic supports. J Chem Technol Biotechnol. 72(3):249–254.
- Bialecka-Florjanczyk E, Fabiszewska AU, Krzyczkowska J, Kurylowicz A. 2018. Synthetic and natural lipase inhibitors. Mini-Rev Med Chem. 18(8):672–683.
- Birari RB, Bhutani KK. 2007. Pancreatic lipase inhibitors from natural sources: unexplored potential. Drug Discov Today. 12(19-20):879–889.
- Bisswanger H. 2014. Enzyme assays. Perspect Sci. 1(1-6):41–55.
- Buchholz T, Melzig M. 2015. Polyphenolic compounds as pancreatic lipase inhibitors. Planta Med. 81(10):771–783.
- Carrière F, Renou C, Lopez V, De Caro J, Ferrato F, Lengsfeld H, De Caro A, Laugier R, Verger R. 2000. The specific activities of human digestive lipases measured from the in vivo and in vitro lipolysis of test meals. Gastroenterology. 119(4):949–960.
- Chauhan D, George G, Sridhar SNC, Bhatia R, Paul AT, Monga V. 2019. Design, synthesis, biological evaluation, and molecular modeling studies of rhodanine derivatives as pancreatic lipase inhibitors. Archiv der Pharmazie. 352(10):1900029.
- Daneschvar HL, Aronson MD, Smetana GW. 2016. FDA-approved anti-obesity drugs in the United States. Am J Med. 129(8):e871–e879.
- de la Garza AL, Milagro FI, Boque N, Campión J, Martínez JA. 2011. Natural inhibitors of pancreatic lipase as new players in obesity treatment. Planta Med. 77(8):773–785.
- Di L, Kerns EH. 2006. Biological assay challenges from compound solubility: strategies for bioassay optimization. Drug Discov Today. 11(9-10):446–451.
- Emimmal MES, Sankar V. 2019. Synthesis and pancreatic lipase inhibitory activity of phenacyl esters of n-aroyl amino acids. Curr Enzyme Inhib. 15(2):133–143.
- Freitas L, Bueno T, Perez VH, Santos JC, de Castro HF. 2007. Enzymatic hydrolysis of soybean oil using lipase from different sources to yield concentrated of polyunsaturated fatty acids. World J Microbiol Biotechnol. 23(12):1725–1731.
- Gilham D, Lehner R. 2005. Techniques to measure lipase and esterase activity in vitro. Methods. 36(2):139–147.
- Gupta N, Rathi P, Gupta R. 2002. Simplified para-nitrophenyl palmitate assay for lipases and esterases. Anal Biochem. 311(1):98–99.
- Jeong JY, Jo YH, Kim SB, Liu Q, Lee JW, Mo EJ, Lee KY, Hwang BY, Lee MK. 2015. Pancreatic lipase inhibitory constituents from morus alba leaves and optimization for extraction conditions. Bioorg Med Chem Lett. 25(11):2269–2274.
- Kordel M, Hofmann B, Schomburg D, Schmid RD. 1991. Extracellular lipase of Pseudomonas sp. strain ATCC 21808: purification, characterization, crystallization, and preliminary X-ray diffraction data. J Bacteriol. 173(15):4836–4841.
- Kwon C-S, Sohn HY, Kim SH, Kim JH, Son KH, Lee JS, Lim JK, Kim J-S. 2003. Anti-obesity effect of Dioscorea nipponica makino with lipase-inhibitory activity in rodents. Biosci Biotechnol Biochem. 67(7):1451–1456.
- Lerner H, Mehler L. 2019. FDA approval and surveillance process for anti-obesity drugs and devices. In: Morton JM, Brethauer SA, DeMaria EJ, Kahan S, Hutter MM, editors. Quality in obesity treatment. Cham: Springer International Publishing; p. 375–383.
- Lewis DR, Liu DJ. 2012. Direct measurement of lipase inhibition by orlistat using a dissolution linked in vitro assay. Clin Pharmacol Biopharma. 1. doi:10.4172/2167-065X.1000103.
- Li Y, Hu M, McClements DJ. 2011. Factors affecting lipase digestibility of emulsified lipids using an in vitro digestion model: proposal for a standardised pH-stat method. Food Chem. 126(2):498–505.
- Lowe ME. 1999. Assays for pancreatic triglyceride lipase and colipase. Methods Mol Biol. 109:59–70.
- Mateos-Díaz E, Rodríguez JA, de los Ángeles Camacho-Ruiz M, Mateos-Díaz JC. 2012. High-throughput screening method for lipases/esterases. In: Sandoval G, editor. Lipases and phospholipases: Methods and protocols. Totowa, NJ: Humana Press; p. 89–100.
- Mendes AA, Oliveira PC, de Castro HF. 2012. Properties and biotechnological applications of porcine pancreatic lipase. J Mol Catal B: Enzym. 78:119–134.
- Menteşe E, Karaali N, Akyüz G, Yılmaz F, Ülker S, Kahveci B. 2016. Synthesis and evaluation of α-glucosidase and pancreatic lipase inhibition by quinazolinone-coumarin hybrids. Chem Heterocycl Compd. 52(12):1017–1024.
- Naka Y, Nakamura T. 1994. Effects of colipase, bile salts, Na+, and Ca2+ on pancreatic lipase activity. Biosci Biotechnol Biochem. 58(11):2121–2122.
- Newman DJ, Cragg GM. 2012. Natural products as sources of new drugs over the 30 years from 1981 to 2010. J Nat Prod. 75(3):311–335.
- Ninomiya K, Matsuda H, Shimoda H, Nishida N, Kasajima N, Yoshino T, Morikawa T, Yoshikawa M. 2004. Carnosic acid, a new class of lipid absorption inhibitor from sage. Bioorg Med Chem Lett. 14(8):1943–1946.
- Obesity and Overweight [Internet]: World Health Organization (WHO) [posted June 9, 2021]; [cited Jun 15, 2021]. https://www.who.int/news-room/fact-sheets/detail/obesity-and-overweight.
- Pinsirodom P, Parkin KL. 2001. Lipase assays. Curr Protoc Food Anal Chem. 00(1):C3.1.1–C3.1.13.
- Plou FJ, Ferrer M, Nuero OM, Calvo MV, Alcalde M, Reyes F, Ballesteros A. 1998. Analysis of tween 80 as an esterase/lipase substrate for lipolytic activity assay. Biotechnol Tech. 12(3):183–186.
- Pohanka M. 2019. Biosensors and bioassays based on lipases, principles and applications, a review. Molecules. 24(3):616.
- Popkin BM, Du S, Green WD, Beck MA, Algaith T, Herbst CH, Alsukait RF, Alluhidan M, Alazemi N, Shekar M. 2020. Individuals with obesity and COVID-19: A global perspective on the epidemiology and biological relationships. Obes Rev. 21(11):e13128.
- Rajan L, Palaniswamy D, Mohankumar SK. 2020. Targeting obesity with plant-derived pancreatic lipase inhibitors: A comprehensive review. Pharmacol Res. 155:104681.
- Schrauwen P, Westerterp KR. 2000. The role of high-fat diets and physical activity in the regulation of body weight. Br J Nutr. 84(4):417–427.
- Scopes RK. 1993. Protein purification: principles and practice. 2nd ed. New York: Springer Science & Business Media. Chapter 9: Measurement of enzyme activity: 253-254.
- Sergent T, Vanderstraeten J, Winand J, Beguin P, Schneider Y-J. 2012. Phenolic compounds and plant extracts as potential natural anti-obesity substances. Food Chem. 135(1):68–73.
- Sridhar S, Bhurta D, Kantiwal D, George G, Monga V, Paul AT. 2017. Design, synthesis, biological evaluation and molecular modelling studies of novel diaryl substituted pyrazolyl thiazolidinediones as potent pancreatic lipase inhibitors. Bioorg Med Chem Lett. 27(16):3749–3754.
- Sridhar S, Ginson G, Reddy PV, Tantak MP, Kumar D, Paul AT. 2017. Synthesis, evaluation and molecular modelling studies of 2-(carbazol-3-yl)-2-oxoacetamide analogues as a new class of potential pancreatic lipase inhibitors. Bioorg Med Chem. 25(2):609–620.
- Sridhar SNC, George G, Verma A, Paul AT. 2019. Natural products-based pancreatic lipase inhibitors for obesity treatment. In: Akhtar MS, Swamy MK, Sinniah UR, editors. Natural bio-active compounds. Vol. 1: Production and applications. Singapore: Springer Singapore. p. 149–191.
- Vasudevan PT, López-Cortés N, Caswell H, Reyes-Duarte D, Plou FJ, Ballesteros A, Como K, Thomson T. 2004. A novel hydrophilic support, cofoam, for enzyme immobilization. Biotechnol Lett. 26(6):473–477.
- Winkler F, d'Arcy A, Hunziker W. 1990. Structure of human pancreatic lipase. Nature. 343:771–774.
- Winkler UK, Stuckmann M. 1979. Glycogen, hyaluronate, and some other polysaccharides greatly enhance the formation of exolipase by serratia marcescens. J Bacteriol. 138(3):663–670.
- Zou B, Hu Y, Yu D, Xia J, Tang S, Liu W, Huang H. 2010. Immobilization of porcine pancreatic lipase onto ionic liquid modified mesoporous silica sba-15. Biochem Eng J. 53(1):150–153.