Abstract
Metarhizium anisopliae (Hypocreales: Clavicipitaceae, M. anisopliae), as an invertebrate fungal pathogen, has played a significant role in the control of many agricultural pests and human disease vectors. M. anisopliae is typically used as a chemical in dry or liquid formulations of large numbers of aerial conidia. The conidia can directly infect arthropod pests by penetrating their cuticular layer. The availability of the complete genome of M. anisopliae and capable techniques for its transformation have brought several developments in its use as a pest controller. For prospects, more in-depth research to improve fermentation and formulation technologies is needed, while multistress-tolerant and engineering entomopathogenic strains are selected to promote the widespread acceptance and usefulness of M. anisopliae as a cost-effective fungal biopesticide. Here, we provide an overview of the pathogenesis and formulation strategies, as well as the host ranges and molecular approaches of increasing virulence and efficacy. Several studies of this fungus pathogen demonstrated that M. anisopliae can be an efficient biocontrol agent and has great potential for further research and development.
1. Background
Agricultural pests, such as ticks, termites, grasshoppers, and locusts, have caused great economic losses in many countries and regions in the world (Nourrisson et al. Citation2017; Savary et al. Citation2019). Furthermore, human disease vectors, such as mosquitos, are responsible for about one million deaths annually (World Health Organization Citation2017). To date, chemical insecticides are the most frequently and widely used to control agricultural pests and human disease vectors (Gupta et al. Citation2019). However, the quantity and frequency of chemical pesticide spraying have increased annually, which has resulted in increased resistance among pests (Damalas and Koutroubas Citation2018). The large-scale use of chemical pesticides also pollutes the environment, including soil and water, and poses great risks to humans and animals (Deng et al. Citation2019a). An eco-friendly alternative to chemical pesticides is biopesticide, which has the characteristics of a wide range of sources, great potential for improvement, low drug resistance, and lower nontarget toxicity, including lack of mammalian toxicity (Walia et al. Citation2017). Entomopathogenic fungi are biological agents that do not produce harmful effects on the environment; thus, they could be called ‘green pesticides’ (Sandhu et al. Citation2017; Lovett et al. Citation2019a). Their high efficiency of killing hosts and great biodiversity represent many possibilities for biological control agents, which can help reduce the use of chemicals and improve sustainable development (Damalas and Koutroubas Citation2018).
Metarhizium anisopliae (M. anisopliae) is a common insect pathogen, belonging to Fungi, Ascomycota, Sordariomycetes, Hypocreales, Clavicipitaceae, Metarhizium (Lovett et al. Citation2019a). Recently, M. anisopliae has been assigned to M. anisopliae. It is widely distributed in the soil of plant rhizosphere or arthropod carcass as a saprophyte (Rehner and Kepler Citation2017). M. anisopliae can infect many important crop pests but also human disease vectors (Lovett et al. Citation2019a). Moreover, this fungus is considered asexual because no teleomorph has been observed, and conidia are its form of infection (Lovett et al. Citation2019a). The infection process of M. anisopliae includes adhesion, spore germination, appressorium formation, host penetration, in vivo development, and host death (Gao et al. Citation2011). However, compared to chemical pesticides, M. anisopliae has a slower killing speed, which takes approximately five days under ideal conditions (Fang et al. Citation2007). Furthermore, pest control efficiency is easily affected by environmental conditions, such as humidity, temperature, and ultraviolet radiation (Gašić and Tanović Citation2013). With a better understanding of the processes and molecular mechanisms of host infection, the virulence of M. anisopliae can be enhanced by genetic engineering or the development of a suitable formulation, which could greatly improve the application and efficacy of M. anisopliae (Lovett et al. Citation2019b). This review mainly introduces the host infection pathogenesis of M. anisopliae and the application and development of M. anisopliae in different regions of the world.
2. Historical perspective
The Metarhizium genus was initially composed of four varieties, including M. anisopliae, M. guizhouense, M. pingshaense, and M. taii (Bischoff et al. Citation2009). With the improvement of DNA sequencing technology, more than 30 species of the genus Metarhizium have been isolated and identified (Fernández-Bravo et al. Citation2021). In Russia, Elie Metchnikoff studied an insect disease of wheat cockchafers, and he called the disease green muscardine and determined that the infecting agent was M. anisopliae (Vega et al. Citation2009). Subsequent studies showed that the cereal cockchafer and sugar beet weevil could be killed via direct infection of M. anisopliae conidia (Zhang et al. Citation2018). Since then, hundreds of fungi have been identified and developed as biocontrol agents. M. anisopliae has been extensively studied because of its environmental friendliness, safety for humans and mammals, narrow host range, and ease of mass production (Zhang et al. Citation2018). It has been reported that M. anisopliae can infect a variety of arachnid and insect hosts, including agricultural pests and human disease vectors (Gopal et al. Citation2006). As we learn more about the pathogens, their hosts and their environment, microbial control has also evolved.
3. Pathogenesis of M. anisopliae
M. anisopliae infects susceptible hosts by penetrating the cuticle directly (Freimoser et al. Citation2005). For ease of description, the infection process can be divided into: (1) conidia adherence to the host cuticle; (2) conidia germination and development; (3) appressorium formation; (4) cuticle penetration; (5) colonization of hemolymph; (6) extrusion and sporulation (Figure ) (Gao et al. Citation2011). Metarhizium spp. has species-specific genes in its infection pattern, such as the M. anisopliae adhesin-like protein 1 (Mad1) and high osmolarity glycerol (Hog) pathway Hog1 kinase genes during germination (San and Mun Citation2017). The major obstacle to insect infection is the complexity of the host's cuticle, which consists mainly of proteins and chitin (Charnley Citation2003). The secretion of protease is considered to be an important pathogenic factor for fungi attached to the cuticle layer (Butt et al. Citation2016).
Figure 1. Overview of the basic infection cycle depicted by M. anisopliae in invertebrates. The infection process can be divided into: (1) conidia adherence to the host cuticle; (2) conidia germination and development; (3) appressorium formation; (4) cuticle penetration; (5) colonization of hemolymph; (6) extrusion and sporulation.
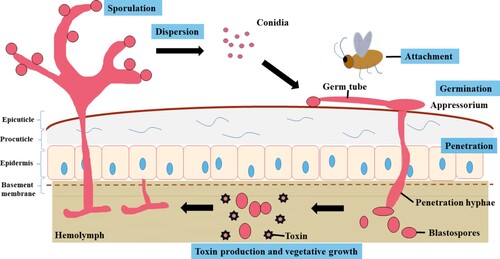
3.1 Conidia adhesion
In the first stage of infection, conidia deposit on the host cuticle and then adhere (San and Mun Citation2017; Schrank and Vainstein Citation2010). There are hydrophobic proteins in the outer layer of conidia, which promote the adhesion of the hydrophobic epidermis and conidia (Ment et al. Citation2010a; Greenfield et al. Citation2014). The cell walls of conidia of M. anisopliae also contain exogenous lectins, which combine with epidermal glycoprotein of the host to form a specific bond and play a role in identifying the host (Samson et al. Citation1988). Adhesion refers to hydrophobic interactions between lipid layers covering the cutin of arthropods and spore surface proteins (such as hydrophobic proteins). Lipids in the cuticular layer are the first barrier of the arthropod against pathogenic microorganisms and strengthen the importance of lipid enzymes in the early stages of infection (Schrank and Vainstein Citation2010). Moreover, lipid degradation is related to the prepenetration growth of M. anisopliae on the host epidermis (Schrank and Vainstein Citation2010). The degradation of the host lipid layer is of great significance for the identification of susceptible hosts and the production of the first nutrient molecules that support the germination of conidia (Pedrini et al. Citation2007). Furthermore, lipase can be identified on the surface of conidia (Schrank and Vainstein Citation2010). The conidia of less-virulent strains have poor adhesion to the host epidermis (Beys-Da-Silva et al. Citation2013). Adhesin protein Mad1 is of great significance in the attachment of swelling conidia to the host surface (Wang and St Leger Citation2007b). It was speculated that Mad1 could replace degraded hydrophobins upon changes in the conidia cell wall (Wang and St Leger Citation2007b).
3.2 Conidia germination and development
When conidia adhere to the host cuticle, the germination of conidia is initiated, and different lengths of germ tubes are produced (Ment et al. Citation2010a). Trehalase can be observed in the early stage of germination and it utilizes trehalose, which is common in host hemolymph. The activity of trehalase may provide glucose for energy production (San and Mun Citation2017). Spores swell and produce germ tubes that differentiate into appressorium after germination (Lovett and St Leger Citation2015). The growth of the germ tube is strongly oriented to the epidermis, and the germ tube adheres to the upper epidermis surface through mucus secreted by the hypha apex. Then, the germ tube gradually elongates to produce infection pegs (Hui et al. Citation2004). Amino acids, proteins, sugars, lipids, and other general nutrients can germinate the conidia of M. anisopliae. Two adhesin-encoding genes, Mad1 and Mad2, are respectively related to the pathogenesis of insects or the colonization of plant roots (Butt et al. Citation2013). Mad1 protein is expressed in the early stage of insect infection, while Mad2 protein is expressed only after fungal hyphae emerge and conidiate on the insect cadaver. Mad1 protein is also in charge of cell division and proper cytoskeleton organization, and the deletion of the Mad1 gene causes a delay in the germination of conidia (Barelli et al. Citation2011).
3.3 Appressorium formation
The germ tube differentiates into the appressorium before penetrating the epidermis (Senthil-Nathan Citation2015). Appressorium contains a large number of mitochondria, Golgi bodies, endoplasmic reticulum, and ribosomes. Through exuberant attachment metabolism, M. anisopliae can concentrate mechanical and dissolve energy into a small part of the host epidermis, thus promoting fungal invasion. The appressorium is covered with a thick mucilage layer, and the morphology of the appressorium varies greatly, ranging from elliptic to irregular, presenting a mulberry shape due to mucous accumulation (Hui et al. Citation2004). The number of appressoria and its structure are related to the thallus itself, host, and nutritional conditions. It has been demonstrated that the ornithine decarboxylase (ODC1) gene and Metarhizium perilipin-like protein (MPL1) gene are responsible for the formation of appressoria. The ODC1 gene is upregulated during the germination of conidia and the differentiation of the germ tube to form the appressorium (Wang and St. Leger Citation2007c; Pulido et al. Citation2011). The MPL1 gene regulates lipid homeostasis and appressorium differentiation (San and Mun Citation2017). MPL1 is mainly expressed when the fungus is engaged in lipid accumulation (Wang and St Leger Citation2007b). Fang et al. proved that specific inhibitors of protein kinase A could delay the expression of cuticle degrading enzymes and the formation of appressorium (Fang et al. Citation2009). In addition, it is reported that protein kinase A is involved in ergosterol biosynthesis and maintains turgor pressure in the appressorium. This expansion pressure produces the pressure required to penetrate the host cuticle (San and Mun Citation2017).
3.4 Cuticle penetration
In the process of penetration, physical pressure and cuticle degradation are two key factors. Besides, the efficiency of physical pressure mainly depends on appressorium formation, and lipid droplet formation provides the major physical pressure. Protein, chitin, and wax are important compositions in insect cuticles. Protease plays an important role in the degradation of the host epidermis, and its activity is the main factor determining the infection ability of M. anisopliae (Fang et al. Citation2009). M. anisopliae produces subtilisin-like enzyme (PR1), trypsin-like enzyme (PR2), acid protease (PR3), chymotrypsin, and carboxypeptidase with different catalytic properties, which digest the arthropods’ protein-rich plasma membrane (Moraes et al. Citation2003). The types and amount of protein produced by M. anisopliae are unique to each host, which can explain its ability to infect many different hosts (San and Mun Citation2017). Trypsins are only produced in specific hosts, such as cockroaches and beetles (Santi et al. Citation2010a). Chitinase activity is regulated by chitin degradation products, that is, induction inhibition mechanism. Pretreatment of insect epidermis with PR1, which is related to the pathogenesis of M. anisopliae, can enhance the subsequent chitinase activity (Lin et al. Citation2011). Different chitinase subtypes are produced in different hosts (Senthil-Nathan Citation2015). P450 monoxygenase is a key factor for cuticle penetration. The importance of monoterminal oxidation to M. anisopliae is due to the alkyl branch at the β position causing steric inhibition of terminal oxidizing enzymes. The single cytochrome P450 monoxygenase family 52 (MrCYP52) is required by M. anisopliae for rapid hydrolysis of alkanes and that this provides nutrition for germination and formation of infection structures (St. Leger et al. Citation1986). In addition, besides destroying host antifungal proteins that block pathogen invasion, release amino acids, and generate amines to regulate pH, epidermal degradation enzymes can also use host proteins as nutrients (Hui et al. Citation2004). The optimal pH values of PR1 and PR2 are approximately 8, which are generated only under alkaline conditions (Stleger et al. Citation1987). Therefore, the alkalinity of the infected epidermis is a physiological signal of toxicity factors (Hui et al. Citation2004). In the case of nutritional-deficiency, PR1 penetrates the cuticle by hydrolyzing the cuticular proteins (Carneiro et al. Citation2015). Furthermore, the production of PR1 was inhibited by exogenous carbon and nitrogen sources (Santi et al. Citation2010a). PR3 is an acidic protease with low isoelectric point. At present, its composition and action mechanism are still unclear. Chitinase is also the main determinant of pathogenicity (Hui et al. Citation2004). Carbon competition between pathogens and hosts may determine the rate and extent of infection (Seyoum et al. Citation2002). Lipase exists on the surface of conidia, which can release free fatty acids by enhancing the hydrophobic interaction between conidia and host, and enhance the adhesion between conidia and host through its lipolytic activity, so as to promote the adhesion between conidia and host (San and Mun Citation2017). Protease, lipase, and chitinase degrade the cuticle together, enabling effective penetration and utilization of nutrients in the hemocoel of the host for successful infection (Santi et al. Citation2010b). St Leger et al. concluded that the epidermal degradation enzymes of M. anisopliae are only synthesized at a pH that can play an effective role, regardless of the presence of epidermal substances in the culture medium (Leger et al. Citation1998). In addition, the pH of the surrounding area changes during M. anisopliae infection, which enables the production of extracellular enzymes and their activities. Environmental pH also regulates the expression of toxic genes (Hui et al. Citation2004).
3.5 Colonization of hemolymph
Once the fungus breaks through the cuticular layer and the lower epidermis, it starts to invade and proliferate in the hemolymph, which may be restricted to the hemolymph until the death of the host (Seyoum et al. Citation2002). In vivo and in the culture of infected insects, M. anisopliae produces a family of cyclic peptide toxins called destruxins (DTXs) (Hsiao and Ko Citation2001). DTXs of M. anisopliae are the first cyclic peptide insect-borne fungal toxins to be systematically studied. Trehalases are produced to convert trehalose from host hemolymph into glucose for energy production, which may represent an adaptation to the host environment (Santi et al. Citation2010b). In addition, to protect conidia from ultraviolet radiation and reactive oxygen species formed at high temperatures, catalase and peroxidases are found on the surface of conidia (San and Mun Citation2017). Fungi can use sugar as nutrition and consume trehalose, thus reducing the utilization rate of sugar as a host nutrient (Santi et al. Citation2010b). Moreover, Mad1 protein initiates gene expression involved in the cell cycle, enabling hyphae to rapidly proliferate and differentiate in the hemolymph of the host (Wang and St. Leger Citation2007c). These proteins target the cytoskeleton and regulate cell division during the cell cycle (San and Mun Citation2017).
3.6 Extrusion and sporulation
With the development of host colonization, the nutrients are exhausted and the fungus produces hyphae, which will appear and produce conidia on the surface of the dead host (Gabarty et al. Citation2014). M. anisopliae then forms a denser network of green spores on the cadavers of infected hosts, ending the infection (San and Mun Citation2017).
4. Toxins
M. anisopliae produces a large number of fungal secondary metabolites, including DTXs, which induce membrane depolarization by opening Ca2+channels, resulting in paralysis and death of the host insects (Hsiao and Ko Citation2001). DTXs are a class of insecticidal, phytotoxic, and anti-viral cyclic peptide insect-borne fungal toxins and are the most deeply studied toxins of the entomopathogenic fungi (Schrank and Vainstein Citation2010). These compounds are composed of an α-hydroxy acid and five amino acids to form a cyclic hexadepsipeptide (Gabarty et al. Citation2014). To date, 38 DTXs have been reported and the majority are produced by M. anisopliae (Schrank and Vainstein Citation2010). DTXs can be divided chemically into five basic groups labeled A to E (Pedras et al. Citation2002). Destruxins A, E, and B show significant insecticidal activity (Sree et al. Citation2008). Both destruxins B (DB) and desmethyl-DB have phytotoxicity to the plants of Brassica (Hu et al. Citation2006). Moreover, it has been reported that DB can suppress the expression of the hepatitis B viral surface antigen in human hepatoma Hep3B cells, while the chromosomes of Hep3B cells carry integrated viral genes, which suggests that DB may have a promising future as a specific anti-HBV drug (Chen et al. Citation1997). Furthermore, these toxins assume a significant role in weakening host immune defense by damaging the Malpighian tubules and the muscular system, leading to feeding and affecting excretion as well as mobility difficulties (Schrank and Vainstein Citation2010). Infected insects often seek out warmer places to raise their body temperature, thereby inhibiting the growth of the infected microbes (Elliot and Thomas Citation2002). DTXs can reduce host mobility to undermine behavior defense mechanisms (Schrank and Vainstein Citation2010).
Swainsonine, a sugar analogue from M. anisopliae isolate F-3622, was first reported by Hino et al. (Citation1985; Batta Citation2003; Roberts and Leger Citation2004). Swainsonine has great potential as a chemotherapeutic drug because its glycosylation changes are associated with the processes of some diseases (Sim and Perry Citation1997). In addition to its direct effects on oligosaccharide processing in cancer cells, it also has immunostimulating activity (Roberts and Leger Citation2004). Effects of swainsonine, such as enhancing lymphocyte proliferation and augmenting cytotoxicities of lymphokine-activated killer and natural killer cells, have been demonstrated (Sim and Perry Citation1997). In addition to its therapeutic potential, swainsonine is also widely used as a biochemical tool in studies of the nature and biological roles of N-linked oligosaccharides and alpha mannosidases (Sim and Perry Citation1997).
5. M. anisopliae as a Biological Control Agent
Mosquitoes transmit various pathogens, such as Plasmodium and dengue virus, causing illness and death in humans (Alkhaibari et al. Citation2016); World Health Organization Citation2020). Longevity, fecundity, and blood-feeding are the most important factors affecting the possibility of mosquitoes transmitting diseases (Scholte et al. Citation2006). It has been reported that M. anisopliae infection can reduce the survival time of mosquitoes, including Anopheles, Aedes, and Culex (Daoust and Roberts Citation1982; Riba et al. Citation1986a; Riba et al. Citation1986b; Scholte et al. Citation2003; Scholte et al. Citation2007; Lwetoijera et al. Citation2010; Paula et al. Citation2019; Choi et al. Citation2020; Shoukat et al. Citation2020; Vivekanandhan et al. Citation2020) (Figure ). Scholte et al. contaminated Anopheles gambiae (Diptera: Culicidae, An. gambiae) with the oil-formulated conidia of M. anisopliae, and they found that M. anisopliae infection reduced fecundity and blood-feeding of the adult female malaria vector An. gambiae (Scholte et al. Citation2006). Similarly, Scholte et al. and Shoukat et al. reported that the fecundity of the female dengue virus vector Aedes aegypti (Diptera: Culicidae, Ae. aegypti) and Aedes albopictus (Diptera: Culicidae, Ae. albopictus) was reduced by M. anisopliae infection (Scholte et al. Citation2006; Shoukat et al. Citation2020). They also found that when Ae. aegypti females were coinfected with M. anisopliae and dengue virus type 2 (DENV-2), the infection rates of DENV-2 in the heads and midguts were significantly reduced (Scholte et al. Citation2007; Garza-Hernández et al. Citation2013). Moreover, a transgenic approach can be used to improve the virulence of M. anisopliae for mosquitoes or interfere with the development of pathogens in the mosquito (Wassermann et al. Citation2016). In addition, ticks are considered to be the main vectors of animal diseases, second only to mosquitoes as human disease vectors. These arthropods are distributed all over the world, and diseases spread by human ticks range from bacteria to protozoa, viruses, and even fungi (Parola and Raoult Citation2001). It has been demonstrated that M. anisopliae can kill the larvae, nymphs, and adults of many genera and species of ticks (Table ) (Mwangi et al. Citation1995; Zhioua et al. Citation1997; Kirkland et al. Citation2004; Ment et al. Citation2010b; Ren et al. Citation2014; Beys-da-Silva et al. Citation2020; Molaei et al. Citation2020; Abuowarda et al. Citation2020). Therefore, M. anisopliae may have potential as a biocontrol agent for vector-borne disease control.
Figure 2. Mycotoxic effects of M. anisopliae. (A) M. anisopliae cultured on Potato Dextrose Agar Medium. At the initial stage, the colonies were white and hairy. In the sporulation stage, there were clumps of green conidia in the middle of the colony. (B) The cadavers of Aedes aegypti infected by M. anisopliae or not. (C) The cadavers of Anopheles stephensi infected by M. anisopliae or not.
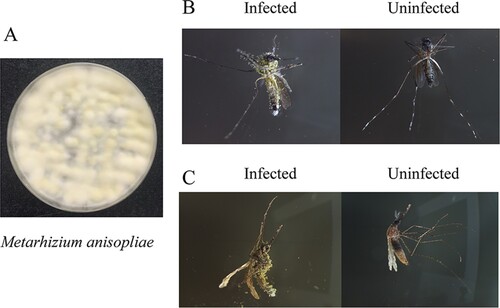
Table 1. Agricultural pests and human disease vectors sensitive to M. anisopliae.
Termites are important pests that cause severe damage to crops, living trees and wood-based materials (Rath Citation2000). It has been reported that M. anisopliae can be used to control many termites (Table ) (Hänel Citation1982; Zober Citation1995; Moino et al. Citation2002; Shimizu and Yamaji Citation2003; Yanagawa and Shimizu Citation2007; Dong et al. Citation2009; Pikkheng et al. Citation2009; Kin et al. Citation2017; Mitaka et al. Citation2017; Keppanan et al. Citation2018; Fu et al. Citation2020). The first report of termite fungal biological control agent is a single isolate C4-B, which is identified as M. anisopliae taxonomically (Wright et al. Citation2005). In addition, locusts represent probably the most striking of all insect pests and are abundant insects of deserts and dry grasslands (Scanlan et al. Citation2001). Traditionally, chemical pesticides have been used to control swarms and bands, but this is under strict scrutiny. M. anisopliae has been tested successfully against Schistocerca gregaria (Orthoptera: Acrididae), Chortoicetes terminifera (Orthoptera: Acrididae), Locusta migratoria (Orthoptera: Oedipodidae) and Oxya chinensis (Orthoptera: Acrididae) (Table ) (Zober Citation1995; Xia et al. Citation2000; Hunter et al. Citation2001; Peng et al. Citation2008; Abro et al. Citation2019; Liu et al. Citation2019; Abdellaoui et al. Citation2020). In Australia, field trials have shown that efficacy depends on the application rate and vegetation cover, while the rate of development of both Metarhizium and the locust is affected by temperature (Hunter et al. Citation2001). However, one challenge of using M. anisopliae is to overcome the behavioral fever of locusts, which can inactivate the conidia (Hunter et al. Citation2001). It has been reported that heat-tolerant genes can be transferred into M. anisopliae by genetic engineering to overcome this challenge (San and Mun Citation2017; Keppanan et al. Citation2017). Thus, M. anisopliae has desirable attributes for the development of a mycoinsecticide against pests.
In addition, M. anisopliae has been applied to control Spodoptera litura (Lepidoptera: Noctuidae), Carposina niponensis (Lepidoptera: Carposinadae), Galleria mellonella (Lepidoptera: Pyralidae), Prodenia litura (Lepidoptera: Noctuidae), Pantana phyllostachysae (Lepidoptera: Lymantriidae), Blattella germanica (Blattodea: Ectobiidae), Dendrolimus punctatus (Lepidoptera: Lasiocampidae), Triatoma infestans (Hemiptera: Reduviidae), Helicoverpa armigera (Lepidoptera: Noctuidae), Hyblaea puera (Lepidoptera: Hyblaeidae), Callosobruchus maculatus (Coleoptera: Chrysomelidae), Musca domestica (Diptera: Muscidae), Dysdercus peruvianus (Hemiptera: Pyrrhocoridae), Anastrepha luden (Diptera: Tephritidae), Chilo partellus (Lepidoptera: Crambidae), Panstrongylus megistus (Hemiptera: Reduvidae), Monochamus alternatus (Coleoptera: Cerambycidae), Curculio nucum (Coleoptera: Curculionidae), Frankliniella occidentalis (Thysanoptera: Thripidae), Encarsia Formosa (Hymenoptera: Aphelinidae), Trialeurodes vaporariorum (Hemiptera: Aleyrodidae), Plutella xylostella (Lepidoptera: Plutellidae), Zeugodacus cucurbitae (Diptera: Tephritidae), Sogatella furcifera (Hemiptera: Delphacidae), Nilaparvata lugens (Hemiptera: Delphacidae), Ostrinia furnacalis (Lepidoptera: Crambidae), Ostrinia nubilalis (Lepidoptera: Crambidae), Diatraea saccharalis (Lepidoptera: Crambidae), Triatoma sordida (Hemiptera: Triatominae), Cnaphalocrocis medinalis (Lepidoptera: Pyralidae), Spodoptera littoralis (Lepidoptera: Noctuidae), Alphitobius diaperinus (Coleoptera: Tenebrionidae), Pieris rapae (Lepidoptera: Paleidae), Plutella xylostella (Lepidoptera: Plutellidae), Dorysthenes granulosus (Coleoptera: Cerambycidae), Caryedon serratus (Coleoptera: Bruchidae), Peregrinus maidis (Hemiptera: Delphacidae), Rhyzopertha dominica (Coleoptera Bostrichidae), Tribolium castaneum (Coleoptera: Tenebrionidae), Cryptolestes ferrugineus (Coleoptera: Laemophloeidae), Liposcelis paeta (Psocoptera: Liposcelididae), Ephestia kuehniella (Lepidoptera: Pyralidae), Thaumatotibia leucotreta (Lepidoptera: Tortricidae), Spoladea recurvalis (Lepidoptera: Crambidae), Tuta absoluta (Lepidoptera: Gelechiidae), Anabrus simplex (Orthoptera: Tettigoniidae) (Table ) (Sree et al. Citation2008; Ment et al. Citation2010b; Keppanan et al. Citation2017; Yaginuma Citation1990; Rustiguel et al. Citation2018; De Oliveira et al. Citation2018; Luz et al. Citation1998; Hong et al. Citation2017; Kirubakaran et al. Citation2014; Chiuo and Hou Citation1993; Destéfano et al. Citation2004; Tefera and Pringle Citation2003; Zhang et al. Citation2002; Guogui Citation2003; Cherry et al. Citation2005; Cassiano et al. Citation2008; Lawo et al. Citation2008; Remadevi et al. Citation2010; Lopes and Alves Citation2011; Santi et al. Citation2011; Sharififard et al. Citation2011; Cheng et al. Citation2016; Oreste et al. Citation2016; Baker et al. Citation2018; Toledo-Hernández et al. Citation2018; El Husseini Citation2019; Onsongo et al. Citation2019; Tang et al. Citation2019; Chao et al. Citation2020; Fite et al. Citation2020; Kim et al. Citation2020; Li et al. Citation2021; Ozdemir et al. Citation2020; Rangel et al. Citation2020; Rice et al. Citation2020; Zafar et al. Citation2020; Bilal et al. Citation2012; Mohanty et al. Citation2008; Ojeda-Chi et al. Citation2010; Sbaraini et al. Citation2021; Ekesi et al. Citation2001; Toledo et al. Citation2010; Ashraf et al. Citation2017; Saeed et al. Citation2020; Athanassiou et al. Citation2017; Mkiga et al. Citation2020; Acheampong et al. Citation2020; Opisa et al. Citation2019; Contreras et al. Citation2014; Rangel et al. Citation2021). In summary, due to its safety and lack of arthropod resistance, M. anisopliae has good potential in the control of agricultural pests and vectors of human diseases (Wang and St Leger Citation2007a).
6. Molecular approaches to improve the virulence and efficacy of M. anisopliae
Poor efficacy has hindered the application of Metarhizium; hence, we urgently need to find methods to maximize environmental persistence and virulence of Metarhizium (Wang and St Leger Citation2007a). One approach to improve virulence is genetic manipulation. M. anisopliae provides a large list of genes that can be combined in a single pathogen vector because they use different proteins to adhere to the host, penetrate host cuticles, and overcome host defenses (Lovett and St Leger Citation2018). Many natural and synthetic genes have been inserted into the genome of M. anisopliae to enhance its virulence and efficacy against pests (Table ).
Table 2. The major approaches for modifying entomopathogens to enhance the virulence of M. anisopliae.
The scorpion excitatory sodium channel blocker, Androctonus australis Hector insect toxin (AaIT), is an insect-specific neurotoxin (Lovett and St Leger Citation2018). The recombinant expression of AaIT in fungi can increase their virulence against insect pests and disease vectors (Deng et al. Citation2019b). M Pava-Ripoll et al. demonstrated that recombinant M. anisopliae AaIT-Ma549 was dramatically more virulent than wild-type against Hypothenemus hampei larvae (Pava-Ripoll et al. Citation2008). Wang et al. also showed that the high-level expression of AaIT toxin in M. anisopliae increased its fungal toxicity to larval Manduca sexta caterpillars by 22-fold and Ae. aegypti adults by 9-fold (Table ) (Wang and St Leger Citation2007a).
Fang et al. engineered M. anisopliae to express the eight repeats of salivary gland and midgut peptide 1 ([SM1]8); a single-chain human antibody that agglutinates sporozoites; an antimicrobial toxin scorpine; or a fusion protein of [SM1]8 and scorpine. These recombinant fungi not only killed An. gambiae, but also reduced sporozoite counts of An. gambiae by 70% to 90%. M. anisopliaeexpressing scorpion and an [SM1]8: scorpion fusion protein reduced the number of sporozoites by 98%, indicating that M. anisopliae-mediated inhibition of Plasmodium development may be a powerful weapon for malaria control (Fang et al. Citation2011).
M. anisopliae exhibits an increase in catalase-peroxidase activity during its germination and growth (Kawasaki and Aguirre Citation2001). Morales Hernandez et al. found that catalase overexpression can reduce the germination time and increase the pathogenicity of M. anisopliae (Morales Hernandez et al. Citation2010). Thus, they isolated the genomic copy of M. anisopliae catalase-1 (cat1) gene and overexpressed it in the fungus. They found that increased cat1 expression was accompanied by increased H2O2 tolerance and germination rate, all of which enhanced the virulence against Plutella xylostella larvae (Morales Hernandez et al. Citation2010).
In addition, wild M. anisopliae isolates showed no pathogenicity to Spodoptera litura larvae (Lee et al. Citation2006). Zhang et al. genetically modified M. anisopliae by inserting the insect midgut-specific toxin Vip3Aal gene of Bacillus thuringiensis. The expression of this toxin allows M. anisopliae to infect S. litura larvae through the ingestion of conidia rather than through the cuticle penetration (Zhang et al. Citation2014).
7. M. anisopliae formulations
In addition to strain selection and genetic modification, formulations can also have a significant impact on improving the efficacy of biological pesticides. An ideal formulation is helpful to the handling and application of biopesticides and reduces the chances of nontarget organisms coming into contact with fungal spores.
Bukhari et al. devised formulations of M. anisopliae spores in aqueous, dry, synthetic oil (ShellSol T and Ondina oil 917) for the control of Anopheles larvae (Table ) (Bukhari et al. Citation2011). Among these formulations, synthetic oil is easily miscible and suitable for water surfaces and improves persistence (Abdul-Ghani et al. Citation2012). Their results have shown that the delivery system of fungal conidia suspended in ShellSol T solvent was significantly more virulent to An. gambiae and Anopheles stephensi (Diptera, Culicidae) larvae than that of unformulated conidia (Bukhari et al. Citation2011). However, dry and aqueous formulated M. anisopliae spores lost their pathogenicity quickly after being applied to the water surface (Bukhari et al. Citation2011).
Table 3. Selected examples of formulations using M. anisopliae in various habitats.
Inyang et al. took the third instar larvae of Phaedon cochleariae as the target host to study the effect of simulated rain on the persistence of oil formulations of M. anisopliae conidia when applied to oilseed rape foliage (Inyang et al. Citation2000). Their results suggested that sunflower oil formulations enhanced the infectivity of M. anisopliae for P. cochleariae in both laboratory and field experiments (Inyang et al. Citation2000). This viscous oil carrier could improve the adhesion of M. anisopliae conidia to plant surfaces and reduce displacement by rain (Inyang et al. Citation2000).
Oil carriers, such as sunflower, peanut, Ondina, soybean, canola and mineral oil, as well as cornstarch oil can transport the inoculum to parts of the insect's body, which is more conducive to germination than the application of conidia in Tween (Table ) (Batta Citation2003; Paula et al. Citation2019; Sharififard et al. Citation2011; Pereira and Roberts Citation1991; Dhar et al. Citation1996; Okumu et al. Citation2007; Brito et al. Citation2008; Leemon et al. Citation2008; Albernaz et al. Citation2009; Kaaya and Hedimbi Citation2012; Seye et al. Citation2012; Camargo et al. Citation2012; Rodrigues et al. Citation2019; Muniz et al. Citation2020). Oil carriers also seem to improve spore germination at low relative humidities and prolong spore viability, even at high temperatures (Inyang et al. Citation2000).
Michalaki et al. utilized diatomaceous earth (DE) in combination with an M. anisopliae formulation to treat wheat/flour. Their research is the first work on the combination of M. anisopliae and DE (Michalaki et al. Citation2006). Kavallieratos et al. demonstrated that the combined use of DE and M. anisopliae could be of practical significance to pest control, and this combination could be used against adults of three stored-grain beetle species (Table ). DEs can remove certain epidermis lipids from insects, allowing germinated M. anisopliae conidia to more easily infiltrate insect blood (Michalaki et al. Citation2006).
Alginates have been used to encapsulate fungal structures for the biocontrol of entomopathogens and weeds Pereira and Roberts (Citation1991), (Walker and Connick Citation1983) found that the alginate formulations are very effective in preventing fungi from inactivation due to high temperatures and artificial solar radiation. Thus, they used an Alginates formula to control adult Diabrotica undecimpunctata (Table ) (Pereira and Roberts Citation1991).
In addition to the above formulations, there are also other formulations that can improve the efficacy of M. anisopliae, such as aqueous, granular formulations consisting of ground chicken feed pellets with conidial powder and polymerized cellulose gel combined with surfactants (Kaaya and Hedimbi Citation2012; Walker and Connick Citation1983; Rohde et al. Citation2006; Reis et al. Citation2008; Rice et al. Citation2019).
8. Conclusions and future perspectives
M. anisopliae has been considered an important entomopathogenic fungus in the biocontrol of many pests and human disease vectors throughout the world since it was isolated and identified nearly 150 years ago. In this review, we mainly summarized the historical perspective, pathogenesis, toxins, M. anisopliae as a biological control, and molecular approaches to increase its virulence as well as the formulations of M. anisopliae. Generally, conidia, also known as asexual spores, are the main form of M. anisopliae that infects susceptible hosts. Once conidia touch the cuticular layer of a sensitive host, the infection begins with adhesion and germ tube formation. Then the expression of genes related to fungal infection structures, hydrolytic enzymes, and host defense mechanisms is triggered by both the insect pathogen and host. Normally, a sign of a successful fungal infection is the cleavage of the hosts’ epidermis. When the fungus arrives in the hemolymph through the growing hyphal, the host body will become colonized. M. anisopliae also produces numerous toxins, including DTXs and swainsonine. DTXs play an important role in weakening host immune defense, damaging the muscle system and Malpighian tubule, affecting host excretion, and resulting in feeding and mobility difficulties. The discoveries about the ecological roles displayed by M. anisopliae with multiple effects will help its eventual commercialization.
Although great progress has been made in the control of diseases and pests by using biopesticides, the development of fungal biological control agents still faces some problems. First, most biopesticide formulations, including spores, filaments, and other living preparations, cannot be easily combined with anti-corrosive ingredients, which affect the use and preservation of fungal pesticides. Second, the use of biopesticides is greatly affected by environmental conditions, thus leading to unstable efficacy. Third, compared with that of chemical pesticides, the price of biological pesticides is relatively high. Thus, researchers should make full use of the rich microbial resources in the world to screen out and select efficient strains and make continuous breakthroughs in technology to cultivate biocontrol strains with high virulence and productivity.
Disclosure statement
No potential conflict of interest was reported by the author(s).
Declaration of interest statement
No potential conflict of interest was reported by the author(s).
Author contributions statement
Conception and design: SQD; analysis and interpretation of the data: ZYP, STH, JTC, NL, YW and AN; writing – original draft preparation: STH and SQD; writing – review and editing: ZYP, AN and SQD. All authors have agreed to be accountable for all aspects of the work and the published version of the manuscript.
Data availability statement
Data sharing is not applicable to this article as no new data were created or analysed in this study.
Correction Statement
This article has been corrected with minor changes. These changes do not impact the academic content of the article.
Additional information
Funding
References
- Abdellaoui K, Miladi M, Mkhinini M, Boughattas I, Ben Hamouda A, Hajji-Hedfi L, et al. 2020. The aggregation pheromone phenylacetonitrile: Joint action with the entomopathogenic fungus Metarhizium anisopliae var. acridum and physiological and transcriptomic effects on Schistocerca gregaria nymphs. Pestic Biochem Phys. 167:104594. doi:10.1016/j.pestbp.2020.104594.
- Abdul-Ghani R, Al-Mekhlafi AM, Alabsi MS. 2012. Microbial control of malaria: biological warfare against the parasite and its vector. Acta Trop. 121(2):71–84. doi:10.1016/j.actatropica.2011.11.001.
- Abro NA, Wang G, Ullah H, Long GL, Hao K, Nong X, et al. 2019. Influence of Metarhizium anisopliae (IMI330189) and Mad1 protein on enzymatic activities and Toll-related genes of migratory locust. Environ Sci Pollut R. 26(17):17797–17808. doi:10.1007/s11356-019-05158-2.
- Abuowarda MM, Haleem M, Elsayed M, Farag H. 2020. Bio-Pesticide control of the brown dog tick (rhipicephalus sanguineus) in Egypt by using two entomopathogenic fungi (Beauveria bassiana and Metarhizium anisopliae). Int J Vet Sci. 9:175–181.
- Acheampong MA, Hill MP, Moore SD, Coombes CA. 2020. UV sensitivity of Beauveria bassiana and Metarhizium anisopliae isolates under investigation as potential biological control agents in South African citrus orchards. Fungal Biol. 124(5):304–310. doi:10.1016/j.funbio.2019.08.009.
- Albernaz DA, Tai MH, Luz C. 2009. Enhanced ovicidal activity of an oil formulation of the fungus Metarhizium anisopliae on the mosquito Aedes aegypti. Med Vet Entomol. 23(2):141–147. doi:10.1111/j.1365-2915.2008.00792.x.
- Alkhaibari AM, Carolino AT, Yavasoglu SI, Maffeis T, Mattoso TC, Bull JC, et al. 2016. Metarhizium brunneum blastospore pathogenesis in Aedes aegypti larvae: attack on several fronts accelerates mortality. PLoS Pathog. 12(7):e1005715. doi:10.1371/journal.ppat.1005715.
- Ashraf M, Farooq M, Shakeel M, Din N, Hussain S, Saeed N, Shakeel Q, Rajput NA. 2017. Influence of entomopathogenic fungus, Metarhizium anisopliae, alone and in combination with diatomaceous earth and thiamethoxam on mortality, progeny production, mycosis, and sporulation of the stored grain insect pests. Environ Sci Pollut Res. 24(36):28165–28174. doi:10.1007/s11356-017-0383-6.
- Athanassiou CG, Kavallieratos NG, Rumbos CI, Kontodimas DC. 2017. Influence of temperature and relative humidity on the insecticidal efficacy of Metarhizium anisopliae against Larvae of Ephestia kuehniella (Lepidoptera: Pyralidae) on Wheat. J Insect Sci. 17(1). doi:10.1093/jisesa/iew107.
- Baker DK, Rice SJ, Leemon DM, James PJ. 2018. Horizontal transmission of Metarhizium anisopliae (Hypocreales: Clavicipitacea) and the effects of infection on oviposition rate in laboratory populations of Musca domestica (Diptera: Muscidae). Pest Manag Sci. 74(4):987–991. doi:10.1002/ps.4799.
- Barelli L, Padilla-Guerrero IE, Bidochka MJ. 2011. Differential expression of insect and plant specific adhesin genes, Mad1 and Mad2, in Metarhizium robertsii. Fungal Biol. 115(11):1174–1185. doi:10.1016/j.funbio.2011.08.003.
- Batta YA. 2003. Production and testing of novel formulations of the entomopathogenic fungus Metarhizium anisopliae (Metschinkoff) Sorokin (Deuteromycotina: Hyphomycetes). Crop Prot. 22(2):415–422. doi:10.1016/S0261-2194(02)00200-4.
- Beys-Da-Silva WO, Lucélia S, Markus B, Guimarães JA, Augusto S, Vainstein MH. 2013. Susceptibility of Loxosceles sp. to the arthropod pathogenic fungus Metarhizium anisopliae: potential biocontrol of the brown spider. Trans R Soc Trop Med Hyg. 107:59–61. doi:10.1093/trstmh/trs006.
- Beys-da-Silva WO, Rosa RL, Berger M, Coutinho-Rodrigues CJB, Vainstein MH, Schrank A, et al. 2020. Updating the application of Metarhizium anisopliae to control cattle tick Rhipicephalus microplus (Acari: Ixodidae). Exp Parasitol. 208:107812. doi:10.1016/j.exppara.2019.107812.
- Bilal H, Hassan SA, Khan IA. 2012. Isolation and efficacy of entomopathogenic fungus (Metarhizium anisopliae) for the control of Aedes albopictus Skuse larvae: suspected dengue vector in Pakistan. Asian Pac J Trop Bio. 2(4):298–300. doi:10.1016/S2221-1691(12)60026-4.
- Bischoff JF, Rehner SA, Humber RA. 2009. A multilocus phylogeny of the Metarhizium anisopliae lineage. Mycologia. 101(4):512–530. doi:10.3852/07-202.
- Brito ES, de Paula AR, Vieira LP, Dolinski C, Samuels RI. 2008. Combining vegetable oil and sub-lethal concentrations of Imidacloprid with Beauveria bassiana and Metarhizium anisopliae against adult guava weevil Conotrachelus psidii (Coleoptera: Curculionidae). Biocontrol Sci Techn. 18:665–673. doi:10.1080/09583150802195965.
- Bukhari T, Middelman A, Koenraadt CJ, Takken W, Knols BG. 2010. Factors affecting fungus-induced larval mortality in Anopheles gambiae and Anopheles stephensi. Malaria J. 9(1):1–15. doi:10.1186/1475-2875-9-22.
- Bukhari T, Takken W, Koenraadt CJ. 2011. Development of Metarhizium anisopliae and Beauveria bassiana formulations for control of malaria mosquito larvae. Parasit Vectors. 4:23. doi:10.1186/1756-3305-4-23.
- Butt TM, Coates CJ, Dubovskiy I, Ratcliffe NA. 2016. Entomopathogenic fungi: new insights into host-pathogen interactions. Adv Genet. 94:307. doi:10.1016/bs.adgen.2016.01.006.
- Butt TM, Greenfield BPJ, Greig C, Maffeis TGG, Taylor JWD, Piasecka J, Dudley E, Abdulla A, Dubovskiy IM, Garrido-Jurado I, et al. 2013. Metarhizium anisopliae pathogenesis of mosquito larvae: a verdict of accidental death. Plos One. 8(12):e81686. doi:10.1371/journal.pone.0081686.
- Camargo MG, Golo PS, Angelo IC, Perinotto WM, Sá FA, Quinelato S, et al. 2012. Effect of oil-based formulations of acaripathogenic fungi to control Rhipicephalus microplus ticks under laboratory conditions. Vet Parasitol. 188(1-2):140–147. doi:10.1016/j.vetpar.2012.03.012.
- Carneiro LOMP, Vieira TP, Dini AF, Luiz dAW, Tinti DON. 2015. Differential expression of the pr1A gene in Metarhizium anisopliae and Metarhizium acridum across different culture conditions and during pathogenesis. Genet Mol Biol. 38(1):86–92. doi:10.1590/S1415-475738138120140236.
- Cassiano JA, Destéfano RHR, Baracho MS, Nääs IA, Salgado DD. 2008. Analysis of entomogenous fungus Metarhizium anisopliae to control Alphitobius diaperinus in poultry buildings. BJVRAS. 45(5):348–353.
- Chao Y, Wang M, Dai W, Dong F, Wang X, Zhang F. 2020. Synergism between Hydramethylnon and Metarhizium anisopliae and Their Influence on the Gut Microbiome of Blattella germanica (L.). Insects. 11(8):538. doi:10.3390/insects11080538.
- Charnley AK. 2003. Fungal pathogens of insects: Cuticle degrading enzymes and toxins. Adv Bot Res. 40:241–321. doi:10.1016/S0065-2296(05)40006-3.
- Chen HC, Chou CK, Sun CM, Yeh SF. 1997. Suppressive effects of destruxin B on hepatitis B virus surface antigen gene expression in human hepatoma cells. Antivir Res. 34(3):137–144. doi:10.1016/S0166-3542(97)01031-0.
- Cheng Y, Liu T, Zhao Y, Geng W, Chen L, Liu J. 2016. Evaluation of pathogenicity of the fungi Metarhizium anisopliae and Beauveria bassiana in hazelnut weevil (Curculio nucum L., Coleoptera, Curculionidae) larvae. Indian J Microbiol. 56(4):405–410. doi:10.1007/s12088-016-0614-4.
- Cherry AJ, Abalo P, Hell K. 2005. A laboratory assessment of the potential of different strains of the entomopathogenic fungi beauveria bassiana (balsamo) vuillemin and metarhizium anisopliae (metschnikoff) to control callosobruchus maculatus (F.) (coleoptera: bruchidae) in stored cowpea. J Stored Prod Res. 41(3):295–309. doi:10.1016/j.jspr.2004.04.002.
- Chiuo WC, Hou RF. 1993. Infection of the Asian corn borer, Ostrinia furnacalis Guenee (Lep., Pyralidae), with entomopathogens under screen house conditions. J Appl Entomol. 115:246–253. doi:10.1111/j.1439-0418.1993.tb00386.x.
- Choi CJ, Lee JY, Woo RM, Shin TY, Gwak WS, Woo SD. 2020. An effective entomopathogenic fungus Metarhizium anisopliae for the simultaneous control of Aedes albopictus and Culex pipiens mosquito adults. J Asia-Pac Entomol. 23(2):585–590. doi:10.1016/j.aspen.2020.04.007.
- Contreras J, Mendoza JE, Martínez-Aguirre MR, García-Vidal L, Izquierdo J, Bielza P. 2014. Efficacy of enthomopathogenic fungus Metarhizium anisopliae against Tuta absoluta (Lepidoptera: Gelechiidae). J Econ Entomol. 107(1):121–124. https://pubmed.ncbi.nlm.nih.gov/24665693.
- Damalas CA, Koutroubas SD. 2018. Current status and recent developments in biopesticide use. Agriculture. 8(1):1. doi:10.3390/agriculture8010013.
- Daoust RA, Roberts DW. 1982. Virulence of natural and insect-passaged strains of Metarhizium anisopliae to mosquito larvae. J Invertebr Pathol. 40(1):107–117. doi:10.1016/0022-2011(82)90042-8.
- Deng S, Huang Q, Wei H, Zhou L, Yao L, Li D, et al. 2019a. Beauveria bassiana infection reduces the vectorial capacity of Aedes albopictus for the Zika virus. J Pest Sci. 92(2):781–789. doi:10.1007/s10340-019-01081-0.
- Deng SQ, Zou WH, Li DL, Chen JT, Huang Q, Zhou LJ, et al. 2019b. Expression of Bacillus thuringiensis toxin Cyt2Ba in the entomopathogenic fungus Beauveria bassiana increases its virulence towards Aedes mosquitoes. PLoS Negl Trop Dis. 13(7):e0007590. doi:10.1371/journal.pntd.0007590. (in Chinese).
- De Oliveira DGP, Lopes RB, Rezende JM, Delalibera Jr I. 2018. Increased tolerance of Beauveria bassiana and Metarhizium anisopliae conidia to high temperature provided by oil-based formulations. J Invertebr Pathol. 151:151–157.
- Destéfano RHR, Destéfano SAL, Messias CL. 2004. Detection of Metarhizium anisopliae var. anisopliae within infected sugarcane borer Diatraea saccharalis (Lepidoptera, Pyralidae) using specific primers. Genet Mol Biol. 27:245–252. doi:10.1590/S1415-47572004000200020.
- Dhar R, Dawar H, Garg S, Basir SF, Talwar GP. 1996. Effect of volatiles from neem and other natural products on gonotrophic cycle and oviposition of anopheles stephensi and An. culicifacies (diptera: culicidae). J Med Entomol. 33(2):195–201. doi:10.1093/jmedent/33.2.195.
- Dong C, Zhang J, Huang H, Chen W, Hu Y. 2009. Pathogenicity of a new China variety of Metarhizium anisopliae (M. anisopliae var. Dcjhyium) to subterranean termite Odontotermes formosanus. Microbiol Res. 164(1):27–35. doi:10.1016/j.micres.2006.11.009.
- Ekesi S, Egwurube EA, Akpa AD, Onu I. 2001. Laboratory evaluation of the entomopathogenic fungus, Metarhizium anisopliae for the control of the groundnut bruchid, Caryedon serratus on groundnut. J Stored Prod Res. 37(4):313–321. https://pubmed.ncbi.nlm.nih.gov/11463393.
- El Husseini MM. 2019. Efficacy of the entomopathogenic fungus, Metarhizium anisopliae (Metsch.), against larvae of the cotton leafworm, Spodoptera littoralis (Boisd.) (Lepidoptera: Noctuidae), under laboratory conditions. Egypt J Biol Pest Co. 29:1–3. doi: 10.1186/s41938-019-0156-2.
- Elliot SL, Thomas BMB. 2002. Host-Pathogen interactions in a varying environment: temperature, behavioural fever and fitness. Proc Biol Sci. 269(1500):1599–1607. doi:10.1098/rspb.2002.2067.
- Fang W, Pava-Ripoll M, Wang S, Leger RS. 2009. Protein kinase A regulates production of virulence determinants by the entomopathogenic fungus, Metarhizium anisopliae. Fungal Genet Biol. 46(3):277–285. doi:10.1016/j.fgb.2008.12.001.
- Fang W, Pei Y, Bidochka MJ. 2007. A regulator of a G protein signalling (RGS) gene, cag8, from the insect-pathogenic fungus Metarhizium anisopliae is involved in conidiation, virulence and hydrophobin synthesis. Microbiology. 153(4):1017–1025. doi:10.1099/mic.0.2006/002105-0.
- Fang W, Vega-Rodríguez J, Ghosh AK, Jacobs-Lorena M, Kang A, Leger RJS. 2011. Development of transgenic fungi that kill human malaria parasites in mosquitoes. Science. 331(6020):1074–1077. doi:10.1126/science.1199115.
- Fernández-Bravo M, Gschwend F, Mayerhofer J, Hug A, Widmer F, Enkerli J. 2021. Land-Use type drives soil population structures of the entomopathogenic fungal genus metarhizium. Microorganisms. 9(7). doi:10.3390/microorganisms9071380.
- Fite T, Tefera T, Negeri M, Damte T, Sori W. 2020. Evaluation of Beauveria bassiana, Metarhizium anisopliae, and Bacillus thuringiensis for the management of Helicoverpa armigera (Hubner)(Lepidoptera: Noctuidae) under laboratory and field conditions. Biocontrol Sci Techn. 30:278–295. doi:10.1080/09583157.2019.1707481.
- Freimoser FM, Hu G, Leger RJS. 2005. Variation in gene expression patterns as the insect pathogen Metarhizium anisopliae adapts to different host cuticles or nutrient deprivation in vitro. Microbiology. 151(2):361. doi:10.1099/mic.0.27560-0.
- Fu R, Zhou L, Feng K, Lu X, Luo J, Tang F. 2020. Effects of Serratia marcescens (SM1) and its interaction with common biocontrol agents on the termite, Odontotermes formosanus (Shiraki). J Forestry Res. 1–5. doi:10.1007/s11676-020-01122-w.
- Gabarty A, Salem HM, Fouda MA, Abas AA, Ibrahim AA. 2014. Pathogencity induced by the entomopathogenic fungi beauveria bassiana and metarhizium anisopliae in agrotis ipsilon (hufn.). J Radiat Res Appl Sci. 7(1):95–100. doi:10.1016/j.jrras.2013.12.004.
- Gao Q, Jin K, Ying S-H, Zhang Y, Xiao G, Shang Y, et al. 2011. Genome sequencing and comparative transcriptomics of the model entomopathogenic fungi Metarhizium anisopliae and M. acridum. Plos Genet. 7(1):e1001264. doi:10.1371/journal.pgen.1001264.
- Garza-Hernández JA, Rodríguez-Pérez MA, Salazar MI, Russell TL, Adeleke MA, de Luna-Santillana EdJ, et al. 2013. Vectorial capacity of Aedes aegypti for dengue virus type 2 is reduced with co-infection of Metarhizium anisopliae. Plos Negl Trop Dis. 7(3):e2013–e2013. doi:10.1371/journal.pntd.0002013.
- Gašić S, Tanović B. 2013. Biopesticide formulations, possibility of application and future trends. Pestic Fitomed. 28(2):97–102. doi:10.2298/PIF1302097G.
- Gopal M, Gupta A, Thomas GV. 2006. Prospects of using Metarhizium anisopliae to check the breeding of insect pest, Oryctes rhinoceros L. in coconut leaf vermicomposting sites. Bioresource Technol. 97(15):1801–1806. doi:10.1016/j.biortech.2005.09.005.
- Greenfield BPJ, Lord AM, Dudley E, Butt TM. 2014. Conidia of the insect pathogenic fungus, Metarhizium anisopliae, fail to adhere to mosquito larval cuticle. Roy Soc Open Sci. 1(2):140193. doi:10.1098/rsos.140193.
- Guogui C. 2003. Screening of the superior strains of Beauveria bassiana of pantana phyllostachysae and practical application. Scientia Silvae Sinicae. 39(2):102–108. (in Chinese).
- Gupta RC, Miller Mukherjee IR, Malik JK, et al. 2019. Chapter 26 - insecticides. In: R.C. Guptas, editor. Biomarkers in toxicology (second edition). Pittsburgh: Academic Press; p. 455–475.
- Hänel H. 1982. The life cycle of the insect pathogenic fungus Metarhizium anisopliae in the termite Nasutitermes exitiosus. Mycopathologia. 80(3):137–145. doi:10.1007/BF00437576.
- Hino M, Nakayama O, Tsurumi Y, Adachi K, Shibata T, Terano H, Imanaka H. 1985. Studies of an immunomodulator, swainsonine. I. enhancement of immune response by swainsonine in vitro. J Antibiot. 38:926–935. doi:10.7164/antibiotics.38.926.
- Hong M, Peng G, Keyhani NO, Xia Y. 2017. Application of the entomogenous fungus, Metarhizium anisopliae, for leafroller (Cnaphalocrocis medinalis) control and its effect on rice phyllosphere microbial diversity. Appl Microbiol Biot. 101:6793–6807. doi:10.1007/s00253-017-8390-6.
- Hsiao YM, Ko JL. 2001. Determination of destruxins, cyclic peptide toxins, produced by different strains of Metarhizium anisopliae and their mutants induced by ethyl methane sulfonate and ultraviolet using HPLC method. Toxicon. 39:837–841. doi:10.1016/S0041-0101(00)00217-8.
- Hu QB, Ren SX, Wu JH, Chang JM, Musa PD. 2006. Investigation of destruxin A and B from 80 Metarhizium strains in China, and the optimization of cultural conditions for the strain MaQ10. Toxicon. 48(5):491–498. doi:10.1016/j.toxicon.2006.06.018.
- Hui Q, Zhen-Qiang WU, Shi-Zhong L. 2004. Metarhizium anisopliae and its mechanism for killing insects. Pesticides. 08:4. doi:10.16820/j.cnki.1006-0413.2004.08.002.
- Hunter DM, Milner RJ, Spurgin PA. 2001. Aerial treatment of the Australian plague locust, Chortoicetes terminifera (Orthoptera: Acrididae) with Metarhizium anisopliae (Deuteromycotina: Hyphomycetes). Bull Entomol Res. 91(2):93–99. doi:10.1079/BER200080.
- Inyang EN, McCartney HA, Oyejola B, Ibrahim L, Pye BJ, Archer SA, et al. 2000. Effect of formulation, application and rain on the persistence of the entomogenous fungus Metarhizium anisopliae on oilseed rape. Mycol Res. 104(6):653–661. doi:10.1017/S0953756200002641.
- Kaaya GP, Hedimbi M. 2012. The use of entomopathogenic fungi, Beauveria bassiana and Metarhizium anisopliae, as bio-pesticides for tick control. Int J Agr Sci. 2:245–250.
- Kawasaki L, Aguirre J. 2001. Multiple catalase genes are differentially regulated in Aspergillus nidulans. J Bacteriol. 183(4):1434–1440. doi: 10.1128/jb.183.4.1434-1440.2001.
- Keppanan R, Sivaperumal S, Aguila LCR, Hussain M, Bamisile BS, Dash CK, Wang L. 2018. Isolation and characterization of Metarhizium anisopliae TK29 and its mycoinsecticide effects against subterranean termite Coptotermes formosanus. Microb Pathog. 123:52–59. doi:10.1016/j.micpath.2018.06.040.
- Keppanan R, Sivaperumal S, Kanta DC, Akutse KS, Wang L. 2017. Molecular docking of protease from Metarhizium anisopliae and their toxic effect against model insect Galleria mellonella. Pestic Biochem Phys. 138:8–14. doi:10.1016/j.pestbp.2017.01.013.
- Kim JC, Lee SJ, Kim S, Lee MR, Baek S, Park SE, Kim JS. 2020. Management of pine wilt disease vectoring Monochamus alternatus adults using spray and soil application of Metarhizium anisopliae JEF isolates. J Asia-Pac Entomol. 23:224–233. doi:10.1016/j.aspen.2019.12.012.
- Kin PK, Moslim R, Azmi WA, Kamarudin N, Ali SRA. 2017. Genetic variation of entomopathogenic fungi, Metarhizium anisopliae and Isaria amoenerosea and their pathogenicity against subterranean termite, Coptotermes curvignathus. J Oil Palm Res. 29:35–46. doi:10.21894/jopr.2017.2901.04.
- Kirkland BH, Westwood GS, Keyhani NO. 2004. Pathogenicity of entomopathogenic fungi Beauveria bassiana and Metarhizium anisopliae to Ixodidae tick species Dermacentor variabilis, Rhipicephalus sanguineus, and Ixodes scapularis. J Med Entomol. 41(4):705–711. doi:10.1603/0022-2585-41.4.705.
- Kirubakaran SA, Sathish-Narayanan S, Revathi K, Chandrasekaran R, Senthil-Nathan S. 2014. Effect of oil-formulated Metarhizium anisopliae and Beauveria bassiana against the rice leaffolder Cnaphalocrocis medinalis Guenée (Lepidoptera: Pyralidae). Archiv Fr Pflanzenschutz. 47(8):977–992. doi:10.1080/03235408.2013.828388.
- Lawo NC, Mahon RJ, Milner RJ, Sarmah BK, Higgins TJV, Romeis J. 2008. Effectiveness of Bacillus thuringiensis-transgenic Chickpeas and the Entomopathogenic Fungus Metarhizium anisopliae in Controlling Helicoverpa armigera (Lepidoptera: Noctuidae). Appl Environ Microbiol. 74(14):4381–4389. doi:10.1128/AEM.00484-08.
- Lee MK, Miles P, Chen JS. 2006. Brush border membrane binding properties of Bacillus thuringiensis Vip3A toxin to Heliothis virescens and Helicoverpa zea midguts. Biochem Biophys Res Commun. 339(4):1043–1047. doi:10.1016/j.bbrc.2005.11.112.
- Leemon DM, Turner L, Jonsson NN. 2008. Pen studies on the control of cattle tick (Rhipicephalus (Boophilus) microplus) with Metarhizium anisopliae (Sorokin). Vet Parasitol. 156(3-4):248–260. doi:10.1016/j.vetpar.2008.06.007.
- Leger RJS, Joshi L, Roberts DW. 1998. Ambient pH is a major determinant in the expression of cuticle-degrading enzymes and hydrophobin by Metarhizium anisopliae. Appl Environ Microbiol. 64(2):709–713. doi: 10.1109/35.883500.
- Li J, Xie J, Zeng D, Xia Y, Peng G. 2021. Effective control of Frankliniella occidentalis by Metarhizium anisopliae CQMa421 under field conditions. J Pest Sci. 94(1):111–117. doi:10.1007/s10340-020-01223-9.
- Lin L, Fang W, Liao X, Wang F, Wei D, St Leger RJ. 2011. The MrCYP52 cytochrome P450 monoxygenase gene of Metarhizium robertsii is important for utilizing insect epicuticular hydrocarbons. PloS One. 6(12):e28984. doi:10.1371/journal.pone.0028984.
- Liu Y, Cheng Y, Li H, Nong X, Luke B. 2019. Virulence of Metarhizium anisopliae against 3rd instar nymphs of Locusta migratoria manilensis under different temperatures. Chin J Biol Cont. 35(4):642–647. (in Chinese).
- Lopes RB, Alves SB. 2011. Differential susceptibility of adults and nymphs of Blattella germanica (L.) (Blattodea: Blattellidae) to infection by Metarhizium anisopliae and assessment of delivery strategies. Neotrop Entomol. 40(3):368–374. doi: 10.1590/S1519-566X2011000300010.
- Lovett B, Bilgo E, Diabate A, St Leger R. 2019a. A review of progress toward field application of transgenic mosquitocidal entomopathogenic fungi. Pest Manag Sci. 75(9):2316–2324. doi:10.1002/ps.5385.
- Lovett B, Bilgo E, Millogo SA, Ouattarra AK, Sare I, Gnambani EJ, et al. 2019b. Transgenic Metarhizium rapidly kills mosquitoes in a malaria-endemic region of Burkina Faso. Science. 364(6443):894–897. doi: 10.1126/science.aaw8737.
- Lovett B, St Leger RJ. 2015. Stress is the rule rather than the exception for Metarhizium. Curr Genet. 61:253–261. doi:10.1007/s00294-014-0447-9.
- Lovett B, St Leger RJ. 2018. Genetically engineering better fungal biopesticides. Pest Manag Sci. 74(4):781–789. doi:10.1002/ps.4734.
- Luz C, Tigano MS, Silva IG, Cordeiro CM, Aljanabi SM. 1998. Selection of Beauveria bassiana and Metarhizium anisopliae isolates to control Triatoma infestans. Mem Inst Oswaldo Cruz. 93(6):839–846. doi: 10.1590/s0074-02761998000600026.
- Lwetoijera DW, Sumaye RD, Madumla EP, Kavishe DR, Mnyone LL, Russell TL, et al. 2010. An extra-domiciliary method of delivering entomopathogenic fungus, Metharizium anisopliae IP 46 for controlling adult populations of the malaria vector, Anopheles arabiensis. Parasite Vector. 3(1):18. doi:10.1186/1756-3305-3-18.
- Ment D, Gindin G, Rot A, Soroker V, Glazer I, Barel S, Samish M. 2010a. Novel technique for quantifying adhesion of Metarhizium anisopliae conidia to the tick cuticle. Appl Environ Microbiol. 76:3521–3528. doi: 10.1128/aem.02596-09.
- Ment D, Gindin G, Soroker V, Glazer I, Rot A, Samish M. 2010b. Metarhizium anisopliae conidial responses to lipids from tick cuticle and tick mammalian host surface. J Invertebr Pathol. 103(2):132–139. doi:10.1016/j.jip.2009.12.010.
- Michalaki MP, Athanassiou CG, Kavallieratos NG, Batta YA, Balotis GN. 2006. Effectiveness of Metarhizium anisopliae (Metschinkoff) Sorokin applied alone or in combination with diatomaceous earth against Tribolium confusum Du Val larvae: Influence of temperature, relative humidity and type of commodity. Crop Prot. 25(5):418–425. doi:10.1016/j.cropro.2005.07.003.
- Mitaka Y, Kobayashi K, Matsuura K. 2017. Caste-, sex-, and age-dependent expression of immune-related genes in a Japanese subterranean termite, Reticulitermes speratus. Plos One. 12(4):e0175417. doi:10.1371/journal.pone.0175417.
- Mkiga AM, Mohamed SA, du Plessis H, Khamis FM, Akutse KS, Ekesi S. 2020. Metarhizium anisopliae and Beauveria bassiana: pathogenicity, horizontal transmission, and their effects on reproductive potential of Thaumatotibia leucotreta (Lepidoptera: Tortricidae). J Econ Entomol. 113(2):660–668. doi:10.1093/jee/toz342.
- Mohanty SS, Raghavendra K, Mittal PK, Dash AP. 2008. Efficacy of culture filtrates of Metarhizium anisopliae against larvae of Anopheles stephensi and Culex quinquefasciatus. J Ind Microbiol Biot. 35(10):1199–1202. doi:10.1007/s10295-008-0434-6.
- Moino A, Alves SB, Lopes RB, Neves PMOJ, Pereira RM, Vieira SA. 2002. External development of the entomopathogenic fungi Beauveria bassiana and Metarhizium anisopliae in the subterranean termite Heterotermes tenuis. Sci Agr. 59(2):267–273.
- Molaei G, Little EA, Stafford IIIKC, Gaff H. 2020. A seven-legged tick: report of a morphological anomaly in Ixodes scapularis (Acari: Ixodidae) biting a human host from the Northeastern United States. Ticks Tick-Borne Dis. 11(1):101304. doi:10.1016/j.ttbdis.2019.101304.
- Moraes CKD, Schrank A, Vainstein MH. 2003. Regulation of extracellular chitinases and proteases in the entomopathogen and acaricide Metarhizium anisopliae. Curr Microbiol. 46(3):205–210. doi:10.1007/s00284-002-3863-x.
- Morales Hernandez CE, Padilla Guerrero IE, Gonzalez Hernandez GA, et al. 2010. Catalase overexpression reduces the germination time and increases the pathogenicity of the fungus Metarhizium anisopliae. Appl Microbiol Biotechnol. 87(3):1033–1044. doi:10.1007/s00253-010-2517-3.
- Muniz ER, Paixão FR, Barreto LP, Luz C, Arruda W, Angelo IC, Fernandes ÉK. 2020. Efficacy of Metarhizium anisopliae conidia in oil-in-water emulsion against the tick Rhipicephalus microplus under heat and dry conditions. Biocontrol. 65(3):339–351. doi:10.1007/s10526-020-10002-5.
- Mwangi EN, Hassanali A, Essuman S, Myandat E, Moreka L, Kimondo M. 1995. Repellent and acaricidal properties of Ocimum suave against Rhipicephalus appendiculatus ticks. Exp Appl Acarol. 19(1):11–18. doi: 10.1007/bf00051933.
- Nourrisson C, Dupont D, Lavergne RA, Dorin J, Forouzanfar F, Denis J, et al. 2017. Species of Metarhizium anisopliae complex implicated in human infections: retrospective sequencing study. Clin Microbiol Infec. 23(12):994–999. doi:10.1016/j.cmi.2017.05.001.
- Ojeda-Chi MM, Rodriguez-Vivas RI, Galindo-Velasco E, Lezama-Gutiérrrez R. 2010. Laboratory and field evaluation of Metarhizium anisopliae (Deuteromycotina: Hyphomycetes) for the control of Rhipicephalus microplus (Acari: Ixodidae) in the Mexican tropics. Vet Parasitol. 170:348–354. doi:10.1016/j.vetpar.2010.02.022.
- Okumu FO, Knols BG, Fillinger U. 2007. Larvicidal effects of a neem (Azadirachta indica) oil formulation on the malaria vector Anopheles gambiae. Malaria J. 6(1):1–8. doi:10.1186/1475-2875-6-63.
- Onsongo SK, Gichimu BM, Akutse KS, Dubois T, Mohamed SA. 2019. Performance of Three Isolates of Metarhizium anisopliae and Their Virulence against Zeugodacus cucurbitae under Different Temperature Regimes, with Global Extrapolation of Their Efficiency. Insects. 10(9):270. doi:10.3390/insects10090270.
- Opisa S, du Plessis H, Akutse KS, Fiaboe KKM, Ekesi S. 2019. Horizontal transmission of Metarhizium anisopliae between Spoladea recurvalis (Lepidoptera: Crambidae) adults and compatibility of the fungus with the attractant phenylacetaldehyde. Microb Pathogenesis. 131:197–204. doi:10.1016/j.micpath.2019.04.010.
- Oreste M, Bubici G, Poliseno M, Tarasco E. 2016. Effect of Beauveria bassiana and Metarhizium anisopliae on the Trialeurodes vaporariorum-Encarsia formosa system. J Pest Sci. 89(1):153–160. doi:10.1007/s10340-015-0660-4.
- Ozdemir IO, Tuncer C, Erper I, Kushiyev R. 2020. Efficacy of the entomopathogenic fungi; Beauveria bassiana and Metarhizium anisopliae against the cowpea weevil, Callosobruchus maculatus F. (Coleoptera: Chrysomelidae: Bruchinae). Egypt J Biol Pest Co. 30(1):1–5. doi: 10.1186/s41938-020-00219-y.
- Parola P, Raoult D. 2001. Ticks and tickborne bacterial diseases in humans: an emerging infectious threat. Clin Infect Dis. 32(6):897–928. doi:10.1086/319347.
- Paula AR, Ribeiro A, Lemos FJA, Silva CP, Samuels RI. 2019. Neem oil increases the persistence of the entomopathogenic fungus Metarhizium anisopliae for the control of Aedes aegypti (Diptera: Culicidae) larvae. Parasite Vector. 12. doi:10.1186/s13071-019-3415-x.
- Pava-Ripoll M, Posada FJ, Momen B, Wang C, et al. 2008. Increased pathogenicity against coffee berry borer, Hypothenemus hampei (Coleoptera: Curculionidae) by Metarhizium anisopliae expressing the scorpion toxin (AaIT) gene. J Invertebr Pathol. 99(2):220–226. doi:10.1016/j.jip.2008.05.004.
- Pedras MS, Irina Zaharia L, Ward DE. 2002. The destruxins: synthesis, biosynthesis, biotransformation, and biological activity. Phytochemistry. 59(6):579–596. doi: 10.1016/s0031-9422(02)00016-x.
- Pedrini N, Crespo R, Juárez MP. 2007. Biochemistry of insect epicuticle degradation by entomopathogenic fungi. Comp Biochem Physiol C Toxicol Pharmacol. 146(1-2):124–137. doi:10.1016/j.cbpc.2006.08.003.
- Peng G, Wang Z, Yin Y, Zeng D, Xia Y. 2008. Field trials of Metarhizium anisopliae var. acridum (Ascomycota: Hypocreales) against oriental migratory locusts, Locusta migratoria manilensis (Meyen) in Northern China. Crop Prot. 27(9):1244–1250. doi:10.1016/j.cropro.2008.03.007.
- Pereira RM, Roberts DW. 1991. Alginate and cornstarch mycelial formulations of entomopathogenic fungi, Beauveria bassiana and Metarhizium anisopliae. J Econ Entomol. 84:1657–1661.
- Pikkheng H, Bong CFJ, Jugah K, Rajan A. 2009. Evaluation of Metarhizium anisopliae var. anisopliae (Deuteromycotina: Hyphomycete) Isolates and their Effects on Subterranean Termite Coptotermes curvignathus (Isoptera: Rhinotermitidae). Am J Agric Biol Sci. 4(4):289–297. doi:10.3844/ajabssp.2009.289.297.
- Pulido JM, Guerrero IP, Martínez IdJMa, Valadez BC, Guzman JCT, Solis ES, et al. 2011. Isolation, characterization and expression analysis of the ornithine decarboxylase gene (ODC1) of the entomopathogenic fungus, Metarhizium anisopliae. Microbiol Res. 166(6):494–507. doi:10.1016/j.micres.2010.10.002.
- Rangel DEN, Bignayan HG, Golez HG, Keyser CA, Evans EW, Roberts DW. 2021. Virulence of the insect-pathogenic fungi Metarhizium spp. to Mormon crickets, Anabrus simplex (Orthoptera: Tettigoniidae). B Entomol Res. 1–8. doi:10.1017/S0007485321000663.
- Rangel DEN, Piedrabuena AE, Roitman I, Messias CL. 2020. Laboratory and field studies for the control of Chagas disease vectors using the fungus Metarhizium anisopliae. Arch Insect Biochem. 105(4):e21745. doi:10.1002/arch.21745.
- Rath AC. 2000. The use of entomopathogenic fungi for control of termites. Biocontrol Sci Techn. 10(5):563–581. doi:10.1080/095831500750016370.
- Rehner SA, Kepler RM. 2017. Species limits, phylogeography and reproductive mode in the Metarhizium anisopliae complex. J Invertebr Pathol. 148:60–66. doi:10.1016/j.jip.2017.05.008.
- Reis RC, Fernandes EK, Bittencourt VR. 2008. Fungal formulations to control Rhipicephalus sanguineus engorged females. Ann Ny Acad Sci. 1149(1):239–241. doi:10.1196/annals.1428.030.
- Remadevi OK, Sasidharan TO, Balachander M, Bai NS. 2010. Metarhizium based mycoinsecticides for forest pest management. J Biopestic. 3(2):470–473.
- Ren Q, Sun M, Guan G, Liu Z, Chen Z, Liu A, et al. 2014. Susceptibility of the tick Haemaphysalis qinghaiensis to isolates of the fungus Metarhizium anisopliae in China. Exp Appl Acarol. 64(2):253–258. doi:10.1007/s10493-014-9790-2.
- Riba G, Bouvier-Fourcade I, Caudal A. 1986a. Isoenzymes polymorphism in Metarhizium anisopliae (Deuteromycotina: Hyphomycetes) entomogenous fungi. Mycopathologia. 96(3):161–169. doi:10.1007/BF00437383.
- Riba G, Keita A, Soares GG, Ferron P. 1986b. Comparative studies of Metarhizium anisopliae and Tolypocladium cylindrosporum as pathogens of mosquito larvae. J Am Mosq Control Assoc. 2(4):469–473. doi:10.2307/3495228.
- Rice SJ, Baker DK, Leemon DM. 2019. Development of mycoinsecticide formulations with Beauveria bassiana and Metarhizium anisopliae for the control of lesser mealworm, Alphitobius diaperinus, in chicken broiler houses. Biocontrol. 64(5):489–500. doi:10.1007/s10526-019-09951-3.
- Rice SJ, Baker DK, Mayer DG, Leemon DM. 2020. Mycoinsecticide formulations of Beauveria bassiana and Metarhizium anisopliae reduce populations of lesser mealworm, Alphitobius diaperinus, in chicken-broiler houses. Bioll Control. 144:104234. doi:10.1016/j.biocontrol.2020.104234.
- Roberts DW, Leger RJS. 2004. Metarhizium spp., cosmopolitan insect-pathogenic fungi: mycological aspects. Adv Appl Microbiol. 54:1–70.
- Rodrigues J, Borges PR, Fernandes ÉKK, Luz C. 2019. Activity of additives and their effect in formulations of metarhizium anisopliae s.l. IP 46 against aedes aegypti adults and on post mortem conidiogenesis. Acta trop. 193:192–198. doi:10.1016/j.actatropica.2019.03.002.
- Rohde C, Alves LF, Neves PM, Alves SB, Da Silva ER, De Almeida JE. 2006. Selection of Beauveria bassiana (Bals.) Vuill. and Metarhizium anisopliae (Metsch.) Sorok. isolates against Alphitobius diaperinus (Panzer)(Coleoptera: Tenebrionidae). Neotrop Entomol. 35(2):231–240. doi: 10.1590/s1519-566(2006000200012.
- Rustiguel CB, Fernández-Bravo M, GuimarãEs LHS, Quesada-Moraga E. 2018. Different strategies to kill the host presented by Metarhizium anisopliae and Beauveria bassiana. Can J Microbiol. 64(3):191–200. doi:10.1139/cjm-2017-0517.
- Saeed N, Wakil W, Farooq M, Shakeel M, Arain MS, Shakeel Q. 2020. Evaluating the combination of Metarhizium anisopliae and an enhanced form of diatomaceous earth (Grain-Guard) for the environmentally friendly control of stored grain pests. Environ Monit Assess. 192(4):210. doi:10.1007/s10661-020-8189-2.
- Samson RA, Evans HC, Latgé J-P. 1988. Fungal pathogenesis. In: R.A. Samson, H.C. Evans, J.-P. Latgé, editor. Atlas of entomopathogenic fungi. Berlin, Heidelberg: Springer Berlin Heidelberg; p. 128–139.
- San AKM, Mun HS. 2017. Mode of infection of metarhizium spp. fungus and their potential as biological control agents. J Fungi. 3(2):30. doi:10.3390/jof3020030.
- Sandhu SS, Shukla H, Aharwal RP, Kumar S, Shukla S. 2017. Efficacy of entomopathogenic fungi as green pesticides: current and future prospects. In: D.G. Panpatte, Y.K. Jhala, R.V. Vyas, H.N. Shelat, editor. Microorganisms for green revolution: volume 1: microbes for sustainable crop production. Singapore: Springer Singapore; p. 327–349.
- Santi L, Beys da Silva WO, Berger M, Guimarães JA, Schrank A, Vainstein MH. 2010a. Conidial surface proteins of Metarhizium anisopliae: source of activities related with toxic effects, host penetration and pathogenesis. Toxicon. 55(4):874–880. doi:10.1016/j.toxicon.2009.12.012.
- Santi L, e Silva LAD, da Silva WOB, Corrêa APF, Rangel DEN, Carlini CR, Vainstein MH. 2011. Virulence of the entomopathogenic fungus Metarhizium anisopliae using soybean oil formulation for control of the cotton stainer bug, Dysdercus peruvianus. World J Microb Biot. 27(10):2297–2303. doi:10.1007/s11274-011-0695-5.
- Santi L, Silva WOB, Pinto ANFM, Schrank A, Vainstein MH. 2010b. Metarhizium anisopliae host-pathogen interaction: differential immunoproteomics reveals proteins involved in the infection process of arthropods. Fungal Biol. 114(4):312–319. doi:10.1016/j.funbio.2010.01.006.
- Savary S, Willocquet L, Pethybridge SJ, Esker P, McRoberts N, Nelson A. 2019. The global burden of pathogens and pests on major food crops. Nat Ecol Evol. 3(3):430–439.
- Sbaraini N, Hu J, Roux I, Phan CS, Motta H, Rezaee H, Schrank A, Chooi YH, Staats CC. 2021. Polyketides produced by the entomopathogenic fungus Metarhizium anisopliae induce Candida albicans growth. Fungal Genet Biol. 152:103568. doi:10.1016/j.fgb.2021.103568.
- Scanlan JC, Grant WE, Hunter DM, Milner RJ. 2001. Habitat and environmental factors influencing the control of migratory locusts (Locusta migratoria) with an entomopathogenic fungus (Metarhizium anisopliae). Ecol Modell. 136(2-3):223–236. doi:10.1016/S0304-3800(00)00424-5.
- Scholte EJ, Knols BG, Takken W. 2006. Infection of the malaria mosquito Anopheles gambiae with the entomopathogenic fungus Metarhizium anisopliae reduces blood feeding and fecundity. J Invertebr Pathol. 91(1):43–49. doi:10.1016/j.jip.2005.10.006.
- Scholte EJ, Njiru BN, Smallegange RC, Takken W, Knols BG. 2003. Infection of malaria (Anopheles gambiae s.s.) and filariasis (Culex quinquefasciatus) vectors with the entomopathogenic fungus Metarhizium anisopliae. Malar J. 2:29. doi:10.1186/1475-2875-2-29.
- Scholte EJ, Takken W, Knols BG. 2007. Infection of adult Aedes aegypti and Ae. albopictus mosquitoes with the entomopathogenic fungus Metarhizium anisopliae. Acta Trop. 102(3):151–158. doi:10.1016/j.actatropica.2007.04.011.
- Schrank A, Vainstein MH. 2010. Metarhizium anisopliae enzymes and toxins. Toxicon. 56(7):1267–1274. doi:10.1016/j.toxicon.2010.03.008.
- Senthil-Nathan S. 2015. A review of biopesticides and their mode of action against insect pests. In: P. Thangavel, G. Sridevi, editor. Environmental sustainability: role of green technologies. New Delhi: Springer India; p. 49–63.
- Seye F, Ndiaye M, Faye O, Afoutou JM. 2012. Evaluation of entomopathogenic fungus Metarhizium anisopliae formulated with suneem (neem oil) against Anopheles gambiae sl and Culex quinquefasciatus adults. Malaria Chemotherapy Cont Elim. 1(1-6).
- Seyoum E, Bateman RP, Charnley AK. 2002. The effect of Metarhizium anisopliae var acridum on haemolymph energy reserves and flight capability in the desert locust, Schistocerca gregaria. J Appl Entomol. 126(2-3):119–124. doi:10.1046/j.1439-0418.2002.00609.x.
- Sharififard M, Mossadegh M, Vazirianzadeh B, Zarei-Mahmoudabadi A. 2011. Interactions between Entomopathogenic Fungus, Metarhizium anisopliae and Sublethal Doses of Spinosad for Control of House Fly, Musca domestica. Iran J Arthropod Borne Dis. 5(1):28–36. doi:10.1042/BSE0510193.
- Shimizu S, Yamaji M. 2003. Effect of density of the termite, Reticulitermes speratus Kolbe (Isoptera: Rhinotermitidae), on the susceptibilities to Metarhizium anisopliae. Appl Entomol Zool. 38(1):125–130. doi:10.1303/aez.2003.125.
- Shoukat RF, Hassan B, Shakeel M, Zafar J, Li S, Freed S, Xu X, Jin F. 2020. Pathogenicity and transgenerational effects of Metarhizium anisopliae on the demographic parameters of Aedes albopictus (Culicidae: Diptera).”. J Med Entomol. 57(3):677–685.
- Sim KL, Perry D. 1997. Analysis of swainsonine and its early metabolic precursors in cultures of Metarhizium anisopliae. Glycoconjugate J. 14(5):661–668. doi:10.1023/A:1018505130422.
- Sree KS, Padmaja V, Murthy YL. 2008. Insecticidal activity of destruxin, a mycotoxin fromMetarhizium anisopliae (hypocreales), againstSpodoptera litura (lepidoptera: noctuidae) larval stages. Pest Manag Sci. doi:10.1002/ps.1480.
- St. Leger RJ, Charnley AK, Cooper RM. 1986. Cuticle-degrading enzymes of entomopathogenic fungi: synthesis in culture on cuticle. J Invertebr Pathol. 48(1):85–95. doi:10.1016/0022-2011(86)90146-1.
- Stleger RJ, Charnley AK, Cooper RM. 1987. Characterization of cuticle-degrading proteases produced by the entomopathogen Metarhizium anisopliae. Arch Biochem Biophys. 253(1):221–232. doi:10.1016/0003-9861(87)90655-2.
- Tang J, Liu X, Ding Y, Jiang W, Xie J. 2019. Evaluation of Metarhizium anisopliae for rice planthopper control and its synergy with selected insecticides. Crop Prot. 121:132–138. doi:10.1016/j.cropro.2019.04.002.
- Tefera T, Pringle KL. 2003. Food consumption by Chilo partellus (Lepidoptera: Pyralidae) larvae infected with Beauveria bassiana and Metarhizium anisopliae and effects of feeding natural versus artificial diets on mortality and mycosis. J Invertebr Pathol. 84(3):220–225. doi:10.1016/j.jip.2003.11.001.
- Toledo AV, de Remes Lenicov AMM, López Lastra CC. 2010. Histopathology caused by the entomopathogenic fungi, Beauveria bassiana and Metarhizium anisopliae, in the adult planthopper, Peregrinus maidis, a maize virus vector. J Insect Sci. 10:35. doi:10.1673/031.010.3501.
- Toledo-Hernández RA, Toledo J, Sánchez D. 2018. Effect of Metarhizium anisopliae (Hypocreales: Clavicipitaceae) on food consumption and mortality in the Mexican fruit fly, Anastrepha ludens (Diptera: Tephritidae). Int J Trop Insect Sci. 38(3):254–260.
- Vega FE, Goettel MS, Blackwell M, Chandler D, Jackson MA, Keller S, et al. 2009. Fungal entomopathogens: new insights on their ecology. Fungal Ecol. 2(4):149–159. doi:10.1016/j.funeco.2009.05.001.
- Vivekanandhan P, Swathy K, Kalaimurugan D, Ramachandran M, Kweka EJ. 2020. Larvicidal toxicity of Metarhizium anisopliae metabolites against three mosquito species and non-targeting organisms. PLOS ONE. 15(5):e0232172. doi:10.1371/journal.pone.0232172.
- Walia S, Saha S, Tripathi V, Sharma KK. 2017. Phytochemical biopesticides: some recent developments. Phytochem Rev. 16(5):989–1007. doi:10.1007/s11101-017-9512-6.
- Walker HL, Connick WJ. 1983. Sodium alginate for production and formulation of Mycoherbicides. Weed Sci. 31:333–338.
- Wang C, St Leger RJ. 2007a. A scorpion neurotoxin increases the potency of a fungal insecticide. Nat Biotechnol. 25(12):1455–1456. doi:10.1038/nbt1357.
- Wang C, St Leger RJ. 2007b. The MAD1 adhesin of Metarhizium anisopliae links adhesion with blastospore production and virulence to insects, and the MAD2 adhesin enables attachment to plants. Eukaryot Cell. 6:808–816. doi:10.1128/EC.00409-06.
- Wang C, St. Leger RJ. 2007c. The Metarhizium anisopliae perilipin homolog MPL1 regulates lipid metabolism, appressorial turgor pressure, and virulence. J Biol Chem. 282(29):21110–21115. doi:10.1074/jbc.M609592200.
- Wassermann M, Selzer P, Steidle JLM, Mackenstedt U. 2016. Biological control of Ixodes ricinus larvae and nymphs with Metarhizium anisopliae blastospores. Ticks Tick Borne Dis. 7(5):768–771. doi:10.1016/j.ttbdis.2016.03.010.
- World Health Organization. 2017. Global vector control response 2017–2030. https://www.who.int/vector-control/publications/global-control-response/en/ [Accessed May 1, 2022].
- World Health Organization. 2020. Malaria, https://www.who.int/health-topics/malaria#tab=tab_1 [Accessed May 1, 2022].
- Wright MS, Raina AK, Lax AR. 2005. A strain of the fungus Metarhizium anisopliae for controlling subterranean termites. J Econ Entomol. 98(5):1451–1458. doi: 10.1603/0022-0493-98.5.1451.
- Xia Y, Dean P, Judge AJ, Gillespie JP, Clarkson JM, Charnley AK. 2000. Acid phosphatases in the haemolymph of the desert locust, Schistocerca gregaria, infected with the entomopathogenic fungus Metarhizium anisopliae. J Insect Physiol. 46(9):1249–1257. doi: 10.1016/s0022-1910(00)00045-7.
- Yaginuma K. 1990. Detection of fungi pathogenic to peach fruit moth, Carposina niponensis Walsingham from soil. Bull Fruit Tree Res Stn. 17:77–89.
- Yanagawa A, Shimizu S. 2007. Resistance of the termite, Coptotermes formosanus Shiraki to Metarhizium anisopliae due to grooming. BioControl. 52(1):75–85. doi:10.1007/s10526-006-9020-x.
- Zafar J, Shoukat RF, Zhang Y, Freed S, Xu X, Jin F. 2020. Metarhizium anisopliae Challenges Immunity and Demography of Plutella xylostella. Insects. 11(10):694. doi:10.3390/insects11100694.
- Zhang F, Sun XX, Zhang XC, Zhang S, Lu J, Xia YM, et al. 2018. The interactions between gut microbiota and entomopathogenic fungi: a potential approach for biological control of Blattella germanica (L.). Pest Manag Sci. 74(2):438. doi:10.1002/ps.4726.
- Zhang L, Ying HS, Feng MG. 2014. Assessment of oral virulence against Spodoptera litura, acquired by a previously non-pathogenic Metarhizium anisopliae isolate, following integration of a midgut-specific insecticidal toxin. Biol Control. 79:8–15. doi:10.1016/j.biocontrol.2014.08.001. (in Chinese).
- Zhang S, Lizhen F, Yun J. 2002. Changes of some biochemical estimates in the hemolymph and body wall of Dendrolimus punctatus infected by Metarhizium anisopliae. Entomol Knowledg. 39(4):297–300. doi: 10.1007/s11769-002-0041-9.
- Zhioua E, Browning M, Johnson PW, Ginsberg HS, LeBrun RA. 1997. Pathogenicity of the entomopathogenic fungus Metarhizium anisopliae (Deuteromycetes) to Ixodes scapularis (Acari: Ixodidae). J Parasitol. 83(5):815–818. doi:10.2307/3284273.
- Zober MH. 1995. Metarhizium anisopliae, a fungal pathogen of Reticulitermes flavipes (Isoptera: Rhinotermitidae). Mycologia. 87(3):354–359.