Abstract
Recent discoveries of microplastics in cities, suburbs, and even remote locations, far from microplastic source regions, have raised the possibility of long-distance transmission of microplastics in many ecosystems. A little is known scientifically about the threat that it posed to the environment by microplastics. The problem’s apparent size necessitates the rapid development of reliable scientific advice regarding the ecological risks of microplastics. These concerns are brought on by the lack of consistent sample and identification techniques, as well as the limited physical analysis and understanding of microplastic pollution. This review provides insight regarding some unaddressed issues about the occurrence, fate, movement, and impact of microplastics, in general, with special emphasis on primary microplastics. The approaches taken in the earlier investigations have been analyzed and different recommendations for future research have been suggested.
1. Introduction: general aspects of microplastics
Plastics, regarded as a miracle material, have freed humanity from the socioeconomic constraints brought on by a scarcity of natural resources. Globally, fossil fuel feedstock accounts for 97–99% of plastics while the remaining 1–3% are derived from bio (plant) based. In the last few decades, the amount of plastic manufactured annually around the world has dramatically expanded, rising from 2 million tonnes in 1950 – 381 million tonnes in 2015.Citation1 After 2000, more than 50% of the total amount of manufactured plastic was introduced into the market. It is anticipated that their production would rise, even more, hitting approximately 600 million tonnes in 2025 (). About 42% of the plastic produced is consumed by the packaging business, with the construction and building sector using the remaining 19%. Numerous "essentials" in our daily lives, such as cling film, face masks, medical supplies, coffee mugs, and shopping bags are made of single-use plastic (SUP). The recent Covid-19 outbreak increased the usage of SUPs, particularly gloves, facemasks, and personal protective equipment (PPE) kits, which added 3.5% more to the world’s solid waste percentage. The US, India, and China are the largest producers of SUP, respectively.Citation2
Figure 1. (a) Plastic production till 2025 and (b) primary plastic production by industrial sector, 2015.
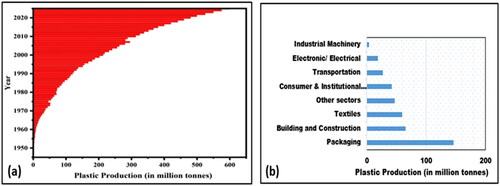
Plastics are ubiquitous in the environment due to their high manufacturing rates and longevity. Initially, larger plastic trash drew the attention of scientists and the general public. Tiny plastic particles, however, were found in the marine environment as early as the 1970s.Citation3 Microplastics (MPs) in the environment have gained more attention over the past several years and are now a prominent field of study. MPs are typically characterized as plastic particles that are less than 5 mm in size and have a wide range of shapes (including fibers, spheres, and fragments), sizes, colors, and compositions. Further, MPs can be categorized into primary and secondary regardless of their sizes. For application, for instance, in medical equipment and in personal care items like cosmetics and soaps, primary MPs are found in microscopic sizes (fibers, microbeads).Citation4 Large plastic items, when in contact with the environment, physical, chemical, and biological factors cause the plastic to break down into tiny fragments, creating secondary MPs, including mulch breakage debris and tire-wear debris, among others. Terrestrial and aquatic ecosystems are found to contain enormous and diverse MPs. As MPs are difficult to degrade, they remain in the environment for a long time, which results in significant and harmful environmental pollution.Citation5
This review addresses substantial knowledge gaps about the origin, destiny, migration, and effects of MPs, emphasising on primary MPs, which are becoming more widely acknowledged as a severe environmental threat. This study aims to provide a comprehensive understanding of the widespread presence of MPs in both terrestrial and aquatic ecosystems, their behavior, after entering the environment, as well as their potential negative effects on human and environmental health by integrating previous research findings. The goal of the review is to facilitate the development of evidence-based policies and interventions targeted at reducing the detrimental effects of MPs pollution by clarifying the sources, patterns of distribution, and ecological implications of primary MPs. Additionally, it offers guidelines for establishing effective risk management approaches for MPs by highlighting crucial areas for future research. This review seeks to give a thorough understanding of primary MPs and inspire the development of effective mitigation techniques, through a perspective, on which a relatively limited research is available.
2. Occurrence and distribution of MPs
Industrial, domestic and coastal activities are the prime anthropogenic actions that have resulted in the presence of these harmful plastic particles in the ecosystem (aquatic and terrestrial). In addition, the fragmentation of huge plastic garbage and residential runoff, comprising MPs fragments and microbeads (used in cosmetics and other consumer goods), are the major contributors to the entry of MPs into the aquatic ecosystem ().Citation6 The air-blasted resin pellets and powders that are released by the plastic manufacturing sectors also end up contaminating the aquatic environment. MPs pollution in the marine ecosystem is also caused by coastal activities like aqua tourism, marine industries, and fishing.Citation7 MPs pollution in soils is mostly attributed to sewage irrigation, plastic trash, agricultural facilities (such as agricultural compost), surface runoff, fertilizer use and atmospheric deposition.Citation8 In addition, MPs are produced during the stacking, disposal, or burning of plastic trash. Other sources of MPs in the environment include clothes, industrial dust emissions, housing infrastructure, and other daily life activities. MPs can be suspended and transported by air in the atmosphere since they are naturally low in weight.Citation9 MPs temporal and spatial distribution across the soil, aquatic systems, and the atmosphere is controlled by the transit of those materials, as these can migrate between multiple ecological divisions. For instance, through human activity, wind erosion, and runoff, MPs in the soil can also be carried into neighboring environmental matrices including the water and air.Citation10 Their movement within the soil is influenced by a number of aspects, including soil texture, cracking, biotic transport (soil biodiversity and soil community composition), and aggregation.Citation11
The vertical transportation of MPs in the soil is facilitated by agricultural methods, soil fissures, root growth, and soil pores. Organisms have also been observed to be involved in the mobility and distribution of MPs in soil.Citation8 Additionally, it’s possible for MPs in the soil to get into groundwater. Pollution from MPs in the environment may end up in the oceans and on land. Numerous elements, including wind direction, wind speed, particle density, and rain, have an impact on the transportation of MPs and their fallout or deposition in the atmosphere.Citation12 While some MPs settle through dry and wet deposition processes, others remain suspended in the atmosphere or eventually settle into aquatic and terrestrial ecosystems.Citation13 Further, under intense and ongoing hydrodynamic events like waves, rainstorms, ocean currents, monsoons, and tides, the aquatic environment is a dynamic region, and thus, MPs can travel between the marine environment and the land.Citation11 The fundamental characteristics of MPs, such as shape, density, color and size, can have significant impact on their transportation.Citation14
3. Classification and sources of MPs
MPs are classified into two main types based on their origin and manufacturing processes: primary and secondary. Primary MPs are intentionally manufactured particles, such as microbeads in cosmetics, resin pellets used in plastic production, and fibers from textiles. MPs in the micro-meter size are specifically manufactured for use in industrial abrasives for sandblasting, such as polyester or acrylic beads, plastic pre-production pellets (‘nurdles’), or personal care products like exfoliating agents in creams and cleansers comprising polyethylene ‘microbeads’. Primary MPs particles are most likely to reach wastewater treatment streams, after being washed down by industrial or domestic drainage systems. In spite of the capability of a few sewage treatment works to evacuate up to 99.9% the absolute number of particles entering the system may still permit a noteworthy number to bypass filtration frameworks, and be discharged into the freshwater environment with wastes.Citation15
Secondary MPs are created as a consequence of the fragmentation of the macro and meso-plastic litter, resulting from the degradation and disintegration of larger plastic items, such as bottles, bags, and packaging materials owed to environmental factors like UV radiations, mechanical abrasion, and microbial activity.Citation16 High temperatures and UV radiation can induce chemical changes in plastics, causing them to become brittle and therefore more prone to the fragmentation. Fragmentation allows greater surface area and particle count per unit mass. The contributing factors of fragmentation in marine waters are both wave action and sunlight. Plastic fragmentation is considered to occur readily on land, particularly at the soil surface, as a consequence of exposure to ultraviolet radiation from the sun, assisted also by temperature variations that are usually greater than those found in sea water. Despite the fact that microfibres are secondary particles, they will be released into the environment alongside primary MPs, via sewage effluents as well as sludge disposal. As a result, based on similar release routes, the fate and transfer of such fibers could be more closely linked with that of primary MPs. Understanding the distinction between the primary and the secondary sources is crucial for comprehensively assessing the impact of MPs on the environment and human health as depicted in .
3.1. Primary MPs
MPs particles are employed as exfoliants in some personal care product segments, such as hand cleaners, facial cleaners, and toothpastes.Citation17 Polyolefin particles exhibiting a size of 74-420 μm and an amorphous frame, with no sharp edges were characterized as suitable for use as exfoliants in a US patent for skin cleaners containing plastic microparticles.Citation18 Polyolefins used in them include polyethylene (PE), polypropylene (PP), and polystyrene (PS). Scrubs have been shown to contain PE microbeads in concentrations ranging from 0.4 to 10.6%, with sizes ranging from 40 to 1000 μmCitation17. According to a Cosmetics Europe survey, PE accounted for 93% of the MPs used in skin cleaner products in these countries in 2012. MPs are also utilized in medical settings, such as in dental tooth polish and also as delivery systems for pharmaceutical active ingredients.Citation19 MPs from medical and personal care products can be released into the environment post used via wastewater. MPs are also used in industrial abrasives used for air-blasting the paint off the metal surfaces and cleaning various types of engines, as well as drilling fluids for oil and gas exploration.Citation20 MPs made of poly allyl diglycol carbonate, acrylic, PS, melamine, and polyester (PES) are among the materials found in industrial abrasives.Citation21 These may enter the environment if used in open systems or improperly disposed off. Another significant source of primary MPs is the raw material utilized to make plastic products (pre-production plastics), specifically plastic resin flakes or pellets and fluff or plastic powder that can enter the environment as a result of improper handlings, such as through run-off from processing plants or accidental loss during transit. Furthermore, degranulate generated during the recycling of plastic and remnants from plastic processing factories can eventually reach the aquatic or terrestrial ecosystems.
Glitter, a form of MPs, comprised of tiny, flat, shiny particles, is commonly used in household items, cosmetics, and arts and crafts. It is typically made of MylarTM, a type of polyester film known as BoPET (biaxially-oriented polyethylene terephthalate), which has a melting point of 260 °C and a real density of 1.38 g/cm3,Citation22 These particles are water-insoluble and often coated with metal to enhance the reflectivity. While plastic glitters come in a wide range of colors, shapes, and sizes; their environmental impact is a growing concern due to their potential to harm the ecosystems, as they can easily enter the environment and pose risks to life and human health. Though, some manufacturers have introduced biodegradable glitter alternatives made from plant-derived materials; despite this, the widespread use of plastic glitters continues, to raise the environmental and health concerns. Therefore, it is essential to address the ecological and health implications of glitter as a primary MPs, and to explore sustainable alternatives to mitigate its environmental impacts.Citation23
3.2. Secondary MPs
Secondary MPs are produced when bigger plastic materials break down into smaller pieces. The four human activities responsible for the plastic entery into the environment are thought to be general littering, the dumping of plastic waste, loss from poorly managed landfill sites, and waste collection.Citation24 Additionally, waste from the recycling facilities is lost due to wind contributes significantly toward the MP polluton.Citation25 A huge portion of the plastics, that is produced and used for packaging of goods, with a limited shelf life is habitually dumped in the landfills and is often covered periodically with soil or synthetic material to prevent the trash from being blown away. During natural calamities like strong seas, tsunamis, and hurricanes, substantial amounts of these plastic particles may be released into the ocean.Citation25 Another significant source of MPs found in the environment is low-density polyethylene (LDPE) films, which are employed in enormous quantities to raise soil temperature, protect crops, suppress weeds, and hold irrigation water in the soil (a process known as "plastic mulching"). The particles from these thin plastic foils could wind up in the soil if they crumble. Additionaly, a significant culprit of MPs release in the fibers released after washing PES blankets, fleeces, and shirts in home washing machines. During a single wash, the tested PES fleece released over 1900 fibers in total. Also, synthetic textile fibers are discharged into the air during routine use or tumble drying.Citation26 There are numerous other places from where MPs can enter the environment, such as car tyre abrasion and application of paints & protective coating. Abrasion from other plastic materials, such as household plastics, results in the release of MPs as well.Citation27 Material lost or discarded from fishing boats, aquaculture facilities, merchant ships, recreational boats, offshore oil or gas platforms, and during military operations are examples of ocean-based sources of marine litter. The dominant form in the environment is still unknown, but it is likely location-dependent; for example, the primary MPs may be more significant in areas close to wastewater discharge sources than secondary MPs, which may predominate in open waters. provides the spatial and temporal pattern in the abundance of Primary MPs.
Table 1. Spatial and temporal pattern in the abundance of primary MPs.
4. Characteristics of MPs
MPs pollution of the environment is now recognized as a risk to ecosystem and researchers have begun to address this field as an issue of importance in recent years, after recognizing the comparative lack of studies on MPs. The effects of MPs on soil ecosystems (both below and above ground) are almost unexplored. Furthermore, the effects of MPs on terrestrial systems vary depending on the type of MPs. It has been demonstrated that the physical and chemical characteristics of MPs influence their ability to bind pollutants.Citation51
4.1. Physical properties of MPs
4.1.1. Size
The sampling and analytical techniques are frequently used to identify the sizes of micro/nano-plastics. While MPs come in a variety of sizes, the most prevalent range from 1 µm to 5 mm, nano-plastics (NPs) are approximately <100 nm in size.Citation52 The research on MPs size has gradually shrunk to less than 2000 µm, due to the progress made in the qualitative and quantitative approaches for MPs. The presence of smaller MPs in the atmosphere warrants additional investigation, as evidenced by the abundance of fluorescent non-fibrous particles <20 µm and smaller fibers that were found in the total atmospheric deposition in downtown London.Citation53 These investigations demonstrated that MPs, which can be inhaled and consumed, make up a non-negligible portion of the atmospheric particulate matter. Additionally, the research suggests that inhaling MPs could be harmful to human health.
4.1.2. Shape
The most frequent morphologies in atmospheric MPs are fragmented and fibrous. In addition, there are other shapes such as spheres, flakes, and films. MPs have distinct physical characteristics depending on their shape. Fibers are typically very thin, flakes are typically thick and asymmetrical, and films are typically thin and flexible. Overall, the primary MPs morphology at the time of manufacture, surface deterioration and erosion processes, and residence time in the environment all affect the form of MPs in the atmosphere.Citation54
4.1.3. Color
One of the key aspects for the identification of plastic debris and potential contaminants during sample preparation is color. There have been reports of MPs/NPs in a variety of colors, including green, red, blue, orange, grey, yellow, white, brown, off-white, and tan. For the visual evaluation of MPs particles in the atmosphere, color is generally a helpful parameter. While low-density polyethylene (LDPE) has been given opaque colors, polypropylene has been clear and transparent. When reporting and interpreting results, it is important to take into account that MPs discoloration can happen during sample preparation (H2O2-oxidative digestion) and weathering.Citation55 Like natural foods, brightly colored NPs particles are frequently consumed by marine species. MPs in the colors black, blue, green, and red are primarily consumed by copepods, zooplankton, fish larvae, and euphausiid.Citation56
4.1.4. Crystallinity
Plastics density, permeability, and swelling characteristics are affected by the organized structural links known as crystallinity.Citation57 As MPs/NPs spend more time in their surroundings, their crystallinity changes. The polymer’s amorphous area breaking down encourages overall crystallinity and shrinks MPs/NPs size.Citation11 Crystallite materialization may change MPs/NPs from their counterparts and change their toxicity. It ultimately affects the surface area, particle shape, size, and chemical characteristics such as the adsorption of contaminants and additives, which in turn affects the ingestion rate.Citation58
4.1.5. Surface properties
Surface chemistry and surface area are the two main components of surface property. Particles in the nanoscale have a significant impact because MPs/NPs surface area grows as particle size decreases.Citation58 The deterioration of the plastic surface by photo- and oxidative processes results in the formation of new functional groups when OH radicals, O and N oxides, and other photo-generated radicals are interacted with.Citation59 Due to the disruption of the plastic’s surface area by these pathways—chemical leaching, creation of angular-shaped NPs from primary microbeads, and ingestion—microscopic particles are liberated.Citation57 Furthermore, because the microbial community uses the oxygenated binding sites, surface chemistry has a substantial impact on particle-biota interactions.Citation60
4.2. Chemical properties of MPs
MPs comprise additives (plasticizers, antioxidants), polymers, dyes, and pollutants that get adsorbed on the surface. When plastics are produced, utilized, and deteriorate, these chemicals are easily discharged into the environment.Citation61,Citation62
4.2.1. Chemical additives
To increase the product’s efficiency, additives are added during the production of plastic to achieve the desired color, and transparency. These additions increase the polymer’s resistance to degradation by biological (bacteria and fungi), mechanical, physical (light radiation, ozone, and temperature) and electrical agents.Citation63 Additives include pigments, ultraviolet stabilizers, flame retardants, lubricants, and plasticizers as well as inert or reinforcing fillers. Additional ingredients include wood, clay or linen, graphite, cellulose pulp, glass fibers, jute, rock flour, cotton flakes and kaolin.Citation64 Low molecular weight additives embedded in the polymer matrix are brittle and can escape out into the neighboring water bodies (Polyethylene terephthalate (PET) oligomers from food trays and bottles; flame retardants from electronic items; nonylphenol from food contact materials; Lead (Pb) from un-plasticized PVC pipes; and Antimony (Sb) from PET water bottles).Citation59 The majority of MPs and NPs are additives and fundamental polymeric substances made from plastics and chemicals absorbed from the environment and are linked to plastic toxicity (i.e., monomers, plasticizers, solvents, additives-dyes, and catalysts).Citation60 These substances disturb the endocrine system, when ingested or inhaled, leading to metabolic diseases, hormonal imbalance, neuro-developmental abnormalities, reproductive issues and asthma. The impact of various additives in plastics on human health is given in .
Table 2. The impact of various additives in plastics on human health.Citation11,Citation65,Citation66
4.2.2. Chemical structure
Higher sorption capacity may result from a chemical structure with a wide surface area or high porosity. For instance, PET had the highest sorption rate, while PE had the largest sorption capacity. The PE, with a higher sorption capacity, leads to more pollutant diffusion, due to its bigger surface area, wider space between polymer chains, and free volume.Citation67 As opposed to PE, PET has a smaller surface area and a glassy polymeric structure, which do not facilitate diffusion into the material and so have a faster sorption rate.
4.2.3. Chemical interactions
Pollutant affinity for MPs varies due to chemical interactions such as hydrogen bonding, electrostatic interaction, π–π interaction, hydrophobic interaction, or van der Waals force.Citation68 For example, because of the amide groups, hydrogen bonding between polyamide (PA) and antibiotics caused strong affinity.Citation69 The difference in the surface charges between amine-modified polystyrene (PS-NH2) and carboxyl-functionalized polystyrene (PS-COOH), caused embryonic apoptosis, oxidative stress, cell death, and disruption of the cell membrane in the embryos of the sea urchin Paracentrotus lividus.
5. Bioaccumulation of MPs
5.1. Transfer mode of MPs in human body
MPs enter the human body through three main routes: ingestion, inhalation and skin contact ().Citation70 Due to their prevalence in the water supplies and food chain, MPs are easily consumed.Citation71 MPs are not able to penetrate the epidermal membrane being too thin, but they might still enter through hair follicles, wounds, or sweat glands. As they can easily enter the environment and pose risks to marine life and human health. The fact is that human body is exposed to MPs through all three channels, although environmental and seafood particles pose the highest danger of absolute exposure.
Figure 4. Three main routes by which plastic particles enter the body: Inhalation, ingestion, and skin contact.
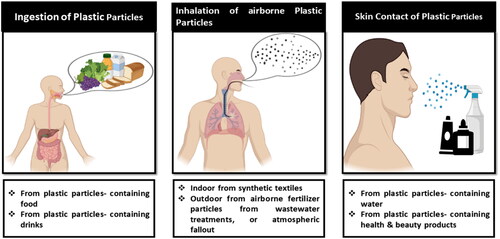
5.1.1. Gastric exposure
Recent investigations on MPs exposure and toxicity have shown that ingesting plastic particles is the main way that people are exposed to.Citation72 There are studies demonstrating that MPs are being consumed through food and drink, but there aren’t any explicitly examining the toxicity of NPs in individuals.Citation73 Human feces samples were initially examined, and the results revealed that plastic particles were being ejected, supporting the theory that people are consuming these particles through water and food. These observations, along with studies on ingested uptake in environmental models, unequivocally demonstrate that MPs and NPs consumption by humans will be pervasive.Citation74 It would be vital to investigate their pathway via the GI tract and identify whether these particles translocate across the gut epithelia or persist in the gut lumen. MPs ability to penetrate at the paracellular level is uncertain since the pores at the tight junction channels i.e., relevant have a maximum functional size of about 1.5 nm. It is possible that they will enter via lymphatic tissue, and it is more likely that they enter through endocytosis or phagocytosis and invade the Peyer’s patches’ microfold (M) cells.Citation75
5.1.2. Pulmonary exposure
Inhalation is the second most likely way for a human to come into contact with NPs. Indoor spaces have airborne plastic particles, mostly from synthetic textiles, which can cause occupational exposure or unintentional inhalation.Citation76 Exposure in outdoor areas may occur by inhaling contaminated aerosols from the ocean’s waves or airborne fertilizer particles from dry wastewater treatment processes. The lung’s alveolar surface area is substantial—about 150 m2—and its tissue barrier is very thin—less than 1 µm. Nanoparticles can distribute throughout the entire human body because this barrier is permeable enough to pass through and into the capillary blood system.Citation72
5.1.3. Dermal exposure
MPs/NPs are also commonly found in beauty and health products, especially in body and facial cleansers that are used directly on the skin.Citation77 Although there isn’t enough evidence to draw firm conclusions on the impacts of nanocarriers, skin penetration depends heavily on the size of the particles and the health of the skin. Only one study describes that the synthetic textile causes the penetration of nanoparticles on the epidermal barrier at a very minute quantity. The stratum corneum, the skin’s outermost layer, serves as a barrier to protect the skin from injury, microbial agents, and chemicals. Corneocytes comprise the stratum corneum, which is encased in lamellae of hydrophilic lipids which include long-chain free fatty acids, cholesterol, and ceramide. The skin could be exposed to plastic particles by contact with contaminated water or through the use of beauty and health products.Citation75 Plastic particles might enter the body through sweat glands, skin wounds, or hair follicles because MPs and NPs are hydrophobic, making it unlikely to pass through the stratum corneum and be absorbed.
5.2. Transfer in the bodies of animals (terrestrial and aquatic)
5.2.1. Terrestrial animals
MPs are ingested orally, where they enter the body, travel through the digestive system and is subsequently eliminated. However, a small number of MPs can persist for days in the intestine of organsms.Citation78 Cells can absorb MPs in the intestine through endocytotic processes.Citation79 MPs that enter the intestines have also been demonstrated to induce inflammation and mechanical damage. Because of this damage to the intestinal epithelium, MPs can easily spread to other tissues and organs of organisms or enter their bloodstream through intestinal capillaries. Recently, it was shown that NPs can permeate the mucosa and muscularis layers of the intestine, pass beyond the gut epithelium, and then be transferred into other organs (such as the liver) by blood circulation.Citation80 This was done using an ex vivo salmon (Salmo salmar) gut sac exposure system.Citation81 Additionally, MPs can physically break up into smaller pieces as they go through the digestive system, which helps to facilitate their translocation.Citation82 An inhalation investigation in maternal rats also revealed that 20 nm NPs translocated to the fetal liver, brain, heart, kidney, and lungs by intratracheal instillation in addition to placenta, heart, and spleen during gestation.Citation83
5.2.2. Aquatic animals
MPs in aquatic animals’ gill canals could be directly injected, translocated to the gastrointestinal tract, or intercepted and then conveyed to the capillaries via the gill filaments.Citation84 Exposure to MPs caused several types of damage to fish gills, such as the lifting of epithelial, inner opercular membrane swelling, and aneurysms in secondary lamellae. Another potential pathway for MPs entrance into animal bodies is through the epidermis. By adhering to animals’ damaged skin during the skin’s healing phase, MPs can infiltrate muscle tissue. According to certain reports, some plastic that was wrapped around sea turtles eventually grew into their muscles.Citation85
6. Fate of MPs
MPs appear to have no limits in the environment, and they are now pervasive throughout all ecosystems in the world (). Plastic waste that has been released into the environment can be carried by the wind, swept from the land to surface waterways during rain, particularly with stormwater runoff, and transported in both freshwater and seawater. Large rivers are expected to transport a significant amount of micro- and macro-plastics into the oceans, although there aren’t many studies to support this hypothesis. Over 50% of the world’s population lives within 80 kilometers of the sea, thus, wind transport of macro-plastics to the oceans may be considerable. Very small MPs, which could be mobilized from open landfills, for example, may potentially be appropriate for airborne transport.
Depending on the density, plastics will either hover or submerge in water. Plastics having a density less than freshwater (about 1000 kg/m3) or seawater (about 1030 kg/m3) will be buoyant. The majority of consumer plastics, including PE, are buoyant in saltwater (). Plastics are typically colonized by a variety of species in aquatic environments. By increasing the density, this fouling can cause formerly buoyant objects to sink beneath the water’s surface. The density of a plastic object may decrease if it becomes fouled by other species, causing it to resurface in the water. The specific density of MPs may also vary due to erosion, and wind-driven mixing of the upper water layer may cause previously buoyant MPs to sink. Depending on ocean currents, local winds, and shoreline geography, plastics can be transported over great distances. Beaches and oceanic gyres become overrun with buoyant plastic trash, and benthonic debris which occurs on the sea floor in the region with little circulation. Because smaller particles at the water’s surface are less wind-exposed than larger ones, the mobility of MPs in surface waters may vary from that of macro-plastics.Citation87 Animals activity and aggregation of particles may also have an impact on the distribution of MPs. MPs can be covered by silt on beaches and in subtidal sediments, where they can be found at great depths. The movement of MPs throughout the terrestrial environment is still mostly unknown. It was established that the assimilation of micro- and macro-plastic particles into the soil is facilitated by terrestrial organisms like moles and earthworms. Plastic’s photo-oxidative degradation is somewhat effective on a beach surface, but very slowly for plastics buried in soil or beach silt, or deep ocean.Citation88
Table 3. Plastic material densities that are frequently observed in aquatic ecosystems.Citation86
7. Ecological risks of MPs
7.1. Effects of MPs on the aquatic ecosystem
Atmospheric MPs penetrate the aquatic ecosystems by both dry and wet deposition. Thus, atmospheric deposition is a significant cause of the occurrence of MPs in the aquatic environment. Due to their longevity, MPs are persistent in aquatic conditions. Some MPs, might sink to the ocean floor as a consequence of having a higher density than sea water, whereas other MPs that are less dense than seawater frequently float on the water. Organisms in freshwater and the ocean may consume MPs.
7.1.1. Effects on aquatic animals
Diverse aquatic organisms, including invertebrates, mammals, fish, shellfish, and fish, consume MPs that enter the aquatic ecosystem. Both active ingestion of seawater and passive ingestion of prey can expose mammals to MPs.Citation89 Because of the chemicals involved, MPs can have an impact on the gut microbiome of aquatic animals.Citation90 They may build up in the gastrointestinal tracts of aquatic animals, preventing them from ingesting and properly digesting food.Citation91 Aquatic animals may become poisonous or even die as a result of this. Ingestion of modest dosages of MPs does not always result in the rapid death of organisms. MPs can have a major toxicological impact on fish, including the introduction of oxidative stress and inflammation in the gut. Non-aquatic animals like penguins and seagulls have been known to accidentally consume MPs that float on the water’s surface. The MPs wafting on the water are susceptible to intentional or unintentional feeding by zooplankton because they resemble their food in size and colour.Citation92 Microfibres, mostly generated from synthetic textiles, seem to have a higher possibility than other fibers to infiltrate the food chain, as a consequence of ther size and shape. Ther size permit them to be promptly consumed by the aquatic organisms and are more susceptible to becoming entwined in large lumps inside the gut, instigating blockages.Citation93 In addition to having a deleterious impact on the flora and fauna directly, MPs can concentrate on living beings, reach the food chain, progress to various trophic levels, and potentially pose a risk to human health.
7.1.2. Effects on aquatic microorganisms
MPs increase the amount of inorganic and organic carbon in the aquatic environment, which increases microbial activityCitation94 and can cause additional CO2 to be released into the aquatic environment resulting from changes in the CH4 cycle. The MPs invasion altered the structure and composition of the bacterial communities and reduced the diversity and richness of the biofilm.Citation95 Food webs and carbon metabolism in aquatic environments are impacted by changes in the aquatic microbiome. Microorganisms prefer using MPs as novel substrates because of their buoyancy, hydrophobic surface, and capacity for long-distance transmission.Citation96 The colonization of pathogenic microbes on the surface of MPs may impact the transfer of deadly microbes into the aqueous environment.Citation97
7.1.3. Effects on hydrophytes
MPs that float on the water’s surface inhibit phytoplankton from absorbing light, which prohibits them from offering oxygen and food to aquatic organisms.Citation98 The architecture of phytoplankton communities can be drastically altered by high MPs concentrations.Citation99 Food webs and ecological processes in the aquatic environment will be impacted by the modifications to phytoplankton colonies. MPs also impair chlorella’s capacity for the process of photosynthesis through oxidative stress and physical deterioration.Citation100 Algae could become more prevalent in some areas of the phytoplankton habitat. Because they are generally denser than water, common MPs gravitate into aquatic habitats. Submerged plants will experience oxidative stress, antioxidant defenses, and chlorophyll fluorescence characteristics because of these MPs, which will result in decreased plant height.Citation101 Plants submerged in water may be exposed to MPs through their leaves or roots.Citation102 The presence of microfibrils in the cell wall provided evidence that MPs had penetrated the plant.
7.2. Effects of MPs on the terrestrial ecosystem
MPs are introduced into the terrestrial environment through littering and the dumping of sewer sludge to land. MPs have the capability to penetrate the terrestrial environment through atmospheric transport and deposition. Studies report that both dry and wet deposition are ways in which atmospheric MPs penetrate the terrestrial environment.Citation103 Tyre plastic may escape into the ground from the road.Citation104 MPs from the atmosphere that enter the terrestrial environment either linger in the soil for a long period or decay gradually. MPs build up significantly in the soil, potentially endangering terrestrial ecosystems.
7.2.1. Effects of MPs on the soil biophysical environment
The soil ecosystem is negatively impacted by MPs in a variety of ways depending on their type, size, and shape. Wearing clothing has also been shown to cause textiles to emit MPs into the air, which are then deposited in soil.Citation105 When textile waste is dumped as litter or thrown away in landfills, it can disintegrate and cause fibers to leak into the ground. Examples include single-use face masks, ropes, tarpaulins, and misplaced clothing.Citation106 It is believed that organisms like earthworms have the ability to move considerable quantities of MPs from the soil surface to greater depths.Citation105 MPs, which resemble soil particles in size and form, have less of an effect on the soil’s structure.Citation107 Since fibrous MPs have a very different form from soil non-linear particles, it is possible that they can coil around soil particles more effectively. MPs shapes have the power to change the biophysical characteristics of the soil. According to a study, MPs can alter the soil’s structure and impair its bulk density and water-holding capacity.Citation108 Types of MPs have an impact on how MPs and soil pore space interact. As opposed to PES beads, polyacrylic beads and PE fragments did not significantly lessen soil bulk density.
7.2.2. Effects of MPs on soil organisms
MPs pollution changes the soil’s physicochemical characteristics, which impacts soil hydrodynamics and microbiological activity.Citation109 Different soil microorganisms are affected by MPs in different ways. The microbial populations in the soil may unavoidably change as a result, having effects on the soil’s flora and fauna. MPs affect the composition of the microbial community in the soil. It was discovered that PE considerably altered the composition of bacterial communities and had an impact on their succession.Citation110 By altering the soil environment, MPs have an impact on the evolution of soil microorganism.Citation111 MPs can provide nutrients and organic carbon to the soil, and bacteria that make use of this resource have a higher chance of surviving.
Moreover, MPs can be consumed by soil animals. Consumption of MPs may cause soil animals to receive insufficient nutrients, experience oxidative stress, and suffer intestine injury, which will impede their ability to develop and reproduce.Citation112 Lower concentrations of MPs had an impact on the mortality of soil animals in spite of the presence of tissue and immune system damage.Citation8 Future studies are required to determine whether the immune system and histopathology can assist as possible indications of sensitivity to ingested MPs. The effects of MPs on soil creatures might vary, depending on their size and concentration. Through experimentation, it was discovered that the presence of MPs could have a considerable impact on the diversity and abundance of nematode and microarthropod communities.
The soil food chain may be affected by the way soil fauna reacts to the addition of MPs. The presence of MPs can drastically alter the soil fauna through extractable chemicals in MPs.Citation113 MPs from the atmosphere may contaminate soil and have an impact on plant growth as well as on the leaves of the plants through dry and wet deposition. MPs present in the air can cling to leaf surfaces and even enter plants through stomata. Because of the morphology of the leaf stomata, the majority of the MPs that attached to and entered the leaf were found to be fibrous.Citation114 Stomata in plant leaves take in NPs and send them downward to the roots. MPs foliar absorption may impact a plant’s ability to photosynthesis.Citation115 Foliar MPs exposure can significantly slow growth by reducing the area of leaf, weight of dry crop and height of crop.Citation116
7.3. Effects of MPs on the biogeochemical cycles of soil carbon and nitrogen
The carbon and nitrogen cycles, soil microorganisms, climate change, and greenhouse gases (GHGs) are all interconnected in significant ways. According to certain research, MPs deposited into the soil can change the makeup and operation of microbial communities, which in turn can influence the carbon and nitrogen cycles in the soil.Citation117 According to a study, MPs additions can considerably increase CO2 emissions from soil.Citation58 This might accelerate global warming and lead to additional severe environmental issues. The addition of MPs had no impact on soil nitrogen emissions, though. The size of MPs has an impact on the type of dissolved organic carbon in soil. According to a study, PVC hindered sediment nitrification and denitrification, while PUF and PLA encouraged it. The soil’s ability to hold nitrogen was diminished in MPs-treated soils, as MPs modify the porosity of the soil, which could further impact the nitrogen cycle.Citation117 MPs could affect the carbon, nitrogen, and phosphorus-related genes and enzymes in soil bacteria, which in turn could affect the soil nutrient cycle.Citation118
7.4. Effect on human health
As per studies, humans are primarily in continuous contact with environmental MPs through inhalation, ingestion, and skin contact.
7.4.1. Inhalation by respiration
Gravity causes MPs to settle in different parts of the human respiratory system. The body’s concentration of MPs can be decreased through the clearance mechanism. The clearance system may be slowed down by MPs particle toxicity brought on by cytotoxicity, excessive dust load, oxidative stress, and particulate matter translocation, thus impacting the overall human health.Citation119 Because only MPs having sizes less than 10 µm can be ingested, an MPs size influences its respiratory potential. Since, MPs smaller than 10 µm were found both indoors and outdoors, they can also enter the human body though inhalation. Even though mucociliary clearance occurs for the majority of the coarse inhalable particles in the upper airway, some tiny particles can evade this system and settle deep inside the lungs.Citation120 As, fragments are mostly transmitted by air, 31 synthetic polymer particles and fibers, of which 87.5% fragmented and 12.5% were fibers, were found in the lung tissue of 13 of the 20 autopsies of the deceased, validating this theory. The upper respiratory tract retains the majority of the MPs that are inhaled. The duration and intensity of MPs exposure also have an impact on the impacts on human health. High levels of MPs in the environment could pose serious threats to people’s health.Citation121 A mannequin could simulate inhaling 272 MPs within 24 h using a breathing thermal manikin.Citation120 When it comes to exposure to airborne MPs, young children are typically more at risk than adults.Citation122 Low concentrations of atmospheric MPs typically expose the general public. However, practitioners who are exposed to high levels of MPs on the job, run the risk of developing occupational illnesses.Citation123 The size and form of MPs have a significant impact on cytotoxicity.. Lung cells exposed to MPs experienced significant morphological alterations and a reduction in cell growth.Citation124 Human lung epithelial cells’ internalization, cell viability, cell cycle, and apoptosis may be impacted by NPs.Citation125 It was shown that exposure to MPs caused inflammation, oxidative damage, and disruption of intercellular junctional proteins in normal human lung epithelial cells BEAS-2B.Citation126 Respiratory illnesses, both acute and chronic, may develop from this. Among other things, metals, possible PAH transporters, plastic additives, and airborne MPs were suspected of producing chemical toxicity.Citation75
The inflammatory potential of MPs with additives was often higher than that of MPs without additives.Citation123 Ultrafine particles (UFPs) may be inhaled and diffused from the lungs into the bloodstream, or they may be ingested after airway mucociliary clearance. These inhaled or ingested particles may continue to travel in the bloodstream and become harmful to other organs after translocation, including the liver, embryo, brain, etc.Citation121
7.4.2. Oral ingestion
MPs are typically found in the food and water consumed by humans. Additionally, a lot of MPs are present in commonly used tap water, bottled drinks, and alcohol. In tests of tap water and various brands of bottled water from around the world, MPs particles were found.Citation73 95% of MPs particles having size in the range of 6–100 µm, or 80% of MP particles with a size of 5–20 µm, were found in bottled water. Moreover, alcohol, salt, and shellfish all contain human-caused MPs pollution. Some MPs that reach the human digestive tract are expelled through fecesCitation127 and some are still seen in human babies. The human body can develop abrasions, perforations, and even obstructions in the digestive tract as a result of MPs ingestion.Citation128 All plastic particles exhibited high resistance to artificial digestive juices, as demonstrated by the effects of artificial in vitro digestion on five types of MPs, and the primary stages of the human gastrointestinal tract do not break down particles.Citation129 PE could decrease cell functions and alter the composition of intestinal flora, which would be dangerous for human health, according to in vitro simulation studies on human cells Caco-2 and intestinal flora.Citation130 On the other hand, when PS of various sizes was utilized on Caco-2 cells, the particles did not harm or inflame the cells.Citation131 MPs at the ambient level are unlikely to provide a severe health concern to humans when consumed orally. Epidemiological studies, in vivo animal experiments, and in vitro cell, culture techniques are used in the majority of current investigations on MPs in the human digestive system. MPs present in the human body can accrue in the digestive system or go to other organs e.g., liver, kidney, by oral consumption.Citation132 Since MPs get decolorized in the digestive tract, the majority of MPs samples found in human digestion were clear laments.Citation133 It is thus far uncertain whether the MPs pigments stick around in people’s bodies and harm them.
7.4.3. Skin contact and dermal absorption
Pollutants in the form of particles have been linked to skin ageing. Particles from traffic can worsen wrinkles in the nasolabial creases and freckles on the cheeks and forehead. Nevertheless, tiny particulate matter is frequently viewed as a significant environmental hazard that leads to skin illnesses. Skin illnesses can develop and worsen as a result of various air pollution particulates that cause oxidative stress by releasing pro-inflammatory cytokines and reactive oxygen species (ROS). Further study in this area is required because there is no direct indication that atmospheric MPs can enter the skin.
8. Identification methods in MPs analysis
Monitoring of MPs in a range of biotic and abiotic environmental matrices can give fundamental scientific data on their pollution status, concentration hotspots, historical trends, and the fate and exposure of organisms. MPs monitoring studies demand accurate and comparable sampling and analytical techniques. In addition, proper qualitative and/or quantitative analytical procedures for MPs are needed for laboratory accumulation, toxicity, and weathering research. However, because MPs research is a recently reemerging topic, analytical methodologies for MPs are still in the early stages of development. MPs in environmental samples can be analyzed using extraction, isolation (or separation), identification, and quantification techniques (or classification).Citation134 Traditionally, preproduction resin pellets and other large MPs in the 1–5 mm size range have been identified by visual sorting with the naked eye.Citation135 However, in order to successfully perform an ecological risk assessment, quantification at the smallest scale is essential due to the ubiquitous environmental presence of MPs and their harmful biological consequences.
Researchers have been unable to create a uniform classification of MPs data due to the vast range of sizes of MPs and the complexity of their colors, shapes, and polymer kinds, which makes it more challenging to compare data. Using a single analytical technique, it is challenging to completely and accurately identify MPs of different sizes, shapes, and polymer types from complex environmental matrices. Therefore, it has become common practise to combine more than two analytical methodologies. MPs examination often involves two steps: physical evaluation of putative plastics (using a microscope, for example) and chemical characterization (using a spectrometer, for example) to confirm the presence of plastics. Each technique, as well as different combinations of them, has benefits and drawbacks.
8.1. Visual methods
Large MPs (1–5 mm) were commonly found on beaches and, to a lesser extent, in surface waters before the term "MPs" gained popularity. The size range of MPs is rather wide, hence sorting and identification was typically done side by side in a tray using forceps and the human eye. This visual method can be used to identify some small plastics, and colorful plastic pieces and pre-production resin pellets that vary in size from 2 to 5 mm and can be recognized by sight. There is a greater likelihood that small plastic particles will be missed by sorting in high strandline samples taken from beaches with high concentrations of interfering inorganic and organic materials, or in ambiguous plastics (approximately 1 mm in size) with colors similar to the interfering particles. However, for both specialists and nonprofessional volunteers who have undergone brief instruction, visual sorting and identification of big MPs offers an easy, straightforward, and quick procedure.Citation136
8.2. Stereo (or dissecting) microscopy
A popular method for identifying MPs with sizes in the hundreds of micron range is stereo- (or dissecting) microscopy (e.g., neuston net samples).Citation137 Microscopy-enhanced photos give objects’ surface textures and structural details in great detail, which is crucial for recognizing confusing, plastic-like particles. Although most particles in this size range can typically be identified by microscopy, it can be challenging to confidently identify plastics in sub-micron-sized particles (<100 µm) that lack a characteristic color or shape.Citation138 The microscopic detection of MPs on filter paper can be hampered by sediment samples whose light sediment particles are poorly separated by density. Microscopic inspection is further complicated by biogenic elements from silt and neuston net samples that have not been entirely removed by chemical digestion. According to numerous field monitoring studies, the majority of the MPs in water, sediment, and biota were made up of fibres.Citation139 However, by using only a microscope, it was challenging to distinguish between fibers that were synthetic (like polyester) and natural (like colored cotton).
8.3. Scanning electron microscopy (SEM)
Images of plastic-like particles can be seen at extremely high magnification and clarity using scanning electron microscopy (SEM). The separation of MPs from organic particles is made easier by high-resolution pictures of the surface roughness of the particles. The elemental makeup of the same object is revealed by additional investigation using energy-dispersive X-ray spectroscopy (EDS). Carbon-dominant plastics can be distinguished from inorganic particles by looking at the elemental makeup of the particles. The number of samples that can be processed using SEM-EDS is constrained by its cost and the time and effort required for sample preparation and analysis. Plastic colors cannot be utilized in SEM as identifiers. The technique is advised for additional elemental analysis and surface characterization of particular plastic particles.Citation139
In some instances, other cutting-edge microscopy methods have been utilized to identify plastic particles. In laboratory accumulation and toxicity investigations, polyethylene (PE) particles were successfully identified using polarized optical microscopy. Polarized light transmission can be affected by the crystal structure of plastics, and this transmission can then be measured.
8.4. Fourier transform infrared (FTIR) spectroscopy
A FTIR can be used for the identification to disclose the polymer composition as well as the abundance of MPs, which can assst in identifying identifying the source. The weathering status of MPs is also shown by the proportional content of oxygenated bonds (such as carbonyl groups) in the IR spectra. By using this technology, it is simple to identify carbon-based polymers, and the distinctive spectra produced by various bond compositions, which allow the plastics to be distinguished from other organic and inorganic particles. A reliable polymer spectrum library permits the identification of particular polymer types in addition to the confirmation of plastics. Micro-FTIR (m-FTIR) is used to conduct microscopic observation of micro-sized plastic-like particles before spectroscopic identification on a single platform by shifting between the object lens and IR probe, which is necessary for small MPs. FTIR measurement for MPs is accessible in transmission, reflectance, and attenuated total reflectance (ATR) modes.Citation140 The sample preparation step is not necessary for thick and opaque MPs in the reflectance and ATR modes, in contrast to the transmission mode. Additionally, the ATR mode creates stable spectra from uneven MPs surfaces, whereas the reflectance mode creates unstable spectra. Theoretically, it is possible to detect MPs as small as the ATR probe’s IR beam aperture (10 µm, for example). With the identification of plastic-like particles by microscopy and subsequent chemical confirmation by spectroscopy, m-ATR-FTIR now offers a promising way to identify MPs in environmental samples. However, it can be challenging to get distinct spectra for MPs with a maximum length of 50 µm, necessitating numerous attempts. Surface contact analysis is a type of measurement used in ATR-FTIR. MPs that are extremely worn or delicate may be damaged by the pressure from the ATR probe, and small plastic particles may be attracted to or pushed off the filter paper by electrostatic interaction with the probe tip. When used in contact analysis, remains of hard, sharp inorganic particles from sand samples can easily harm an ATR probe manufactured of germanium. By employing the IR spectra to identify each plastic-like particle, it is possible to limit the possibility of missing plastic particles and false-positive quantification of non-plastic particles. m-FTIR equipment is quite pricey. It takes a lot of time to use an ATR probe to identify each and every plastic-like particle. Samples may contain a large number of moderately or severely weathered MPs that are often formed of complicated and occasionally composite materials. Therefore, to acquire clear spectra and correctly interpret them, an expert operator is needed. A professional judgment based on a detailed examination of the spectrum is required when weathered plastics exhibit spectra of different polymers that have poor hitting potential in libraries. This may result in decisions that are made arbitrarily or based on statistical data and the criteria for meeting the percentage values. The human labor required in the FTIR process can be reduced by using pre-programmed, semi-automatic mapping without the requirement for microscopic pre-selection of particles for analysis.
It is uncommon to employ single FTIR mapping for routine examination of complicated field samples since it requires longer operating durations and only covers a small region for the chosen wavenumbers of certain polymer types. When compared to single beam mapping, the shortcomings of the focal plane array (FPA)-based reflectance imaging method could be approved. This method could provide information for the identification of MPs (150-250 µm) on larger surface areas, more quickly, and without sacrificing spatial resolution. Although FTIR operation time is greatly reduced by semi-automatic mapping technology, it still takes at least 9 h to scan a single filter paper. To enable frequent use of this approach in MPs analysis, other challenges must be resolved, that include the potential for plastics to be aggregated and the potential for plastics with irregular shapes to cause refractive errors in the reflectance mode. Therefore, after the analysis, each location and spectrum must now be manually validated. Smaller plastics (e.g., <100 µm) need additional identification due to the reflectance mode’s limits in producing clear spectra for tiny plastic particles. This needs more time for identification by ATR-FTIR or microscopy.
8.5. Raman spectroscopy
Micro-plastics have also been identified by using Raman spectroscopy. Each polymer has a distinct spectrum due to the molecular structure and atom composition that influence the backscattered light frequencies produced when a laser beam strikes an item. Raman analysis not only distinguishes between different types of plastics but also offers profiles of each sample’s polymer makeup, much like FTIR. Raman spectroscopy is analogous to the FTIR method when it comes to the nondestructive chemical analysis and microscopy combination, including the need for pricey equipment. In difficult MPs identification, the various reactions and spectra from FTIR and Raman spectroscopy can conflict with one another. Raman spectroscopy, which uses a narrower laser beam than FTIR, can identify MPs as small as a few millimeters in size. The advantage of Raman spectroscopy’s non-contact examination is that the MPs samples are preserved for potential future analysis. MPs in zooplankton samples can be found using confocal microscopy and Raman spectroscopy. Raman spectroscopy is sensitive to the addition and pigment compounds included in MPs, which makes it difficult to distinguish between different types of polymers.
8.6. SERS (surface-enhanced Raman spectroscopy)
SERS is a rapid, noninvasive spectroscopic analytical technique that uses distinct molecular vibrational fingerprints to identify analytes at the single-molecule level.Citation141 Most molecules in a typical Raman scattering process produce weak Raman signals due to their relatively lower Raman scattering cross-section. Increasing the Raman signals to greater than or equal to the fifth order in SERS requires the use of plasmonic nanostructures composed of Ag, Au, Cu, and Al. The primary mechanism behind this amplification is the activation of localized surface plasmons, which came about as a result of incoming visible light interacting with noble metallic nanoparticles.Citation142 Specifically, light that corresponds with the electron oscillation frequency produces a localized surface plasmon resonance, which increases the electrical field at the particle surface.Citation143 Instead of being uniformly distributed across the plasmonic nanostructure, the produced electromagnetic field is typically limited to sharp edges and tiny interparticle gaps, creating plasmonic "hotspots." Moreover, the elevated electromagnetic field intensifies both excitation and emitted radiation, leading to the notable increase in SERS signals that are independent of analyte type. This phenomenon, known as electromagnetic SERS enhancement, is one of the two established theories for SERS signal amplification.Citation141 One of the challenges in SERS NPs detection might be optimizing the plasmonic hotspot for a strong SERS signal. It is commonly known that structures with sharp edges, are more effective at enhancing scattering strength. Therefore, employing optimally sized and shaped plasmonic nanoparticles to fabricate SERS substrates could be a great option.Citation144,Citation145
When utilizing SERS, it is essential to choose an appropriate laser power level. It is well known that, depending on the intensity of the laser, the temperature surrounding a hot spot can rise to much greater temperatures than 200 °C. This temperature is greater than most common polymers’ glass transition temperature. To overcome these challenges, it is required to optimize the procedure by regulating the laser’s power, analyte types, and excitation wavelength.Citation144
8.7. AFM-IR
AFM-IR, or AFM with infrared spectroscopy, is a relatively new method in the sciences that examines events at the nanoscale. In AFM-IR, an AFM scans the surface of the material to provide an image with a high spatial resolution of as low as 50 nm, whereas infrared spectroscopy elucidates the mechanical and thermal properties of MPs. For the detection of PE, PET, and polycaprolactone with a particle size of less than 500 μm, this approach has shown noteworthy success. With spatial resolution below the traditional optical diffraction limits, it is utilized for compositional mapping and chemical analysis. With remarkable precision and detail, this approach provides physiochemical characteristics. It enables further MPs and NPs characterization, including hydrophobicity and conductivity. The technique requires a sample pre-processing step to make presumed MPs accessible to AFM.
8.8. Mass spectroscopy
Using mass spectrometry in conjunction with gas and liquid chromatography, one can analyze MPs by examining the distinct products that are produced during the pyrolyzed or hydrolyzed of MPs. These mass approaches have considerable potential for MPs identification and quantification, especially NPs, due to their outstanding sensitivity.
8.8.1. Inductively coupled plasma mass spectroscopy
The development of isotope tagging technology resulted in the invention of single particle inductively coupled plasma mass spectroscopy (ICP-MS), a novel mass spectrometry method for measuring MPs. This innovative method has made it feasible to measure the size and quantity of nanoparticles. For metal-based nanoparticles in environmental materials, this technique is often employed.Citation146 For instance, at a comparatively low detection limit of 8.4 × 105 particles/L, ICP-MS has been used to evaluate the size and number concentration of the model Au-coated MPs (at submicrometer scale). Nevertheless, its utilization necessitated extensive sample preparation due to its reliance on an indirect evaluation of the Au coating.Citation147 It has been shown that ICP-MS can assess model MPs particle sizes and number concentrations by tracking 13 C during the process.Citation148
8.8.2. Pyrolysis gas chromatography–mass spectrometry (Pyr-GC–MS)
Mass spectrometry-based destructive analysis following the pyrolysis of suspected polymers is becoming more and more popular. This technique employs the thermal degradation of MPs in an inert atmosphere to reveal the structural composition of a macromolecule. Sample preparation for this approach is minimal, and direct injection is a possibility based on the environmental matrix. This method overcomes the problem of potential plastic underestimation because it is employed regardless of particle size and provides information on the mass of polymers in the samples.Citation149
When analyzing complex matrices, pretreatment procedures that include digestion or separation before injecting the material in the Pyr-GC-MS may be error-prone. As a result, the combination of pressurized liquid extraction (PLE) and other techniques has emerged as a potentially effective method to eradicate certain polymers (PVC, PMMA, PS, PE and PP) from intricate environmental samples. Because it can be difficult to prevent interference when working with environmental samples, double-shot pyrolysis in conjunction with PLE has also been employed.Citation150 In addition to polymer types, Pyr-GC-MS may identify plastic additives in samples from the first shot, when low-temperature thermally desorbs the interference. But the second shot contains the real polymer identification and quantification. It can provide an estimate of the mass of polymers in each sample rather than a particle count. Pyr-GC-MS, like other methods without optical interrogation, does not reveal the physical form of plastic waste.Citation151
8.8.3. Liquid chromatography–tandem mass spectrometry (LC–MS/MS)
Minimal quantities of polymers can be detected using LC-MS/MS. This a versatile instrument used in medicine, pharmacology and the assessment of environmental exposures to minute heat-labile, nonvolatile chemicals. Polycarbonate, PET and nylon-based MPs were successfully identified and quantitatively analyzed in sewage sludge using LC-MS/MS.Citation152,Citation153 This method can be detrimental since it necessitates the depolymerization of macromolecules before examination. The mass and quantity of monomers produced during depolymerization are disclosed by the detection method; however, the size, number, color, and shape of the MPs remain unknown. The method can also yield information about the size profile distribution when combined with size fractionation. Additionally, this method necessitates a substantial financial investment, knowledgeable operators, and meticulous sample preparation—which is usually vulnerable to experimental contamination.Citation154
8.8.4. Matrix-assisted laser desorption/ionization time-of-flight (MALDI-TOF) mass spectrometry
MALDI-TOF is a mass spectrometry technique that uses an ion source of MALDI and a detector of TOF as the mass analyzer. MPs investigations may examine this technique, which has been utilized since 1996 to detect whole bacterial cells without pretreatment. The device has proven to be effective in differentiating between different types of polymers. In conjunction with imaging techniques, it can also make it easier to examine the shape, size, color, and composition of MPs. More specifically, MALDI-TOF is a crucial tool for complex polymer characterization. Recently, PS and PET in complex biological materials have been identified and quantified using this mass spectrometric approach.Citation155 Perhaps one of the preferred analytical techniques is this one due to its wide mass range of detection.Citation156
8.9. Shrinking surface bubble deposition (SSBD)
The Shrinking Surface Bubble Deposition (SSBD) method is an innovative way to identify MPs in an array of environmental samples. The method concentrates the suspended particles in the water and deposits them on a substrate for observation and characterization by using plasmonic heating of mixed silver NPs with ambient water samples to produce a bubble through laser excitation. This method enables the direct visualization and identification of NPs in a range of water samples.Citation157
8.10. Flow cytometry
Flow cytometry is an extremely sensitive tool for detecting fluorescence signals and light scattering, which enables quick quantification of fluorescence-stained (NR, for example) MPs. It has been widely applied to the detection and analysis of MPs, such as molecules, cells, and microbes in liquid samples that are hydrodynamically concentrated into a stream so that they flow past incident light beams from one or more lasers one at a time. Sensitive photomultiplier tubes monitor parameters including light scatter and fluorescence, providing information about surface roughness/granularity (side scatter), size (forward scatter) and fluorescence from pigments or dye markers. A significant benefit of flow cytometry, aside from its sensitivity, is the elimination of human subjectivity because the method does not necessitate the visual interpretation of particles.Citation158,Citation159
8.11. Thermal analysis
Recently, the thermo-analytical method—which gauges changes in polymer physical and chemical characteristics based on their thermal stability—was put to the test for identifying MPs. The technique of differential scanning calorimetry (DSC) can be used to examine the thermal characteristics of polymeric materials. Each plastic product has unique DSC characteristics; hence the approach needs reference materials to distinguish between different polymer kinds. DSC can be helpful in locating particular principal MPs for which reference materials are accessible, like polyethylene microbeads. Polyvinyl chloride (PVC), polyamide (PA), polyester (PES), polyethylene terephthalate (PET), and polyurethane (PU) could not be detected by thermogravimetry (TGA) in combination with DSC because their phase transition signals overlaps. TGA combined the benefits of a larger sample size with TGA compared to pyrolysis (pyro)-GC-MS and higher resolution with GC-MS compared to DSC when paired with solid-phase extraction (SPE), which was then coupled with thermal desorption gas chromatography mass spectrometry (TDS-GC-MS). The TGA-SPE-TDS-GC-MS approach was used to quantitatively identify PE injected into soil and mussel samples. A polypropylene (PP), polystyrene (PS), and polymer combination were also examined using this method.Citation139
Thermal analysis, however, is a destructive technique that precludes the further study of the MP sample. Although DSC analysis is quick and relatively easy, it is not always successful in distinguishing MPs from other polymer products in environmental samples. Chemical additives in MPs can be simultaneously analyzed using thermal analysis and GC-MS. Bulk samples, and single particles, can be examined using GC-MS and thermal analysis, which gives weighted (w/w) concentration data for MPs. The bulk analysis does not, however, offer data on the quantity, size, and shape of the MPs that were analyzed. Contrary to FTIR and Raman spectroscopy, this method demands a skilled operator as well as significantly more time and effort for operating the instrument and data analysis. These techniques would be helpful for screening analyses of bulk samples or further corresponding investigations of MPs that have not yet been thoroughly characterized by spectroscopy, but it seems too soon to employ them for routine analysis of MPs from environmental samples.
8.12. Material-supported MPs/NPs detection
8.12.1. Nanopipettes
MPs have grown in importance during the previous 20 years, and numerous research have examined MPs/NPs in environmental sample data. Scientists have conducted extensive research on this subject and have developed a range of innovative analytical techniques for their detection.Citation160–162 It has recently been reported that the analytical community has several potential uses for nanopipettes, which are made by heating glass capillaries during the pull-out process. Nanopipettes became popular as a means of developing chemical and biological sensors and conducting ion transport investigations at the nanoscale.Citation163,Citation164
8.12.2. Microfluidic chips
To swiftly identify MPs in environmental samples, a unique analytical method still needs to be developed and optimised.Citation165–167 Micro-reservoirs leading to microfilters that are intended to capture all MPs particles in an ultra-compact region were used in the fabrication of the micro-optofluidic platform. To identify the type and nature of MPs present in this ultra-compact space, the researchers were able to successfully enable optical spectroscopy and imaging. Furthermore, passive size sorting was used to separate the MPs based on the size ranges in various reservoirs. The distributed sizes of the MPs samples were recovered using a reference technique that included flow cytometry; however, information regarding their chemical nature was lost in the process. In order to verify the possible use of this micro-optofluidic platform, model samples comprising standard MPs with varying sizes and chemical compositions were combined and effectively examined.Citation168
9. Global initiatives and control measures by international organisations, regional unions, and associations
Globally, there is increasing concern about regulating MPs and NPs, and several nations have responded to this concern by enacting different laws and regulations. An outline of a few of these policies is provided below (). In an effort to lessen the negative effects that MPs and NPs have on the environment and human health, these policies combine voluntary actions, regulatory strategies, and international cooperation. The efficacy of these interventions varies and frequently necessitates continual evaluation and accounting in response to novel scientific findings and technological advances.
Table 4. Different policy/strategies/initiatives adopted by the different national/international organisations, regional unions and associations.
10. Recommendations for future research
The pollution of terrestrial and marine ecosystems by MPs is widespread worldwide. Research on a number of significant sources and release channels for MPs has been compiled in this review. Significant MPs loadings are anticipated across a wide range of environmental situations, which suggests a larger yearly discharge than the figure for the marine environment. After intensive literature survey it was found that there are various gaps in management of MPs which present numerous opportunities for future research. The distribution, movement, and destiny of MPs particles are predicted to be influenced by a variety of dynamic processes in terrestrial and freshwater systems because of their enormous complexity. A series of precise guidelines for further research have been identified by this review, as:
10.1. Harmonic progression of approaches and reporting, with improved quality assurance and control (QA/QC) techniques, to assure adequate data quality and enable accurateness between datasets
The vast range of methodological approaches used in the review for the investigations of the presence of MPs in terrestrial and marine ecosystems, including samples of biota, sediment and water is notable. This includes differences in the methods used for sampling, treating samples, using analytical procedures, and analyzing different particle size classes. Additionally, it is impossible to evaluate the quality of given data because many studies do not use a comparable set of QA/QC methods. Findings are frequently presented in a variety of ways, such as by using various measurement units or by publishing simply summary statistics that vary as well (such as minimum/maximum, mean, or median). The inability of diverse research to be compared is the pinnacle of this variability. In addition to providing useful baselines that can monitor the influence of remediation or reduction measures, collaboration of analytical techniques and investigating formats and the publication of data in appropriate repositories will aid in reducing ambiguity in a comprehensive, global overview of the condition of contamination.
10.2. An exhaustive analysis of the fate, sources, and effects of MPs in agricultural environments
The confluence of numerous MPs particle sources and release channels can be seen in agricultural contexts. Although the extent and relevance of this are mostly unclear, particles may also be linked to greater chemical loads from plastic additives (mostly for reducing the photodegradation of mulching films) or sorbed contaminants (such as from wastewater treatment plants). It is challenging to evaluate the relative contributions of various sources under pertinent environmental conditions or the accumulation of particles over time because agricultural settings are relatively uncontaminated and the destiny of particles in agricultural soils is still poorly understood. The effects of MPs contamination in agricultural ecosystems have only recently been the subject of a tiny amount of research. To get a comprehensive understanding of the dangers presented by MPs over spatial and temporal domains, more research is necessary. This is especially crucial considering the possibility that any adverse consequences could affect the security of the food supply and the health of the land.
10.3. Assessment of road-associated MPs particles (RAMP) as a source of MP to the environment
There are significant awareness gaps regarding environmental loadings, transportation from the road to various matrices, and persistence in gully-pots and water treatment systems for MPs particles associated with the road. In order to precisely estimate the amount of these road-associated microparticles released into the environment, including the contributions from various road-related sources, more investigation is urgently required. This is relevant in view of the recent attention received by these road-associated microparticles in a number of analyses of the worldwide major sources of MPs in the environment. Through the use of a novel and improved method for analyzing these microparticles in environmental samples, quantification should be possible.
10.4. Measures and technologies to separate particles within waste water treatment plant system or to reduce MPs emissions to wastewater
The role of wastewater systems, including their plants and combined sewer overflows, as a release channel for MP particles into both terrestrial and marine environments, was underlined in this research. In order to lessen the load on these plants and restrict releases from untreated discharges like combined sewer overflows, attempts should be made to minimize the amount of MPs particles entering wastewater at the source. A large portion of the wastewater produced worldwide is released untreated and is not connected to a WWTP. The environmental emission of MPs in so many nations would be constrained by improvements in wastewater treatment capacity worldwide. Many MPs forms have sewage sludge application on land recognized as a key release mechanism.
10.5. A Better perspective of the controls sustaining the preservation and transport of MPs particles in aquatic systems, including more accurate flux estimates to the marine environment
From their release to their final destination, MPs particles in freshwater systems are expected to travel over a complicated course. Several processes that obstruct downstream transit may be included in this. To establish thresholds and controls on MPs transport in freshwater environments, these dynamics need to be studied further. The bulk of particles is predicted to eventually end up in the marine environment, via fluvial systems. In order to incorporate the necessary complexity and pinpoint the effective constraints on MP’s release to the marine environment, estimates for this flow must be based on process-based research. As well as identifying zones of MPs build-up in freshwater systems, further research on the dynamics of MPs movement and spatial patterns of contamination can also highlight the places most at risk from potential contamination-related negative effects. This will aid in concentrating efforts to safeguard freshwater environments.
10.6. Further study of the occurrence of MPs in terrestrial and aquatic organisms, with an explicit focus on particle types such as RAMP
The impact of MPs pollution on ecosystems and human health has prompted extensive ecotoxicological studies. However, there is a lack of evidence regarding the uptake of MPs in actual field settings. To address this, a more comprehensive analysis of particle absorption in actual environmental conditions is essential to provide a contextual understanding of ecotoxicological investigations and to enhance risk assessments. Exposure to MPs is a critical factor in evaluating risk, and their presence in the environment can have detrimental consequences for both ecosystems and human health. Furthermore, existing studies have primarily focused on the impact of individual materials, with limited consideration for mixtures of biodegradable MPs and MPs at concentration ratios that reflect real environmental circumstances. It is imperative for future studies to incorporate such mixtures to better understand the combined effects and interactions of different types of MPs. Moreover, the concentration of MPs used in many experiments exceeds those found in the actual environment. Therefore, the concept of "environmentally relevant concentration" has become a significant consideration in toxicity experiments. Additionally, the duration of exposure is a crucial factor, as the continuous exposure to MPs in natural environmental conditions and the slower depuration process compared to other particles can significantly influence the outcomes of ecotoxicological studies.
10.7. Assessment of environment sinks for MPs particles at spatial and temporal scales
This analysis identified a number of potential locations that, in terrestrial and freshwater settings, could serve as transient, long-term, or even permanent sinks for MPs particles. Initial research has looked at the accumulation of some of these particles and their possible residence times. However, study is needed to determine the spatial and temporal scales on which these environments act as stores, as well as the possibility that they could later become sources of MPs contamination through reworking and remobilization. This is required to develop more appropriate and better-targeted remediation procedures rather than relying on quick solutions and acquire a better long-term perspective on environmental damage.
11. Comprehensive strategies for mitigating MPs pollution in textile lifecycles
11.1. The design and production pathway
One strategy for reducing microfibre shedding has been suggested: switching textile patterns to use more natural fibers. Besides this, the increased release of microfibres may be caused by the manufacturing procedures used to create synthetic fibers, yarns, textiles, and goods. Particularly, an essential component in the development of MPs is the use of abrasive friction during manufacture. Microfibre release while using a product could be decreased by employing alternate production techniques or textile construction techniques.
11.2. The use and caretaking pathway
The requirement that all washing machines have a specific microfiber filter by January 2025 was first introduced in France in 2020. Utilizing a washing machine filter can cut the emission of microfibres by as much as 80%. The release of microfibers is also impacted by the utilization of detergent and fabric softener. By designing non-aggressive, liquid detergents that are efficient at low temperatures and don’t rinse off textile finishes, some of which guard against fiber breaking, detergent producers can help reduce the shedding of microfibers. Powder detergents shouldn’t be used on synthetic fabrics because they create friction and break fibers.
11.3. Change in consumer behavior
According to numerous studies, the first few washes of new clothing are when microfibre releases are particularly high. This indicates that fast fashion, in which clothes are worn briefly before being replaced frequently, is responsible for a significant portion of microfibre releases. Therefore, modifications in consumer purchasing habits are required, as well as understanding of the effect that new apparel has on MPs pollution. More regenerative business models, which encourage less consumption and longer use, could allow such a transformation. Reused items typically shed fewer microfibres when washed than new ones, which has the added benefit of reducing both the quantity of new purchases and trash generation. Moreover, preparing an old article for reuse requires fewer resources than the production of a new similar one, thus providing a further environmental benefit.
11.4. The disposal and end-of life processing pathway
In addition to promoting textile reuse and recycling, which would decrease MPs emissions during production and use, textile waste collection and end-of-life treatment can also reduce secondary MPs contamination by preventing littering, improperly managed waste, and textiles being blown by the wind from open landfills. Due to improper disposal, the dumping of trash from open landfills, or the washing of synthetic clothing in untreated waste water, there is a risk that microfibres will spread.
11.5. Increasing knowledge and awareness
To promote research aimed at filling these information gaps on the release, spread, and effects of NPs and MPs, continued public and corporate support is required. To solve the complex difficulties related to various primary MPs contamination from textiles, intensive interdisciplinary collaboration across technical, behavioral, and regulatory measures is required.
12. Conclusions
Concern over MPs in the environment has recently come under fire for being a distraction from other problems endangering the health of the global environment. The impact of plastics on the environment is undoubtedly complex, and innovative approaches are needed to find answers. The fact is that, the outlaw or replacement of plastics is nearly imposible, because of their beneficial qualities and our growing reliance on them. The concern is that both their improper management and expansion into the natural environment are growing, as is their global utilization. Over time, these plastics will break down into MPs and NPs. The effects of these on toxicology or the ecosystem are not fully understood at this time. To comprehend how organic chemical partition coefficients to plastics are changed in the presence of sediment and soil, studies on the dynamic interactions between plastic particles, plasticizer additives, and environmental pollutants also need to be increased. In order to comprehend the mechanisms governing the bioaccumulation of plasticizers and co-transported compounds, studies of chemical dynamics within the guts of organisms are also required. Further investigation into MPs is required to determine their global distribution and potential human health exposure. This requires additional field sampling and the application of standardized analytical techniques.
Acknowledgment
The authors declare that no funds, grants, or other support were received during the preparation of this manuscript.
Additional information
Funding
References
- Ritchie H, Roser M. Plastic Pollution. Our World Data.https://ourworldindata.org/plastic-pollution. 2018.
- Kiran BR, Kopperi H, Venkata Mohan S. Micro/nano-plastics occurrence, identification, risk analysis and mitigation: challenges and perspectives. Rev Environ Sci Biotechnol. 2022;21(1):169–203. doi:10.1007/s11157-021-09609-6.
- Napper IE, Thompson RC. Plastic debris in the marine environment: history and future challenges. Glob Challenges. 2020;4(6):1900081. doi:10.1002/gch2.201900081.
- Bashir SM, Kimiko S, Mak C-W, Fang JK-H, Gonçalves D. Personal care and cosmetic products as a potential source of environmental contamination by microplastics in a densely populated Asian city. Front Mar Sci. 2021; 8:1–11. doi:10.3389/fmars.2021.683482.
- Yang H, Dong H, Huang Y, Chen G, Wang J. Interactions of microplastics and main pollutants and environmental behavior in soils. Sci Total Environ. 2022;821:153511. doi:10.1016/j.scitotenv.2022.153511.
- Mdlalose L, Chimuka L. Mitigation approaches to prevent microplastics effects in the aquatic environment: exploration of microbeads from personal care and cosmetic products. Int J Environ Res. 2022;16(5):84. doi:10.1007/s41742-022-00464-z.
- Chatterjee S, Sharma S. Microplastics in our oceans and marine health. F Actions Sci Reports. 2019; Special Issue 19 :54–61.
- Li J, Song Y, Cai Y. Focus topics on microplastics in soil: analytical methods, occurrence, transport, and ecological risks. Environ. Pollut. 2020;257:113570. doi:10.1016/j.envpol.2019.113570.
- Dris R, Gasperi J, Mirande C, et al. A first overview of textile fibers, including microplastics, in indoor and outdoor environments. Environ. Pollut. 2017;221:453–458. doi:10.1016/j.envpol.2016.12.013.
- Zhang B, Yang X, Chen L, Chao J, Teng J, Wang Q. Microplastics in soils: a review of possible sources, analytical methods and ecological impacts. J Chem Tech Biotech. 2020;95(8):2052–2068. doi:10.1002/jctb.6334.
- Campanale C, Stock F, Massarelli C, et al. Microplastics and their possible sources: the example of Ofanto River in Southeast Italy. Environ Pollut. 2020;258:113284. doi:10.1016/j.envpol.2019.113284.
- Allen S, Allen D, Phoenix VR, et al. Atmospheric transport and deposition of microplastics in a remote mountain catchment. Nat Geosci. 2019;12(5):339–344. doi:10.1038/s41561-019-0335-5.
- Rochman CM. Microplastics research from sink to source. Science. 2018;360(6384):28–29. doi:10.1126/science.aar7734.
- Eerkes-Medrano D, Thompson RC, Aldridge DC. Microplastics in freshwater systems: a review of the emerging threats, identification of knowledge gaps and prioritisation of research needs. Water Res. 2015;75:63–82. doi:10.1016/j.watres.2015.02.012.
- Horton AA, Walton A, Spurgeon DJ, Lahive E, Svendsen C. Microplastics in freshwater and terrestrial environments: evaluating the current understanding to identify the knowledge gaps and future research priorities. Sci Total Environ. 2017;586:127–141. doi:10.1016/j.scitotenv.2017.01.190.
- Näkki P, Setälä O, Lehtiniemi M. Bioturbation transports secondary microplastics to deeper layers in soft marine sediments of the Northern Baltic Sea. Mar. Pollut. Bull. 2017;119(1):255–261. doi:10.1016/j.marpolbul.2017.03.065.
- Lassen C, Hansen SF, Magnusson K, et al. Microplastics occurrence, effects and sources of releases to the environment in Denmark. Denmark: Danish Environmental Protection Agency; 2015. Report No. 1793/2015: 1–205.
- Heilig ML. United States patent office. SIGGRAPH Comput Graph. 1994;28(2):131–134. doi:10.1145/178951.178972.
- Sundt P, Per-Erik Schultze FS. Sources of microplastic- pollution to the marine environment. Miljodirektoratet: Mepex Nor Environ Agency; 2014. Report No. M-321/2015: 86
- Essel R, Engel L, Carus M and Ahrens RH. Sources of microplastics relevant to marine protection in Germany. Germany: Federal Environment Agency; 2015. Texte 64/2015, Project No. 31969, Report No. (UBA-FB) 002146/E: 1–45.
- Eriksen M, Mason S, Wilson S, et al. Microplastic pollution in the surface waters of the Laurentian Great Lakes. Mar Pollut Bull. 2013;77(1–2):177–182. doi:10.1016/j.marpolbul.2013.10.007.
- Leslie HA. Review of microplastics in cosmetics. IVM Inst Environ Stud. 2014;476(July):33.
- Yurtsever M. Glitters as a source of primary microplastics: an approach to environmental responsibility and ethics. J Agric Environ Ethics. 2019;32(3):459–478. doi:10.1007/s10806-019-09785-0.
- Kibria MG, Masuk NI, Safayet R, Nguyen HQ, Mourshed M. Plastic waste: challenges and opportunities to mitigate pollution and effective management. Int J Environ Res. 2023;17(1):20. doi:10.1007/s41742-023-00507-z.
- Whitacre DM, ed. Reviews of Environmental Contamination and Toxicology, USA: Springer Cham, 2014; Vol. 227.
- Rillig MC. Microplastic in terrestrial ecosystems and the soil? Environ Sci Technol. 2012;46(12):6453–6454. doi:10.1021/es302011r.
- Luo Y, Awoyemi OS, Naidu R, Fang C. Detection of Microplastics and Nanoplastics Released from a Kitchen Blender Using Raman Imaging. J. Hazard. Mater. 2023, 453, 131403: 1-9. doi: 10.1016/j.jhazmat.2023.131403.
- Wang T, Li B, Zou X, et al. Emission of primary microplastics in mainland China: invisible but not negligible. Water Res. 2019;162:214–224. doi:10.1016/j.watres.2019.06.042.
- van Wezel A, Caris I, Kools SAE. Release of primary microplastics from consumer products to wastewater in the Netherlands. Environ Toxicol Chem. 2016;35(7):1627–1631. doi:10.1002/etc.3316.
- Xia B, Sui Q, Du Y, et al. Secondary PVC microplastics are more toxic than primary PVC microplastics to Oryzias melastigma embryos. J Hazard Mater. 2022;424:127421. doi:10.1016/j.jhazmat.2021.127421.
- Magnusson K, Norén F. Screening of microplastic particles in and down-stream a wastewater treatment plant. Stockholm, Sweden: IVL Swedish Environmental Research Institute; 2014. Report C55.
- Talvitie J, Heinonen M, Pääkkönen J-P, et al. Do wastewater treatment plants act as a potential point source of microplastics? Preliminary study in the Coastal Gulf of Finland, Baltic Sea. Water Sci Technol J Int Assoc Water Pollut Res. 2015;72(9):1495–1504. doi:10.2166/wst.2015.360.
- Murphy F, Ewins C, Carbonnier F, Quinn B. Wastewater treatment works (WwTW) as a source of microplastics in the aquatic environment. Environ Sci Technol. 2016;50(11):5800–5808. doi:10.1021/acs.est.5b05416.
- Mintenig SM, Int-Veen I, Löder MGJ, Primpke S, Gerdts G. Identification of microplastic in effluents of waste water treatment plants using focal plane array-based micro-Fourier-transform infrared imaging. Water Res. 2017;108:365–372. doi:10.1016/j.watres.2016.11.015.
- Ziajahromi S, Neale PA, Rintoul L, Leusch FDL. Wastewater treatment plants as a pathway for microplastics: development of a new approach to sample wastewater-based microplastics. Water Res. 2017;112:93–99. doi:10.1016/j.watres.2017.01.042.
- Gies EA, LeNoble JL, Noël M, et al. Retention of microplastics in a major secondary wastewater treatment plant in Vancouver, Canada. Mar Pollut Bull. 2018;133:553–561. doi:10.1016/j.marpolbul.2018.06.006.
- Gündoğdu S, Çevik C, Güzel E, Kilercioğlu S. Microplastics in municipal wastewater treatment plants in Turkey: a comparison of the influent and secondary effluent concentrations. Environ Monit Assess. 2018;190(11):626. doi:10.1007/s10661-018-7010-y.
- Conley K, Clum A, Deepe J, Lane H, Beckingham B. Wastewater treatment plants as a source of microplastics to an urban estuary: removal efficiencies and loading per capita over one year. Water Res. X. 2019;3:100030. doi:10.1016/j.wroa.2019.100030.
- Lv X, Dong Q, Zuo Z, Liu Y, Huang X, Wu W-M. Microplastics in a municipal wastewater treatment plant: fate, dynamic distribution, removal efficiencies, and control strategies. J Clean Prod. 2019;225:579–586. doi:10.1016/j.jclepro.2019.03.321.
- Magni S, Binelli A, Pittura L, et al. The fate of microplastics in an Italian wastewater treatment plant. Sci. Total Environ. 2019;652:602–610. doi:10.1016/j.scitotenv.2018.10.269.
- Leslie HA, Brandsma SH, van Velzen MJM, Vethaak AD. Microplastics en route: field measurements in the Dutch river delta and Amsterdam canals, wastewater treatment plants, North Sea sediments and biota. Environ Int. 2017;101:133–142. doi:10.1016/j.envint.2017.01.018.
- Iyare PU, Ouki SK, Bond T. Microplastics removal in wastewater treatment plants: a critical review. Environ Sci Water Res Technol. 2020;6(10):2664–2675. doi:10.1039/D0EW00397B.
- Dris R, Gasperi J, Rocher V, Saad M, Renault N, Tassin B. Microplastic contamination in an urban area: a case study in Greater Paris. Environ Chem. 2015;12(5):592–599. doi:10.1071/EN14167.
- Lee H, Kim Y. Treatment characteristics of microplastics at biological sewage treatment facilities in Korea. Mar Pollut Bull. 2018;137:1–8. doi:10.1016/j.marpolbul.2018.09.050.
- Simon M, van Alst N, Vollertsen J. Quantification of microplastic mass and removal rates at wastewater treatment plants applying focal plane array (FPA)-based Fourier transform infrared (FT-IR) imaging. Water Res. 2018;142:1–9. doi:10.1016/j.watres.2018.05.019.
- Wisniowska E, Moraczewska-Majkut K, Nocon W. Efficiency of microplastics removal in selected wastewater treatment plants – preliminary studies. DWT. 2018;134:316–323. doi:10.5004/dwt.2018.23418.
- Kleinschmidt JM, Janosik AM. Microplastics in Florida, United States: a case study of quantification and characterization with intertidal snails. Front Ecol Evol. 2021;9:1–12. doi:10.3389/fevo.2021.645727.
- Sobhani Z, Luo Y, Gibson CT, et al. Collecting microplastics in gardens: case study (i) of soil. Front Environ Sci. 2021;9:1–11. doi:10.3389/fenvs.2021.739775.
- Ragusa A, Notarstefano V, Svelato A, et al. Raman microspectroscopy detection and characterisation of microplastics in human breastmilk. Polymers. 2022;14(13):1–14. doi:10.3390/polym14132700.
- Kılıç E, Yücel N, Şahutoğlu SM. Microplastic composition, load and removal efficiency from wastewater treatment plants discharging into Orontes River. Int J Environ Res. 2023;17(2):25. doi:10.1007/s41742-023-00514-0.
- Yang L, Zhang Y, Kang S, Wang Z, Wu C. Microplastics in soil: a review on methods, occurrence, sources, and potential risk. Sci Total Environ. 2021;780:146546. doi:10.1016/j.scitotenv.2021.146546.
- Murray A, Örmeci B. Removal effectiveness of nanoplastics (<400 Nm) with separation processes used for water and wastewater treatment. Water. 2020;12(3):635. doi:10.3390/w12030635.
- Wright SL, Ulke J, Font A, Chan KLA, Kelly FJ. Atmospheric microplastic deposition in an urban environment and an evaluation of transport. Environ Int. 2020;136:105411. doi:10.1016/j.envint.2019.105411.
- Zhang Y, Kang S, Allen S, Allen D, Gao T, Sillanpää M. Atmospheric microplastics: a review on current status and perspectives. Earth-Science Rev. 2020;203:103118. doi:10.1016/j.earscirev.2020.103118.
- Allen S, Allen D, Moss K, Le Roux G, Phoenix VR, Sonke JE. Examination of the ocean as a source for atmospheric microplastics. PLOS One. 2020;15(5):e0232746. doi:10.1371/journal.pone.0232746.
- Botterell ZLR, Beaumont N, Dorrington T, Steinke M, Thompson RC, Lindeque PK. Bioavailability and effects of microplastics on marine zooplankton: a review. Environ Pollut. 2019;245:98–110. doi:10.1016/j.envpol.2018.10.065.
- Lambert S, Scherer C, Wagner M. Ecotoxicity testing of microplastics: considering the heterogeneity of physicochemical properties. Integr Environ Assess Manag. 2017;13(3):470–475. doi:10.1002/ieam.1901.
- Gao H, Yan C, Liu Q, Ding W, Chen B, Li Z. Effects of plastic mulching and plastic residue on agricultural production: a meta-analysis. Sci Total Environ. 2019;651(Pt 1):484–492. doi:10.1016/j.scitotenv.2018.09.105.
- Schwinghammer L, Krause S, Schaum C. Determination of large microplastics: wet-sieving of dewatered digested sludge, co-substrates, and compost. Water Sci Technol. 2020;84(2):384–392. doi:10.2166/wst.2020.582.
- McGivney E, Cederholm L, Barth A, et al. Rapid physicochemical changes in microplastic induced by biofilm formation. Front Bioeng Biotechnol. 2020;8:1–14. doi:10.3389/fbioe.2020.00205.
- Gandara E Silva PP, Nobre CR, Resaffe P, Pereira CDS, Gusmão F. Leachate from microplastics impairs larval development in brown mussels. Water Res. 2016;106:364–370. doi:10.1016/j.watres.2016.10.016.
- O’Connor IA, Golsteijn L, Hendriks AJ. Review of the partitioning of chemicals into different plastics: consequences for the risk assessment of marine plastic debris. Mar Pollut Bull. 2016;113(1–2):17–24. doi:10.1016/j.marpolbul.2016.07.021.
- Hale RC, Seeley ME, La Guardia MJ, Mai L, Zeng EY. A global perspective on microplastics. JGR Oceans. 2020;125(1):1–40. doi:10.1029/2018JC014719.
- Dobslaw D, Woiski C, Kiel M, Kuch B, Breuer J. Plant uptake, translocation and metabolism of PBDEs in plants of food and feed industry: a review. Rev Environ Sci Biotechnol. 2021;20(1):75–142. doi:10.1007/s11157-020-09557-7.
- Engwa GA, Ferdinand PU, Nwalo FN, Unachukwu MN. In: Karcioglu O, Arslan B, eds. Mechanism and Health Effects of Heavy Metal Toxicity in Humans. Rijeka: IntechOpen; 2019: 1–13.
- Verla AW, Enyoh CE, Verla EN, Nwarnorh KO. Microplastic–toxic chemical interaction: a review study on quantified levels, mechanism and implication. SN Appl Sci. 2019;1(11):1400. doi:10.1007/s42452-019-1352-0.
- Amelia TSM, Khalik WMAWM, Ong MC, Shao YT, Pan H-J, Bhubalan K. Marine microplastics as vectors of major ocean pollutants and its hazards to the marine ecosystem and humans. Prog Earth Planet Sci. 2021;8(1):12. doi:10.1186/s40645-020-00405-4.
- Ma H, Pu S, Liu S, Bai Y, Mandal S, Xing B. Microplastics in aquatic environments: toxicity to trigger ecological consequences. Environ Pollut. 2020;261:114089. doi:10.1016/j.envpol.2020.114089.
- Li J, Zhang K, Zhang H. Adsorption of antibiotics on microplastics. Environ Pollut. 2018;237:460–467. doi:10.1016/j.envpol.2018.02.050.
- Rahman A, Sarkar A, Yadav OP, Achari G, Slobodnik J. Potential human health risks due to environmental exposure to nano- and microplastics and knowledge gaps: a scoping review. Sci Total Environ. 2021;757:143872. doi:10.1016/j.scitotenv.2020.143872.
- Carbery M, O’Connor W, Palanisami T. Trophic transfer of microplastics and mixed contaminants in the marine food web and implications for human health. Environ Int. 2018;115:400–409. doi:10.1016/j.envint.2018.03.007.
- Lehner R, Weder C, Petri-Fink A, Rothen-Rutishauser B. Emergence of nanoplastic in the environment and possible impact on human health. Environ Sci Technol. 2019;53(4):1748–1765. doi:10.1021/acs.est.8b05512.
- Mason SA, Welch VG, Neratko J. Synthetic polymer contamination in bottled water. Front Chem. 2018;6:1–11. doi:10.3389/fchem.2018.00407.
- Ge H, Yan Y, Wu D, Huang Y, Tian F. Potential Role of LINC00996 in Colorectal Cancer: A Study Based on Data Mining and Bioinformatics. Onco. Targets. Ther. 2018;11:4845–4855. doi:10.2147/OTT.S173225.
- Yee MS-L, Hii L-W, Looi CK, et al. Impact of microplastics and nanoplastics on human health. Nanomater. 2021;11(2):1–22. doi:10.3390/nano11020496.
- Stapleton PA. Toxicological considerations of nano-sized plastics. AIMS Environ Sci. 2019;6(5):367–378. doi:10.3934/environsci.2019.5.367.
- Hernandez LM, Yousefi N, Tufenkji N. Are there nanoplastics in your personal care products? Environ Sci Technol Lett. 2017;4(7):280–285. doi:10.1021/acs.estlett.7b00187.
- Dawson A, Huston W, Kawaguchi S, et al. Uptake and depuration kinetics influence microplastic bioaccumulation and toxicity in Antarctic Krill (Euphausia Superba). Environ Sci. Technol. 2018;52(5):3195–3201. doi:10.1021/acs.est.7b05759.
- Mitrano DM, Wick P, Nowack B. Placing nanoplastics in the context of global plastic pollution. Nat Nanotechnol. 2021;16(5):491–500. doi:10.1038/s41565-021-00888-2.
- Clark NJ, Khan FR, Mitrano DM, Boyle D, Thompson RC. Demonstrating the translocation of nanoplastics across the fish intestine using palladium-doped polystyrene in a salmon gut-sac. Environ. Int. 2022;159:106994. doi:10.1016/j.envint.2021.106994.
- McIlwraith HK, Kim J, Helm P, Bhavsar SP, Metzger JS, Rochman CM. Evidence of microplastic translocation in wild-caught fish and implications for microplastic accumulation dynamics in food webs. Environ Sci Technol. 2021;55(18):12372–12382. doi:10.1021/acs.est.1c02922.
- So MWK, Vorsatz LD, Cannicci S, Not C. Fate of plastic in the environment: from macro to nano by macrofauna. Environ Pollut. 2022;300:118920. doi:10.1016/j.envpol.2022.118920.
- Fournier SB, D’Errico JN, Adler DS, et al. Nanopolystyrene translocation and fetal deposition after acute lung exposure during late-stage pregnancy. Part Fibre Toxicol. 2020;17(1):55. doi:10.1186/s12989-020-00385-9.
- Hu L, Chernick M, Lewis AM, Ferguson PL, Hinton DE. Chronic microfiber exposure in adult Japanese Medaka (Oryzias Latipes). PLOS One. 2020;15(3):e0229962. doi:10.1371/journal.pone.0229962.
- Hu L, Zhou Y, Wang Y, Zhang D, Pan X. Transfer of micro(nano)plastics in animals: a mini-review and future research recommendation. J Hazard Mater Adv. 2022;7:100101. doi:10.1016/j.hazadv.2022.100101.
- Driedger AGJ, Dürr HH, Mitchell K, Van Cappellen P. Plastic debris in the Laurentian Great Lakes: a review. J Great Lakes Res. 2015;41(1):9–19. doi:10.1016/j.jglr.2014.12.020.
- Long M, Moriceau B, Gallinari M, et al. Interactions between microplastics and phytoplankton aggregates : impact on their respective fates. Mar Chem. 2015;175:39–46. doi:10.1016/j.marchem.2015.04.003.
- Musa IO, Auta HS, Ilyasu US, et al. Micro- and nanoplastics in environment: degradation, detection, and ecological impact. Int J Environ Res. 2023;18(1):1. doi:10.1007/s41742-023-00551-9.
- Zantis LJ, Carroll EL, Nelms SE, Bosker T. Marine mammals and microplastics: a systematic review and call for standardisation. Environ Pollut. 2021;269:116142. doi:10.1016/j.envpol.2020.116142.
- Lu L, Luo T, Zhao Y, Cai C, Fu Z, Jin Y. Interaction between microplastics and microorganism as well as gut microbiota: a consideration on environmental animal and human health. Sci Total Environ. 2019;667:94–100. doi:10.1016/j.scitotenv.2019.02.380.
- Cole M, Lindeque P, Fileman E, et al. Microplastic ingestion by zooplankton. Environ. Sci. Technol. 2013;47(12):6646–6655. doi:10.1021/es400663f.
- He M, Yan M, Chen X, et al. Bioavailability and toxicity of microplastics to zooplankton. Gondwana Res. 2022;108:120–126. doi:10.1016/j.gr.2021.07.021.
- EEA. Plastic in textiles : towards a circular economy for synthetic textiles in Europe. https://www.eea.europa.eu/publications/plastic-in-textiles-towards-a. European Environment Agency| Briefing No 25/2020. Published Month June 29, 2021
- Romera-Castillo C, Pinto M, Langer TM, Álvarez-Salgado XA, Herndl GJ. Dissolved organic carbon leaching from plastics stimulates microbial activity in the ocean. Nat Commun. 2018;9(1):1430. doi:10.1038/s41467-018-03798-5.
- Miao L, Wang P, Hou J, et al. Distinct community structure and microbial functions of biofilms colonizing microplastics. Sci Total Environ. 2019;650(Pt 2):2395–2402. doi:10.1016/j.scitotenv.2018.09.378.
- McCormick AR, Hoellein TJ, London MG, Hittie J, Scott JW, Kelly JJ. Microplastic in surface waters of urban rivers: concentration, sources, and associated bacterial assemblages. Ecosphere. 2016;7(11):e01556. doi:10.1002/ecs2.1556.
- Gong M, Yang G, Zhuang L, Zeng EY. Microbial biofilm formation and community structure on low-density polyethylene microparticles in lake water microcosms. Environ Pollut. 2019;252(Pt A):94–102. doi:10.1016/j.envpol.2019.05.090.
- Smith M, Love DC, Rochman CM, Neff RA. Microplastics in seafood and the implications for human health. Curr Environ Heal Reports. 2018;5(3):375–386. doi:10.1007/s40572-018-0206-z.
- Hitchcock JN. Microplastics can alter phytoplankton community composition. Sci Total Environ. 2022;819(January):153074. doi:10.1016/j.scitotenv.2022.153074.
- Mao Y, Ai H, Chen Y, et al. Phytoplankton response to polystyrene microplastics: perspective from an entire growth period. Chemosphere. 2018;208:59–68. doi:10.1016/j.chemosphere.2018.05.170.
- Senavirathna MDHJ, Zhaozhi L, Fujino T. Root adsorption of microplastic particles affects the submerged freshwater macrophyte Egeria Densa. Water Air Soil Pollut. 2022;233(3):80. doi:10.1007/s11270-022-05556-2.
- Zhang C, Jian M-F, Chen Y-M[, et al. Effects of polystyrene microplastics (PS-MPs) on the growth, physiology, and biochemical characteristics of Hydrilla verticillata. Ying Yong Sheng Tai Xue Bao [J Appl Ecol]. 2021;32(1):317–325. doi:10.13287/j.1001-9332.202101.037.
- Klein M, Fischer EK. Microplastic abundance in atmospheric deposition within the metropolitan area of Hamburg, Germany. Sci Total Environ. 2019;685:96–103. doi:10.1016/j.scitotenv.2019.05.405.
- Baensch-Baltruschat B, Kocher B, Stock F, Reifferscheid G. Tyre and road wear particles (TRWP) – a review of generation, properties, emissions, human health risk, ecotoxicity, and fate in the environment. Sci Total Environ. 2020;733:137823. doi:10.1016/j.scitotenv.2020.137823.
- SAPEA, Science Advice for Policy By European Academies. A Scientific Perspective on Microplastics in Nature and Society. Berlin: SAPEA; 2019; Evidence Review Report No. 4: 1-173. doi: 10.26356/microplastics.
- Henry B, Laitala K, Klepp IG. Microfibres from apparel and home textiles: prospects for including microplastics in environmental sustainability assessment. Sci Total Environ. 2019;652:483–494. doi:10.1016/j.scitotenv.2018.10.166.
- Gong J, Xie P. Research progress in sources, analytical methods, eco-environmental effects, and control measures of microplastics. Chemosphere. 2020;254:126790. doi:10.1016/j.chemosphere.2020.126790.
- de Souza Machado AA, Lau CW, Till J, et al. Impacts of microplastics on the soil biophysical environment. Environ Sci Technol. 2018;52(17):9656–9665. doi:10.1021/acs.est.8b02212.
- de Souza Machado AA, Lau CW, Kloas W, et al. Microplastics can change soil properties and affect plant performance. Environ Sci Technol. 2019;53(10):6044–6052. doi:10.1021/acs.est.9b01339.
- Wang J, Huang M, Wang Q, Sun Y, Zhao Y, Huang Y. LDPE microplastics significantly alter the temporal turnover of soil microbial communities. Sci Total Environ. 2020;726:138682. doi:10.1016/j.scitotenv.2020.138682.
- Rillig MC, de Souza Machado AA, Lehmann A, Klümper U. Evolutionary implications of microplastics for soil biota. Environ Chem. 2019;16(1):3–7. doi:10.1071/EN18118.
- Zhang Y, Zhang X, Li X, He D. Interaction of microplastics and soil animals in agricultural ecosystems. Curr Opin Environ Sci Heal. 2022;26:100327. doi:10.1016/j.coesh.2022.100327.
- Ding J, Liu C, Chen Q, et al. Extractable additives in microplastics: a hidden threat to soil fauna. Environ Pollut. 2022;294:118647. doi:10.1016/j.envpol.2021.118647.
- Liu K, Wang X, Song Z, Wei N, Li D. Terrestrial plants as a potential temporary sink of atmospheric microplastics during transport. Sci Total Environ. 2020;742:140523. doi:10.1016/j.scitotenv.2020.140523.
- Luo X, Bing H, Luo Z, Wang Y, Jin L. Impacts of atmospheric particulate matter pollution on environmental biogeochemistry of trace metals in soil-plant system: a review. Environ Pollut. 2019;255:113138. doi:10.1016/j.envpol.2019.113138.
- Lian J, Liu W, Meng L, et al. Foliar-applied polystyrene nanoplastics (PSNPs) reduce the growth and nutritional quality of lettuce (Lactuca Sativa L.). Environ Pollut. 2021;280:116978. doi:10.1016/j.envpol.2021.116978.
- Meng F, Yang X, Riksen M, Geissen V. Effect of different polymers of microplastics on soil organic carbon and nitrogen – a mesocosm experiment. Environ Res. 2022;204:111938. doi:10.1016/j.envres.2021.111938.
- Huang D, Wang X, Yin L, et al. Research progress of microplastics in soil-plant system: ecological effects and potential risks. Sci Total Environ. 2022;812:151487. doi:10.1016/j.scitotenv.2021.151487.
- Wang Y, Huang J, Zhu F, Zhou S. Airborne microplastics: a review on the occurrence, migration and risks to humans. Bull Environ Contam Toxicol. 2021;107(4):657–664. doi:10.1007/s00128-021-03180-0.
- Vianello A, Jensen RL, Liu L, Vollertsen J. Simulating human exposure to indoor airborne microplastics using a breathing thermal manikin. Sci Rep. 2019;9(1):8670. doi:10.1038/s41598-019-45054-w.
- Alderete TL, Jones RB, Chen Z, et al. Exposure to traffic-related air pollution and the composition of the gut microbiota in overweight and obese adolescents. Environ Res. 2018;161:472–478. doi:10.1016/j.envres.2017.11.046.
- Soltani NS, Taylor MP, Wilson SP. Quantification and exposure assessment of microplastics in Australian indoor house dust. Environ Pollut. 2021;283:117064. doi:10.1016/j.envpol.2021.117064.
- Shi W, Cao Y, Chai X, et al. Potential health risks of the interaction of microplastics and lung surfactant. J Hazard Mater. 2022;429:128109. doi:10.1016/j.jhazmat.2021.128109.
- Goodman KE, Hare JT, Khamis ZI, Hua T, Sang Q-XA. Exposure of human lung cells to polystyrene microplastics significantly retards cell proliferation and triggers morphological changes. Chem Res Toxicol. 2021;34(4):1069–1081. doi:10.1021/acs.chemrestox.0c00486.
- Xu M, Halimu G, Zhang Q, et al. Internalization and toxicity: a preliminary study of effects of nanoplastic particles on human lung epithelial cell. Sci Total Environ. 2019;694:133794. doi:10.1016/j.scitotenv.2019.133794.
- Dong C-D, Chen C-W, Chen Y-C, Chen H-H, Lee J-S, Lin C-H. Polystyrene microplastic particles: in vitro pulmonary toxicity assessment. J Hazard Mater. 2020;385:121575. doi:10.1016/j.jhazmat.2019.121575.
- Zhang J, Wang L, Trasande L, Kannan K. Occurrence of polyethylene terephthalate and polycarbonate microplastics in infant and adult feces. Environ Sci Technol Lett. 2021;8(11):989–994. doi:10.1021/acs.estlett.1c00559.
- Fackelmann G, Sommer S. Microplastics and the gut microbiome: how chronically exposed species may suffer from gut dysbiosis. Mar Pollut Bull. 2019;143:193–203. doi:10.1016/j.marpolbul.2019.04.030.
- Stock V, Fahrenson C, Thuenemann A, et al. Impact of artificial digestion on the sizes and shapes of microplastic particles. Food Chem Toxicol. 2020;135:111010. doi:10.1016/j.fct.2019.111010.
- Huang W, Yin H, Yang Y, Jin L, Lu G, Dang Z. Influence of the co-exposure of microplastics and tetrabromobisphenol A on human gut: simulation in vitro with human cell Caco-2 and gut microbiota. Sci Total Environ. 2021;778:146264. doi:10.1016/j.scitotenv.2021.146264.
- Stock V, Böhmert L, Lisicki E, et al. Uptake and effects of orally ingested polystyrene microplastic particles in vitro and in vivo. Arch Toxicol. 2019;93(7):1817–1833. doi:10.1007/s00204-019-02478-7.
- Deng Y, Zhang Y, Lemos B, Ren H. Tissue accumulation of microplastics in mice and biomarker responses suggest widespread health risks of exposure. Sci Rep. 2017;7(1):46687. doi:10.1038/srep46687.
- Ibrahim YS, Tuan Anuar S, Azmi AA, et al. Detection of microplastics in human colectomy specimens. JGH Open. 2021;5(1):116–121. doi:10.1002/jgh3.12457.
- Tan M, Sun Y, Gui J, Wang J, Chen X, Wu D. The efficiency of different digestion and separation methods for extracting microplastics in typical organic solid waste. Int J Environ Res. 2022;16(2):16. doi:10.1007/s41742-022-00394-w.
- Heo NW, Hong SH, Han GM, et al. Distribution of small plastic debris in cross-section and high strandline on Heungnam Beach, South Korea. Ocean Sci J. 2013;48(2):225–233. doi:10.1007/s12601-013-0019-9.
- Hidalgo-Ruz V, Thiel M. Distribution and abundance of small plastic debris on beaches in the SE Pacific (Chile): a study supported by a citizen science project. Mar Environ Res. 2013;87–88:12–18. doi:10.1016/j.marenvres.2013.02.015.
- Desforges J-PW, Galbraith M, Dangerfield N, Ross PS. Widespread distribution of microplastics in subsurface seawater in the NE Pacific Ocean. Mar. Pollut. Bull. 2014;79(1–2):94–99. doi:10.1016/j.marpolbul.2013.12.035.
- Song YK, Hong SH, Jang M, et al. A comparison of microscopic and spectroscopic identification methods for analysis of microplastics in environmental samples. Mar Pollut Bull. 2015;93(1–2):202–209. doi:10.1016/j.marpolbul.2015.01.015.
- Shim WJ, Hong SH, Eo SE. Identification methods in microplastic analysis: a review. Anal Methods. 2017;9(9):1384–1391. doi:10.1039/C6AY02558G.
- Song YK, Hong SH, Jang M, et al. Large accumulation of micro-sized synthetic polymer particles in the sea surface microlayer. Environ. Sci. Technol. 2014;48(16):9014–9021. doi:10.1021/es501757s.
- Han XX, Rodriguez RS, Haynes CL, Ozaki Y, Zhao B. Surface-enhanced raman spectroscopy. Nat Rev Methods Prim. 2022;1(1):87. doi:10.1038/s43586-021-00083-6.
- Mogha NK, Shin D. Nanoplastic detection with surface enhanced Raman spectroscopy: present and future. TrAC Trends Anal Chem. 2023;158:116885. doi:10.1016/j.trac.2022.116885.
- Li P, Long F, Chen W, Chen J, Chu PK, Wang H. Fundamentals and applications of surface-enhanced raman spectroscopy–based biosensors. Curr Opin Biomed Eng. 2020;13:51–59. doi:10.1016/j.cobme.2019.08.008.
- Mandal P, Tewari BS. Progress in surface enhanced Raman scattering molecular sensing: a review. Surf Interf. 2022;28:101655. doi:10.1016/j.surfin.2021.101655.
- Yılmaz D, Günaydın BN, Yüce M. Nanotechnology in food and water security: on-site detection of agricultural pollutants through surface-enhanced Raman spectroscopy. Emergent Mater. 2022;5(1):105–132. doi:10.1007/s42247-022-00376-w.
- Jiménez-Lamana J, Marigliano L, Allouche J, Grassl B, Szpunar J, Reynaud S. A novel strategy for the detection and quantification of nanoplastics by single particle inductively coupled plasma mass spectrometry (ICP-MS). Anal Chem. 2020;92(17):11664–11672. doi:10.1021/acs.analchem.0c01536.
- Lai Y, Dong L, Li Q, et al. Counting nanoplastics in environmental waters by single particle inductively coupled plasma mass spectroscopy after cloud-point extraction and in situ labeling of gold nanoparticles. Environ Sci Technol. 2021;55(8):4783–4791. doi:10.1021/acs.est.0c06839.
- Laborda F, Trujillo C, Lobinski R. Analysis of microplastics in consumer products by single particle-inductively coupled plasma mass spectrometry using the carbon-13 isotope. Talanta. 2021;221:121486. doi:10.1016/j.talanta.2020.121486.
- Randhawa JS. Advanced analytical techniques for microplastics in the environment: a review. Bull Natl Res Cent. 2023;47(1):174. doi:10.1186/s42269-023-01148-0.
- Okoffo ED, Ribeiro F, O’Brien JW, et al. Identification and quantification of selected plastics in biosolids by pressurized liquid extraction combined with double-shot pyrolysis gas chromatography–mass spectrometry. Sci Total Environ. 2020;715:136924. doi:10.1016/j.scitotenv.2020.136924.
- Blomberg J. The Mechanism Behind Internal Injector Deposits [Thesis] Stockholm, Sweden: KTH Royal Institute of Technology; 2023
- Peng C, Tang X, Gong X, Dai Y, Sun H, Wang L. Development and application of a mass spectrometry method for quantifying nylon microplastics in environment. Anal. Chem. 2020;92(20):13930–13935. doi:10.1021/acs.analchem.0c02801.
- Zhang J, Wang L, Kannan K. Quantitative analysis of polyethylene terephthalate and polycarbonate microplastics in sediment collected from South Korea, Japan and the USA. Chemosphere. 2021;279:130551. doi:10.1016/j.chemosphere.2021.130551.
- Ng KT, Rapp-Wright H, Egli M, et al. High-throughput multi-residue quantification of contaminants of emerging concern in wastewaters enabled using direct injection liquid chromatography-tandem mass spectrometry. J Hazard Mater. 2020;398:122933. doi:10.1016/j.jhazmat.2020.122933.
- Lin Y, Huang X, Liu Q, Lin Z, Jiang G. Thermal fragmentation enhanced identification and quantification of polystyrene micro/nanoplastics in complex media. Talanta. 2020;208:120478. doi:10.1016/j.talanta.2019.120478.
- Adhikari S, Kelkar V, Kumar R, Halden RU. Methods and challenges in the detection of microplastics and nanoplastics: a mini-review. Polym Int. 2022;71(5):543–551. doi:10.1002/pi.6348.
- Moon S, Martin LMA, Kim S, et al. Direct observation and identification of nanoplastics in ocean water. Sci Adv. 2024;10(4):eadh1675. doi:10.1126/sciadv.adh1675.
- Tse Y-T, Lo H-S, Chan SM, Sze ET. Flow cytometry as a rapid alternative to quantify small microplastics in environmental water samples. Water. 2022;14(9):1436. doi:10.3390/w14091436.
- Kaile N, Lindivat M, Elio J, Thuestad G, Crowley QG, Hoell IA. Preliminary results from detection of microplastics in liquid samples using flow cytometry. Front Mar Sci. 2020;7: 1–12. doi:10.3389/fmars.2020.552688.
- Pérez-Guevara F, Roy PD, Kutralam-Muniasamy G, Shruti VC. A central role for fecal matter in the transport of microplastics: an updated analysis of new findings and persisting questions. J Hazard Mater Adv. 2021;4:100021. doi:10.1016/j.hazadv.2021.100021.
- Mansa R, Zou S. Thermogravimetric analysis of microplastics: a mini review. Environ Adv. 2021;5:100117. doi:10.1016/j.envadv.2021.100117.
- Rai PK, Kumar V, Sonne C, Lee SS, Brown RJC, Kim K-H. Progress, prospects, and challenges in standardization of sampling and analysis of micro- and nano-plastics in the environment. J Clean Prod. 2021;325:129321. doi:10.1016/j.jclepro.2021.129321.
- Ho VTTX, Park H, An S, et al. Coumarin–lipoic acid conjugates on silver nanoparticle-supported nanopipettes for in situ dual-mode monitoring of intracellular Cu(II) and potential chemodynamic therapy applications. Sensors Actuators B Chem. 2021;344:130271. doi:10.1016/j.snb.2021.130271.
- Nie X-L, Liu H-L, Pan Z-Q, et al. Recognition of plastic nanoparticles using a single gold nanopore fabricated at the tip of a glass nanopipette. Chem Commun. 2019;55(45):6397–6400. doi:10.1039/C9CC01358J.
- Boni W, Arbuckle-Keil G, Fahrenfeld NL. Inter-storm variation in microplastic concentration and polymer type at stormwater outfalls and a bioretention basin. Sci Total Environ. 2022;809:151104. doi:10.1016/j.scitotenv.2021.151104.
- Balestra V, Bellopede R. Microplastic pollution in show cave sediments: first evidence and detection technique. Environ Pollut. 2022;292:118261. doi:10.1016/j.envpol.2021.118261.
- Rist S, Hartmann NB, Welden NAC. How fast, how far: diversification and adoption of novel methods in aquatic microplastic monitoring. Environ Pollut. 2021;291:118174. doi:10.1016/j.envpol.2021.118174.
- Elsayed AA, Erfan M, Sabry YM, et al. A microfluidic chip enables fast analysis of water microplastics by optical spectroscopy. Sci Rep. 2021;11(1):10533. doi:10.1038/s41598-021-89960-4.
- Matthews C, Moran F, Jaiswal AK. A review on European Union’s strategy for plastics in a circular economy and its impact on food safety. J Clean Prod. 2021;283:125263. doi:10.1016/j.jclepro.2020.125263.
- Mitrano DM, Wohlleben W. Microplastic regulation should be more precise to incentivize both innovation and environmental safety. Nat Commun. 2020;11(1):5324. doi:10.1038/s41467-020-19069-1.
- Puharinen S-T. Achieving good marine environmental status in the EU – implications of the marine strategy framework directive for member states and blue economic activities. Mar Policy. 2023;155:105712. doi:10.1016/j.marpol.2023.105712.
- Anagnosti L, Varvaresou A, Pavlou P, Protopapa E, Carayanni V. Worldwide actions against plastic pollution from microbeads and microplastics in cosmetics focusing on European policies. Has the issue been handled effectively? Mar Pollut Bull. 2021;162:111883. doi:10.1016/j.marpolbul.2020.111883.
- Alava JJ, Lukyanova ON, Ross PS, Shim WJ.. Report of Working Group 31 on Emerging Topics in Marine Pollution. Canada: North Pacific Marine Science Organization (PICES); 2020. PICES Scientific Report No. 56
- Akhbarizadeh R, Yu JT, Ead L, et al. Reductions of plastic microbeads from personal care products in wastewater effluents and lake waters following regulatory actions. ACS ES&T Water. 2024;4(2):492–499. doi:10.1021/acsestwater.3c00526.
- Wuennenberg L, Tan CM. Plastic waste in Canada. Canada: International Institute for Sustainable Development; 2019
- Hu J, Miao L, Han J, Zhou W, Qian X. Waste separation behavior with a new plastic category for the plastic resource circulation: survey in Kansai, Japan. J Environ Manage. 2024;349:119370. doi:10.1016/j.jenvman.2023.119370.
- Agamuthu P, Mehran SB, Norkhairah A, Norkhairiyah A. Marine debris: a review of impacts and global initiatives. Waste Manag. Res. 2019;37(10):987–1002. doi:10.1177/0734242X19845041.
- Shooshtarian S, Maqsood T, Wong PSP, Yang RJ, Khalfan M. Review of waste strategy documents in Australia: analysis of strategies for construction and demolition waste. IJETM. 2020;23(1):1–21. doi:10.1504/IJETM.2020.110147.
- Sun Y, Liu S, Wang P, Jian X, Liao X, Chen W-Q. China’s roadmap to plastic waste management and associated economic costs. J Environ Manage. 2022;309:114686. doi:10.1016/j.jenvman.2022.114686.
- Meng M, Wen Z, Luo W, Wang S. Approaches and policies to promote zero-waste city construction: China’s practices and lessons. Sustainability. 2021;13(24):13537. doi:10.3390/su132413537.
- Venus O. National marine microbead policy in developed nations: how microbead bans have influenced microplastic pollution in waterways and begun the trend towards international collaboration [Thesis]. Ann Arbor, US: University of Michigan; 2020.
- Lopez EMB. Marine debris removal : a case study on South Korean marine litter management [Thesis]. Kingston, US: University of Rhode Island; 2021.
- Carlini G, Kleine K. Advancing the international regulation of plastic pollution beyond the United Nations environment assembly resolution on marine litter and microplastics. Rev Euro Comp Intl Enviro. 2018;27(3):234–244. doi:10.1111/reel.12258.
- Borg S, Attard FG, Vella de Fremeaux PM. Research Handbook on Ocean Governance Law. Cheltenham, UK: Edward Elgar Publishing; 2023.
- Synn K, Thesis JM. Strengthening global governance on marine plastic litter. 2020.