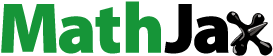
ABSTRACT
In irrigated rice fields, plant-mediated transfer of CH4 from submerged soils to the atmosphere raise the possibility of genotypic differences in CH4 emissions. Previous research has been contradictory, and varietal differences in rice CH4 emissions in Latin America have not been examined. A field experiment in Colombia tested whether irrigated rice emissions might be reduced using a breeding line, an inbred variety, and two rice hybrids. Data was collected on CH4 emissions, phenotypic, root, and grain yield parameters. Variations observed in CH4 emissions, grain yield, root length, and root surface area were in the order Hybrid 2 > Hybrid 1 > breeding line > inbred variety. CH4 emissions per unit area were between 29% and 62% greater for the hybrids than the inbred variety and breeding line, and CH4 emissions per unit grain yield were comparable across genotypes. Our findings suggest that differences in root characteristics and aboveground biomass explain genetic influences on CH4 emissions. The transition to low-emission rice production systems can be accelerated by using differences in productivity and root qualities among cultivars.
Key policy highlights
CH4 emission intensities were comparable among the breeding line, inbred variety and hybrids.
Breeding for shorter roots, lower root volume, and surface area without compromising yields may be beneficial in reducing rice-based methane emissions.
High-yielding, low-CH4 emission rice should be classified as a clean development technology that qualifies for carbon credits.
1. Introduction
Deficits in rice supply are common in Latin America and the Caribbean (LAC) region, where per capita rice consumption is approximately 30 kg per year. However, imports are used to make up the shortfall (Durand-Morat & Bairagi, Citation2021; Maclean et al., Citation2002). Irrigated rice, a known anthropogenic source of methane (CH4), currently accounts for over 70% of the LAC region’s total rice-growing area (Espinosa, Citation2002). Expansion of rice cultivation areas through the utilisation of available land and water resources is possible in countries such as Brazil, Colombia, and Venezuela (EMSAL Citation2021).
Globally, rice production is responsible for an estimated 10–12% of total CH4 emissions from anthropogenic sources (Ciais et al., Citation2013). Asian countries account for 90% of total global rice production, which is significantly higher than the estimated<5% of global rice production in the LAC region (EMSAL Citation2021). The disproportionately lower rice growing area in LAC implies a minor contribution of rice production systems to global methane emission compared to the Asian region (FAOSTAT, Citation2018). Nonetheless, rice production systems in the LAC region should aim to harness irrigation water resources to produce more rice without increasing total anthropogenic CH4 emissions (S. F. Islam et al., Citation2018).
Methanogens that produce CH4 flourish under anaerobic conditions characteristic of flooded rice fields (Sass & FM, Citation1997). Molecular diffusion, ebullition, and plant-assisted transport are the mediums used to carry CH4 from the soil to the atmosphere (Khosa et al., Citation2010). Previous studies have reported that>90% of methane emitted from rice fields is lost through plant-mediated transport (Butterbach-Bahl et al., Citation1997; Holzapfel-Pschorn et al., Citation1986; Wassmann & Aulakh, Citation2000). Yet, despite the importance of plants in transmitting CH4 from the soil to the atmosphere, research on varietal differences in CH4 emissions is limited.
Climate change mitigation efforts in rice production systems have primarily focused on cultural practices, including fertiliser placement (Gaihre et al., Citation2015, Citation2018; S. M. M. Islam et al., Citation2018, Citation2022), agricultural residue management (Wassmann et al., Citation1993; Yagi et al., Citation1997), and irrigation management (Cheng et al., Citation2022; S. M. M. Islam et al., Citation2016, Citation2020; Wassmann et al., Citation2000). However, currently, it is very challenging for farmers to change their fertilisation practices, residue and irrigation management in the absence of supportive legislation and economic incentives (Chirinda et al., Citation2018). To overcome this challenge exploiting varietal differences in CH4 emissions has been proposed as a more cost-effective technique for decreasing CH4 emissions in LAC because the associated adjustment would not dramatically alter farming practices (Chirinda et al., Citation2018; Gogoi et al., Citation2008).
Previous research (Gogoi et al., Citation2008; Qin et al., Citation2015; Wang et al., Citation2017) has revealed that genotypic differences in CH4 emissions exist, implying the possibility of selecting rice varieties with lower CH4 emissions. Gogoi et al. (Gogoi et al., Citation2008) observed that in northeast India, high-yielding rice varieties such as IR-36 had lower CH4 emissions compared to traditional varieties. In the Gogoi et al. (Gogoi et al., Citation2008) study, the differences in CH4 emissions were attributed to differences in aboveground biomass accumulation. They conclude that cultivating high-yielding rice varieties and hybrids may be key for food security and climate change mitigation (Gogoi et al., Citation2008). In a separate study, Zhang et al. (Zhang et al., Citation2019) examined a rice hybrid and variety in China and found that root form and physiological parameters were adversely and significantly linked with total CH4 emissions. The rice hybrid used in the study by Zhang et al. (Zhang et al., Citation2019) produced less CH4 and had higher yields than those observed in the studied rice variety.
The concept of genotypes that emit less CH4 without compromising yield is a compelling solution for fighting climate change and achieving food security goals (Balakrishnan et al., Citation2018). Therefore, monitoring CH4 emissions from different varieties and hybrids is necessary to identify genotypes that reduce CH4 emissions without compromising grain yield. Furthermore, to guide future breeding efforts, it is necessary to understand the mechanisms that drive CH4 emission reductions. In the present study, We hypothesised that hybrids produce less CH4 per unit yield because they had higher harvest indexes than varieties.
2. Materials and methods
From June to October 2019, a designed experiment was conducted on a rice field at the International Center of Tropical Agriculture (CIAT) in Cali, Colombia. The field was laid out in a randomised complete block design with four treatments in four replicates. Four rice cultivars were used in the study: a Colombian inbred variety (135 days maturity), a CIAT experimental breeding line previously chosen for its increased root length (130 days maturity), and two hybrids [Hybrid 1 (126 days maturity) and Hybrid 2 (128 days maturity)] chosen for their higher yield performance in CIAT field trials compared to check-inbred-varieties. On the 17th of June 2019, twenty-one-day-old rice seedlings were transplanted into field plots. The inbred variety and breeding line had similar plant populations (250,000 plants per hectare) but higher than those used for the hybrids (111,111 plants per hectare). shows the soil parameters in the experimental field. The neutral soil pH (7.4) and high available phosphorous (49 mg kg−1) levels indicate good soil conditions for rice production. All the experimental plots were under continuous flooding during the growing season, and water was constantly applied to maintain the flooded conditions in plots with all the rice genotypes. The experimental site was mechanically prepared, irrigated and puddled before seedling transplanting. The gross plot size was 20 m2, while the harvested net plot was 12 m2. Nitrogen fertiliser was applied at 180 kg N ha−1 and 200 kg N ha−1 for the varieties and hybrids, respectively, in three split applications of 10%, 70%, and 20% at 2, 10, and 55 days after transplanting, respectively. Similar rates of phosphorus (65 kg ha−1) and potassium (90 kg ha−1) were applied to all plots. Five aboveground plant samples were obtained from each treatment gross plot at four growth phases. The samples were dried at 60°C for 72 hours. The dry weight of plants from different net plots at harvest maturity was divided into leaves, stems, roots, and panicles. AgroTronix MT-PRO moisture metre was also used to determine the grain moisture content at harvest maturity. At maturity, root length, volume, and surface area were measured according to (Ogawa et al., Citation2014). Roots from five plants were collected with a core sampler, washed, and scanned with an EPSON scanner before being analysed with WinRhizo.
Table 1. Soil properties at the study site.
From July 10 to 25 September 2019, gas measurements were conducted on 16 separate days during the rice growing season. Around fertilisation, gas samples were collected more frequently. Specifically, four gas samples were collected from each treatment plot one day before and three days after fertilisation. For the rest of the rice growing season, gas measurements were conducted weekly until harvest. All samplings were in the morning (8:00 to 11:00 a.m.). The static-closed chamber technique was utilised for gas sampling (31). Plastic buckets (114 L volume and 80 cm height) were used with custom-made chamber bases (40 cm height) that contained a canal, allowing for a water seal during chamber deployment. The static chambers had vents to facilitate pressure equilibration and a fan to mix the air (Chirinda et al., Citation2017). During each sampling campaign, at four 15-minute intervals (0, 15, 30, 45 mins), gas samples (20 mL) were obtained from the closed static chambers in each treatment plot using a syringe and injected into pre-evacuated vials before analysis. The methane concentration in the vials was determined using gas chromatography (Shimadzu GC-20C14) equipped with a Porapak K column and a flame ionisation detector (FID). The carrier gas was nitrogen (N2). Methane fluxes were calculated using the gas concentration rate, ideal gas equation and sampling time.
∆C/∆t is the concentration change (ppm-CH4 h−1); V is chamber volume (m3); A is chamber area (m2); ρ is gas density (0.717 kg m−3 at 0°C); and T is the mean air temperature inside the chamber (°C). The cumulative fluxes for the monitoring period were calculated using linear interpolation and expressed as emissions per unit area (absolute emissions). CH4 emission intensities were calculated using the ratio of cumulative emissions to grain yield, which was expressed in mg CH4 per kg grain dry matter yield.
3. Statistical analyses
The normality of the data was checked. We used SAS’s proc mixed method to evaluate the data on crop parameters because it was normally distributed. The models investigated the effects of genotype on various crop parameters. As random effects, the replicates were included. Pairwise comparisons were performed using Differences of Least Square Means in conjunction with these models. The methane data were not normally distributed, and none of the transformation procedures yielded variance homogeneity or normal distribution. To examine the paired difference in methane emissions between the genotypes, we utilised a non-parametric approach (the Wilcoxon Signed Rank test), which does not need the assumption of normality. While non-parametric methods may not provide the same breadth of statistical insights as parametric methods, they are helpful when flux data is non-normal (Corre et al., Citation1996).
4. Results
Aboveground biomass accumulation was comparable in all the genotypes during the vegetative growth stage (). However, the hybrids had significantly higher aboveground biomass than the other genotypes at 50% flowering and physiological maturity. Hybrid 2 had a significantly longer root system than other genotypes at maturity (). Root volume and surface area followed the same trend as root length, but the breeding line’s values were comparable to those in Hybrid 2 (which had the biggest root volume) and higher than those in Hybrid 1 and the inbred variety.
Figure 1. Aboveground biomass accumulation by the four rice cultivars at four growth stages (active tillering, maximum tillering, 50% flowering and maturity). The error bars represent the standard error of the means.
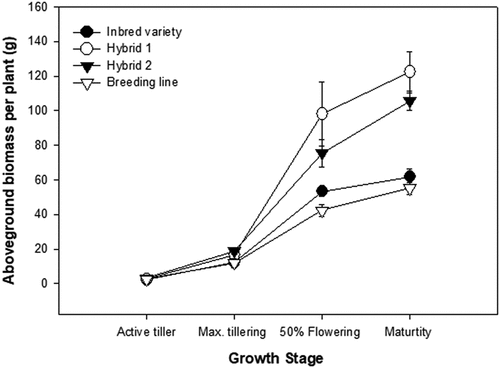
Table 2. Root parameters measured during the rice-growing season.
Tiller numbers at maximum tillering, flowering and physiological maturity were significantly higher for the inbred variety, which had a higher plant count (25 per m2) than the hybrids (11 per m2) (). However, despite the high plant count (25 per m2), the breeding line had similar tillers to the hybrids at maximum tillering and flowering. The hybrids produced similar grain yields, but the yield was higher than the other genotypes (). For Hybrid 1 and Hybrid 2, the relative increase in yield over the inbred variety was 26.4% and 26.7%, respectively. Hybrids 1 and 2, correspondingly, exhibited 46.0% and 46.3% higher grain yields than the breeding line. The different genotypes had similar daily methane fluxes (). Cumulative methane emissions followed the order: Hybrid 2 > Hybrid 1 > breeding line > inbred variety (). Hybrid 2 emitted 18.5% more than Hybrid 1, which emitted 22.2% more than the breeding line, while the breeding line emitted 3.2% more CH4 compared to the inbred variety. Even though the hybrids had much higher absolute CH4 emissions (i.e. emissions per unit area) than the breeding line and inbred variety, CH4 emission intensities (i.e. emissions per unit yield) were comparable across genotypes.
Figure 2. Methane emission dynamics of four rice cultivars observed during the monitoring period. Arrow indicates the date of the third N fertilisation.
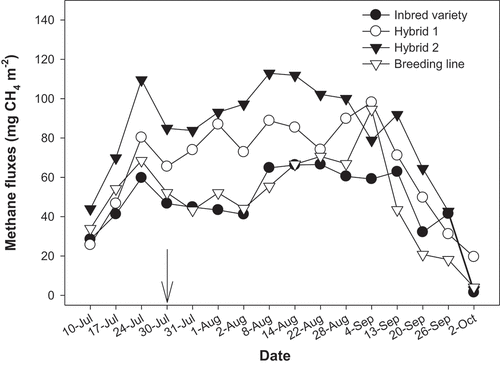
Table 3. Tiller numbers (per m2) counted during three growth stages of the rice-growing seasons.
Table 4. Cumulative CH4 emissions, grain yield and grain quality parameters.
During the maximum tillering period, the mean daily CH4 fluxes were significantly higher in the hybrids than in the in-bred variety and breeding line (). However, the mean daily CH4 fluxes were similar in the in-bred variety and the breeding line. On the other hand, mean daily CH4 emissions were similar for the hybrids. Though not significantly different, similar patterns in mean daily CH4 fluxes were observed during the flowering period. During the rice maturing period, the daily CH4 fluxes were significantly higher in Hybrid 2 than in the breeding line ().
Table 5. Mean daily methane fluxes (mg CH4 m−2 d−1) during the maximum tillering, flowering and physiological maturity period.
When we excluded the breeding line and only focused on the commercial hybrids and in-bred variety, we observed linear relationships (r2>0.98) between mean daily CH4 emissions and selected plant parameters, including aboveground biomass at maximum tillering, root surface area, volume and length ().
Figure 3. For the commercial genotypes only (excluding the breeding line), the linear relationships between mean daily methane emissions (measured during the respective periods) and (a) biomass at maximum tillering, (b) root surface area at maturity, (c) root volume at maturity, and (d) root length at maturity.
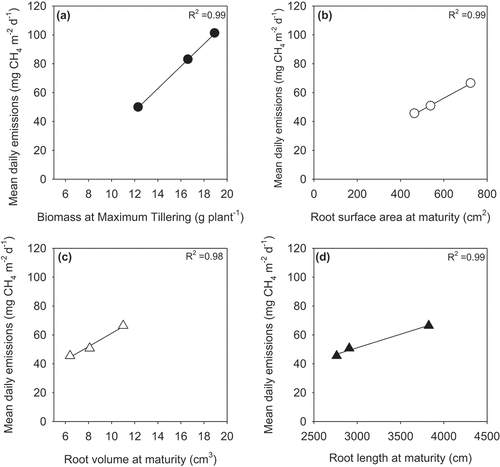
5. Discussion
Based on the dominance of rice-mediated CH4 transmission from the submerged soil to the atmosphere (Jia & Cai, Citation2003), previous studies have shown that genetic variations in rice influence CH4 emissions from irrigated rice systems (Baruah et al., Citation2010; Jia & Cai, Citation2003; Qin et al., Citation2015). The current study rejected our hypothesis that the studied rice hybrids produce less CH4 per unit yield. However, we observed differences in absolute CH4 emissions, with the inbred variety and breeding line resulting in significantly lower CH4 emissions than those obtained for the rice hybrids. Though we explored several plant parameters (supplementary material), focusing on the commercial inbred variety and the hybrids, we observed a strong relationship between mean daily CH4 emissions to aboveground at maximum tillering and root parameters (root length, volume and surface area) measured during the physiological maturity period. This finding is in corroboration with previous studies that observed differences in phenotypic parameters such as aboveground biomass and root characteristics are major drivers of differences in CH4 emissions between rice genotypes (Baruah et al., Citation2010; Khosa et al., Citation2010; Su et al., Citation2015). The lower grain yields and CH4 emissions observed in the inbred variety, despite its higher tiller numbers, suggest that several of these tillers were unproductive, and the high tiller numbers did not translate to high CH4 emissions. The low CH4 emissions may be because high tiller numbers in the inbred variety may have enhanced CH4 oxidation due to a bigger volume of aerenchyma increasing oxygen movement from the atmosphere to the rhizosphere (Bhattacharyya et al., Citation2019).
The close alignment between CH4 emissions and different root parameters is unsurprising as the roots of different genotypes have been reported to influence the soil methanotrophic community composition in various ways (Aulakh et al., Citation2000). Root influences on the methanotrophic community are probably driven by the fact that different rice cultivars appear to devote more photosynthetic products to root exudation than others (Win et al., Citation2012). The root exudates provide an organic substrate for microbial organisms that soil methanogens can use as C sources for CH4 production (Setyanto et al., Citation2004). In addition, the release of root exudates, including simple sugars (electron donors), enhances soil anaerobiosity creating conditions conducive to CH4 production by affecting the soil redox potential (Gutierrez et al., Citation2014; Wassmann & Aulakh, Citation2000). Wassmann and Aulakh (Wassmann & Aulakh, Citation2000) further highlight that variations in the quantity and quality of exudates may emanate from differences in rice genotypes. In this study, we did not monitor the content and composition of root exudates; however, we suspect that the root length, volume and surface area may have influenced the quantity of root exudates released into the rhizosphere (Williams et al., Citation2022). Hybrid 2, which had the largest root length, volume and surface area at maturity, consistently had the highest CH4 emissions throughout the season, further suggesting the importance of root characteristics in driving CH4 production. On the other hand, the inbred variety with the least aboveground biomass at maximum tillering, 50% flowering and maturity, the shortest root length and the smallest root volume and surface area also had the lowest absolute CH4 emissions.
In corroboration with observations made by Minoda and Kimura (Minoda & Kimura, Citation1994), and Aulakh et al (Aulakh et al., Citation2001), the high aboveground biomass observed in the hybrids at 50% flowering and physiological maturity may have contributed to increased root exudates and, consequently, the high CH4 emissions. On the other hand, the high aboveground biomass observed for the hybrids may have resulted in greater photosynthetic rates that translated to higher grain yields compared to those observed for the inbred variety and breeding line (Ogawa et al., Citation2014; Pittelkow et al., Citation2013; Zhou et al., Citation2017). According to our findings, the hybrids had high grain yields and CH4 emissions, while the inbred variety and breeding line had low grain yields and low emissions resulting in similar CH4 emission intensities for all the studies genotypes.
Under continuously flooded rice production systems, CH4 oxidation in the anaerobic soil is expected to be low. However, the rice aerenchyma system may also support the movement of atmospheric oxygen into the rhizosphere to support root respiration and contribute to CH4 oxidation (Gerard & Chanton, Citation1993). Previous studies have shown that about 90% of produced CH4 during rice maturation is oxidised (Sass et al., Citation1992; Schütz et al., Citation1989), which may explain the reduction in the emitted CH4 from 13 September 2019 (88 days after transplanting).
The main objective of rice farmers is to maximise yields. Our findings show that adopting rice hybrids is critical for achieving this goal. However, farmers have to incur upfront costs of seeds and additional inputs (i.e. fertilisers) on the assumption of recuperating these costs to form a higher-yielding crop. However, other factors, such as extreme weather events caused by climate change and variability and pest and disease attacks, may negatively impact the yield potential resulting in losses for farmers. Inbred varieties whose seeds can be reused without compromising yields and require lower fertiliser inputs may thus be attractive for resource-limited farmers, but their productivity is lower. Notwithstanding the importance of inbred varieties in sustainable agriculture, similarities of CH4 emission intensities among the genotypes suggest the possibility of simultaneously meeting food security and climate change mitigation goals through hybrids. Nonetheless, to further reduce CH4 emissions, it may be necessary to consider combining the adoption of rice hybrids with other proven practices such as Alternate Wetting and Drying (AWD) and residue management.
6. Conclusion
Our findings suggest that breeding for shorter roots, smaller root volume, and surface area without sacrificing yields could help reduce rice-based CH4 emissions. To further encourage the use of high-yielding, low-CH4 emission rice cultivars, further research should be conducted to generate additional evidence that supports the classification of those genotypes as clean development technologies that qualify for carbon credits. While low absolute emissions could result from a low-yielding rice variety (per unit area), farmers will need more acreage to produce enough rice to alleviate the region’s rice shortages. Promoting hybrids may be a more promising strategy for simultaneously achieving food security and emission reduction targets. However, hybrid seeds are more expensive than inbred varieties, which may make the latter to be a more attractive and sustainable option for resource-constrained farmers. Therefore, to support sustainable rice production in the LAC region, improved management and technological options should be promoted considering the multiple socio-economic contexts.
Acknowledgments
This research was supported by the CGIAR Research Program on Climate Change, Agriculture and Food Security (CCAFS) and the Global Research Alliance on Agricultural Greenhouse Gases (GRA) through their CLIFF-GRADS program. CCAFS is carried out with support from CGIAR Trust Fund and through bilateral funding agreements. For details, please visit https://ccafs.cgiar.org/donors. We are grateful to the International Center for Tropical Agriculture (CIAT), Palmira, Colombia, with the rice program the Physiology Group and Phenomics Platform for their support with different methodologies in physiology and root development and the Latin American Fund for Irrigated Rice (FLAR), Palmira, Colombia, for hosting the first author who was the recipient of the scholarship and to the Government of New Zealand for providing the financial support.
Disclosure statement
No potential conflict of interest was reported by the authors.
Data availability statement
The data used to support the findings of this study are available from the corresponding author upon request.
References
- Aulakh, M. S., Bodenbender, J., Wassmann, R., & Rennenberg, H. (2000). Methane transport capacity of rice plants. II. Variations among different rice cultivars and relationship with morphological characteristics. Nutrient Cycling in Agroecosystems, 58(1/3), 367–375. https://doi.org/10.1023/A:1009839929441
- Aulakh, M. S., Wassmann, R., Bueno, C., Rennenberg, H., & Rennenberg, H. (2001). Characterization of root exudates at different growth stages of rice (Oryza sativa L.) cultivars. Plant Biology, 3(2), 139–148. https://doi.org/10.1055/s-2001-12905
- Balakrishnan, D., Kulkarni, K., Latha, P. C., & Subrahmanyam, D. (2018). Crop improvement strategies for mitigation of methane emissions from rice. Emirates Journal of Food and Agriculture, 30, 45–462.
- Baruah, K. K., Gogoi, B., & Gogoi, P. (2010). Plant physiological and soil characteristics associated with methane and nitrous oxide emission from rice paddy. Physiology and Molecular Biology of Plants, 16(1), 79–91. https://doi.org/10.1007/s12298-010-0010-1
- Bhattacharyya, P., Dash, P. K., Swain, C. K., Padhy, S. R., Roy, K. S., Neogi, S., Berliner, J., Adak, T., Pokhare, S. S., Baig, M. J., & Mohapatra, T. (2019). Mechanism of plant mediated methane emission in tropical lowland rice. The Science of the Total Environment, 651, 84–92. https://doi.org/10.1016/j.scitotenv.2018.09.141
- Butterbach-Bahl, K., Papen, H., & Rennenberg, H. (1997). Impact of gas transport through rice cultivars on methane emission from paddy fields. Plant, Cell & Environment, 20(9), 1175–1183. https://doi.org/10.1046/j.1365-3040.1997.d01-142.x
- Cheng, H., Shu, K., Zhu, T., Wang, L., Liu, X., Cai, W., Qi, Z., & Feng, S. (2022). Effects of alternate wetting and drying irrigation on yield, water and nitrogen use, and greenhouse gas emissions in rice paddy fields. Journal of Cleaner Production, 349, 131487. https://doi.org/10.1016/j.jclepro.2022.131487
- Chirinda, N., Arenas, L., Katto, M., Loaiza, S., Correa, F., Isthitani, M., Loboguerrero, A., Martínez-Barón, D., Graterol, E., Jaramillo, S., Torres, C., Arango, M., Guzmán, M., Avila, I., Hube, S., Kurtz, D., Zorrilla, G., Terra, J., Irisarri, P. … Bayer, C. (2018). Sustainable and low greenhouse gas emitting rice production in Latin America and the Caribbean: A review on the transition from ideality to reality. Sustainability, 10(3), 671. https://doi.org/10.3390/su10030671
- Chirinda, N., Arenas, L., Loaiza, S., Trujillo, C., Katto, M., Chaparro, P., Nuñez, J., Arango, J., Martinez-Baron, D., Loboguerrero, A., Becerra Lopez-Lavalle, L., Avila, I., Guzmán, M., Peters, M., Twyman, J., García, M., Serna, L., Escobar, D., Arora, D. … Barahona, R. (2017). Novel technological and management options for accelerating transformational changes in rice and livestock systems. Sustainability, 9(11), 1891. https://doi.org/10.3390/su9111891
- Ciais, P., Sabine, C., Bala, G., Bopp, L., Brovkin, V. (2013). ‘Carbon and Other Biogeochemical Cycles’. Climate Change 2013 –The Physical Science Basis, Contribution of Working Group I to the Fifth Assessment Report of the Intergovernmental Panel on Climate Change, Cambridge University Press, 465–570.
- Corre, M. D., van Kessel, C., & Pennock, D. J. (1996). Landscape and seasonal patterns of nitrous oxide emissions in a semiarid region Soil Sci. Soil Science Society of America Journal, 60(6), 1806–1816. https://doi.org/10.2136/sssaj1996.03615995006000060028x
- Durand-Morat, A., & Bairagi, S. (2021). International Rice Outlook: International Rice Baseline Projections 2020-2030. Research Reports and Research Bulletins. Retrieved from https://scholarworks.uark.edu/aaesrb/49, Series Number 1006.
- Espinosa, J. (2002). Rice nutrition management in Latin America. Better Crops International, 16, 36–39.
- FAOSTAT. (2018). Food and agriculture data. https://www.fao.org/faostat/en/#data. Retrieved January 31, 2023.
- FLAR Monitoring and Follow-up Survey of the Rice Sector in Latin America – EMSAL. (2021). Latin American Fund for Irrigated Rice.
- Gaihre, Y. K., Singh, U., Islam, S. M. M., Huda, A., Islam, M. R., Sanabria, J., Satter, M. A., Islam, M. R., Biswas, J. C., Jahiruddin, M., & Jahan, M. S. (2018). Nitrous oxide and nitric oxide emissions and nitrogen use efficiency as affected by nitrogen placement in lowland rice fields. Nutrient Cycling in Agroecosystems, 110(2), 277–291. https://doi.org/10.1007/s10705-017-9897-z
- Gaihre, Y. K., Singh, U., Islam, S. M., Huda, A., Islam, M., Satter, M. A., Sanabria, J., Islam, R., & Shah, A. (2015). Impacts of urea deep placement on nitrous oxide and nitric oxide emissions from rice fields in Bangladesh. Geoderma, 259–260, 370–379. https://doi.org/10.1016/j.geoderma.2015.06.001
- Gerard, G., & Chanton, J. (1993). Quantification of methane oxidation in the rhizosphere of emergent aquatic macrophytes: Defining upper limits. Biogeochemistry, 23(2), 79–97. https://doi.org/10.1007/BF00000444
- Gogoi, N., Baruah, K. K., & Gupta, P. K. (2008). Selection of rice genotypes for lower methane emission. Agronomy for Sustainable Development, 28(2), 181–186. https://doi.org/10.1051/agro:2008005
- GRiSP (Global Rice Science Partnership). (2013). Rice almanac (4th ed., pp. 283). International Rice Research Institute.
- Gutierrez, J., Atulba, S. L., Kim, G., & Kim, P. J. (2014). Importance of rice root oxidation potential as a regulator of CH4 production under waterlogged conditions. Biology and Fertility of Soils, 50(5), 861–868. https://doi.org/10.1007/s00374-014-0904-0
- Holzapfel-Pschorn, A., Conrad, R., & Seiler, W. (1986). Effects of vegetation on the emission of methane from submerged paddy soil. Plant and Soil, 92(2), 223–391. https://doi.org/10.1007/BF02372636
- Islam, S. M. M., Gaihre, Y. K., Biswas, J. C., Singh, U., Ahmed, M. N., Sanabria, J., & Saleque, M. A. (2018). Nitrous oxide and nitric oxide emissions from lowland rice cultivation with urea deep placement and alternate wetting and drying irrigation. Scientific Reports, 8(1), 17623. https://doi.org/10.1038/s41598-018-35939-7
- Islam, S. M. M., Gaihre, Y. K., Islam, M. R., Akter, M., Mahmud, A. A., Upendra, S., & Sander, B. O. (2020). Effects of water management on greenhouse gas emissions from farmers’ rice fields in Bangladesh. The Science of the Total Environment, 734, 139382. 2020. https://doi.org/10.1016/j.scitotenv.2020.139382
- Islam, S. M. M., Gaihre, Y. K., Islam, M. R., Khatun, A., & Islam, A. (2022). Integrated plant nutrient systems improve rice yields without affecting greenhouse gas emissions from lowland rice cultivation. Sustainability, 14, 11338. https://doi.org/10.3390/su141811338
- Islam, S. M. M., Gaihre, Y. K., Shah, A. L., Singh, U., Sarkar, M. I. U., Abdus Satter, M., Sanabria, J., & Biswas, J. C. (2016). Rice yields and nitrogen use efficiency with different fertilizers and water management under intensive lowland rice cropping systems in Bangladesh. Nutrient Cycling in Agroecosystems, 106(2), 143–156. https://doi.org/10.1007/s10705-016-9795-9
- Islam, S. F., Groenigen, J. W. V., Jensen, L. S., Sander, B. O., & de Neergaard, A. (2018). The effective mitigation of greenhouse gas emissions from rice paddies without compromising yield by early-season drainage. The Science of the Total Environment, 612, 1329–1339. https://doi.org/10.1016/j.scitotenv.2017.09.022
- Jia, Z., & Cai, Z. (2003). Effects of rice plants on methane emission from paddy fields. The Journal of Applied Ecology, 14, 2049–2053.
- Khosa, M. K., Sidhu, B. S., & Benbi, D. K. (2010). Effect of organic materials and rice cultivars on methane emission from rice field. Journal of Environmental Biology, 31(3), 281–285.
- Maclean, J. L., Dawe, D. C., & Hardy, B. (2002). Rice Almanac: Sourcebook for the most important economic activity on earth (Hettel GP, Eds). International Rice Research Institute, CABI Publishing.
- Minoda, T., & Kimura, M. (1994). Contribution of photosynthesized carbon to the methane emitted from paddy fields, Geophys. Geophysical Research Letters, 21(18), 2007–2010. https://doi.org/10.1029/94GL01595
- Ogawa, S., Selvaraj, M. G., Fernando, A. J., Lorieux, M., Ishitani, M., McCouch, S., & Arbelaez, J. D. (2014). N-and P-mediated seminal root elongation response in rice seedlings. Plant and Soil, 375(1–2), 303–315. https://doi.org/10.1007/s11104-013-1955-y
- Pittelkow, C. M., Adviento-Borbe, M. A., Hill, J. E., Six, J., van Kessel, C., & Linquist, B. A. (2013). Yield-scaled global warming potential of annual nitrous oxide and methane emissions from continuously flooded rice in response to nitrogen input. Agriculture, Ecosystems & Environment, 177, 10–20. https://doi.org/10.1016/j.agee.2013.05.011
- Qin, X., Li, Y., Wang, H., Li, J., Wan, Y., Gao, Q., Liao, Y., & Fan, M. (2015). Effect of rice cultivars on yield-scaled methane emissions in a double rice field in South China. Journal of Integrative Environmental Sciences, 12(Suppl. 1), 47–66. https://doi.org/10.1080/1943815X.2015.1118388
- Sass, R. L., Fisher, F. M., Wang, Y. B., Turner, F. T., & Jund, M. F. (1992). Methane emission from rice fields: The effect of floodwater management. Global Biogeochemical Cycles, 6(3), 249–262. https://doi.org/10.1029/92GB01674
- Sass, R. L., & FM, F. (1997). Methane emissions from rice paddies: A process study summary. Nutrient Cycling in Agroecosystems, 49(1/3), 119–127. https://doi.org/10.1023/A:1009702223478
- Schütz, H., Holzapfel-Pschorn, A., Conrad, R., Rennenberg, H., & Seiler, W. (1989). A three-year continuous record on the influence of daytime season and fertilizer treatment on methane emission rates from an Italian rice paddy field. Journal of Geophysical Research, 94(D13), 16405–16416. https://doi.org/10.1029/JD094iD13p16405
- Setyanto, P., Rosenani, A. B., Boer, R., Fauziah, C. I., & Khanif, M. J. (2004). The effect of rice cultivars on methane emission from irrigated rice field. Indonesian Journal of Agricultural Science, 5(1), 20–31. https://doi.org/10.21082/ijas.v5n1.2004.p20-31
- Su, J., Hu, C., Yan, X., Jin, Y., Chen, Z., Guan, Q., Wang, Y., Zhong, D., Jansson, C., Wang, F., Schnürer, A., & Sun, C. (2015). Expression of barley SUSIBA2 transcription factor yields high-starch low-methane rice. Nature, 523(7562), 602–606. 7562. https://doi.org/10.1038/nature14673
- Wang, C., Lai, D. Y. F., Sardans, J., Wang, W., Zeng, C., Peñuelas, J., & Hui, D. (2017). Factors Related with CH4 and N2O Emissions from a Paddy Field: Clues for Management implications. PloS One, 12(1), e0169254. https://doi.org/10.1371/journal.pone.0169254
- Wassmann, R., & Aulakh, M. S. (2000). The role of rice plants in regulating mechanisms of methane emissions. Biology and Fertility of Soils, 31(1), 20–29. https://doi.org/10.1007/s003740050619
- Wassmann, R., Lantin, R. S., Neue, H. U., Buendia, L. V., Corton, T. M., & Lu, Y. (2000). Characterization of methane emissions from rice fields in Asia. III. Mitigation options and future research needs. Nutrient Cycling in Agroecosystems, 58(1/3), 23–36. https://doi.org/10.1023/A:1009874014903
- Wassmann, R., Papen, H., & Rennenberg, H. (1993). Methane emission from rice paddies and possible mitigation strategies. Chemosphere, 26(1–4), 201–217. https://doi.org/10.1016/0045-6535(93)90422-2
- Williams, A., Langridge, H., Straathof, A. L., Muhamadali, H., Hollywood, K. A., Goodacre, R., & de Vries, F. T. (2022). Root functional traits explain root exudation rate and composition across a range of grassland species. The Journal of Ecology, 110(1), 21–33. https://doi.org/10.1111/1365-2745.13630
- Win, K. T., Nonaka, R., Win, A. T., Sasada, Y., Toyota, K., Motobayashi, T., & Hosomi, M. (2012). Comparison of methanotrophic bacteria, methane oxidation activity, and methane emission in rice fields fertilized with anaerobically digested slurry between a fodder rice and a normal rice variety. Paddy Water Environment, 10(4), 281–289. https://doi.org/10.1007/s10333-011-0279-x
- Yagi, K., Tsuruta, H., & Minami, K. (1997). Possible options for mitigating methane emission from rice cultivation. Nutrient Cycling in Agroecosystems, 49(1/3), 213–220. https://doi.org/10.1023/A:1009743909716
- Zhang, H., Liu, H., Hou, D., Zhou, Y., Liu, M., Wang, Z., Liu, L., Gu, J., & Yang, J. (2019). The effect of integrative crop management on root growth and methane emission of paddy rice. The Crop Journal, 7(4), 444–457. https://doi.org/10.1016/j.cj.2018.12.011
- Zhou, Q., Ju, C. X., Wang, Z. Q., Zhang, H., Liu, L. J., Yang, J. C., & Zhang, J. H. (2017). Grain yield and water use efficiency of super rice under soil water deficit and alternate wetting and drying irrigation. Journal of Integrative Agriculture, 16(5), 1028–1043. https://doi.org/10.1016/S2095-3119(16)61506-X