ABSTRACT
Indigenous legume crops are pivotal in providing proteins and food security to sub-Saharan African rural communities, but most of these crops are underutilized because of the so-called hard-to-cook (HTC) phenomenon in combination with inadequate processing techniques. This review studies the case of bambara groundnut, which is third in importance after groundnut and cowpea and especially adapted to semi-arid areas. Published data on the HTC phenomenon implicate microstructural and compositional changes as factors leading to its development. Useful and sustainable techniques to process HTC legumes in developing countries include cooking with alkaline salts, milling, roasting, fermentation, and malting. Improvement of these processing techniques in relation to nutrient bioaccessibility, safety, and consumer acceptance of the products is urgently needed. Recommendations are to lessen the problems of food security in sub-Saharan African countries through, amongst other means, the optimization of bambara groundnut processing methods.
Introduction
In sub-Saharan Africa, indigenous crops such as legumes play an important role, especially during times of famine, by providing nutrition and a source of income.(Citation1–Citation3) Promoting indigenous, drought-tolerant crops is a possible way of alleviating the impacts of climate change and successive droughts experienced in arid and semi-arid regions.(Citation4) Bambara groundnut (Vigna subterranea (L.) Verdc.) is an underutilized indigenous crop, which is cultivated by subsistence women farmers throughout Zimbabwe,(Citation5–Citation7) as well as other tropical and subtropical countries in sub-Saharan Africa and some parts of Latin America and Asia.(Citation7–Citation9) Its origin has been traced back to the Sahelian region of present-day West Africa, and its name is derived from the Bambara tribe, who at present live in Mali.(Citation9,Citation10)
Bambara groundnut is commonly grown as a rotational crop with legumes like groundnut (Arachis hypogea) and cowpea (Vigna unguiculata) and cereals like maize (Zea mays), sorghum (Sorghum bicolor), and millet (Pennisetum glaucum) in agroecological regions that receive minimal annual rainfall.(Citation5,Citation7) In Zimbabwe, five agroecological regions are distinguished, numbered in descending order of agricultural productivity, soil type, and annual rainfall.(Citation11,Citation12) Ninety percent of the rural areas in Zimbabwe are situated in agroecological regions III, IV, and V, which receive low rainfall (≤800 mm) in relatively short seasons, making them unsuitable for intensive cropping of maize.(Citation11–Citation13) According to Hillocks et al.,(Citation5) the land area considered appropriate for bambara groundnut cultivation was, for example: Swaziland 100%, Uganda 98%, Zambia 95%, Burundi 89%, Zimbabwe 84%, and Central African Republic 79%. In Zimbabwe, bambara groundnut is considered an important crop for improving food security because of its capacity to tolerate harsh climatic conditions under a low-input agricultural system where many other crops fail.(Citation14–Citation16) The most recent production figures from Zimbabwe’s Central Statistics Office in 1997 show a countrywide production estimate of 15,138 × 103 kg, with 10,161 × 103 kg from the rural areas, 3219 × 103 kg from resettlement areas, 1694 × 103 kg and 64 × 103 kg from small-scale and large-scale commercial farms, respectively.(Citation16) Like the other legumes, bambara groundnut improves soil fertility (i.e., increases the level of soil nitrogen) through a symbiotic relationship with bacteria that form root nodules.(Citation17)
In Africa, bambara groundnut is ranked third in importance after groundnut and cowpea,(Citation8,Citation18,Citation19) and although research findings suggest that bambara groundnut is a crop with great nutritional and agronomic potential, it remains one of the most neglected.(Citation7) The major factor limiting the utilization of bambara groundnut is the hard-to-cook (HTC) phenomenon, which develops during high temperature (30–40 °C) and high relative humidity (RH) (>75%) storage in tropical and subtropical regions.(Citation2,Citation5,Citation20–Citation23) In Zimbabwe and other sub-Saharan countries, bambara groundnut is consumed in numerous ways at different stages of maturity. Freshly harvested pods are eaten as snacks after boiling for approximately an hour.(Citation2,Citation5,Citation7,Citation16,Citation21) HTC bambara groundnuts may be roasted and eaten as snacks.(Citation7) The seeds may also be milled into flour, baked to make small flat cakes and bread.(Citation24) Additionally, thin porridge(Citation25) and stiff porridge have been made from the flour.(Citation7) In Eastern Africa, bambara groundnuts are roasted, milled, and the flour is used to make a soup, a relish, and also a substitute for coffee.(Citation26)
HTC bambara groundnuts require longer boiling time (namely 3–4 hours),(Citation7) and therefore higher-energy expenditure to become edible as compared to cowpea or common bean (Phaseolus vulgaris).(Citation5) Cooking time of other legumes is reported to be: for soybean (Glycine max) 3.6 hours,(Citation27) common bean 1.5 hours,(Citation28) cowpea 2.4 hours,(Citation29) and mung bean (Vigna radiata) 0.5 hours.(Citation30) The HTC problem may not seem serious for people living in the developed worlds, who have energy resources and state-of-the-art techniques to utilize HTC legumes. However, in Zimbabwe boiling HTC bambara groundnuts is challenging, especially for those of lower socioeconomic status, who rely on firewood to provide energy for cooking and heating.(Citation31,Citation32) According to Bressani,(Citation33) a longer cooking time and high-energy consumption are important factors limiting bean preparation and consumption. In Zimbabwe, firewood constitutes 49% of the total energy used,(Citation34) with over 90% of rural and urban households depending on firewood energy as a result of countrywide power shortages experienced on a daily basis.(Citation34,Citation35) Recent studies show that on average a household in Zimbabwe uses approximately 8 m3 of firewood per year for domestic purposes.(Citation32,Citation34) Therefore, it is a concern that, while demand for firewood continues to grow in rural Zimbabwe, rapid land use and deforestation have reduced the supply of firewood, leading individuals to travel to forests that are very far from their homesteads for collection.(Citation36) Consequently, due to limited energy supply available for boiling, consumption of bambara groundnut is reduced. Additionally, it was found in other legumes that hardening of legume seeds causes a decrease in nutritional quality (e.g., in vitro protein digestibility),(Citation37) and extended boiling leads to reduced palatability and reduced protein digestibility.(Citation38,Citation39) Furthermore, HTC phenomena in bambara groundnut result in hard-to-mill properties, which are a concern to processors, who find it difficult to find proper dehulling and milling techniques.(Citation5,Citation21,Citation22,Citation40)
Amarteifio et al.(Citation14) and de-Kock(Citation7) described the variation in preferences for bambara groundnut seeds between Botswanan and Zimbabwean consumers; consumers from the southern and central districts of Botswana prefer the white and cream-colored landraces, whereas those in the northern part close to the Zimbabwean border prefer the red landraces. Red seeds were reported to be more popular than cream-colored seeds in Zimbabwe.(Citation5) In Ghana, consumers who boil bambara groundnut prefer the cream-colored seeds (low in tannin) and those in flour production choose the red-colored seeds (high in tannin).(Citation41)
Utilization, market potential, and crop improvement of bambara groundnut have been reviewed previously by Hillocks et al.,(Citation5) who stressed the importance of the crop, but gave limited information on the methods of its processing after the development of the HTC phenomenon. The present review investigates published data on nutritional information and the physical properties of bambara groundnut relative to the development of the HTC phenomenon. For each constituent, the average, minimum, and maximum values are reported after conversion to the same unit. Further, current methods of managing HTC in legumes are categorized as chemical, physical, and biological treatments. Evaluation of the applicability and sustainability of these processing methods is with reference to resource-limited communities. Knowledge on HTC phenomena and assessment of changes that occur during storage of legumes will enable the improvement of current bambara groundnut processing procedures. Finally, formulation of research needs will depend on identified knowledge gaps. Research needs will target to strengthen the knowledge base of this important neglected legume.
Nutritional composition of bambara groundnut seeds
Biochemical analysis of the composition of carbohydrate, fat, and protein reveals that bambara groundnut produces an almost balanced diet.(Citation20,Citation42) – present the average, minimum, and maximum values of chemical composition (i.e., proximate, mineral, and vitamin content) of bambara groundnut seeds, respectively. In comparison to other legumes, bambara groundnut has a lower protein content. Like most legumes, the sulfur containing are the limiting amino acids, such as methionine and cysteine; nevertheless, bambara groundnut seeds contain a relatively high proportion of methionine as a percentage of the protein,(Citation5,Citation9,Citation43–Citation45) and all other essential and nonessential amino acids meet FAO requirements.(Citation27,Citation43) Moreover, the protein score (i.e., amino acid score of the most limiting amino acid) is 80% for bambara groundnut as compared to 74% for soybean, 65% for groundnut, and 64% for cowpea, showing that bambara groundnut has a high protein quality.(Citation45)
Table 1. Proximate composition of bambara groundnut seeds.
Table 2. Mineral composition of bambara groundnut seeds.
Table 3. Vitamin composition of bambara groundnut seeds.(Citation140)
Bambara groundnut contains higher levels of fatty acids (i.e., palmitic and linoleic acids) in comparison to groundnut.(Citation46,Citation47) Furthermore, bambara groundnut contains a substantial quantity of vitamin A, thiamine, riboflavin, niacin, carotene, and trace quantities of ascorbic acid.(Citation48,Citation49) Bambara groundnut contains micronutrients such as zinc and iron,(Citation42) calcium and potassium, but is poor in phosphorus and magnesium.(Citation9) The red seeds have been reported to contain almost twice as much iron as the cream seeds; thus, they are proposed to be valuable in areas where iron deficiency is a problem.(Citation9,Citation50) According to a survey in Northern Ivory Coast, bambara groundnut seeds were useful in treating cases of anemia. Milk (i.e., a watery extract produced from milled bambara groundnut) was reported to compare favorably in flavor and mineral composition with cowpea and soybean milk.(Citation51) A drawback in bambara groundnut and other legumes is the lower bioaccessibility of the proteins and minerals due to antinutrient factors such as tannins, trypsin inhibitors, lectins, and phytates.(Citation42,Citation52,Citation53)
Physical properties of bambara groundnut seeds
Food materials respond in a distinctive, specific way to physical treatments involving mechanical, thermal, electrical, and electromagnetic processes. The response of food materials to processing gives essential quantitative knowledge for the rational design of methods and, in this case, for the estimation of the response of HTC bambara groundnut to different processing methods.(Citation54–Citation57) A better understanding of the way bambara groundnut responds to physical, biological, and chemical treatments allows for optimum design of sustainable processing methods and machinery to ensure maximum utilization of bambara groundnut.(Citation56)
Important physical characteristics of raw and processed bambara groundnut seeds include particle size and shape, mass, volume, particle and bulk density, porosity, rapture strength, and surface area. Physical properties of bambara groundnut and their relation to moisture content have been studied.(Citation58–Citation60) These physical properties differ amongst bambara groundnut landraces and are dependent on inherent seed properties, interactions of chemical components during growth, and seed storage conditions.(Citation61) The physical properties of bambara groundnut landraces have been reported to be indispensable determinants of the behavior of the seeds during cooking and rehydration.(Citation20) Since physical properties are expected to change during processing, recognition of these changes helps to avoid potential processing failures.(Citation56)
Seed coat color and thickness
Seed color and seed coat thickness are important parameters to evaluate in order to recommend the bambara groundnut landraces that should be suitable for boiling and milling for resource limited communities. According to Beninger and Hosfield,(Citation62) the presence of flavonoids (i.e., flavonol glycosides, anthocyanins), condensed tannins (proanthocyanidins), and carotenoids determines the seed coat color of legumes. In addition, concentrations of tannins located mainly in the seed coat correlate with seed color.(Citation41,Citation63) Dark-colored bambara groundnut seeds (i.e., black) contained higher concentrations of tannins as compared to light-colored (i.e., cream) seeds.(Citation20,Citation51,Citation64) presents the average seed coat thickness of bambara groundnut seeds. The relationship between seed color and seed coat thickness was reported.(Citation20) The studied bambara groundnut landraces (cream, brown, maroon, black) from Northern Ghana had distinct variations in the seed sizes and seed coat thicknesses.(Citation20) Two varieties of cream-colored bambara groundnut were found to have smaller seed sizes (thousand seed weight) and thinner seed coats (0.11 and 0.12 mm) as compared to the other three dark-colored varieties (brown, maroon, and black) (0.20 mm). Seed coat thickness was measured using a pearl chrome-plated micrometer and was not significantly (p > 0.05) different for the three dark-colored varieties.(Citation20)
Table 4. Physical characteristics of bambara groundnut seeds.
Seed hardness and rupture strength
Hardness is a parameter that measures the ability of the seed to resist breakage, penetration, and scratching.(Citation56) Grain hardness has been related to kernel density and power requirement during the milling process (i.e., dehulling and size reduction), and the physical characteristics and quality of final products.(Citation65) In addition, a correlation was found between seed hardness and seed weight. The role played by seed hardness in the determination of the milling quality of legume seeds is reported to be due to cell wall structure and composition.(Citation66) Further, rupture strength is the force required to initiate seed rupture and it affects milling performance and water absorption of seeds such that seeds with low rupture strength will soften easily during cooking and are easy to handle in flour production. The difficulty in milling of legumes is due to the presence of fiber (cotyledon cell-wall materials) and the strong binding of large amounts of insoluble proteins to starch.(Citation67)
Linear dimensions
Physical dimensions of seeds such as length, width, and thickness are important in designing postharvest operations of grading and sorting. These linear dimensions determine the particle size of the seed, which is mainly important in determining the required power for size reduction operations and also the quality of the final product.(Citation56) Measuring linear dimensions is important for further determination of geometric average diameter (Dg), sphericity, surface area, volume, and porosity. presents the reported length, width, and thickness of bambara groundnut. The three major perpendicular dimensions of bambara groundnut were measured using a digital Vernier calliper,(Citation54,Citation59) at average moisture contents ranging from (dry basis) 7.2% to 9%(Citation59) and 5% to 35% (wet basis).(Citation54) From these findings, dimensions increased at higher moisture content and were a function of the moisture content of the seeds. The average dimensions of bambara groundnut seeds were length (11.5–12.7 mm), width (9.4–10.6 mm), and thickness (9.2–10.5 mm). The seed size of the bambara groundnut seeds was graded as large (≥10.5 mm), medium (9.50–10.49 mm), and small (<9.50 mm).(Citation59) The Dg of the seed was generally higher for larger seeds. The Dg was calculated using the = Dg formula and ranged from 9.8 to 11.1 mm.(Citation59) Germ lengths constituted about 55–72% of the total seed length, while germ widths made up 50–70% of the seed width.(Citation20,Citation59)
Volume and surface area of seed
According to Baryeh,(Citation54) the volume of bambara groundnut seeds increased with increase in moisture content from 425 mm3 at 5% moisture content to 900 mm3 at 25% moisture content.(Citation54) After 25% moisture content, the volume change was reported to be very little.(Citation54) Surface area is an important physical parameter in thermal processing since heat transfer is proportional to surface area. The dimensions of the seed, namely seed surface area and seed volume, increased nonlinearly with increase in moisture content. It was observed that on water absorption the seeds expanded in length, width, thickness, and geometric diameter within the moisture range of 5–25% (wet basis) and displayed no dimensional change thereafter.(Citation54)
Density of seed
Density of a material expressed in units of mass per unit volume is the amount of that material occupying a certain space.(Citation56) presents the average values of true density and bulk density. At all seed moisture contents studied, true density was higher than bulk density. The true density decreased proportionally when the moisture content increased from 5% to 35%, indicating a lower seed weight increase in comparison to its volume increase when its moisture content increased. Bulk density can be calculated as the ratio of the bulk weight and the volume of the container (kg/L).(Citation54) It is a physical property that affects the structural load and is an important parameter in designing drying and storage equipment.(Citation54) Seed density is the ratio of the weight of a single seed to its volume, whereas the bulk density is the defined amount of seeds in a vessel or a container that also includes the void spaces between the seeds.(Citation54)
Porosity of seed
Porosity of the seed is the ratio of volume of void spaces in the material over the total volume; its value depends on the value of true density and bulk density. The average values of porosity of bambara groundnut seeds are shown in ; porosity variation is not as high as is found in other seeds.(Citation54) The porosity of bambara groundnut seeds was reported to increase nonlinearly to 43.8% at a low moisture content (20%) and then decrease nonlinearly to 40.5% at a high moisture content (35%). Porosity is an important measure in bambara groundnut processing as it influences the size reduction properties and drying rate.(Citation54) Porosity permits air and liquids to flow through a mass of seed particles referred to as a packed bed in drying operations.(Citation56) Packed beds of seeds with low porosity (i.e., a low percentage of air space) are more resistant to fluid flow and have low heat transfer, thus they are more difficult to dry, heat, and cool.
Sphericity of seed
Sphericity of the seed is the measure that indicates deviation of the seed from a perfectly round sphere.(Citation56) The average values of sphericity of bambara groundnut seeds are shown in . Higher values of sphericity (>70%) show less deviation of the shape from a sphere.(Citation68) This parameter is important in determining terminal velocity, the drag coefficient, and the Reynolds number, which are important in dehulling, size reduction, and grading processes. Bambara groundnut seeds are sphere-shaped (oval) and have a high aspect ratio. According to Mpotokwane et al.,(Citation59) the sphericity of bambara groundnut seeds decreased nonlinearly with increase in moisture content. Small-sized seeds had higher sphericity and aspect ratio as compared to large seeds.(Citation59) Seeds that are more spherical occupy more volume. The variation in sphericity is due to inherent seed properties and also the influence of moisture content.(Citation68)
Water absorption
The extent of controlling the HTC phenomenon in legumes can be determined by water absorption and cooking properties. Water absorption and cooking kinetics of seeds are influenced by their intrinsic (i.e., physical and chemical) properties;(Citation60) thus, bambara groundnut varieties with varying compositions will also vary in cooking times.(Citation68) Research on water absorption and cooking characteristics of bambara groundnut is useful since it allows the evaluation of the process in terms of sustainability, nutritional and sensorial quality of the final product.(Citation58) Mathematical modeling of water absorption and cooking processes is applicable in optimizing these processing operations to reduce cooking time of legumes. The Peleg’s, the first order, and the sigmoid models are widely used to study the behavior of legumes during rehydration. A study by Kaptso et al.(Citation60) evaluated the physical properties of bambara groundnut and cowpea varieties (two each). Two varieties of cowpeas (coded GC and WC) and two varieties of bambara groundnuts (coded WB and BB) were purchased from local markets in Ngaoundere (for WB and BB), Garoua (for GC), and Bafoussam (for WC). The study determined the diffusivity of liquid water in the seeds during soaking at different temperatures as well as the required energy of activation. The cowpea varieties (WC and GC) and bambara groundnut seeds (BB and WB) studied had water absorption kinetics that followed the first order, Peleg with a proposed sigmoid model.(Citation60) This means that the absorption curve exhibited a lag phase, which was followed by a phase of a time–linear relationship of water absorption and, finally, one of a slower rate of absorption.
Substantial varietal variations were observed regarding the physical properties (i.e., water absorption kinetics and effective diffusivity) of the seeds.(Citation58,Citation60) The saturation water content was higher for the cowpea varieties (1.39–1.50 g/g) and lower for the bambara groundnut varieties (0.88–1.00 g/g). The proposed sigmoid model significantly described the water absorption kinetics, regardless of the variety and temperature. The effective diffusivity of the seeds was reported to differ in the order of cowpea (GC variety) > bambara groundnut (WB variety) > bambara groundnut (BB variety) > cowpea (WC variety) and increased as the soaking temperature increased from 35 °C to 45 °C. The variation in the effective diffusivity at 25 °C and 35 °C was observed to be linearly correlated (R2 > 0.82; p < 0.05) to the percent seed coat, which suggests that seed coat thickness played a major role in water absorption.(Citation60) In addition, the effective diffusivity varied with temperature according to the Arrhenius equation and the resulting activation energy varied from 78.8 kJ mol–1 for cowpea (GC variety) to 11.2 kJ mol–1 for bambara groundnut (WB variety).(Citation60)
Physical properties responsible for water absorption in cowpeas were studied by Sefa-Dedeh et al.,(Citation69) who found that inherent seed properties (i.e., seed coat thickness, volume, hilum size) and protein content are of importance depending upon rehydration stage. Additionally, Annan et al.(Citation45) reported a significant difference in the rate of water absorption of bambara groundnut varieties with different seed sizes. The same observations were found in sorghum seeds as smaller seeds had higher rates of water absorption as compared to larger seeds.(Citation20) According to Plahar and Annan,(Citation70) for several soybean cultivars studied, the percent seed coat values had a significant effect on the relative time for maximum water absorption. Additionally, Sefa-Dedeh et al.(Citation69) also indicated that seeds with thinner coats have a faster rate of water absorption during the initial soaking period (0–6 hours), after which hydration occurred at the same rate. Enwere and Hung(Citation22) observed that maximum water absorption decreased with increase in temperature; for example, when soaking at 25 °C, water absorption was 11 hours and at 60 °C it decreased to 4 hours. The water absorption profiles were comparable to those reported for cowpeas,(Citation69) with the exception that bambara groundnut took a longer time to reach maximum water absorption.
According to Poulter and Caygill,(Citation71) bambara groundnut seeds had a high water absorption during soaking and doubled weight after approximately 36 hours; at this stage seed coats became loose and were easily removed. A study by Ojimelukwe(Citation64) observed the changes in textural properties of bambara groundnut during cooking. Additionally, the cooking time was shorter for the cream and brown-colored cultivars as compared to the red and black-colored cultivars. Estimation of tannin content of bambara groundnut seeds showed that tannin contents of the red and black seed cultivars (0.96 g CE/100 g and 1.I g CE/100 g, respectively) were considerably higher (p ≤ 0.05) than the tannin contents of cream and brown seed cultivars (0.68 g CE/100 g and 0.72 g CE/100 g, respectively).(Citation64) The higher tannin contents of the red and black seed cultivars are hypothesized to cause the lower protein availability of these seeds as a result of the interactions of tannins with proteins, thus leading to prolonged cooking to ensure edibility of legumes.(Citation64)
Development of HTC phenomena
Legumes are grown, harvested, dried, and then stored until consumption.(Citation31,Citation72) Storage under hot and humid conditions, as encountered in many subtropical and tropical African countries, renders legumes prone to a hardening phenomenon characterized by extended cooking time to ensure adequate softening during cooking.(Citation31,Citation38,Citation73–Citation76) HTC in legumes is associated mainly with modifications that occur in the cotyledons and seed coats.(Citation31,Citation77–Citation79) Several intricate factors, external and internal to the tissue, simultaneously contribute to events leading to the HTC phenomenon.(Citation80) Several mechanisms suggested for the HTC phenomenon in legume seeds are shown in . The factors are categorized as structural (i.e., autolysis of cytoplasmic organelles, weakling plasmalemma integrity, and lignification of middle lamella) and compositional changes (i.e., formation of insoluble pectate, lipid oxidation, and polymerization, phytic catabolism, interactions of proteins and phenolic compounds and polymerization of phenolic compounds, deposition of lignin-like material).(Citation73,Citation78,Citation81,Citation82)
A study by Hincks and Stanley (Citation81) proposed that the HTC phenomenon in legumes proceeds by two mechanisms, namely (i) the ‘pectin-phytate’ and (ii) the ‘lignification’ mechanism, which both occur at high temperatures and humidity. As such, bean hardening during storage is due to phytate hydrolysis and deposition of lignin-like material, which strengthen the cell walls. Structural components associated with HTC development include seed coat, cell walls and membranes, middle lamella and starch granules; and compositionally, phenolic compounds, proteins, nonstarch polysaccharides (NSPs), phytic acid, and lignin.(Citation80) These alterations cause reduced cell separation of HTC legumes during cooking and consequently an extended cooking time.(Citation83)
Microstructure properties in relation to development of HTC phenomena
Scanning electron microscopy provides an in-depth look at the microstructure of plant food materials as affected by changes (i.e., physical and chemical) during postharvest storage and processing.(Citation76,Citation84) The HTC phenomenon is characterized by the restricted softening of the cotyledons upon cooking. The relationship between microscopic properties and development of HTC phenomenon was reported.(Citation76,Citation85) Pirhayati et al.(Citation85) studied the chemical and microstructural changes in HTC pinto bean (P. vulgaris) and easy-to-cook lentil (Lens culinaris). The major structural changes observed under the scanning electron microscope were deteriorations in cytoplasmic contents of the cooked cotyledon cells of hard seeds as compared to limited changes in cooked soft beans.(Citation85)
The comparison of the structural changes of the seed coats of black beans (P. vulgaris) stored for 2 years at ambient conditions (ACs) and refrigerated hypobaric conditions (RHCs) showed a disruption of the parenchymal cell layers and large intercellular spaces between the cell layers of beans stored under AC.(Citation76) Additionally, the middle lamellae were mostly dissolved in soft beans during cooking, but in hard beans they were intact and binding the cells together.(Citation84) The parenchymal cell layers of the beans stored under RHC exhibited slight disruption of the cell layers. Cotyledons of black beans stored under RHC exhibited many large intercellular spaces typical of normal beans. On the contrary, the beans stored under AC exhibited cotyledon cells with few small intercellular spaces typical of HTC beans.(Citation76)
Mafuleka et al.(Citation86) described the changes occurring in decorticated white and red bean (P. vulgaris) during HTC phenomenon development as elevated phytase activities and slight, but nonsignificant increases in lignin levels in both bean genotypes maintained under adverse storage conditions (8 months). The mechanism involving phytic acid degradation appeared to be the dominant system influencing the HTC phenomenon in the white, and to some extent, the red bean genotypes for the storage period of up to 8 months.(Citation86)
Compositional changes in relation to the development of the HTC phenomenon
Several researchers have described the changes in legume hull, cell wall,(Citation87–Citation90) and cotyledon in relation to legume hardening during storage.(Citation38,Citation86,Citation91) In addition, changes in phytase were reported in Malawian white and red bean; phytase was also suggested to be an indicator for cookability of common beans.(Citation86) Further, phenolic compounds (i.e., tannins and phenolic acids) have been noted relative to HTC development in legumes.(Citation77,Citation92) A study by Shiga et al.(Citation93) described the solubilization pattern of carioca bean hull NSPs during soaking and subsequent cooking after storage at 30 °C and 75% RH for 8 months. In another study by Shiga and Lajolo(Citation94) and Shiga et al.,(Citation88) all plant cell wall constituents were reported to have increased during storage (i.e., acid and neutral detergent fibers, lignin, cellulose, and hemicellulose).
Lignification and phenolic compounds
Several studies have reported the changes that occur in lignin, condensed tannins, and phytase during storage and their contribution to HTC development in different legumes such as common beans and cowpea.(Citation37,Citation85,Citation95–Citation98) The lignification mechanisms hypothesize the migration of aromatic compounds from seed coats to cell wall surfaces where they act as precursors in lignification-like reactions. An investigation was undertaken by Hincks and Stanley(Citation81) to establish the potential contribution of lignification in the HTC phenomenon in common bean (P. vulgaris) using scanning electron microscopy. In their findings, cell wall material from hard beans had a lamellate appearance, not observed in the control; thus, cellulose deposition, a precursor of lignification, was implicated. A study by Molina et al.(Citation99) reported an increase in lignified protein in bean cotyledons (P. vulgaris) during storage at ACs (25 °C and 70% RH). This was corroborated by Hincks and Stanley(Citation81) and Srisuma et al.,(Citation92) who reported substantial increases in Klason lignin and lignified protein contents that were correlated to an increase in cooking time. However, Srisuma et al.(Citation92) detected insignificant changes in lignin content during bean hardening and stated a significant increase in free hydroxycinnamic acids, which was hypothetically suggested to be linked with increased hardening.
In a study by Garcia et al.,(Citation73) HTC carioca beans (P. vulgaris L. var. Carioca) were reported to have more phenolics (three times) linked with the soluble-pectic fraction and an increased content of pectates.(Citation73) The researchers proposed that the presence of more hydroxycinnamic acids (i.e., ferulic, p-coumaric, ferulic, and sinapic acid) bound to the soluble pectin,(Citation100) and their probable involvement in cross-linkages, could contribute to changes in cell adherence. Subsequently, this would alter the cell separation upon cooking of HTC beans. These findings were similar to the earlier findings of Garcia and Lajolo(Citation79) and Machado et al.,(Citation100) who observed an increase in the concentration of free phenolic acids in HTC beans, showing that the HTC phenomenon affected potential nutritive properties of dry beans. The analysis of soluble pectin fractions (FT-IR-DRIFTS) by Maurer et al.(Citation80) showed that more phenolic compounds were related with the soluble pectin fraction of HTC carioca beans (red and black) as compared to control carioca beans. The consequence of increased phenolics in the HTC beans was thought to be a reduced cell wall separation during cooking, which ultimately would contribute to prolonged cooking time of HTC beans to reach the same tenderness.(Citation80)
The relationship of storage temperature and cookability of faba bean (Vicia faba) was reported by Nasar-Abbasa et al.(Citation101) and showed significant increases in acid detergent fiber and lignin contents with increasing storage temperatures. In addition, considerable reductions in total free phenolics, particularly in the seed coat, were observed at high-temperature storage (12 months). A study by Pirhayati et al.(Citation85) evaluated the chemical changes of ‘hard-to-cook’ pinto bean and small-type lentil. In the study, phytic acid decreased by 36–61% and total phenolic content by 43–61% during storage, whilst seed hardness increased 3–6 times.
Starch alteration in relation to HTC development
A study by Hohlberg and Stanley(Citation75) proposed that compositional and microstructural modifications occur in black bean (P. vulgaris) starch during storage and that this change is irrespective of the environment. The changes in the starch are one of the contributions in the reduced acceptability of hard beans and a major cause of significant postharvest losses.(Citation31) According to Garcia and Lajolo,(Citation79) an important quality indicator for bean consumers in Latin America is the presence of a thick viscous broth in cooked bean. As such, a less thick viscous broth formed in HTC beans during cooking could relate to changes of starch properties induced by the hot and humid storage conditions. HTC phenomena result in prolonged cooking time, and subsequently a reduction in nutritional value and changes in the sensorial quality due to poor properties of the cooking broth.(Citation79) Furthermore, Garcia and Lajolo(Citation79) reported the changes in starch during bean storage to be an end result of hardening and aging, and not as a contributory agent of HTC development. Scanning electron microscopy of starch granules and seed breakages isolated from hard beans revealed granule resistance to amyloglucosidase attack and a higher degree of crystallinity. Additionally, under polarized light microscopy, starch granules of HTC beans had an increased birefringence as compared to starch granules of the control beans.(Citation79) Moreover, an increase of the gelatinization temperature of starch isolated from HTC beans (5 years storage) was observed from differential scanning calorimeter thermograms, whereas starch isolated from soft bean (control) seeds showed no such change.(Citation79) In a similar study by Hohlberg and Stanley,(Citation75) an increase in starch gelatinization temperature during different storage conditions of black beans was not related to the hardening process. Garcia and Lajolo(Citation79) concluded that the changes in the starch of HTC beans could be an outcome of the hardening process rather than a causative mechanism. However, consumers can use starch alteration as a method of screening the cooking quality of common beans and evaluate its acceptance.
NSPs in relation to HTC
The carbohydrates of bambara groundnut are mainly composed of starch polysaccharides and NSPs (see ) with smaller amounts of reducing and nonreducing sugars.(Citation9) In a study by Brough and Azam-Ali,(Citation43) approximately 12.8% total NSP found (i.e., resistant to acid and enzyme hydrolysis) were suggested to be lignified seed coat material and low-molecular-weight phenolic compounds. The ratio of insoluble to soluble fractions of bambara groundnut NSP was 57:43; with cellulose accounting for 36% of the total NSP. presents the constituent monosaccharides of the individual NSP fractions. The insoluble fraction is predominantly composed of glucose, arabinose, and xylose, whereas uronic acids, xylose, and galactose were the components of the soluble NSP of bambara groundnut.(Citation43) NSPs are present as arabino-xylans, noncellulosic glucose and xylose as xyloglucans since these are typical hemicellulosic components of seeds. In addition, xylans are reportedly contained in the bambara groundnut seed coats, thus dehulling of seeds may affect the digestibility of the flour produced. Dehulling decreases the proportion of xylose in the insoluble fraction, thereby reducing the amount of material resistant to enzyme and acid hydrolysis.(Citation43)
Table 5. Composition of nonstarch polysaccharides (NSP) of bambara groundnut.(Citation43)
Table 6. Composition of sugar constituents of nonstarch polysaccharides (NSP) of bambara groundnut.(Citation43)
The soluble portion of the cell wall was predominantly composed of pectins consisting of galacturonic acid, rhamnose, arabinose, galactose, and uronic acid and side chain molecules linked to the rhamnogalacturonan and arabinogalactan backbones.(Citation43) Previous researches have shown the influence of NSP in the hardening process of legumes.(Citation43) These researchers attributed the changes in microstructure of legume cell wall and polysaccharides during storage to be the major factor in HTC development.(Citation43,Citation88,Citation93) Lower water solubility and lower degradation of galacturonans and arabinose-rich polysaccharides were observed during cooking of aged beans.(Citation94) Additionally, it was proposed that during the cooking process the pectin in the middle lamella is depolymerized by β-elimination of methyl-esterified polygalacturonic acids, promoting an increase in cell separation and legume softening.(Citation85,Citation91)
Protein alteration in relation to HTC development
A study by Hohlberg and Stanley(Citation75) reported a significant increase in proteins (i.e., small polypeptides and free aromatic amino acids) during storage (10 months) of black beans (P. vulgaris) under different environmental conditions. Small polypeptides and free aromatic amino acids are products of large protein hydrolysis. The concurrence in the increase of these simple proteins and development of HTC in beans suggests a possible link between these phenomena.(Citation75) The suggested mechanism is the probable migration of the small polypeptides and free aromatic amino acids to the middle lamella where they are polymerized and lignified, possibly by the action of mobilized enzymes at elevated temperatures. These findings were corroborated by Hussain et al.,(Citation102) who reported on the changes in electrophoretic pattern of black bean cotyledon proteins during storage.
Managing the HTC phenomenon by legume processing
Storage of legumes at low temperature and humidity prevents the occurrence of the HTC phenomenon in legumes.(Citation73,Citation83,Citation103) However, such storage conditions are not within reach of the poorest rural communities of Zimbabwe, who lack the infrastructure and resources to implement these. Therefore, effective and efficient processing technologies are required to transform the HTC bambara groundnuts into edible and nutritious foods.(Citation104) The methods of processing HTC legumes are (i) chemical treatments (cooking aids) (see –), (ii) biological treatments (germination and fermentation), and (iii) physical treatments (milling, roasting, and canning) (see ).
Figure 2. Percentage cooking time reduction of cowpeas soaked and cooked in water, cooked in sodium chloride, 4kanwa, alkali potash, citrate buffer, and alkali buffer solutions.(Citation137) 4Kanwa = natural rock salt used as cooking aid in West Africa.
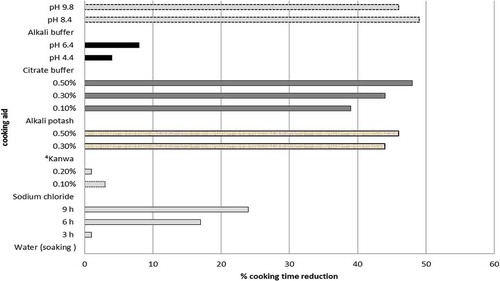
Figure 3. Percentage cooking time reduction of bambara groundnut soaked (0, 3, and 6 hours) and cooked in water, 0.3% 4kanwa and 0.5% kanwa solutions.(Citation2) 4Kanwa = natural rock salt used as cooking aid in West Africa.
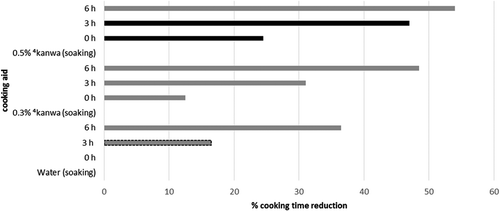
Figure 4. Percentage cooking time reduction of cowpeas cooked in sodium bicarbonate and 4kanwa solutions.(Citation111) 4Kanwa = natural rock salt used as cooking aid in West Africa.
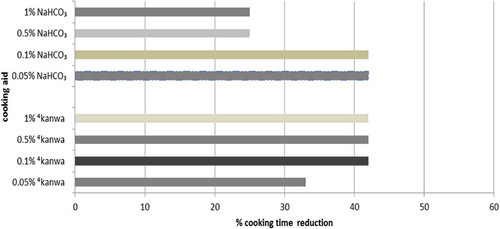
Figure 5. Percentage cooking time reduction of cowpeas cooked in 4kanwa, potassium carbonate, calcium carbonate, sodium carbonate, and sodium bicarbonate solutions. 2CaCO3 = –11%.(Citation114) 4Kanwa = natural rock salt used as cooking aid in West Africa.
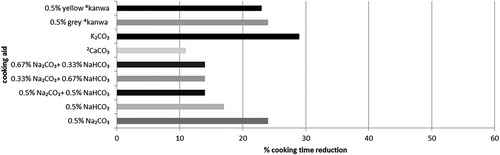
Chemical treatments for managing HTC in legume processing
Effects of cooking aids on cooking time
Previous studies have shown that different salt solutions affect the cooking time of HTC legumes.(Citation2,Citation105,Citation106) Soaking of legumes in the presence of both monovalent (Na+ and K+) and divalent cations (Ca2+ and Mg2+) has been demonstrated to increase the water absorption capacity and subsequently reduce the cooking time of legume seeds.(Citation106,Citation107) A study by de-Leon et al.(Citation107) reported that a salt concentration of 0.5% sodium bicarbonate (NaHCO3) and 2.5% potassium carbonate (KCO3) (w/v) (i.e., a ratio of 8.3:1 of monovalent to divalent cations) was the most effective in increasing bean softening. To optimize the process for bean sensorial properties, the authors suggested the soaking of the beans with a salt solution, thereafter discarding the soaking solution, and cooking with fresh water.(Citation107)
Bellidoa et al.(Citation108) studied the effects of micronization pretreatments on the physicochemical properties of navy and black beans (P. vulgaris L.) by a mixture of different salts (i.e., sodium bicarbonate, sodium carbonate, and dibasic sodium phosphate (Na2HPO4)) and a mixture of acids (i.e., citric and ascorbic acid) and disodium EDTA. The mixture of salts was more effective than the other mixtures in reducing the hardness of micronized black beans.(Citation108) There was no reduction in firmness of navy beans due to EDTA, but EDTA decreased the degree of starch gelatinization. Additionally, higher levels of gelatinized starch and lower-soluble protein levels were significantly associated with a decrease in the hardness of micronized beans.(Citation108) Garcia-Vela et al.(Citation109) described the effects of pH of soaking solutions on the softening of black beans (P. vulgaris); pH of soaking solutions failed to produce significant effects on the softening, but anion type was important in inducing softness. Anion type was effective in the softening process as follows: carbonate was more effective than EDTA (CO3–2 >EDTA–2), and nitrate more effective than sulfate (NO3–1 >SO4–2) and lastly Cl–.(Citation109)
Kanwa (also called trona, kawe, kanwa, potash) is a naturally occurring alkaline rock salt that features in West African populations (Ghana, Nigeria, and Benin) as food tenderizers, for dry beans and other tough foods, with the aim of reducing cooking time.(Citation2,Citation44,Citation110–Citation112) Other kanwa uses include flavoring, preservation, and as a prophylactic agent.(Citation44) Kanwa is sodium sesquicarbonate (Na2CO3, NaHCO3, xH2O) with trace amounts of minerals (namely Ca, Mg, Fe, Zn, S, Si, P, and K). Its composition, color, and flavor vary significantly according to its origin.(Citation44,Citation112) Kanwa is known as gowa in some parts of Zimbabwe, where it is used as a tenderizer in cooking leafy vegetables, common beans, and okra.(Citation113) – give an overview of the cooking time reduction of cowpea and bambara groundnut cooked in different concentrations of cooking aids. A cooking time reduction of up to 42% was achieved upon boiling cowpeas in a 0.5% kanwa solution.(Citation111) Furthermore, a 24% reduction in cooking time of cowpeas cooked in 0.5% gray kanwa and a 29% reduction in 0.5% potassium carbonate solution were also reported.(Citation114) A study by Plahar et al.(Citation2) showed a significant improvement in the cookability of bambara groundnut after cooking with alkaline salts, with an average cooking time reduction of up to 25% upon boiling bambara groundnut in a 0.5% kanwa solution.
Beneficial effects of using salts in cooking have been postulated to be due to both ion exchange and chelation mechanisms occurring between monovalent cations (i.e., Na+ and K+) in solution and divalent cations (Ca2+ and Mg2+) in pectates of the middle lamella.(Citation81,Citation115) This hypothesis is based on the fact that divalent cations can form cross-links between adjacent galacturonic acid residues of pectin molecules in the middle lamella leading to stable structures.(Citation80,Citation109,Citation116) In addition, the mechanism of salt action during the softening process of legumes has also been attributed to a reduction in the protein denaturation temperature caused by carbonate anions.(Citation117) Further, an assumption is that altered pectates have increased water solubility and thermal conductivity, thus facilitating cell separation during cooking of hardened beans.(Citation104) Moreover, the use of salts was reported to improve the water absorption capacity (diffusivity) between beans and its surroundings,(Citation107) and also to increase the water holding capacity of the bean.(Citation116) According to Sefa-Dedeh et al.,(Citation69) water imbibition in legume seeds is dependent compositionally on protein; protein and water interact via ionic and polar groups, which imply that pH and ionic strength of the soaking solution can affect water uptake.(Citation116) Alternatively, according to Uzogara et al.,(Citation105) phenolic compound concentration (tannic acid) was reduced substantially (67%) when cowpeas were cooked in alkaline conditions. Moreover, more phytic acid was lost (27–40%) in beans cooked in sodium bicarbonate as compared to beans cooked in kanwa (11–29%).(Citation105)
Effects of cooking aids on nutritional quality
An evaluation of protein quality (i.e., protein efficiency ratio and digestibility) of HTC beans cooked in salt solutions (0.3:1 and 9.8:1 monovalent to divalent ion ratios) showed significantly lower protein quality at these ratios.(Citation107) The goal of alkaline salt cooking is not only to reduce cooking time but also to retain the sensorial and nutritional quality of food products.(Citation23,Citation44) A combination of high temperature and alkali treatment of proteins has been shown to catalyze the formation of cross-linked amino acid side chains such as lysino-alanine, ornithino-alanine, and lanthionine.(Citation118,Citation119) Moreover, transformations of l-isomers of all amino acids to d-isomers through racemization is favored by high pH, long heat exposure, and steric properties of the various amino acid side chains.(Citation118–Citation120) The presence of d-amino acid residues and lysino-alanine in the protein structure has been shown to decrease digestibility and nutritional quality.(Citation112,Citation118) Cooking cowpeas in kanwa solution was shown to decrease protein quality (i.e., protein efficiency ratio, net protein ratio, and relative net protein ratio).(Citation112)
A study by Madodé et al.(Citation110) showed an insignificant loss of amino acids in 0.5% kanwa cooked cowpeas and an insignificant change in available lysine content. Additionally, the high pH (8–10) and temperature reached during boiling (100 °C) did not lead to the formation of a detectable amount of lysino-alanine. The amount of kanwa added to foods varies with individual preferences and localities and as much as 10% kanwa relative to the weight of cowpeas is reportedly used in parts of Northern Nigeria.(Citation111) The chemical composition (viz., crude lipids and water-soluble fractions) of black beans (P. vulgaris) was evaluated after cooking in kanwa solution.(Citation44) The cooking resulted in a significant reduction of lipids (palmitic, stearic, oleic, or linoleic acids), proteins (essential and nonessential amino acids), and neutral sugars (galactose).(Citation44)
The findings of a study by Uzogara et al.(Citation111) on the quality of cowpeas after boiling in salt solution (kanwa or sodium bicarbonate) were an increased solid leaching, pH, and mineral content of the beans and also the occurrence of dark-colored beans. In comparison, kanwa gave greater increases in some minerals and also resulted in a darker colored product than when cooking in sodium bicarbonate.(Citation111) Pressure cooked beans (in alkaline solutions) produced lighter colored samples and also caused a greater reduction in cooking time.(Citation111) The tenderizing effects of the alkali on cowpeas seemed to be dependent on concentration rather than the pH of the alkaline solutions.(Citation105) Furthermore, Uzogara et al.(Citation105) reported a reduction in the flatus-causing oligosaccharide factors (viz., verbascose, stachyose), which were studied in cooked beans and cowpeas.(Citation105,Citation110)
Physical treatments for managing HTC in bambara groundnut processing
Milling and roasting
The hardening of bambara groundnuts results in hard-to-mill properties, which are a concern to processors, who find it difficult to find proper dehulling and milling techniques.(Citation21,Citation22,Citation40) The hard-to-mill problem is possibly due to the strong attachment between the hulls and cotyledons as a result of the presence of mucilage and gums at the interface.(Citation121,Citation122) The chemical nature of the mucilage plays an important role in the cooking and milling of bambara groundnut. These mucilage and gums are a network of cellulose microfibrils embedded in a matrix of NSP and proteins.(Citation121)
outlines different methods of processing of HTC bambara groundnuts to different products. Studies by Enwere and Hung(Citation22) and Alobo(Citation123) discussed the milling properties of bambara groundnut. Enwere and Hung(Citation22) reported that bambara groundnut seeds reached optimum manual dehulling efficiency when they absorbed 54 g of water/100 g seed.(Citation22) After processes of soaking and dehulling, dehulling efficiency, dehulling losses, and the yield of cotyledons were significantly different between the dry samples and the conditioned samples. Dehulling efficiency for manual dehulling raw bambara groundnut sample was 0%, whereas after mechanical dehulling (by attrition mill) the dehulling efficiency for raw bambara groundnut increased to 70.5%. This value was significantly different from the dehulling efficiencies of the soaked bambara groundnut seeds (81–88%). The dehulling efficiencies and yields of dehulled cotyledons increased with increase in temperature of soaking water.(Citation22) Alobo(Citation123) soaked bambara groundnut during the production of legume akara pastes and the results showed wrinkled seed coats after 20 minutes of soaking, but seed coats were still difficult to remove manually even after 10–15 hours. The seeds had a relatively low initial rate of water uptake and increased in weight by about 2% after 1 hour of soaking. Bambara groundnut seeds had thick seed coats and these appeared to be responsible for the poor water uptake.
The impact of variety on bambara groundnut flour quality was assessed using different varieties of bambara groundnut and three different processing methods.(Citation2) Processing methods studied included (i) a traditional method (simple milling), (ii) a combination of roasting and milling, and (iii) an improved method (heat treatment, dehulling, and milling). For the improved method, cleaned seeds were soaked in water for 30 minutes, boiled for 25 minutes, and dried at 60–65°C in a hot air dryer for 10 hours, and thereafter milled.(Citation2) The study did not evaluate the dehulling and milling efficiency of the flour production processes. Bambara groundnut flour and their protein concentrates produced by roasting were only evaluated for quality (viz., chemical composition and functional properties).(Citation124) However, there was no evaluation of the effects of roasting temperature on milling and dehulling efficiency of bambara groundnut in the study. A study by Abiodun and Adepeju(Citation125) evaluated the effect of milling (dry and wet) on the chemical and antinutritional composition of bambara groundnut flour. Bambara groundnut seeds were processed into flour by (i) raw milling and (ii) boiling (10 minutes), removal of seed coats and fine milling of cotyledons. The seed coats were high in fiber when compared to dehulled cotyledon flour samples. Additionally, raw bambara groundnut flour had a higher phytate content, while the dehulled flour had a lower value.(Citation125)
Canning
Afoakwa et al.(Citation10) studied the modeling (viz., response surface methodology and central composite rotatable design for K=3) of bambara groundnut canning by evaluating the combined effects of blanching, soaking, and sodium hexametaphosphate salt on cooking indicators (viz., moisture, ash, leached solids, phytates, tannins, and hardness). Blanching and soaking of the seeds prior to canning led to increases in water absorption and leaching. In addition, there was a significant decline in phytates, tannins, and hardness. Increase in salt concentration during soaking caused a significant (p ≤ 0.05) decrease in phytates, tannins, and an increase in softening of seeds. Bambara groundnuts have been canned in Zimbabwe and were sold in the more upmarket supermarkets because they were relatively expensive and therefore unaffordable for most low-income earners.(Citation3,Citation7)
Biological treatments for managing HTC in bambara groundnut processing
Soaking and germination
Barimalaa and Anoghalu(Citation127) described the effects of soaking and germination on dehulling properties of Nigerian varieties of bambara groundnut seeds. After germination, little effort was needed to remove tough seed coats. Conversely, a significant effort was still required to remove seed coats even after soaking overnight at room temperature. In contrast, improved dehulling properties and reduced soaking time were observed after hot-soaking of bambara groundnut seeds.(Citation127) The contribution of malting to bambara groundnut milling performance and flour yield was studied.(Citation40) Malting increased flour yield of seeds by promoting the loss of the gummy substance between the cells, alterations in cell wall structure, increasing the friability of the seed, and ultimately improving flour extraction during milling. Solar-dried bambara groundnuts produced higher chaff yields, probably because the tenderization of the cotyledons during germination resulted in binding back during the prolonged drying period. Chaff yield is, therefore, a reflection of the effect of the malting and milling processes.(Citation40) Further, malting had the added advantage of decreasing milling energy, reduction in flatus factors, antinutritional factors, and lessening of the ‘beany’ flavor, which is unpleasant to certain cultures. However, the sensorial attributes, namely a darker color and an altered taste of malted flour, were less acceptable to consumers, and these attributes increased with prolonged malting period. A malting period of 1–2 days and drying at 40–50 °C was recommended to produce acceptable malted flour.(Citation40)
Fermentation
Previous researchers reported changes in composition of bambara groundnut during fermentation.(Citation127–Citation131) According to Fadahunsi and Sanni,(Citation131) changes include an increase in crude protein, soluble solids, soluble protein in bambara groundnut tempe after 40 hour fermentation. The data obtained during tempe production showed an increase in the activities of the enzymes amylase, lipase, and proteinase as fermentation progressed. Ademiluyi and Oboh(Citation132) reported a change in antioxidant activity and polyphenol distribution in bambara groundnut during fermentation. Amadi et al.(Citation133) studied the solid-state fermentation of bambara groundnut to produce a dawadawa type of product using a starter culture of Bacillus licheniformis isolated from naturally fermenting bambara groundnut seeds next to three strains of Rhizopus.(Citation133) Sensory evaluation showed that bambara groundnut would be an acceptable food product in the diet as a good protein supplement as its tempe was comparable (p > 0.5) in taste and texture, but was rated higher (p < 0.05) in color and flavor than soybean tempe.(Citation134)
Conclusion and implications for further research
Constraints to processing and utilization of HTC bambara groundnut still exist in transitional countries such as Zimbabwe. shows the potential of bambara groundnut processing methods and an evaluation of their applicability and sustainability for resource-limited communities. As the world is moving to the era of convenience and timesaving, processing of the HTC bambara groundnut seeds into different convenient products will increase the utilization of the legume.(Citation135) Furthermore, food insecurity remains a challenge for transitional countries, and according to Clover,(Citation136) the solution to food security lies in increasing the availability, accessibility, and adequacy of food for everyone. As such, adopting sustainable processing technologies for bambara groundnut can be a source of community resilience to food insecurity. Previously applied methods such as use of cooking aids, physical treatments, and biological treatments are applicable for transitional countries, but sustainability, safety, and quality of the products need appraisal. It is essential to build on these locally developed practices and indigenous knowledge systems to supply culturally acceptable nutritious foods from bambara groundnut.
Essentially, factors that contribute to legume hardening and their interactions with processing treatments need further investigation. In this regard, the mechanism of alkaline salts in legume softening and the contribution of softening indicators, that is, phenolic compounds, pectin, and protein, in cooking time reduction need further investigation. Further, the potential contribution of cell wall degrading enzymes in reducing legume cooking time needs further appraisal. Moreover, since bambara groundnut seeds are highly valued for their nutritional content, it is essential to evaluate the effects of processing on nutrient bioaccessibility, safety, and consumer acceptance of products. With consumers in mind, it is also imperative to understand the physical properties that govern preferences for bambara groundnut seeds with the background knowledge that, for example, consumers in Botswana, Zimbabwe, and Ghana prefer different landraces. The motivation behind bambara groundnut landrace preference for cooking and milling needs to be assessed.
Funding
The authors thank the Netherlands Fellowship Programmes (grant award CF9152/2013) for providing the funds for this project.
Additional information
Funding
References
- Oniang’o, R.K.; Allotey, J.; Malaba, S.J. Contribution of indigenous knowledge and practices in food technology to the attainment of food security in Africa. J. Food Sci. 2004, 69, 87–91.
- Plahar, W.A.; Annan, N.T.; Larweh, P.M.; Golob, P.; Swetman, T.; Greenhaulgh, P.; Coote, C.; Hodges, R. Marketing and processing of bambara groundnuts (W. Africa). Crop Post Harvest Programme. Food Research Institute (FRI): Accra, Ghana, 2002.
- Makanda, I.; Tongoona, P.; Madamba, R.; Icishahayo, D.; Derera, J. Evaluation of bambara groundnut varieties for off-season production in Zimbabwe. Afr. Crop Sci. J. 2008, 16(3), 175–183.
- Odeny, D.A. The potential of pigeonpea (Cajanus cajan (L.) Millsp.) in Africa. Natural Resources Forum. 2007, 31, 297–305.
- Hillocks, R.J.; Bennett, C.; Mponda, O.M. Bambara nut: A review of utilisation, market potential and crop improvement. Afr. Crop Sci. J. 2011, 20(1), 1–16.
- Mabika, V.; Mafongoya, P. Bambara groundnut Vigna subterrenean. In Proceedings of the workshop on Conservation and Improvement of Bambara Groundnut (Vigna subterranea (L.) Verdc.) Harare, Zimbabwe, 14–16 November 1995.
- de-Kock, C. Bambara Groundnut. In Speciality foods of Africa Pvt Ltd, Harare, Zimbabwe. undated: Zimbabwe.
- Massawe, F.J.; Roberts, J.A.; Azam-Ali, S.N.; Davey, M.R. Genetic diversity in bambara groundnut (Vigna subterranea (L.) Verdc) landraces assessed by Random Amplified Polymorphic DNA (RAPD) markers. Genetic Resources and Crop Evolution. 2003, 50, 737–741.
- Bamshaiye, O.M.; Adegbola, J.A.; Bamishaiye, E.I. Bambara groundnut: An under-utilized nut in Africa. Advances in Agricultural Biotechnology 2011, 1, 60–72.
- Afoakwa, E.O.; Budu, A.S.; Merson, A.B. Response surface methodology for studying the effect of processing conditions on some nutritional and textural properties of bambara groundnuts (Voandzei subterranea) during canning. Int. J. Food Sci. Nutr. 2007, 58(4), 270–281.
- Gunjal, K.; Goodbody, S.; Delbaere, J.; Kenefick, E.; Rammala, V. Crop And Food Security Assessment Mission To Zimbabwe. 2009, FAO, WFP.
- Phillips, J.G.; Cane, M.A.; Rosenzweig, C. ENSO, seasonal rainfall patterns and simulated maize yield variability in Zimbabwe. Agric. Forest Meteorol. 1998, 90, 39–50.
- Proctor, S.; Henson, S.; Loader, R.; Masakure, O.; Brouder, A.; Bhila, L.; Sigauke, N. Facilitating The Effective Production And Marketing Of Processed Food Products By Small-Scale Producers In Zimbabwe. 2000, DFID.
- Amarteifio, J.O.; Tibe, O.; Njogu, R.M. The Nutrient Composition Of Bambara Groundnut Landraces (Vigna Subterreanea, (L.) Verdc.) Cultivated In Southern Africa. Agricultura Tropica Et Subtropica. 2010, 43(1).
- Mkandawire, C.H.; Review of Bambara Groundnut (Vigna subterranea (L.) Verdc.). Prod. in Sub-Sahara Afr. Agric. J. 2007, 2(4), 464–470.
- Azam-Ali, S.N.; Sesay, A.; Karikari, S.K.; Massawe, F.J.; Aguilar-Manjarrez, J.; Bannayan, M.; Hampson, K.J. Assessing the potential of underutilized crop. A case study using bambara groundnut. Expl Agric. 2001, 37, 433–472.
- Swanevelder, C.J. Bambara food for Africa (Vigna subterranea). National Department of Agriculture: Republic of South Africa, 1998.
- Azam-Ali, S.; Aguilar-Manjarrez, J.; Bannayan-Avval, M. A Global Mapping System for Bambara Groundnut Production. In Food and Agricultural Organization of United Nations. 2001.
- Berchie, J.N.; Adu-Dapaah, H.K.; Dankyi, A.A.; Plahar, W.A.; Nelson-Quartey, F.; Haleegoah, J.; Asaf-Agyei, J.N.; Addo, J.K. Practices and constraints in Bambara groundnuts production, marketing and consumption in the Brong Abafo and Upper East regions of Ghana. J. Agro. 2011, 9(3), 111–118.
- Nti, C.A. Effects of bambara groundnut (Vigna subterranea) variety and processing on the quality and consumer appeal for its products. Int. J. Food Sci. Technol. 2009, 44, 2234–2242.
- Ani, D.P.; Umeh, J.C.; Ekwe, K.C. Bambara Groundnut as Panacea for Food Security: Profitability and Production Efficiency in Benue State, Nigeria. In Proc. 2nd Int. Symp. on Underutilized Plants Species “Crops for the Future – Beyond Food Security”, 2013.
- Enwere, N.J.; Hung, Y.C. Some chemical and physical properties of bambara groundnut seeds and products. Int. J. Food Sci. Nutr. 1996, 47, 469–475.
- Mazahib, A.M.; Nuha, M.O.; S., S.I.; Babiker, E.E. Some nutritional attributes of bambara groundnut as influenced by domestic processing. Int. Food Res. J. 2013, 20(3), 1165–1171.
- Linnemann, A.R. Bambara groundnut Vigna subterranean. A review. Abstracts on Tropical Agriculture. 1987, 12(7), 9–25.
- Amarteifio, J.O.; Moholo, D. The Chemical Composition of Four Legumes Consumed in Botswana. J. Food Comp. Analy. 1998, 11, 329–332.
- Onwuka, U.N.; Abasiekong, K.S. Production and evaluation of chocolate bars from roasted and unroasted african breadfruit, and bambara groundnut flours. J. Food Proc. Preserv. 2006, 30, 534–548.
- Aykroyd, W.R.; Doughty, J.; Ann, W. Legumes in human nutrition. Food & Agriculture Org., 1982, pp 45–53.
- El‐Tabey Shehata, A.M. Hard‐to‐cook phenomenon in legumes. Food Rev. Int. 1992, 8(2) 191–221.
- Onayemi, O.; Osibogun, O. Effect of different storage and cooking methods on some biochemical, nutritional and sensory characteristics of cowpea (Vigna unguiculata L. Walp). J. Food Sci. 1986, 51(1), 153–156.
- El‐Moniem, G.M.A. Sensory evaluation and in vitro protein digestibility of mung bean as affected by cooking time. J. Sci. Food Agric. 1999, 79(14), 2025–2028.
- Stanley, D.W. Hard Beans—A Problem for Growers, Processors, and Consumers. HortTechnology. 1992, 2(3), 370–378.
- Furness, C.K. Some Aspects of Fuel-wood Usage and Consumption in African Rural and Urban Areas in Zimbabwe Rhodesia. Suid-Afrikaanse Bosboutydskrif. 1979, 117.
- Bressani, R. Grain quality of common beans. Food Rev. Int. 1993, 9(2), 237–297.
- Cotthem, W.v. National Tree Planting Day – 3rd December 2011. In Environment Africa. 2011, Environment-Africa.
- Vermeulen, S.J.; Campbell, B.M.; Matzke, G.E. The Consumption of Wood by Rural Households in Gokwe Communal Area, Zimbabwe. Hum. Ecol. 1996, 24, 479–491.
- Chazovachii, B.; Chitongo, L.; Ndava, J. Reducing Urban Poverty through Fuel Wood Business in Masvingo City, Zimbabwe: A Myth or Reality. Bangl. e-J. Sociol. 2013, 10(1).
- Reyes-Moreno, C.; Okamura-Esparza, J.; Armienta-Rodelo, E.; Gómez-Garza, R.M.; Milán-Carrillo, J. Hard-to-cook phenomenon in chickpeas (Cicer arietinum L): Effect of accelerated storage on quality. Plant Foods for Human Nutrition 2000, 55, 229–241.
- Coelho, C.M.M.; Bellato, C.d.M.; Santos, J.C.P.; Ortega, E.M.M.; Tsai, S.M. Effect of phytate and storage conditions on the development of the ‘hard-to-cook’ phenomenon in common beans. J. Sci. Food Agric. 2007, 87, 1237–1243
- Martın-Cabrejas, M.A.; Esteban, R.M.; Perez, P.; Maina, G.; Waldron, K.W. Changes in Physicochemical Properties of Dry Beans (Phaseolus vulgaris L.) during Long-Term Storage. J. Agric. Food Chem. 1997, 45, 3223–3227.
- Uvere, P.O.; Uwaegbute, A.C.; Adedeji, E.M. Effects of malting on the milling performance and acceptability of bambara groundnut (Voandzeia subterranea Thouars) seeds and products. Plant Foods Hum. Nutr. 1999, 54.
- Ofori, K.; Kumaga, F.K.; Bimi, K.L. Variation in seed size, protein and tannin content of bambara groundnut Vigna subterranean. Tropical Sci. 2001, 41, 100–103.
- Murevanhema, Y.Y.; Jideani, V.A. Potential of Bambara Groundnut (Vigna subterranea (L.) Verdc) Milk as a Probiotic Beverage—A Review. Crit. Rev. Food Sci. Nutr. 2013, 53, 954–967.
- Brough, S.H.; Azam-Ali, S.N. The Effect of Soil Moisture on the Proximate Composition of Bambara Groundnut (Vigna subterranea (L) Verdc). J. Sci. Food Agric. 1992, 60, 197–203.
- Minkaa, S.R.; Mbofunga, C.M.F.; Gandonb, C.; Bruneteau, M. The effect of cooking with kanwa alkaline salt on the chemical composition of black beans (Phaseolus vulgaris). Food Chem. 1999, 64, 145–148.
- Annan, N.T.; Plahar, W.A.; Nti, C.A. Dessemination of improved bambara groundnut processing technologies through a new coalition arrangement to enhance rural livelihoods in Northen Ghana, in Training of Trainees workshop. 2003: Tamale Ghana.
- Basu, S.; Roberts, J.A.; Azam-Ali, S.N.; Mayes, S. Bambara Groundnut, in Genome Mapping and Molecular Breeding in Plants Pulses, Sugar and Tuber Crops; Kole, C., Ed.; Springer-Verlag: Berlin Heidelberg, 2007; pp 161–173
- Ferrao, J.E.M.; Ferrao, A.M.B.C.; Antunes, A.M.G. A mancarra dos Bijagós (Vigna subterranea). Aspectos do seu valor nutricional. [Bambara groundnut (Vigna subterranea). Aspects of its nutritional value]. Garcia de Orta Série de Estudos Agronómicos. 1987, 14, 35–39.
- Adeyeye, E.I.; Olaleye, A.A.; Adesina, A.J. Food Properties of Hull, Dehulled and Whole Seed Samples of Bambara Groundnut (Vigna Subterranea L. Verdc). Glob. J. Sci. Frontier Res. Chem. 2013, 13(2), 9–28.
- Oyenuga, V.A. Nigeria’s Foods and Feeding stuffs: Their chemistry and nutritive value. Ibadan University Press, 1968; pp 109.
- deKock, C. Bambara Groundnut. Speciality Foods of Africa Pvt Ltd, Harare, Zimbabwe. undated.
- Poulter, N.H. Properties of Some Protein Fractions from Bambara Groundnut [Voandzeia subterranea (L.) Tliouars]. J. Sci. Food Agric. 1981, 32, 44–50.
- Yagoub, A.E.-G.A.; Abdalla, A.A. Effect of Domestic Processing Methods on Chemical Composition, In vitro Digestibility of Protein and Starch and Functional Properties of Bambara Groundnut (Voandzeia subterranea) Seed. Res. J. of Agric. Biol. Sci. 2007, 3(1).
- Adebowale, A.A.; Awolala, F.M.; Fetuga, G.O.; Sanni, S.A.; Adegunwa, M.O. Effect of Soaking Pre-Treatments on Nutritional Composition and Functional Properties of Bambara Groundnut (Vigna Subterranea) Flour. In Proc. 2nd Int. Symp. on Underutilized Plants Species “Crops for the Future – Beyond Food Security”, 2013.
- Baryeh, E.A. Physical properties of bambara groundnuts. J. Food Eng. 2001, 47, 321–326.
- Berk, Z. Food Process Engineering and Technology. Elsevier Inc. 2009.
- Wilhelm, L.R.; Suter, D.A.; Brusewitz, G.H. Food & Process Engineering Technology. Amer Society of Agricultural. 2005.
- Heldman, D.R.; Lund, D. Handbook of Food Engineering. CRS Press, Taylor and Francis Group, 2007.
- Jideani, V.A.; Mpotokwana, S.M. Modeling of water absorption of Botswana bambara varieties using Peleg’s equation. J. Food Eng. 2009, 92, 182–188.
- Mpotokwane, S.M.; Gaditlhatlhelwe, E.; Sebaka, A.; Jideani, V.A. Physical properties of bambara groundnuts from Botswana. J. Food Eng. 2008, 89, 93–98.
- Kaptso, K.G.; Njintang, Y.N.; Komnek, A.E.; Hounhouigan, J.; Scher, J. Mbofung, C.M.F.; Physical properties and rehydration kinetics of two varieties of cowpea (Vigna unguiculata) and bambara groundnuts (Voandzeia subterranea) seeds. J. Food Eng. 2008, 86, 91–99.
- Aguilera, J.M.; Stanley, D.W. A Review of textural defects in cooked storage and processing reconstituted legumes - The Influence of structure and composition. J. Food Process. Preserv. 1985, 9, 145–169.
- Beninger, C.W.; Hosfield, G.L. Antioxidant activity of extracts, condensed tannin fractions, and pure flavonoids from Phaseolus vulgaris L. seed coat color genotypes. J. Agric. Food Chem. 2003, 51(27), 7879–7883.
- Caldas, G.V.; Blair, M.W. Inheritance of seed condensed tannins and their relationship with seed-coat color and pattern genes in common bean (Phaseolus vulgaris L.). Theor. Appl. Genet. 2009, 119(1), 131–142.
- Ojimelukwe, P.C. Cooking characteristics of four cultivars of bambara groundnuts seeds and starch isolate. J. Food Biochem. 1998, 23, 109–117.
- Otto, T.; Baik, B.-K.; Czuchajowska, Z. Microstructure of seeds, flours, and starches of legumes. Cereal Chem. 1997, 74(4), 445–451.
- Tyler, R.T. Impact milling quality of grain legumes. J. Food Sci. 1984, 49(3), 925–930.
- Reichert, R.; Oomah, B.; Youngs, C. Factors affecting the efficiency of abrasive‐type dehulling of grain legumes investigated with a new intermediate‐sized, batch dehuller. J. Food Sci. 1984, 49(1), 267–272.
- Dahiya, P.K.; Linnemann, A.R.; Van Boekel, M.A.J.S.; Khetarpaul, N.; Grewal, R.B.; Nout, M.J.R. Mung bean: Technological and nutritional potential. Crit. Rev. Food Sci. Nutr. 2013, 55(5), 670–688.
- Sefa-Dedeh, S.; Stanley, D.W.;Voisey, P.W. Effect of storage time and conditions on the hard-to-cook defect in cowpeas (Vigna unguiculata). J. Food Sci. 1979, 44.
- Plahar, W.A.; Annan, N.T. Evaluation of soybean cultivars for traditional food uses in Ghana: Varietal effects on quality characteristics of soymilk and full-fat soy flour extracts. In NARP Project Report. Food Research Institute: Accra, Ghana, 1996.
- Poulter, N.H.; Caygill, J.C. Vegetable milk processing and rehydration characteristics of bambara groundnut (Voandzeia subterranea (L.) Thouars). J. Sci. Food Agric. 1980, 31, 1158–1163.
- Arogba, S.S.; Abu, J.D. Environmental variables affect the hard-to-cook phenomenon of cowpea (Vigna unguiculata) seed. Plant Foods Hum. Nutr. 1999, 53, 305–311.
- Garcia, E.; Filisetti, T.M.C.C.; Udaeta, J.E.M.; Lajolo, F.M. Hard-to-cook beans (Phaseolus vulgaris): Involvement of phenolic compounds and pectates. J. Agric. Food Chem. 1998, 46, 2110–2116.
- Hincks, M.J.; McCannel, A.; Stanley, D.W. Hard-to-cook defect in black beans. Soaking and cooking processes. J. Agric. Food Chem. 1987, 35(4), 583–588.
- Hohlberg, A.I.; Stanley, D.W. Hard-to-cook defect in black beans. Protein and starch considerations. J. Agric. Food Chem. 1987, 35, 571–576.
- Berrios, J.d.J.; Swanson, B.G.; Cheong, W.A. Structural characteristics of stored black beans (Phaseolus Vulgaris L.). Scanning 1998, 20, 410–417.
- Stanley, D.W. A possible role for condensed hardening tannins in bean. Food Res. Int. 1992, 25, 187–I 192.
- Stanley, D.W.; Aguilera, J.M. A review of textural defects in cooked reconstitued legumes: the influence of structure and composition. J. Food Biochem. 1985, 9, 277–323.
- Garcia, E.; Lajolo, F.M. Starch alterations in hard-to-cook beans (Phaseolus vulgaris). J. Agric. Food Chem. 1984, 42, 612–615.
- Maurer, G.A.; Ozen, B.F.; Maurer, L.J.; Nielsen, S.S. Analysis of hard-to-cook red and black common beans using fourier transform infrared spectroscopy. J. Agric. Food Chem. 2004, 52, 1470–1477.
- Hincks, M.J.; Sanley, D.W. Lignification: Evidence for a role in hard to cook beans. J. Food Bioch. 1986, 11, 41–58.
- Aguilera, J.M.; Rivera, R. Hard-to-cook defect in black beans: Hardening rates, water imbibition and multiple mechanism hypothesis. Food Res. Int. 1992, 25, 101–108.
- del-Valle, J.M.; Stanley, D.W. Reversible and irreversible components of bean hardening. Food Res. Int. 1995, 28(5), 455–463.
- Aguilera, J.M. Why food microstructure. J. Food Eng. 2005, 67, 3–11.
- Pirhayati, M.; Soltanizadeh, N.; Kadivar, M. Chemical and microstructural evaluation of ‘hard-to-cook’ in legumes (pinto bean and small-type lentil. Int. J. Food Sci. Technol. 2011, 46, 1884–1890.
- Mafuleka, M.M.; Ott, D.B.; Hosfield, G.L.; Uebersax, M.A. The role of phytase and lignin in decorticated dry bean (Phaseolus vulgaris) hardening during storage. J. Food Process. Preserv. 1993, 17, 1–20.
- Afoakwa, E.O.; Sefa-Dedeh, S. Changes in cell wall constituents and mechanical properties during post-harvest hardening of trifoliate yam Dioscorea dumetorum (Kunth) pax tubers. Food Res. Int. 2002, 35, 429–434.
- Shiga, T.M.; Lajolo, F.M.; Filisetti, T.M.C.C. Changes in the cell wall polysaccharides during storage and hardening of beans. Food Chem. 2004, 84, 53–64.
- Shomer, I.; Paster, N.; Lindner, P.; Vasiliver, R. The role of cell wall structure in the hard to cook phenomenon in beans (Phaseolus vulgaris). Food Struc. 1990, 9, 139–145.
- Yousif, A.M.; Deeth, H.C.; Caffin, N.A.; Lisle, A.T. Effect of storage time and conditions on the hardness and cooking quality of Adzuki (Vigna angularis). Lebensm.-Wiss. u.-Technol. 2002, 35, 338–343.
- Shiga, T.M.; Cordenunsi, B.R.; Lajolo, F.M. Effect of cooking on non-starch polysaccharides of hard-to-cook beans. Carbohydr. Polym. 2008, 76, 100–109.
- Srisuma, N.; Hammerschmdt, R.; Uebersax, M.A.; Ruengsakulrach, S.; Bennink, M.R.; Hosfield, G.L. Storage induced changes of phenolic acids and the development of hard-to-cook in dry beans (Phaseolus vulgaris, var. Seafarer). J. Food Sci. 1989, 54(2), 311–318.
- Shiga, T.M.; Cordenunsi, B.R.; Lajolo, F.M. The effect of storage on the solubilization pattern of bean hull non-starch polysaccharides. Carbohydrate Polymers. 2011, 83, 362–367.
- Shiga, T.M.; Lajolo, F.M. Cell wall polysaccharides of common beans (Phaseolus vulgaris L.)—composition and structure. Carbohydr. Polym. 2006, 63, 1–12.
- Medoua, G.N.; Mbofung, C.M.F. Hard-to-cook defect in trifoliate yam Dioscorea dumetorum tubers after harvest. Food Res. Int. 2006, 39, 513–518.
- Medoua, G.N.; Mbome, I.l.L.; Agbor-Egbe, T.; Mbofung, C.M.F. Study of the hard-to-cook property of stored yam tubers (Dioscorea dumetorum) and some determining biochemical factors. Food Res. Int. 2005, 38, 143–149.
- Rocha-Guzmana, N.E.; J.A. Gallegos-Infantea; R.F. Gonzalez-Laredoa; P.A. Castillo-Antonio; E. Delgado-Licona; Ibarra-Perez, F. Functional properties of three common bean (Phaseolus vulgaris) cultivars stored under accelerated conditions followed by extrusion. LWT. 2006, 39, 6–10.
- Narasimha, H.V.; Srinivas, T.; Dsesikachar, H.S.R. A histological basis for ‘hard-to-cook’ phenomenon in red gram (Cajanus calan) cultivars. J. Food Sci. 1989, 54(1), 125–131.
- Molina, M.R.; Baten, M.A.; Gomez-Brenes, R.A.; King, K.W.; Bressani, R. Heat treatment: A process to control the development of the hard to cooh phenomena in black beans (Phaseolus vulgaris). J. Food Sci. 1976, 41, 661–666.
- Machado, C.M.; FerruziI, M.G.; Nielsen, S.S. Impact of the hard-to-cook phenomenon on phenolic antioxidants in dry beans (Phaseolus vulgaris). J. Agric. Food Chem. 2008, 56, 3102–3110.
- Nasar-Abbasa, S.M.; Plummera, J.A.; Siddique, K.H.M.; White, P.; Harris, D.; Dods, K. Cooking quality of faba bean after storage at high temperature and the role of lignins and other phenolics in bean hardening. LWT. 2007, 1260–1267.
- Hussain, A.; Watts, B.M.; Bushuk, W. Hard-to-cook phenomenon in beans: Changes in protein electrophoretic patterns during storage. J. Food Sci. 1989, 54(5), 1367–1368.
- Hentges, D.L.; Weaver, C.M.; Nielsen, S.S. Changes of selected physical and chemical components in the development of the hard-to-cook bean defect. J. Food Sci. 1991, 56(2).
- Moreno, C.R.; López, O.P.;Gonzalez, E. Hard to cook phenomenon in common beans — A review. Crit. Rev. Food Sci. Nutr. 1993, 33(3), 227–286.
- Uzogara, S.G.; Morton, I.D.; Daniel, J.W. Changes in some antinutrients of cowpeas (Vigna unguiculata) processed with ‘kanwa’ alkaline salt. Plant Foods for Hum. Nutr. 1990, 40, 249–258.
- Kyriakidis, N.B.; Apostolidis, A.; Papazoglou, L.E.; Karathanos, V.T. Physicochemical Studies of Hard-to-Cook Beans (Phaseolus vulgaris). J. Sci. Food Agric. 1997, 74, 186È192.
- de-Leon, L.F.; Elias, L.G.; Bressani, R. Effect of salt solutions on the cooking time, nutritional and sensory characteristics of common beans (Phaseolus vulgaris). Food Res. Int. 1992, 25, 131–136.
- Bellidoa, G.; Arntfielda, S.D.; Cenkowskib, S.; Scanlon, M. Effects of micronization pretreatments on the physicochemical properties of navy and black beans (Phaseolus vulgaris L.). LWT. 2006, 779–787.
- Garcia-Vela, L.A.; del-Valle, J.M.; Stanley, D.W. Hard-to-cook defect in black beans: The effect of soaking in various aqueous salt solutions. Can. Inst. Sd. Technol. J. 1991, 14, 60–67.
- Madodé, Y.E.; Houssou, P.A.; Linnemann, A.R.; Hounhouigan, D.J.; Nout, M.J.R.; Boekel, M.A.J.S.V. Preparation, consumption, and nutritional composition of West African cowpea dishes. Ecol. Food Nutr. 2011, 50, 115–136.
- Uzogara, S.G.; Morton, I.D.; Daniel, J.W.; Quality Changes and Mineral Content of Cowpea (Vigna unguiculata L. Walp) Seeds Processed with ‘Kanwa’ Alkaline Salt. Food Chem. 1988, 30, 1–18.
- Uzogara, S.G.; Mortona, I.D.; Daniela, J.W.; Emerya, P.W. Effect of ‘Kanwa’ treatment of cowpea (Vigna unguiculata) seed flour on protein quality as measured by growth rate assays in rats. Ecol. Food Nutr. 1991, 25(1), 79–89.
- CTDT. Consumption of Indegenous foods in Zimbabwe. Users guide and recipe book. International Development Research Centre, undated; pp 32–37.
- Madode, Y.E.; Houssou, P.A.; Linnemann, A.R.; Hounhouigan, D.J.; Nout, M.J.;Van Boekel, M.A. Preparation, consumption, and nutritional composition of west African cowpea dishes. Ecol Food Nutr. 2011, 50(2), 115–136.
- Liu, K.; Bourne, M.C. Cellular, biological, and physicochemical basis for the hard to cook defect in legume seeds. Crit. Rev. Food Sci. Nutr. 1995, 35(4), 263–298.
- Garcia-Vela, L.A.; Stanley, D.W. Water-holding capacity in hard-to-cook beans (Phaseolus vulgaris): Effect of pH and ionic strength. J. Food Sci. 1989, 54(4), 1080–1081.
- del-Valle, J.M.; Cottrell, T.J.; Jackman, R.L.; Stanley, D.W. Hard-to-cook defect in black beans: the contribution of proteins to salt soaking effects. Food Res. Int. 1992, 25, 429–436.
- Friedman, M.; Chemistry, biochemistry, nutrition, and microbiology of lysinoalanine, lanthionine, and histidinoalanine in food and other proteins. J. Agric. Food Chem. 1999, 47(4), 1295–1319.
- Friedman, M.; Levin, C.E.; Noma, A.T. Factors governing lysinoalanine formation in soy proteins. J. Food Sci. 1984, 49(5), 1282–1288.
- Karayiannis, N.; Macgregor, J.T.; Bjeldanes, L.F. Lysinoalanine formation in alkali-treated proteins and model peptides. Food Cosm. Toxicol. 1979, 17(6), 585–590.
- Sreerama, Y.N.; Sashikala, V.B.; Pratape, V.M. Effect of enzyme pre-dehulling treatments on dehulling and cooking properties of legumes. J. Food Eng. 2009, 92, 389–395.
- Brough, S.H.; Azam-AIi, S.N.; Taylor, A.J. The potential of bambara groundnut (Vigna subterranea) in vegetable milk production and basic protein functionality systems. Food Chem. 1993, 47, 277–283.
- Alobo, A.P. Production and organoleptic assessment of akara from bambara groundnut (Voandzeia subterranea (L.) Thouars). Plant Foods Hum. Nutr. 1999, 53, 313–320.
- Yusuf, A.A.; Ayedun, H.; Sanni, L.O. Chemical composition and functional properties of raw and roasted Nigerian benniseed (Sesamum indicum) and bambara groundnut (Vigna subterranean. Food Chem. 2008, 111, 277–282.
- Abiodun, A.O.; Adepeju, A.B. Effect of processing on the chemical, pasting and anti-nutritional composition of bambara nut (Vigna subterranea L. Verdc) flour. Adv. J. Food Sci. Technol. 2011, 3(4), 224–227.
- Emmambux, M.N.; Taylor, J.R.N. Morphology, physical, chemical, and functional properties of starches from cereals, legumes, and tubers cultivated in Africa: A review. Starch/Starke. 2013, 65, 715–729.
- Barimalaa, I.S.; Anoghalu, S.C. Effect of processing on certain antinutrients in bambara groundnut (vigna subterranea) cotyledons. J. Sci. Food Agric. 1997, 73, 186–188.
- Fadahunsi, I.F.; Olubunmi, P.D. Microbiological and enzymatic studies during the development of an ‘Iru’ (a local Nigerian indigenous fermented condiment) like condiment from Bambara Nut [Voandzeia subterranea (L) Thours]. Malay. J. Microbiol. 2010, 6, 123–126.
- Oboh, G.; Ademiluyi, A.O.; Akindahunsi, A.A. Changes in polyphenols distribution and antioxidant activity during fermentation of some underutilized legumes. Food Sci. Tech. Int. 2009, 15(41), 41–46.
- Fadahunsi, I.F. The Effect of Soaking, Boiling and Fermentation with Rhizopus oligosporus on the Water Soluble Vitamin Content of Bambara Groundnut. Pak. J. Nutr. 2009, 8(6), 835–840.
- Fadahunsi, I.F.; Sanni, A.I. Chemical and biochemical changes in bambara nut (Voandzeia subterranea (L) Thours) during fermentation to tempeh. Electr. J. Envir. Agric. Food Chemi. 2010, 9(2), 275–283.
- Ademiluyi, A.O.; Oboh, G. Antioxidant properties of condiment produced from fermented bambara groundnut (Vigna subterranean L. Verdc). J. Food Biochem. 2011, 35, 1145–1160.
- Amadi, E.N.; Barimalaa, I.S.; Omosigho, J. Influence of temperature on the fermentation of bambara groundnut (Vigna subterranea) to produce a dawadawa-type product. Plant Foods for Hum. Nutr. 1999, 54, 13–20.
- Amadi, E.N.; Uneze, R.; Barimalaa, I.S.; Achinewhu, S.C. Studies on the production of bambara groundnut (Vigna subterranea) tempe. Plant Foods for Hum. Nutr. 1999, 53, 199–208.
- Lim, T. Vigna subterranea. In Edible Medicinal And Non-Medicinal Plants. Springer, 2012; pp 960–966.
- Clover, J. Food Security in sub-Saharan Africa. African Security Rev. 2003, 12(1), 5–15.
- Onayemi, O. Osibogun, O. Effect of different storage and cooking methods on some biochemical, nutritional and sensory characteristics of cowpea (Vigna unguiculata L. Walp). J Food Sci. 1986, 51(1), 153–156.
- Abu-Salem, F.M. Abou-Arab, A.A. Effect of supplementation of Bambara groundnut (Vigna subterranean L.) flour on the quality of biscuits. Afri. J. Food Sci. 2011, 5(7), 376–383.
- Ijarotimi, O.S. Nutritional composition, microbial status, functional and sensory properties of infant diets formulated from cooking banana fruits (Musa spp, ABB genome) and fermented bambara groundnut (Vigna subterranean L. Verdc) seeds. Nutr Food Sci. 2008, 38(4).
- Kone, M.; Paice, A.G. Toure, Y. Bambara Groundnut [Vigna subterranea (L.) Verdc. (Fabaceae)] Usage in Human Health, in Nuts & Seeds in Health and Disease Prevention. Elsevier, 2011; pp 190–195.
- Nwokolo, E. A nutritional assessment of African Yam bean Sphenostylis stenocarpa (Hochst ex A. Rich) harms and Bambara Groundnut Voandzeia subterranea L. J. Sci. Food Agric. 1987, 41, 123–129.
- Ijarotimi, O. Olopade, A. Determination of amino acid content and protein quality of complementary food produced from locally available food materials in Ondo State, Nigeria. Malay. J Nutri. 2009, 15(1), 87–95.
- Amarteifio, J.O.; Karikari, S.K.Modise, O.J. The proximate composition and mineral composition of six landraces of Botswana bambara groundnut. Trop. Sci. 2002, 42, 188–191
- Amarteifio, J.O.; Tibe, O.; Njogu, R.M. The mineral composition of bambara groundnut (Vigna subterranea (L) Verdc) grown in Southern Africa. Afri. J. Biotechnol. 2006, 5(23), 2408–2411.
- Ijarotimi, O. Olopade, A. Determination of amino acid content and protein quality of complementary food produced from locally available food materials in Ondo State, Nigeria. Malay. J Nutri. 2009, 15(1), 87–95.