ABSTRACT
Blackcurrants are nutrient-rich fruits with a significant amount of bioactive compounds including vitamin C and polyphenols, especially anthocyanins. The high phytochemical content of blackcurrants promotes this fruit to become a valuable functional food ingredient with varying health-promoting activities targeting different consumers including athletes. Athletes experience oxidative stress during intense exercise, which can result in inflammation and reduced exercise performance. Antioxidants such as vitamin C and polyphenols can restore the regular oxidative status of the body. Blackcurrant supplementation has shown potential ergogenic activity to improve athlete performance during high-intensity training. Clinical trials have evaluated the effectiveness of blackcurrant supplementation on exercise performance, fat oxidation, blood lactate levels, muscle fatigue, and cardiac output. Due to the rich nutritional value of blackcurrants, they can be a potential candidate for the development of functional foods targeted at the improved performance of athletes. Blackcurrants can be used as ingredients to develop functional beverages and snacks for athletes as well as gluten-free products for celiac athletes.Blackcurrant is rich in bioactive compounds that can help improve athletic performance. It can be considered a potential bioactive ingredient to develop functional foods for athletes.
Introduction
As a species of the family Grossulariaceae, blackcurrant (BC; Ribes nigrum) is a small dark purple fruit that comes from medium-sized woody shrubs, native to colder climate areas such as northern Europe, Northern Asia, and Central Asia.[Citation1] In Europe, BC is commonly consumed with an annual production of about 180,000 tons, and Poland is the primary exporter worldwide of fresh and processed fruits.[Citation2]
The nutritional composition of BC has been widely studied regarding the growing condition and several phytochemicals have been investigated, such as phenolic compounds,[Citation3] vitamin C [Citation4] (), carotenoids, and phytosterols,[Citation5] both in fresh berries and in berry-derived products,[Citation6] as well as in by-products.[Citation7] In a recent paper, BC European cultivars have been studied by ultra-performance liquid chromatography and tandem mass spectrometry and it was found that anthocyanins were the most dominant group of polyphenols, being the highest cyanidin and delphinidin derivatives, especially delphinidin-3-O-rutinoside.[Citation5]
Figure 1. Chemical structures of phytonutrients present in blackcurrant fruit. The components of this figure are not associated with any copyrights (public domain).
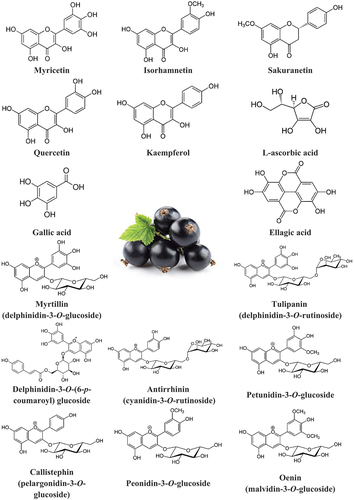
The high nutrient content of BCs has given this fruit a wide range of health-promoting benefits that have been investigated in vitro and in vivo. Two key bioactive properties of BC nutrients are antioxidant and anti-inflammatory activities. BC extracts have shown significant reducing power, and hydroxyl, superoxide anion, 20-azino-bis (3-ethlylbenzothiazoline-6-sulfonic acid), and 1,1-diphenyl-2-picrylhydrazyl radical scavenging activities based on in vitro experiments.[Citation8,Citation9] In addition, BC extracts have shown superior anti-inflammatory activity when compared to chokeberry, rosehip, and hawthorn berries in terms of inhibitory potential of pro-inflammatory enzymes (cyclooxygenase-1 and cyclooxygenase-2).[Citation10] Furthermore, BC supplementation has been successful in increasing the activation of nuclear factor kappa B (NF-κB) in the liver of transgenic mice.[Citation11]
To validate the efficacy of BC extracts on physio-pathological parameters, many clinical trials have been carried out. First of all, substantial research evidenced that the polyphenol-rich juice supported the prevention of cardiovascular disease,[Citation12] and significantly reduced systolic blood pressure (BP) in both normotensive and hypertensive subjects,[Citation13] with the effect being more significant in the latter group.[Citation14] Moreover, in older adults, intake of BC extracts led to significant decreases in systolic and diastolic BP, but there was no effect on 6-minute walk performance or cognitive function variables.[Citation15] Other implications, mainly associated with the berry-derived polyphenolic compounds, have been found to have beneficial effects on postprandial glucose metabolism and oxidative stress, as well as in inflammatory processes and allergies.[Citation16] In recent research, 75 g of BC containing all the berry’s natural constituents reduced sugar-induced excursions, decreased postprandial glycemia and insulinemia, and normalized non-esterified fatty acid levels.[Citation17]
Generally, natural compounds extracted from berry fruits have been studied for antioxidant capacity in an athlete’s performance.[Citation18] The relationship between BC and exercise has also been investigated. Exercise-induced reactive oxygen and nitrogen species (RONS) are unavoidable by-products of increased oxidative metabolism and superoxide anion (O2•–) and nitric oxide (NO). However, RONS are the main free radicals that initiate reactions with other molecules to produce additional reactive free radicals.[Citation19] Although once assumed to be harmful to health, it has recently been shown that these reactive species play a critical role in the body’s adaptive response to exercise, since inhibiting RONS with large doses of dietary antioxidants removed the beneficial effects of exercise.[Citation20] However, severe and prolonged exercise during overtraining increases the formation of muscular damage and induces a detrimental redox environment, resulting in decreased exercise capacity and health.[Citation21]
The rich nutritional composition of BCs and their significant antioxidant activity make them a potential ingredient for developing functional foods targeting athletes. The most common side effect of intensive exercise across all sports fields is oxidative stress. Oxidative stress is a result of an imbalance of the RONS levels in the cells and tissues with the capability of the body to detoxify these harmful reactive species.[Citation22] Natural antioxidants can scavenge RONS and eliminate their harmful effects that could negatively influence the athlete’s performance. In this review, the nutritional composition and bioactive content of BCs are reviewed focusing on their beneficial potential to enhance athletes’ performance. In addition, the current clinical-based evidence of the sports applications of BC supplementation is discussed and evaluated. Finally, the potential BC-based functional food products that could form part of diets useful for enhancing athletes’ performance are discussed.
Nutritional composition of blackcurrants
Carbohydrates
BCs contain reduced amounts of sugar compared to other fruits. Total sugars are commonly present in the range of 7–10 g/100 g and are comprised of 45% fructose, 40% glucose and 15% sucrose. The differences in cultivars account for minor differences in terms of fructose, which is always higher in concentration, while glucose and sucrose may vary more widely.[Citation23] BC concentrates available on the market have higher amounts of sugars (from 20 to 30 g/100 g), with an absence of sucrose that may be the result of inversion, which was recorded to occur in blackberry and apple concentrates.[Citation24] In fact, the carbohydrate content of BC pomace is 2.3% of dry matter according to the reported global composition of BC pomace.[Citation25] Polysaccharides extracted from BC have exhibited strong antioxidant activity based on in vitro assays.[Citation8]
The architectural elements of BCs are pervaded by carbohydrates structures, and it has been found that a peculiar polysaccharide-rich substance is present, in addition to monomeric sugars. This substance consists of chains of rhamnose, mannose, arabinose, galactose, xylose, and glucose in a molar ratio of 11.3:0.9:54.1:29.8:2.0:1.9.[Citation26] Another study has reported the molar ratio of 0.32:0.19:1.00:0.31:0.66:0.09 for rhamnose, mannose, arabinose, galactose, galacturonic acid, and glucose in BC.[Citation27]
In combination with organic acids, sugars are responsible for the taste of these berries, which are highly acidic compared with other species of currants.[Citation2] In fresh fruits, the amount of these acids is reported to be around 5–6%, rising to 16–25% in BC concentrates.[Citation23,Citation24] Organic acids present in BCs are mainly citric acid, which alone represents 75–97% of this category, followed by malic, quinic, and ascorbic acids.[Citation5] Some studies also report minor quantities of succinic, tartaric, and salicylic acids. Titratable acids such as phosphoric acid are present at an additional 3–5% concentration in fresh fruits.[Citation28,Citation29]
Among non-digestible carbohydrates, the dietary fibre in BC has been shown to be represented in an optimal ratio between soluble and insoluble types. BC pomaces at about 5% moisture were reported to present considerable amounts of dietary fibre with soluble fibre ranging from 25 to 30% w/w and insoluble fibre accounting for about 47% w/w.[Citation30] The most represented fibres in this fruit are pectin (acid-soluble and calcium-bound), hemicellulose, cellulose, and lignin.
Based on the reported global composition, BC pomace contains a sum of 61.6% of the fibre in dry matter content that is divided between cellulose (18.2%), hemicellulose (8.8%), and lignin (34.6%).[Citation25]
Lipids
The total amount of lipids in BCs is only 0.41 g/100 g of fresh fruit.[Citation31] However, according to the reported global composition of BC pomace, it contains 14.8% of lipids in dry matter content.[Citation25] According to the chromatographic analysis, BC lipids are mainly comprised of three fatty acids: palmitic, oleic, and linoleic acid ().[Citation25]
Proteins
The amount of protein present in BCs is limited. In the pomace, with around 5% moisture, the relative presence of proteins is between 11 and 13%, while the USDA dataset reports that proteins account for 1.5 g/100 g of fresh weight.[Citation30,Citation31] An example of BC proteins is arabinogalactan proteins, which have galactose and arabinose residues and may additionally include xylose carbohydrate residues as well.[Citation32] In addition, BC pomace contains 16.9% of proteins in dry matter content according to the reported global composition.[Citation25] The amino acid profile of BC pomace detected 18 amino acids, with glutamic acid being the most dominant ().[Citation25]
Table 1. Amino acid profile of blackcurrant pomace.
Vitamins and minerals
BCs are excellent sources of ascorbic acid and less than a portion of 100 g of these fruits are likely to fulfil the daily requirements of vitamin C. Multiple studies investigated the range of concentration of this vitamin among cultivars: a review in 2012 reported an average content of 125–151 mg/100 g fresh weight.[Citation33] Among the different BC cultivars harvested from 2001 to 2005 in Denmark, vitamin C content ranged from 96 to 219 mg/100 g [Citation34] while in another study it was reported to vary between 67 and 204 mg/100 g.[Citation5] Compositional mean data for vitamins and minerals in fresh BC are not fully reported in the literature and the most complete data are found in EFSA nutritional values database.
In addition to vitamin C, BCs are also sources of thiamine (0.05 mg/100 g), riboflavin (0.05 mg/100 g), niacin (0.53 mg/100 g), vitamin K (30 μg/100 g), vitamin B6 (0.08 mg/100 g), and vitamin E (1.32 mg/100 g). Among minerals, mean amounts have been reported for calcium (56.86 mg/100 g), copper (0.11 mg/100 g), magnesium (20.14 mg/100 g), potassium (318.29 mg/100 g), phosphorus (48.86 mg/100 g), iron (1.29 mg/100 g), selenium (0.73 mg/100 g), and zinc (0.29 mg/100 g) Citation31, . On the other hand, a Serbian study reported that a soil management system may heavily modify the concentrations of some micronutrients, such as iron and copper, that were found in quadruple values, and for zinc, which had a 1.5-fold increase compared to standards; sodium was relatively high in this study (20 mg/100 g).[Citation35] A Romanian study reported that local cultivars have a higher amount (about 50 mg/100 g) of magnesium and a lower level (1 mg/100 g) of sodium.[Citation36]
Phytochemicals
BCs are rich in phytochemicals and contain a significant amount of polyphenols ().[Citation37] Phenolic content heavily depends on cultivars and it is reported in the literature that it may range from a minimum of 400 mg to a maximum of 800 mg in 100 g of fresh fruit and from 600 mg to 2800 mg in 100 g of dry fruit.[Citation5,Citation34,Citation38] Anthocyanins are the major group of phenolics found in BC and account for 80–96% of total phenolics.[Citation39] Their amount can vary between 80 and 470 mg/100 g of fresh weight.[Citation40] A recent comprehensive study evaluating the concentration of anthocyanins in eight cultivars over three years reported that the four major BC anthocyanins are delphinidin-3-rutinoside (36.7–63.6%), cyanidin-3-rutinoside (26.4–40.6%), delphinidin-3-glucoside (6.1–17.9%), and cyanidin-3-glucoside (1.3–9.9%).[Citation40] These four anthocyanins represent 97–98% of total BC anthocyanins.
On the other hand, flavonols in BC fruits represent a small portion of total polyphenols (1–6%), with about 20–60 mg/100 g of dry weight and with myricetin glycosides appearing to be the dominant group, quercetin glycosides appearing in a lower concentration.[Citation5] The concentration of flavanols in these fruits is between 10 to 20 mg/100 g of dry weight, with gallocatechins and epigallocatechins accounting for the highest levels in this category. BC is also recognized, similarly to other berries and grapes, to have a high concentration of proanthocyanidins, oligomers or polymers of monomeric flavanols, which was reported to be as high as 400 mg/100 g of fresh fruit with gallocatechins and epigallocatechins being the most prevalent.[Citation41] Yet, BC-processed product derivatives, such as juices and concentrates, have shown to have on average much lower proanthocyanidin contents than the corresponding fruits and the bioavailability of these compounds needs investigation since most have a high degree of polymerization, which may decrease their health benefits.[Citation42]
Among the non-flavonoid compounds in BC, phenolic acids are the most represented and are mainly conjugates of coumaric acids, followed by caffeic acid and ferulic acid (15–25 mg/100 g of dry weight.[Citation5] A couple of cultivars were found to contain more derivatives of caffeic acid than coumaric acid. Another study reporting more specific data on phenolic acids showed that multiple phenolic acids may be additionally extracted from BC such as protocatechuic, vanillic, ellagic, gallic, and syringic acid.[Citation28]
Finally, other interesting BC phytochemicals include monoterpenes such as 3-carene, orcimene, myrcene, sabinene, humulene, α pinene, eucalyptol, and terpinolene, which are responsible for the peculiar aroma profile of this fruit.[Citation43]
It is important to highlight the complexity of the absorption and metabolism of polyphenols. Anthocyanins can be absorbed by the body in their intact form (e.g. cyanidin-3-glycoside), however many polyphenols are present as glycosides that need to undergo hydrolysis to form the polyphenol (aglycone) and the sugar group (glycone) before absorption.[Citation18] The hydrolysis reaction can take place through lactase phlorizin hydrolase present in the microvillar membrane of epithelial cells in the small intestine or through cytosolic β-glucosidase within the epithelial cells. The aglycone product can then be absorbed, though it may undergo conjugation.
Role of blackcurrant bioactive compounds in sports nutrition
The common human intake of polyphenols can reach up to 1 g/day, which is ~10–100 times greater than the intake of other dietary antioxidants.[Citation44] For athletes, it has been recommended that the intake of >1000 mg/day polyphenol supplementation for at least three days (or more) before and following exercise can be effective in enhancing recovery from the muscle damage through antioxidant and anti-inflammatory mechanisms.[Citation18] The most common polyphenol supplementations that have been explored for enhancing sports performance are derived from pomegranate, tart Montmorency cherry, dark chocolate, honey, blackcurrants, and cherry juice.[Citation44]
General considerations on the energy metabolism of skeletal muscle allow to develop a schematic breakdown of physical activity and adaptation that occurs. During extremely brief efforts lasting seconds, such as throws, jumps, or 100- to 400-meter sprints, the most of adenosine triphosphate (ATP) is produced from phosphocreatine while glycogen is decomposed into lactate.[Citation45] The aerobic and oxidative metabolism of carbohydrates and fats occurs only during events lasting several minutes to hours and supplies nearly all the ATP for contracting skeletal muscle in marathons, triathlons, or cross-country skiing.[Citation46] Nowadays, there is no single systematic classification of sports that can be fully exhaustive. However, based on the aerobic or anaerobic metabolism induced by training, it is possible to distinguish different physical activities that have even different implications on nutritional supplementation.[Citation47] Indeed, there is clear evidence that chronic and moderate exercise training improves endogenous antioxidant capacity and studies suggest that aerobic endurance training is more involved than anaerobic ones.[Citation48] For instance, while prolonged exercise increases ROS generation at higher exercise intensities, moderate-intensity exercise is likely to have positive effects, as shown in a study that compared different cycling intensities.[Citation49]
Many hypotheses have been developed with respect to the antioxidant mechanism of polyphenols with respect to athletic performance. Contrary to expectations, recent research reports indicate that the mitochondria are not the main location of reactive oxygen species (ROS) generation in skeletal muscle during exercise.[Citation50] Other sources of ROS in the sarcoplasm are NADPH oxidases (isoform NOX2 is considered the most active in ROS production in muscle fibres) and phospholipase A2, which cleaves membrane phospholipids to release arachidonic acid, a substrate that stimulates ROS-generating enzyme systems ().[Citation51]
Figure 3. Proposed sites and mechanism of the production of reactive oxygen species in contracting skeletal muscles. CAT, catalase; SOD, superoxide dismutase; GPX, glutathione peroxidase; O2.−, superoxide; ·oh, hydroxyl radical; H2O2, hydrogen peroxide; NOX, NADPH oxidase; PLA2, phospholipase A2. Adapted from [Citation51] under the licences of (CC BY-NC-ND 4.0).
![Figure 3. Proposed sites and mechanism of the production of reactive oxygen species in contracting skeletal muscles. CAT, catalase; SOD, superoxide dismutase; GPX, glutathione peroxidase; O2.−, superoxide; ·oh, hydroxyl radical; H2O2, hydrogen peroxide; NOX, NADPH oxidase; PLA2, phospholipase A2. Adapted from [Citation51] under the licences of (CC BY-NC-ND 4.0).](/cms/asset/10f4e035-63ad-4c50-897d-72b54b6f0f44/lfri_a_2162076_f0003_oc.jpg)
In general, antioxidants can be divided into either RONS sequestrants (such as vitamin C, and vitamin E) or Nrf-2 activators (such as resveratrol and flavonoids). The high anti-oxidation capability of anthocyanins is due to donation of a hydrogen atom from the phenolic hydroxyl (OH) group,[Citation52] as well as the antioxidant ability centre of phenolic acids, which is the number and position of phenolic hydroxyls directly related to their radical scavenging activity.[Citation53] It is reported that RONS scavengers prevented the transient elevation of free radicals and, therefore, inhibited the antioxidant endogenous enzymes expression including mitochondrial superoxide dismutase (Mn-SOD) and glutathione peroxidase (GPX).[Citation20] As a consequence, RONS reduction with BC supplements before physical activities is positively considered when muscles are subjected to overstressing, whereas in standard training it negatively influences the adaptative response ().[Citation54]
Figure 4. Physiological impact of blackcurrant anthocyanins and vitamin C on the oxidative stress in athletes.
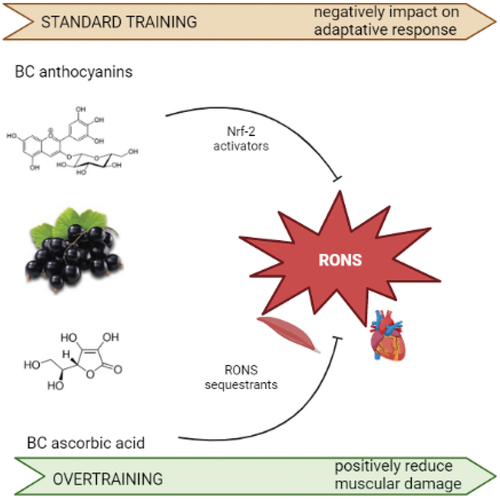
In addition to their radical scavenging property, polyphenols could also act as xenobiotics and consequently stimulate the hermetic cellular response that results in the production of endogenous antioxidants (such as superoxide dismutase, catalase, glutathione peroxidase, glutathione reductase, and glutathione transferase), which can further enhance the antioxidant status of the human body, including athletes ().[Citation55] Furthermore, flavonoids could assist in the recovery from exercise-induced muscle damage by upregulating Nrf2 cellular stress signalling pathway.[Citation56] The findings of a recent meta-analyses showed that flavonoid supplementation can improve muscle strength recovery by 7.14% (95% CI [5.50–8.78], P < .00001) and decrease muscle soreness by 4.12% (95% CI [−5.82 to −2.41] P = .00001).[Citation56] However, it did not influence the recovery of creatine kinase concentrations. The analyses concluded that flavonoids can significantly enhance muscular strength recovery and reduce muscle soreness during a 4-day period post exercise-induced muscle damage.
Figure 5. Mechanism of activation of cellular stress-related signalling pathways by polyphenols resulting in the mobilisation of nuclear factor-erythroid factor 2-related factor 2 (Nrf2) and the activation of the nuclear antioxidant response element (ARE). Activation of the ARE promotes the production of Phase II enzymes and antioxidants (such as glutathione (GSH)). Abbreviations: PI3K - Phosphoinositide 3-kinase, Akt – protein kinase B, PKC – protein kinase C, Grb2 - growth factor receptor-bound protein 2, ERK 1/2 - extracellular signal-regulated kinase 1/2, P – phosphate, and bZIP – basic region-leucine zipper.
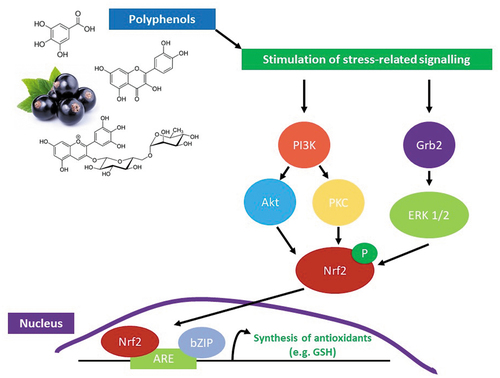
Blackcurrant supplementation and sports performance
In the past decade, exploration of the effectiveness of BC supplementation in the field of sports science has moved to the clinical phase. This includes studying the effect of BC supplementation on exercise performance, fat oxidation, blood lactate levels, muscle fatigue, and cardiac output as summarised in . The New Zealand Blackcurrant (NZBC) variety was preferred among the 15 selected studies as its total anthocyanin content ranges between 336 to 850 mg/100 mL of fruit juice compared to non-NZ BCs (170 to 310 mg/100 mL of juice).[Citation57]
Table 2. Summary of clinical studies investigating the effect of blackcurrant supplementation on sports performance.
Improved performance
Athletic performance differing by even 1% could ascertain the podium position of a competitor.[Citation57] A study [Citation58] reported that a 2.4% increase in cycling time-trial performance as a result of improved endothelial function and a potential increase in peripheral blood flow. Based on the findings of,[Citation59] NZBC supplementation may have beneficial effects on physical performances associated with isometric force steadiness through potential mechanisms like changes in local blood flow influencing contractile function, central nervous system (CNS) functioning, changes in gut microbiota, reduction of synaptic noise and finally antioxidative effects through lowering contraction-induced fatigue. Additionally, across a total of three climbs performed by male rock climbers, the improved higher Hang Time (HT) observed by may be explained by a potential increase in blood flow and improved oxygenation properties, though this may not account for progressive climb performance between the three climbs.[Citation60] As no difference in maximal oxygen uptake (VO2 max) between incremental doses was found, it is safe to assume that NZBC does not have diminishing effects on performance.[Citation61] The oxidative capacity or oxygen half-time recovery (O2HTR), reported by,[Citation62] was significantly improved by 5.3 seconds with NZBC supplementation. However, despite lowered minimum tissue saturation index (min-TSI%), no effect on performance was observed, which may have been a consequence of the discipline of climbers; differentiated between sport climbers and boulderers or by experiment design.
Fat oxidation
The passive benefits of fat oxidation are for individuals addressing weight-related concerns, including the maintenance of health for athletes.[Citation63] A study by [Citation64] suggested that increased adipose tissue lipolysis under resting conditions, along with glycerol and plasma non-esterified fatty acid (NEFA) concentrations being maintained at a higher-level during exercise, may be a potential mechanism for fat oxidation under the influence of NZBC. Interestingly, two studies [Citation63,Citation64] found that females appeared to be more responsive to NZBC supplementation towards exercise-induced fat oxidation compared to males, with a dependency on body composition. Four of the selected studies suggested that improved peripheral blood flow and vascular function allowed plasma fatty acids to be greatly available with enhanced delivery to skeletal muscles, which in turn, potentially increased fat oxidation.[Citation58,Citation61,Citation64,Citation65] A study by [Citation58] further suggested that NZBC may play a role in the upregulation of relevant genes involved in fat oxidation, transport of fatty acids into mitochondria, improved nitric oxide availability and increased peripheral blood flow. The findings of a study [Citation61] suggested that a 7-day intake may result in anthocyanin metabolite accumulation over time resulting in increased fat oxidation. In sport climbing performance, a study [Citation60] identified that the smaller changes obtained in physiological responses on NZBC were indicative of lower physiological distress with long climb times possibly due to improved local blood flow in the forearm. On the other hand, another study [Citation66] reported that blood flow as well as arterial diameter remained unchanged in placebo and was proposed to have been due to the timing of measurement, missing the peak blood flow.
Changes in blood lactate levels
Performing high-intensity exercises can increase the level of blood lactate, which can cause muscle soreness and reduce athletic performance. Research by [Citation67] and [Citation58] reported that NZBC supplementation increased blood/plasma lactate to exhaustion. These findings may have encouraged a faster rate of lactate removal (mass action effect), which improves passive recovery with NZBC. This was confirmed by [Citation68] wherein plasma lactate was lowered at aerobic capacity with NZBC due to vasodilation, causing delayed Onset of Blood Lactate Accumulation (OBLA) and allowing endurance athletes to perform prolonged high-intensity exercises. High lactate values may be a result of intracellular acidosis from the high intensity of repeated exercises, potentially postponing muscle fatigue and reductions in muscle excitability. It was also found that maximum oxygen uptake was obtained at lower lactate measurements, which may indicate the reduced requirement of anaerobic glycolysis to exhaustion. In contrast to these findings, the work of [Citation61] showed no changes in blood lactate levels.
Muscle fatigue
Muscle damage incurred by athletes may ultimately determine the duration for which they can prolong their performance. A study by [Citation69] suggested that improved blood flow by NZBC may have influenced the rapid influx and outflux of neutrophils while enhancing its antioxidant capacity, therefore preserving muscle functionality against oxidative stress, and preventing neutrophil-mediated inflammation. Similarly,[Citation70] found that BC nectar (BCN) provided a protective effect against muscular damage and attenuated the degradative effects of Exercise-Induced Muscle Damage (EIMD). Due to the antioxidative activities of BCs, the negative effects of ROS on sodium-potassium pumps were attenuated, resulting in the possible alleviation or prevention of fatigue. According to,[Citation69] a prolonged NZBC dosing strategy may have increased anthocyanin bioavailability, priming the cells and neutralising the release of ROS for longer periods as well as attenuating oxidative stress-induced damage to the before it occurs. In further agreement,[Citation71] observed consistently low muscle oxygen saturation (SmO2%) under presumed greater oxygen supply during 30% isometric maximal voluntary contractions (iMVC), which may indicate greater extraction and utilisation of oxygen, therefore resulting in lower muscular fatigue.
Cardiac output
Cardiac output determines the amount of blood pumped per minute to the rest of the body, consequently supporting several responses to exercises. A study [Citation62] proved the vasodilation potential of NZBC with improved oxygen delivery. Subsequently, however, intermediate rock climbers obtained no supporting measurements on arterial diameter, but the authors determined that faster time to half recovery (TTHR) may be explained by enhanced capillary function and oxygenation in the forearm muscle.[Citation66] The standard day-to-day artery diameter was found to be between 2.9 ± 0.4to 3.96 ± 0.5%, however, a study [Citation71] found femoral artery diameter increase ranged from 6.0–8.2%, which was attributed to the intake of NZBC. Furthermore, it was speculated that NZBC was effective on vasodilation not localized to just the femoral artery and increased absolute haemoglobin concentration (THb), which is indicative of an increased amount of blood in the muscle. This may explain the cardiovascular responses obtained, which could differ between individuals due to genotype. It was also suggested that several anthocyanin metabolites influenced cardiovascular bioactivity.
Similarly, a group of researchers [Citation72] also proposed that prolonged supplementation allowed anthocyanin metabolites or their synergistic action to alter cardiovascular functions during supine rest with an effect on vascular smooth cell behaviour, leading to decreased total peripheral resistance and mean arterial pressure. Moreover, another study [Citation68] demonstrated that reduced peripheral resistance, which is possibly due to increased nitric oxide production, increased cardiac output and volume at rest, with no changes in heart rate and blood pressure suggests that more complex haemodynamic mechanisms may be involved.
Although NZBC has influenced performance improvement with increased cardiac output and stroke volume, four of the selected studies reported no changes in heart rate compared to the placebo.[Citation61,Citation63,Citation68,Citation72] The heart rate and oxygen uptake at OBLA are characteristic of training endurance adaptations.[Citation68] However, as they remained unaffected, it was speculated that the delayed OBLA may have enhanced endurance performance and may involve different physiological mechanisms compared to other reported supplements such as beta-alanine, leucine and betamethylbutarate. The results of [Citation59] implicated that the longer-lasting anthocyanin metabolites may alter the mechanism that can enhance isometric force steadiness. Furthermore, different dosing forms may provide varying results as demonstrated by [Citation72] wherein encapsulated NZBC extract was used and resulted in reduced mean arterial pressure with higher doses of anthocyanins. In contrast, the NZBC powder dissolved in water presented no changes in arterial pressure with lower doses.[Citation68] The improved absorption rate of anthocyanins and potentially bypassing degradation from saliva may be the cause. Additionally,[Citation61] and [Citation72] observed no significant increase between 600 and 900 mg/day NZBC intake on any cardiovascular parameter during supine rest or on fat oxidation, respectively, which suggests that an upper limit in substrate utilisation or activity may have been limited by mechanisms responsible for anthocyanin absorption.
Potential blackcurrant-based functional products for athletes
Development of functional beverages for athletes
Functional beverages can provide energy and compensate for nutrient loss and fluid loss during physical activities.[Citation73] The addition of antioxidants, such as polyphenols, to these beverages, can promote recovery caused by oxidative stress and muscle damage. For example, an açai (Euterpe oleracea Mart.)-based functional beverage, containing 27.6 mg of anthocyanins per dose, was effective in enhancing the time to exhaustion of elite athletes during short-term high-intensity exercise, which reduced the metabolic stress induced by exercise, decreased perceived exertion, and improved cardiorespiratory responses.[Citation74] Thus, polyphenol (anthocyanin)-rich sources, like BC, can be used to develop functional beverages that will act as potential ergogenic aids in improving athlete performance during high-intensity training.
BC has been incorporated into a few beverages to produce functional products such as yoghurt and powdered beverages. A yoghurt beverage is characterized as a stirred yoghurt with low viscosity. A high-speed agitation is used to homogenize the yoghurt with or without the addition of cold yoghurt. During the agitation, the coagulum is broken. The incorporation of BC pomace extract into yoghurt beverages produced a fortified yoghurt beverage with a significant amount of polyphenols and anthocyanins.[Citation75] In addition, the fortified yoghurt showed enhanced antioxidant activity. In fact, fortification using BC pomace extract improved the yoghurt beverage storage stability.
Powered beverages are another type of functional beverage in the market. They are manufactured with spray-drying to remove the liquid in raw materials, which are designed to mix with water or milk to resemble beverages with fruit or soda flavour. Vitamins or other nutrients (e.g., prebiotics) are usually included in the powered beverages. The beverage powders are convenient to carry, shelf-life stable and nutrient-rich as well as welcomed by consumers, especially athletes. Athletes can use these beverages on different occasions and in sports settings.
A powdered beverage formulated using BC berries and yerba-mate infusion by freeze drying has shown good physical, biochemical, and microbiological stability under industrial storage.[Citation38] This polyphenol-rich beverage powder has shown high antioxidant activity after in vitro simulated gastrointestinal digestion. Again, the high antioxidant activity promotes this powdered beverage for further research in the development of functional beverages for athletes. This study also reported that the sensory analysis scores reflected consumers’ high level of acceptance.
Hydration is essential to athlete performance. Flavouring pure water with fruit flavour aids hydration practice as well as compensating electrolyte loss during sweating. The antioxidant components of BC fruit can improve athlete performance and control oxidative stress as discussed. Thus, it could be a potential flavouring ingredient.
Development of functional snacks for athletes
Athletes performing exercise tend to have a higher metabolism rate and energy consumption, thus will consume more food during the day than non-athletes. Snacks, such as functional crackers, could be a good option for athletes. Crackers are associated with a diet containing high dietary fibre and nutrient content. Crackers produced with white flour contain less fibre because the process of refining removes the grain parts containing high fibre and keeps starchy endosperm. The gross composition of dietary fibres in white flour is 4%. On the other hand, the dietary fibres of BC pomace are around 57%, which is over 16 and 6 times greater than wheat flour and corn flour, respectively.
Pomace powders from BC, redcurrant, chokeberry, rowanberry, and gooseberry contain a higher fibre content, reaching >550 g/kg and fat content of up to 200 g/kg. The residues of the berry also contain bioactive compounds, e.g., anthocyanins that trigger health-promoting effects. Although pomace from BC is a by-product of juice processing after drying and milling processes, it can be incorporated into crackers, leading towards healthier, functional, and innovative products. Non-wheat flour can also be used to produce gluten-free products so that the products are rich in vitamins, phenolic compounds, and dietary fibre.
High dietary fibre is associated with athlete performance.[Citation76] Diets containing high fibre are recommended for weight regulation. Consumption of high dietary fibres can protect against the impacts of cancer, type II diabetes, and negative cardiovascular events.[Citation77] The positive effects of exercise on gut microbiota depend on dietary fibre and protein intake. The consumption of crackers with high dietary fibre and antioxidants could be an option to eat for the athlete to keep their performance. As discussed earlier, the consumption of antioxidants prevents or minimizes the attack of free radicals in skeletal muscle damage and muscle fatigue. Furthermore, based on clinical evidence, dietary fibre can improve athletic performance, by possessing physiological effects in the gastrointestinal tract.[Citation78]
The berry pomaces could be integrated into savoury crackers to produce functional crackers. BC pomace appears to be a potential ingredient due to its nutritional composition, purple colour, delicious flavour, and odour. Incorporating BC pomace as a partial flour substitute in savoury crackers resulted in producing crackers with acid and fruity smell and taste, with higher nutritional value, and without altering the general acceptance of the product.[Citation79] Nevertheless, the dough characteristics and product properties had some weaknesses that could be compensated for by altering the formulation to achieve iso-viscoelastic doughs.
Corn puffs can be considered a potential snack that could turn into a functional snack upon fortification with beneficial antioxidants. Pressed residues of BC obtained after juice extraction have been used as an antioxidant-rich source to fortify corn puffs.[Citation80] The fortified corn puffs had higher polyphenol and antioxidant contents compared to pure corn puffs. The anthocyanins delphinidin-3-O-rutinoside, petunidin-3-O-rutinoside, cyanidin-3-O-glucoside, cyanidin-3-O-rutinoside, delphinidin-3-O-(6’’-p-coumaroyl)-glucoside, and cyanidin-3-O-(6’’-p-coumaroyl)-glucoside were present in the formulated product.
Gluten-free products for celiac athletes
Gluten is a protein mixture in bakery products, whose main compositions are gliadins and glutenins, with limited solubility in water.[Citation81] For gluten formation, gliadins and glutenins should be hydrated with water or other liquid and mixed to form a cohesive network.[Citation82] The gluten network is dependent on the disulfide bonds and non-covalent bond formation during dough preparation, which can be affected by the amount of added water, oil, sugar and temperature.[Citation83] The network provides a stretchy quality in the final products. The texture of bread, such as chewiness, is dependent on gluten formation in the dough.
People affected by celiac disease are incapable of digesting gluten-rich products. Celiac disease is a chronic immune disorder prompted by ingesting gluten that results in damage to the intestinal lining and causes diarrhoea, fatigue, weight loss, bloating and anaemia.[Citation84] For an athlete, the diagnosis of the celiac disease means a lifestyle change. Athletes who suffer from celiac disease can have poor iron, vitamin D, and calcium absorption, which causes anaemia, osteoporosis, and weak bones, respectively.[Citation85] Celiac athletes need to be supplemented with multivitamins and minerals. Recent studies have focused on adding nutrition-rich sources to gluten-free bakery products to improve their nutritional values. BC, as a rich source of nutrients, could be a functional ingredient to produce gluten-free bakery products for celiac athletes and positively influence their performance.
Incorporating defatted BC seeds in gluten-free bread significantly improved the viscoelastic properties of the dough and decreased the consistency coefficient values and flow indices.[Citation86] The produced bread had high dietary fibre, protein, and polyphenols contents. The incorporation of defatted BC seeds in gluten-free recipes did not have a negative impact on the sensory evaluation of the products. In addition, the dehydrated BC juice by-product, containing high polyphenol and dietary fibre contents, has been used to formulate functional gluten-free chocolate cookies.[Citation87] The incorporation of BC into the cookies did not cause any severe organoleptic changes. In fact, it increased the phenolic content and antioxidant activity by 62 and 70%, respectively. Moreover, BC antioxidants showed acceptable bioaccessibility by reaching the large intestine as evaluated through in vitro digestion. Additionally, the total dietary fibre of the product increased 2.5 times reaching a final content that enables it to become a source of fibre according to the Codex Alimentarius. Both coeliac and non-coeliac consumers reported a high level of acceptance of the products based on sensory analysis.
Conclusions
BCs contain a wide range of nutrients and bioactive compounds. This makes BC a rich nutritional and antioxidant source that can be used to develop functional foods. The high vitamin C and polyphenol contents of BCs make them a potential candidate to develop functional foods for athletes. Athletes face oxidative stress when practising intensive exercise, which can reduce their performance. However, clinical studies have shown that BC supplementation can improve athletic performance. BC juice, powder, or pomace can become a functional ingredient to develop functional beverages and snacks for athletes. Yet, only limited products have been developed so far.
Author contribution
Ali Ali Redha: Conceptualization; Data curation; Formal analysis; Resources; Software; Visualization; Writing – original draft; Writing – review & editing.
Shahida Anusha Siddiqui: Writing – original draft.
Reza Zare: Conceptualization; Data curation; Formal analysis; Writing – original draft; Writing – review & editing.
Daniele Spadaccini: Writing – original draft.
Silvia Guazzotti: Visualization; Writing – original draft.
Xi Feng: Writing – original draft.
Nur Alim Bahmid: Writing – original draft.
Yuan Seng Wu: Writing – review & editing.
Fathima Zahraa Ozeer: Writing – original draft.
Rotimi E. Aluko: Writing – review & editing, Supervision.
Disclosure statement
No potential conflict of interest was reported by the authors.
Correction Statement
This article has been corrected with minor changes. These changes do not impact the academic content of the article.
References
- Cortez, R. E.; Gonzalez de Mejia, E. Blackcurrants (Ribes Nigrum): A Review on Chemistry, Processing, and Health Benefits. J. Food Sci. 2019, 84(9), 2387–2401. From NLM Medline. DOI: 10.1111/1750-3841.14781.
- Pott, D. M.; Durán-Soria, S.; Allwood, J. W.; Pont, S.; Gordon, S. L.; Jennings, N.; Austin, C.; Stewart, D.; Brennan, R. M.; Masny, A., et al. Dissecting the Impact of Environment, Season and Genotype on Blackcurrant Fruit Quality Traits. Food Chem. 2023, 402, 134360. DOI: 10.1016/j.foodchem.2022.134360.
- Paunović, S. M.; Mašković, P.; Milinković, M. Chemical Compounds and Biological Activity in Black Currant (Ribes Nigrum L.) Berries Depending on Soil Temperature and Moisture. Erwerbs-Obstbau. 2022, 64(4), 621–629. DOI: 10.1007/s10341-022-00712-8.
- Trych, U.; Buniowska, M.; Skapska, S.; Kapusta, I.; Marszalek, K. Bioaccessibility of Antioxidants in Blackcurrant Juice After Treatment Using Supercritical Carbon Dioxide. Molecules. 2022, 27(3), 3. From NLM Medline. DOI: 10.3390/molecules27031036.
- Tian, Y.; Laaksonen, O.; Haikonen, H.; Vanag, A.; Ejaz, H.; Linderborg, K.; Karhu, S.; Yang, B. Compositional Diversity Among Blackcurrant (Ribes Nigrum) Cultivars Originating from European Countries. J. Agric. Food. Chem. 2019, 67(19), 5621–5633. From NLM Medline. DOI: 10.1021/acs.jafc.9b00033.
- Xue, B.; Hui, X.; Chen, X.; Luo, S.; Dilrukshi, H. N. N.; Wu, G.; Chen, C. Application, Emerging Health Benefits, and Dosage Effects of Blackcurrant Food Formats. J. Funct. Foods. 2022, 95, 105147. DOI: 10.1016/j.jff.2022.105147.
- Azman, E. M.; Nor, N. D. M.; Charalampopoulos, D.; Chatzifragkou, A. Effect of Acidified Water on Phenolic Profile and Antioxidant Activity of Dried Blackcurrant (Ribes Nigrum L.) Pomace Extracts. LWT. 2022, 154, 112733. DOI: 10.1016/j.lwt.2021.112733.
- Xu, Y.; Cai, F.; Yu, Z.; Zhang, L.; Li, X.; Yang, Y.; Liu, G. Optimisation of Pressurised Water Extraction of Polysaccharides from Blackcurrant and Its Antioxidant Activity. Food Chem. 2016, 194, 650–658. From NLM Medline. DOI: 10.1016/j.foodchem.2015.08.061.
- Diaconeasa, Z.; Leopold, L.; Rugina, D.; Ayvaz, H.; Socaciu, C. Antiproliferative and Antioxidant Properties of Anthocyanin Rich Extracts from Blueberry and Blackcurrant Juice. Int. J. Mol. Sci. 2015, 16(2), 2352–2365. From NLM Medline. Diaconeasa, Z.; Iuhas, C.; I.; Ayvaz, H.; Rugina, D.; Stanila, A.; Dulf, F.; Bunea, A.; Socaci, S. A.; Socaciu, C.; Pintea, A. Phytochemical Characterization of Commercial Processed Blueberry, Blackberry, Blackcurrant, Cranberry, and Raspberry and Their Antioxidant Activity. Antioxidants (Basel). 2019, 8 (11). DOI: 10.3390/antiox8110540 From NLM PubMed From NLM PubMednotMEDLINE. DOI: 10.3390/antiox8110540.
- Strugała, P.; Gładkowski, W.; Kucharska, A. Z.; Sokół‐łętowska, A.; Gabrielska, J. Antioxidant Activity and Anti‐inflammatory Effect of Fruit Extracts from Blackcurrant, Chokeberry, Hawthorn, and Rosehip, and Their Mixture with Linseed Oil on a Model Lipid Membrane. Eur. J. Lipid Sci. Technol. 2015, 118(3), 461–474. DOI: 10.1002/ejlt.201500001.
- Balstad, T. R.; Paur, I.; Poulsen, M.; Markowski, J.; Kolodziejczyk, K.; Dragsted, L. O.; Myhrstad, M. C.; Blomhoff, R. Apple, Cherry, and Blackcurrant Increases Nuclear Factor Kappa B Activation in Liver of Transgenic Mice. Nutr. Cancer. 2010, 62(6), 841–848. From NLM Medline. DOI: 10.1080/01635581003695749.
- Luis, A.; Domingues, F.; Pereira, L. Association Between Berries Intake and Cardiovascular Diseases Risk Factors: A Systematic Review with Meta-Analysis and Trial Sequential Analysis of Randomized Controlled Trials. Food Funct. 2018, 9(2), 740–757. From NLM Medline. DOI: 10.1039/c7fo01551h.
- Vendrame, S.; Adekeye, T. E.; Klimis-Zacas, D. The Role of Berry Consumption on Blood Pressure Regulation and Hypertension: An Overview of the Clinical Evidence. Nutrients. 2022, 14(13), 13. From NLM Medline. DOI: 10.3390/nu14132701.
- Tjelle, T. E.; Holtung, L.; Bohn, S. K.; Aaby, K.; Thoresen, M.; Wiik, S. A.; Paur, I.; Karlsen, A. S.; Retterstol, K.; Iversen, P. O., et al. Polyphenol-Rich Juices Reduce Blood Pressure Measures in a Randomised Controlled Trial in High Normal and Hypertensive Volunteers. Br. J. Nutr. 2015, 114(7), 1054–1063. From NLM Medline. DOI: 10.1017/S0007114515000562.
- Cook, M. D.; Sandu, B. H. A. K.; Joyce Ph, D. J. Effect of New Zealand Blackcurrant on Blood Pressure, Cognitive Function and Functional Performance in Older Adults. J. Nutr. Gerontol. Geriatr. 2020, 39(2), 99–113. From NLM Medline. DOI: 10.1080/21551197.2019.1707740.
- Coleman, S. L.; Shaw, O. M. Progress in the Understanding of the Pathology of Allergic Asthma and the Potential of Fruit Proanthocyanidins as Modulators of Airway Inflammation. Food Funct. 2017, 8(12), 4315–4324. From NLM Medline. DOI: 10.1039/c7fo00789b.
- Burton-Freeman, B.; Brzezinski, M.; Park, E.; Sandhu, A.; Xiao, D.; Edirisinghe, I. A Selective Role of Dietary Anthocyanins and Flavan-3-Ols in Reducing the Risk of Type 2 Diabetes Mellitus: A Review of Recent Evidence. Nutrients. 2019, 11(4), 4. From NLM Medline. DOI: 10.3390/nu11040841.
- Bowtell, J.; Kelly, V. Fruit-Derived Polyphenol Supplementation for Athlete Recovery and Performance. Sports Med. 2019, 49(Suppl 1), 3–23. From NLM Medline. DOI: 10.1007/s40279-018-0998-x.
- Al-Horani, R. A. A Narrative Review of Exercise-Induced Oxidative Stress: Oxidative DNA Damage Underlined. Open Sports Sci. J. 2022, 15(1), 1. DOI: 10.2174/1875399X-v15-e2202220.
- Li, S.; Fasipe, B.; Laher, I. Potential Harms of Supplementation with High Doses of Antioxidants in Athletes. J. Exerc. Sci. Fit. 2022, 20(4), 269–275. From NLM PubMed-not-MEDLINE. DOI: 10.1016/j.jesf.2022.06.001.
- Wang, H.; Yang, Z.; Zhang, X.; Xie, J.; Xie, Y.; Gokulnath, P.; Vulugundam, G.; Xiao, J. Antioxidants Supplementation During Exercise: Friends or Enemies for Cardiovascular Homeostasis? J. Cardiovasc. Transl. Res. 2022. From NLM Publisher. DOI: 10.1007/s12265-022-10297-y.
- Pizzino, G.; Irrera, N.; Cucinotta, M.; Pallio, G.; Mannino, F.; Arcoraci, V.; Squadrito, F.; Altavilla, D.; Bitto, A. Oxidative Stress: Harms and Benefits for Human Health. Oxid. Med. Cell Longev. 2017, 2017, 8416763. From NLM Medline. DOI: 10.1155/2017/8416763.
- Heiberg, N.; Måge, F.; Haffner, K. Chemical Composition of Ten Blackcurrant (Ribes nigrumL.) Cultivars. Acta Agric. Scand. - B Soil Plant Sci. 1992, 42(4), 251–254. DOI: 10.1080/09064719209410221.
- Boccorh, R. K.; Paterson, A.; Piggott, J. R. Factors Influencing Quantities of Sugars and Organic Acids in Blackcurrant Concentrates. Zeitschrift für Lebensmitteluntersuchung und -Forschung A. 1998, 206(4), 273–278. DOI: 10.1007/s002170050256.
- Déniel, M.; Haarlemmer, G.; Roubaud, A.; Weiss-Hortala, E.; Fages, J. Hydrothermal Liquefaction of Blackcurrant Pomace and Model Molecules: Understanding of Reaction Mechanisms. Sustain. Energy Fuels. 2017, 1(3), 555–582. DOI: 10.1039/c6se00065g.
- Takata, R.; Yamamoto, R.; Yanai, T.; Konno, T.; Okubo, T. Immunostimulatory Effects of a Polysaccharide-Rich Substance with Antitumor Activity Isolated from Black Currant (Ribes Nigrum L.). Biosci. Biotechnol. Biochem. 2005, 69(11), 2042–2050. DOI: 10.1271/bbb.69.2042.
- Xu, Y.; Guo, Y.; Duan, S.; Wei, H.; Liu, Y.; Wang, L.; Huo, X.; Yang, Y. Effects of Ultrasound Irradiation on the Characterization and Bioactivities of the Polysaccharide from Blackcurrant Fruits. Ultrason. Sonochem. 2018, 49, 206–214. From NLM Medline. DOI: 10.1016/j.ultsonch.2018.08.005.
- Ersoy, N.; Kupe, M.; Gundogdu, M.; Ilhan, G.; Ercisli, S. Phytochemical and Antioxidant Diversity in Fruits of Currant (Ribes Spp.). Notulae Botanicae Horti Agrobotanici Cluj-Napoca. 2018, 46(2), 381–387. DOI: 10.15835/nbha46211103.
- Nigmatzyanov, R.; Sorokopudov, V.; Nazaryuk, N. Biochemical Composition of Black Currant Berries (Ribes Nigrum L.) for Development of New Cultivars in Bashkortostan. BIO Web Conf. 2021, 30, 04004. DOI: 10.1051/bioconf/20213004004.
- Alba, K.; MacNaughtan, W.; Laws, A. P.; Foster, T. J.; Campbell, G. M.; Kontogiorgos, V. Fractionation and Characterisation of Dietary Fibre from Blackcurrant Pomace. Food Hydrocoll. 2018, 81, 398–408. DOI: 10.1016/j.foodhyd.2018.03.023.
- U.S. Department of Agriculture. FoodData Central - Currants, European Black, Raw. 2019. https://fdc.nal.usda.gov/fdc-app.html#/food-details/173963/nutrients (accessed Oct 16 2022).
- Gopalan, A.; Reuben, S. C.; Ahmed, S.; Darvesh, A. S.; Hohmann, J.; Bishayee, A. The Health Benefits of Blackcurrants. Food Funct. 2012, 3(8), 795–809. From NLM Medline. DOI: 10.1039/c2fo30058c.
- Szajdek, A.; Borowska, E. J. Bioactive Compounds and Health-Promoting Properties of Berry Fruits: A Review. Plant Foods Hum. Nutr. 2008, 63(4), 147–156. From NLM Medline. DOI: 10.1007/s11130-008-0097-5.
- Khoo, G. M.; Clausen, M. R.; Pedersen, H. L.; Larsen, E. Bioactivity and Chemical Composition of Blackcurrant (Ribes Nigrum) Cultivars with and Without Pesticide Treatment. Food Chem. 2012, 132(3), 1214–1220. From NLM PubMed-not-MEDLINE. DOI: 10.1016/j.foodchem.2011.11.087.
- Paunović, S. M.; Nikolić, M.; Miletić, R.; Mašković, P. Vitamin and Mineral Content in Black Currant (Ribes Nigrum L.) Fruits as Affected by Soil Management System. Acta Sci. Pol. Hortoru. Cultus. 2017, 16(5), 135–144. DOI: 10.24326/asphc.2017.5.14.
- Nour, V.; Trandafir, I.; Ionica, M. E. Ascorbic Acid, Anthocyanins, Organic Acids and Mineral Content of Some Black and Red Currant Cultivars. Fruits. 2011, 66(5), 353–362. DOI: 10.1051/fruits/2011049.
- Alchera, F.; Ginepro, M.; Giacalone, G. Microwave-Assisted Extraction of Polyphenols from Blackcurrant By-Products and Possible Uses of the Extracts in Active Packaging. Foods. 2022, 11(18), 18. From NLM PubMed-not-MEDLINE. DOI: 10.3390/foods11182727.
- Orjuela-Palacio, J. M.; Zamora, M. C.; Lanari, M. C. Physicochemical and Dynamic Sensory Characterization of a Yerba Mate/Blackcurrant Instant Beverage Powder Rich in Natural Antioxidants. J. Berry Res. 2019, 9(2), 195–208. DOI: 10.3233/jbr-180342.
- Staszowska-Karkut, M.; Materska, M. Phenolic Composition, Mineral Content, and Beneficial Bioactivities of Leaf Extracts from Black Currant (Ribes Nigrum L.), Raspberry (Rubus Idaeus), and Aronia (Aronia Melanocarpa). Nutrients. 2020, 12(2), 2. From NLM Medline. DOI: 10.3390/nu12020463.
- Simerdova, B.; Bobrikova, M.; Lhotska, I.; Kaplan, J.; Krenova, A.; Satinsky, D. Evaluation of Anthocyanin Profiles in Various Blackcurrant Cultivars Over a Three-Year Period Using a Fast HPLC-DAD Method. Foods. 2021, 10(8), 8. From NLM PubMed-not-MEDLINE. DOI: 10.3390/foods10081745.
- Yang, W.; Ma, X.; Laaksonen, O.; He, W.; Kallio, H.; Yang, B. Effects of Latitude and Weather Conditions on Proanthocyanidins in Blackcurrant (Ribes Nigrum) of Finnish Commercial Cultivars. J. Agric. Food. Chem. 2019, 67(51), 14038–14047. From NLM Medline. DOI: 10.1021/acs.jafc.9b06031.
- Hollands, W.; Brett, G. M.; Radreau, P.; Saha, S.; Teucher, B.; Bennett, R. N.; Kroon, P. A. Processing Blackcurrants Dramatically Reduces the Content and Does Not Enhance the Urinary Yield of Anthocyanins in Human Subjects. Food Chem. 2008, 108(3), 869–878. From NLM PubMed-not-MEDLINE. DOI: 10.1016/j.foodchem.2007.11.052.
- Ruiz Del Castillo, M. L.; Dobson, G. Varietal Differences in Terpene Composition of Blackcurrant (Ribes Nigrum L) Berries by Solid Phase Microextraction/Gas Chromatography. J. Sci. Food Agric. 2002, 82(13), 1510–1515. DOI: 10.1002/jsfa.1210.
- D’Angelo, S. Polyphenols: Potential Beneficial Effects of These Phytochemicals in Athletes. Curr. Sports Med. Rep. 2020, 19(17), 260–265. DOI: 10.1249/JSR.0000000000000729.
- Hargreaves, M.; Spriet, L. L. Skeletal Muscle Energy Metabolism During Exercise. Nat. Metab. 2020, 2(9), 817–828. From NLM Medline. DOI: 10.1038/s42255-020-0251-4.
- Maughan, R. J.; Burke, L. M.; Dvorak, J.; Larson-Meyer, D. E.; Peeling, P.; Phillips, S. M.; Rawson, E. S.; Walsh, N. P.; Garthe, I.; Geyer, H., et al. IOC Consensus Statement: Dietary Supplements and the High-Performance Athlete. Br. J. Sports Med. 2018, 52(7), 439–455. From NLM Medline. DOI: 10.1136/bjsports-2018-099027.
- Hawley, J. A.; Lundby, C.; Cotter, J. D.; Burke, L. M. Maximizing Cellular Adaptation to Endurance Exercise in Skeletal Muscle. Cell Metab. 2018, 27(5), 962–976. From NLM Medline. DOI: 10.1016/j.cmet.2018.04.014.
- Mason, S. A.; Trewin, A. J.; Parker, L.; Wadley, G. D. Antioxidant Supplements and Endurance Exercise: Current Evidence and Mechanistic Insights. Redox Biol. 2020, 35, 101471. From NLM Medline. DOI: 10.1016/j.redox.2020.101471.
- Awang Daud, D. M.; Ahmedy, F.; Baharuddin, D. M. P.; Zakaria, Z. A. Oxidative Stress and Antioxidant Enzymes Activity After Cycling at Different Intensity and Duration. Appl. Sci. 2022, 12(18), 18. DOI: 10.3390/app12189161.
- Hudson, M. B.; Smuder, A. J.; Nelson, W. B.; Wiggs, M. P.; Shimkus, K. L.; Fluckey, J. D.; Szeto, H. H.; Powers, S. K. Partial Support Ventilation and Mitochondrial-Targeted Antioxidants Protect Against Ventilator-Induced Decreases in Diaphragm Muscle Protein Synthesis. PLoS One. 2015, 10(9), e0137693. From NLM Medline. DOI: 10.1371/journal.pone.0137693.
- Powers, S. K.; Deminice, R.; Ozdemir, M.; Yoshihara, T.; Bomkamp, M. P.; Hyatt, H. Exercise-Induced Oxidative Stress: Friend or Foe? J. Sport Health Sci. 2020, 9(5), 415–425. From NLM Medline. DOI: 10.1016/j.jshs.2020.04.001.
- Ma, Y.; Feng, Y.; Diao, T.; Zeng, W.; Zuo, Y. Experimental and Theoretical Study on Antioxidant Activity of the Four Anthocyanins. J. Mol. Struct. 2020, 1204, 127509. DOI: 10.1016/j.molstruc.2019.127509.
- Chen, J.; Yang, J.; Ma, L.; Li, J.; Shahzad, N.; Kim, C. K. Structure-Antioxidant Activity Relationship of Methoxy, Phenolic Hydroxyl, and Carboxylic Acid Groups of Phenolic Acids. Sci. Rep. 2020, 10(1), 2611. From NLM PubMed-not-MEDLINE. DOI: 10.1038/s41598-020-59451-z.
- Bellezza, I.; Giambanco, I.; Minelli, A.; Donato, R. Nrf2-Keap1 Signaling in Oxidative and Reductive Stress. Biochim. Biophys. Acta Mol. Cell Res. 2018, 1865(5), 721–733. From NLM Medline. DOI: 10.1016/j.bbamcr.2018.02.010.
- Visioli, F. Chapter 6 - Polyphenols in Sport: Facts or Fads? In Antioxidants in Sport Nutrition; Lamprecht, M., Ed.; Boca Raton: CRC Press/Taylor & Francis, 2015.
- Carey, C. C.; Lucey, A.; Doyle, L. Flavonoid Containing Polyphenol Consumption and Recovery from Exercise-Induced Muscle Damage: A Systematic Review and Meta-Analysis. Sports Med. 2021, 51(6), 1293–1316. From NLM Medline. DOI: 10.1007/s40279-021-01440-x.
- Braakhuis, A. J.; Somerville, V. X.; Hurst, R. D. The Effect of New Zealand Blackcurrant on Sport Performance and Related Biomarkers: A Systematic Review and Meta-Analysis. J. Int. Soc. Sports Nutr. 2020, 17(1), 25. From NLM Medline. DOI: 10.1186/s12970-020-00354-9.
- Cook, M. D.; Myers, S. D.; Blacker, S. D.; Willems, M. E. New Zealand Blackcurrant Extract Improves Cycling Performance and Fat Oxidation in Cyclists. Eur. J. Appl. Physiol. 2015, 115(11), 2357–2365. From NLM Medline. DOI: 10.1007/s00421-015-3215-8.
- Cook, M. D.; Dunne, A.; Bosworth, M.; Willems, M. E. T. Effect of New Zealand Blackcurrant Extract on Force Steadiness of the Quadriceps Femoris Muscle During Sustained Submaximal Isometric Contraction. J. Funct. Morphol. Kinesiol. 2022, 7(2), 2. From NLM PubMed-not-MEDLINE. DOI: 10.3390/jfmk7020044.
- Potter, J. A.; Hodgson, C. I.; Broadhurst, M.; Howell, L.; Gilbert, J.; Willems, M. E. T.; Perkins, I. C. Effects of New Zealand Blackcurrant Extract on Sport Climbing Performance. Eur. J. Appl. Physiol. 2020, 120(1), 67–75. From NLM Medline. DOI: 10.1007/s00421-019-04226-2.
- Cook, M. D.; Myers, S. D.; Gault, M. L.; Edwards, V. C.; Willems, M. E. T. Dose Effects of New Zealand Blackcurrant on Substrate Oxidation and Physiological Responses During Prolonged Cycling. Eur. J. Appl. Physiol. 2017, 117(6), 1207–1216. From NLM Medline. DOI: 10.1007/s00421-017-3607-z.
- Fryer, S.; Giles, D.; Bird, E.; Stone, K.; Paterson, C.; Balas, J.; Willems, M. E. T.; Potter, J. A.; Perkins, I. C. New Zealand Blackcurrant Extract Enhances Muscle Oxygenation During Repeated Intermittent Forearm Muscle Contractions in Advanced and Elite Rock Climbers. Eur. J. Sport. Sci. 2021, 21(9), 1290–1298. From NLM Medline. DOI: 10.1080/17461391.2020.1827048.
- Willems, M. E. T.; Banic, M.; Cadden, R.; Barnett, L. Enhanced Walking-Induced Fat Oxidation by New Zealand Blackcurrant Extract is Body Composition-Dependent in Recreationally Active Adult Females. Nutrients. 2022, 14(7), 7. From NLM Medline. DOI: 10.3390/nu14071475.
- Strauss, J. A.; Willems, M. E. T.; Shepherd, S. O. New Zealand Blackcurrant Extract Enhances Fat Oxidation During Prolonged Cycling in Endurance-Trained Females. Eur. J. Appl. Physiol. 2018, 118(6), 1265–1272. From NLM Medline. DOI: 10.1007/s00421-018-3858-3.
- Hiles, A. M.; Flood, T. R.; Lee, B. J.; Wheeler, L. E. V.; Costello, R.; Walker, E. F.; Ashdown, K. M.; Kuennen, M. R.; Willems, M. E. T. Dietary Supplementation with New Zealand Blackcurrant Extract Enhances Fat Oxidation During Submaximal Exercise in the Heat. J. Sci. Med. Sport. 2020, 23(10), 908–912. From NLM Medline. DOI: 10.1016/j.jsams.2020.02.017.
- Fryer, S.; Paterson, C.; Perkins, I. C.; Gloster, C.; Willems, M. E. T.; Potter, J. A. New Zealand Blackcurrant Extract Enhances Muscle Oxygenation During Forearm Exercise in Intermediate-Level Rock Climbers. Int. J. Sport Nutr. Exerc. Metab. 2020, 30(4), 258–263. From NLM Medline. DOI: 10.1123/ijsnem.2019-0365.
- Perkins, I. C.; Vine, S. A.; Blacker, S. D.; Willems, M. E. New Zealand Blackcurrant Extract Improves High-Intensity Intermittent Running. Int. J. Sport Nutr. Exerc. Metab. 2015, 25(5), 487–493. From NLM Medline. DOI: 10.1123/ijsnem.2015-0020.
- Willems, M. E.; Myers, S. D.; Gault, M. L.; Cook, M. D. Beneficial Physiological Effects with Blackcurrant Intake in Endurance Athletes. Int. J. Sport Nutr. Exerc. Metab. 2015, 25(4), 367–374. From NLM Medline. DOI: 10.1123/ijsnem.2014-0233.
- Hunt, J. E. A.; Coelho, M. O. C.; Buxton, S.; Butcher, R.; Foran, D.; Rowland, D.; Gurton, W.; Macrae, H.; Jones, L.; Gapper, K. S., et al. Consumption of New Zealand Blackcurrant Extract Improves Recovery from Exercise-Induced Muscle Damage in Non-Resistance Trained Men and Women: A Double-Blind Randomised Trial. Nutrients. 2021, 13(8), 8. From NLM Medline. DOI: 10.3390/nu13082875.
- Hutchison, A. T.; Flieller, E. B.; Dillon, K. J.; Leverett, B. D. Black Currant Nectar Reduces Muscle Damage and Inflammation Following a Bout of High-Intensity Eccentric Contractions. J. Diet. Suppl. 2016, 13(1), 1–15. From NLM Medline. DOI: 10.3109/19390211.2014.952864.
- Cook, M. D.; Myers, S. D.; Gault, M. L.; Willems, M. E. T. Blackcurrant Alters Physiological Responses and Femoral Artery Diameter During Sustained Isometric Contraction. Nutrients. 2017, 9(6), 6. From NLM Medline. DOI: 10.3390/nu9060556.
- Cook, M. D.; Myers, S. D.; Gault, M. L.; Edwards, V. C.; Willems, M. E. Cardiovascular Function During Supine Rest in Endurance-Trained Males with New Zealand Blackcurrant: A Dose-Response Study. Eur. J. Appl. Physiol. 2017, 117(2), 247–254. From NLM Medline. DOI: 10.1007/s00421-016-3512-x.
- Orru, S.; Imperlini, E.; Nigro, E.; Alfieri, A.; Cevenini, A.; Polito, R.; Daniele, A.; Buono, P.; Mancini, A. Role of Functional Beverages on Sport Performance and Recovery. Nutrients. 2018, 10(10), 10. From NLM Medline. DOI: 10.3390/nu10101470.
- Carvalho-Peixoto, J.; Moura, M. R.; Cunha, F. A.; Lollo, P. C.; Monteiro, W. D.; Carvalho, L. M.; Farinatti Pde, T. Consumption of Acai (Euterpe Oleracea Mart.) Functional Beverage Reduces Muscle Stress and Improves Effort Tolerance in Elite Athletes: A Randomized Controlled Intervention Study. Appl. Physiol. Nutr. Metab. 2015, 40(7), 725–733. From NLM Medline. DOI: 10.1139/apnm-2014-0518.
- Raikos, V.; Ni, H.; Hayes, H.; Ranawana, V. Antioxidant Properties of a Yogurt Beverage Enriched with Salal (Gaultheria Shallon) Berries and Blackcurrant (Ribes Nigrum) Pomace During Cold Storage. Beverages. 2018, 5(1), 1. DOI: 10.3390/beverages5010002.
- Jakubczyk, K.; Koprowska, K.; Gottschling, A.; Janda-Milczarek, K. Edible Flowers as a Source of Dietary Fibre (Total, Insoluble and Soluble) as a Potential Athlete’s Dietary Supplement. Nutrients. 2022, 14(12), 12. From NLM Medline. DOI: 10.3390/nu14122470.
- Prasad, K. N.; Bondy, S. C. Dietary Fibers and Their Fermented Short-Chain Fatty Acids in Prevention of Human Diseases. Bioact. Carbohydr. Dietary Fibre. 2019, 17, 100170. DOI: 10.1016/j.bcdf.2018.09.001.
- Sugiyama, F.; Yamaguchi, T.; Hu, A.; Kobayashi, A.; Kobayashi, H. Effects of Fiber Supplementation for Four Weeks on Athletic Performance in Japanese College Athletes: A Case Study—Measurement of the Athletic Performance, Salivary Biomarkers of Stress, and Mood, Affect Balance. Health. 2017, 9(3), 556–567. DOI: 10.4236/health.2017.93039.
- Schmidt, C.; Geweke, I.; Struck, S.; Zahn, S.; Rohm, H. Blackcurrant Pomace from Juice Processing as Partial Flour Substitute in Savoury Crackers: Dough Characteristics and Product Properties. Int. J. Food Sci. Technol. 2017, 53(1), 237–245. DOI: 10.1111/ijfs.13639.
- Drożdż, W.; Boruczkowska, H.; Boruczkowski, T.; Tomaszewska-Ciosk, E.; Zdybel, E. Use of Blackcurrant and Chokeberry Press Residue in Snack Products. Pol. J. Chem. Technol. 2019, 21(1), 13–19. DOI: 10.2478/pjct-2019-0003.
- Schopf, M.; Scherf, K. A. Water Absorption Capacity Determines the Functionality of Vital Gluten Related to Specific Bread Volume. Foods. 2021, 10, 2. From NLM PubMed-not-MEDLINE. DOI: 10.3390/foods10020228.
- Ortolan, F.; Steel, C. J. Protein Characteristics That Affect the Quality of Vital Wheat Gluten to Be Used in Baking: A Review. Compr. Rev. Food Sci. Food Saf. 2017, 16(3), 369–381. From NLM PubMed-not-MEDLINE. DOI: 10.1111/1541-4337.12259.
- Pourmohammadi, K.; Abedi, E. Enzymatic Modifications of Gluten Protein: Oxidative Enzymes. Food Chem. 2021, 356, 129679. From NLM Medline. DOI: 10.1016/j.foodchem.2021.129679.
- Sharma, N.; Bhatia, S.; Chunduri, V.; Kaur, S.; Sharma, S.; Kapoor, P.; Kumari, A.; Garg, M. Pathogenesis of Celiac Disease and Other Gluten Related Disorders in Wheat and Strategies for Mitigating Them. Front Nutr. 2020, 7, 6. From NLM PubMed-not-MEDLINE. DOI: 10.3389/fnut.2020.00006.
- Kreutz, J. M.; Adriaanse, M. P. M.; van der Ploeg, E. M. C.; Vreugdenhil, A. C. E. Narrative Review: Nutrient Deficiencies in Adults and Children with Treated and Untreated Celiac Disease. Nutrients. 2020, 12(2), 2. From NLM Medline. DOI: 10.3390/nu12020500.
- Korus, J.; Juszczak, L.; Ziobro, R.; Witczak, M.; Grzelak, K.; SÓJka, M. Defatted Strawberry and Blackcurrant Seeds as Functional Ingredients of Gluten-Free Bread. J. Texture Stud. 2012, 43(1), 29–39. DOI: 10.1111/j.1745-4603.2011.00314.x.
- Gagneten, M.; Archaina, D. A.; Salas, M. P.; Leiva, G. E.; Salvatori, D. M.; Schebor, C. Gluten‐free Cookies Added with Fibre and Bioactive Compounds from Blackcurrant Residue. Int. J. Food Sci. Technol. 2020, 56(4), 1734–1740. DOI: 10.1111/ijfs.14798.