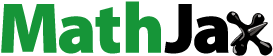
ABSTRACT
Lipophilic micronutrients with health-promoting effects are highly sought after globally. Encapsulation protects these lipophilic micronutrients from oxidative degradation and enables controlled delivery at the targeted absorption site. Pickering emulsion, a particle-stabilized emulsion, has garnered research interest due to its excellent stability and surfactant-free properties. This review provides an up-to-date overview of bio-based Pickering emulsions stabilized by protein-, polysaccharide-, and protein/polysaccharide-based Pickering emulsifiers in delivering lipophilic micronutrients. Firstly, recent developments of bio-based Pickering emulsions in delivering lipophilic micronutrients are presented. The delivery performances of bio-based Pickering emulsions in terms of lipid digestibility and bioaccessibility are highlighted. The pros and cons of the latest characterization techniques for determining the lipid digestion profile and bioaccessibility by using in vitro digestion models of bio-based Pickering emulsions are also presented where the multi-stage static digestion model has advantages such as its ease of operation and relatively cheaper cost over dynamic digestion models. Lastly, the research gaps and suggestions for future research are addressed.
Introduction
According to the United Nations, the world population is estimated to increase from 8 billion to about 10 billion in 2050.[Citation1] The rise of the global population poses a massive challenge to food security, whereby about two billion people are affected by food insecurities worldwide,[Citation2] with Asia, the world’s most populous region, having the highest number of undernourished people. Nutrient security is one of the key elements in the food security.[Citation3] Besides food accessibility and affordability, food must also provide adequate nutrition for individuals to lead healthy lives. However, most of the lipophilic micronutrients are present only in trace amounts in food, and they are scarcely available in some parts of the world. Furthermore, these micronutrients, such as vitamins, tocols, carotenoids, and curcuminoids, are susceptible to oxidative degradation, which adds to the malnutrition conundrum.
As part of the strategy to overcome these nutrient insecurities, incorporating lipophilic micronutrients with various health-enhancing effects into food systems has been receiving burgeoning interest. To date, emulsion-based delivery systems are commonly used for encapsulation, protection, and controlled delivery of lipophilic micronutrients.[Citation4–6] Amphiphilic emulsifiers, such as proteins and molecular surfactants, are generally used to stabilize an oil-in-water emulsion, in which the lipophilic micronutrients are present in the oil phase.[Citation7,Citation8] Nonetheless, surfactant-based emulsions face increasing restrictions owing to the growing demand for green-label products and the need to stabilize and deliver the micronutrients more efficiently.
Pickering emulsion, an emulsion stabilized by solid particles with intermediate wettability, has been garnering increasing research interest owing to its surfactant-free characteristics. The adsorption of solid particles at the oil-water interface creates a thicker interfacial layer that confers superior stability to the Pickering emulsion as compared to the conventional emulsion stabilized by surfactants.[Citation9,Citation10] Bio-based Pickering emulsions, i.e., Pickering emulsions stabilized by solid particles of biological origin, are favorable owing to their biodegradability and food-grade status. Numerous reviews have been published on the formation and application of bio-based Pickering emulsions.[Citation11–14] These reviews mainly focused on the effects of several key parameters, such as particle size, morphology, and surface charge, in governing the emulsion properties, functionalities, and applications. A comprehensive review of the characterization techniques used to evaluate the formation, properties, and stability of Pickering emulsion has also been presented.[Citation15] In a recent review, Sarkar et al. discussed the fundamental colloidal mechanisms involved in modulating the lipid digestion profiles in Pickering emulsions.[Citation16] Different strategies to modulate lipid digestibility and release of bioactives from Pickering emulsion have also been reviewed.[Citation13,Citation17] To the best of our knowledge, no review has discussed the techniques used to characterize the lipid digestibility and bioaccessibility of encapsulated lipophilic micronutrients of bio-based Pickering emulsions. This information is crucial to fully understanding the potential of bio-based Pickering emulsion systems in modulating the delivery and bioaccessibility of lipophilic micronutrients at targeted absorption sites.
Hence, this review intends to provide an up-to-date overview of bio-based Pickering emulsion in delivering lipophilic micronutrients. Firstly, recent developments of bio-based Pickering emulsions in delivering lipophilic micronutrients are presented. The delivery performances of bio-based Pickering emulsions in terms of lipid digestion and bioaccessibility are highlighted. The pros and cons of the latest characterization techniques for determining the lipid digestion profile and bioaccessibility of bio-based Pickering emulsions are subsequently reviewed. Lastly, the research gaps and suggestions for future research are addressed.
Bio-based Pickering emulsion
There has been a growing demand for consuming foods fortified with lipophilic micronutrients owing to their health-promoting effects. Considering the chemical instability of lipophilic micronutrients and the largely aqueous-based human physiology, an oil-in-water emulsion is deemed a suitable choice for improving the stability and bioavailability of these micronutrients. Pickering emulsion, i.e., an emulsion stabilized by solid particles, discovered by Ramsden and Pickering[Citation18,Citation19] in the early 20th century, has captured great research interest owing to its unique features, such as enhanced physical stability[Citation20–23] and improved oxidative stability.[Citation24–27] Furthermore, the excellent stability of Pickering emulsion greatly improved the stability of encapsulated lipophilic micronutrients and enhanced their delivery to the targeted absorption site.[Citation13]
Bio-based Pickering emulsions stabilized by solid particles of biological origin are generally preferred for delivering lipophilic micronutrients owing to the biodegradability and food-grade status of the stabilizing particles. Lately, great attention has been placed on using polysaccharide- and protein-based Pickering emulsifiers to stabilize the emulsion due to their intrinsic biochemical properties that can be exploited to develop ‘designer Pickering emulsions’ for targeted delivery of micronutrients (see ). Targeted delivery is essential to ensure the release of micronutrients at the targeted site of absorption, i.e., the intestine, after passing through various extreme conditions, such as extreme pHs and the presence of digestive enzymes, along the gastrointestinal tract. The lipophilic micronutrients are usually released in the form of mixed micelle upon digestion of the oil phase, a process that can be characterized by the release of free fatty acid (FFA). For any lipophilic micronutrients to provide health benefits to consumers, they must first be available for intestinal absorption which can be quantified by the fraction of encapsulated micronutrients solubilized in the intestinal fluid or better known as bioaccessibility. The developments and performances of bio-based Pickering emulsions in delivering lipophilic micronutrients are highlighted in the following sections.
Table 1. Lipid digestibility and bioaccessibility of bio-based Pickering emulsion in targeted delivery of lipophilic micronutrients.
Protein-based
Pickering emulsifiers from protein sources have gained significant research interest due to their unique biochemical properties and potential for various applications, such as food, pharmaceutical, and cosmetical industries. Proteins have several advantageous features, such as their amphiphilic nature, surface activity, and the ability to form interfacial films.[Citation104,Citation105] These properties enabled the protein-based Pickering emulsifier to stabilize an emulsion by adsorbing at the oil-water interface, forming a physical barrier that prevents droplet coalescence.[Citation106–108] In addition, protein-based Pickering emulsifiers are generally biocompatible and biodegradable. They are abundantly available in natural sources, making them attractive options for green and sustainable emulsion systems.[Citation109,Citation110]
To date, many types of protein-based Pickering emulsifiers, such as kafirin nanoparticles,[Citation38] whey protein isolate particles,[Citation33,Citation35,Citation111] zein particles,[Citation47,Citation49,Citation54,Citation56] ovotransferrin particles,[Citation30–32,Citation52,Citation57] and others have been used in stabilizing and delivering lipophilic micronutrients. For example, astaxanthin is a lipophilic carotenoid possessing potent antioxidant and anti-inflammatory activity.[Citation112,Citation113] However, it is known to be unstable under various environmental stresses, such as high temperature, ionic strength, and light, causing its low bioaccessibility and oral bioavailability.[Citation114,Citation115] In view of this, Ge and co-workers developed a Pickering emulsion stabilized by zein-Adzuki bean seed coat polyphenols nanoparticles to enhance the stability and bioaccessibility of astaxanthin.[Citation49] The covalent nanoparticles were formed via covalent interaction between zein and Adzuki bean seed coat polyphenols under alkaline conditions. The encapsulation of astaxanthin in the zein/Adzuki bean seed coat polyphenols nanoparticles-stabilized Pickering emulsion improved its bioaccessibility by almost three-fold, as compared to that in non-encapsulated forms, i.e., astaxanthin in corn oil. The concentration of Pickering emulsifiers plays a significant role in their dynamic adsorption at the oil/water interface, thereby affecting the interfacial structure of Pickering emulsions and their functionalities. For instance, Jiang et al. modulated the extent of lipolysis and the bioaccessibility of nobiletin (i.e., a lipophilic flavonoid found in citrus peels) by varying the fibril concentration of whey protein isolate fibrils-stabilized Pickering emulsion, see .[Citation33] In their study, the critical overlap concentration (C*) (i.e., the concentration of which the polymer solution transitions from the dilute to the semi-dilute regime as determined by the sudden increase in viscosity) of whey protein fibrils was determined to be at 0.5 wt.%. Below this C*, the fibrils move freely in the aqueous phase, and large emulsion droplets were formed due to insufficient surface coverage. When the concentration was close to C*, the oil droplets were effectively stabilized, forming homogenous emulsion droplets with optimum surface coverage. Above the C*, the fibrils were closer to each other, causing entanglement between them which led to the aggregation of emulsion droplets. Since lipolysis is an interfacial process, the extent of lipolysis strongly depends on the interfacial area of the emulsion droplets with a large interfacial area giving a greater extent of lipolysis, thereby improving the bioaccessibility of nobiletin.
Figure 1. (a) Release profile of FFA and (b) bioaccessibility of nobiletin in Pickering emulsion stabilized by whey protein isolate fibrils after in vitro digestion. (c) Schematic diagram of influence of whey protein isolate fibrils concentration on the emulsion structure and bioaccessibility of nobiletin. Adapted with permission from Jiang, Chen, Wang, Huang, Jin and Huang.[Citation33]
![Figure 1. (a) Release profile of FFA and (b) bioaccessibility of nobiletin in Pickering emulsion stabilized by whey protein isolate fibrils after in vitro digestion. (c) Schematic diagram of influence of whey protein isolate fibrils concentration on the emulsion structure and bioaccessibility of nobiletin. Adapted with permission from Jiang, Chen, Wang, Huang, Jin and Huang.[Citation33]](/cms/asset/b1b0373d-0711-4ebb-a424-7ee83c1f5259/lfri_a_2279585_f0001_oc.jpg)
When an internal phase fraction of a Pickering emulsion exceeds 0.74, the Pickering emulsion is known as a high internal phase Pickering emulsion (HIPPE).[Citation29] The high oil fractions, high viscosity, and gel-like structure of a HIPPE extend the application of Pickering emulsion in the encapsulation, stabilization, and delivery of lipophilic micronutrients.[Citation39,Citation40,Citation42,Citation44,Citation51,Citation53] For instance, Geng and co-workers developed a HIPPE stabilized by soy protein isolate/epigallocatechin-3-gallate covalent composite microgel particles to enhance the stability and bioaccessibility of β-carotene.[Citation53] The incorporation of epigallocatechin-3-gallate, a polyphenolic compound extracted from green tea, with potent antioxidant and anti-tumor properties in the microgel particles successfully improved the β-carotene retention in the HIPPE by about 11%, as compared to that stabilized by soy protein isolate particles only. Interestingly, with the increasing concentration of epigallocatechin-3-gallate used, the extent of lipolysis and bioaccessibility of β-carotene also increases. The thick particle layer not only protects the β-carotene from degradation in acidic gastric conditions but also provides steric hindrance that hinders the aggregation of oil droplets, thereby facilitating the release of β-carotene from the oil droplets to the micelles. In a recent attempt, Li and co-workers employed a co-encapsulation method, i.e., by co-encapsulating β-carotene and puerarin in a meat protein-stabilized HIPPE, to improve the bioaccessibility of puerarin, a natural flavonoid with excellent pharmacological properties.[Citation39] The authors reported delayed oil digestion in the meat protein-stabilized HIPPE as compared to the bulk oil due to the presence of meat protein particles at the oil/water interface that restricted the access of lipase to reach the oil droplets. The co-encapsulation of β-carotene provided protection to the puerarin in gastrointestinal conditions, thereby increasing the bioaccessibility of puerarin by ~ 1.5 fold as compared to HIPPE loaded with puerarin only.
Oleogels composed of organic liquid phases and oleogelators (e.g., candelilla wax, carnauba wax, beeswax, and others) have been widely used in the food industry as fat replacers and delivery carriers for nutraceuticals.[Citation116,Citation117] Recently, oleogel-based Pickering emulsions have captured increasing research attention due to their excellent stability and ability to improve the bioaccessibility of bioactives.[Citation32,Citation41,Citation64,Citation95] For instance, the bioaccessibility of astaxanthin in an oleogel-based Pickering emulsion stabilized by ovalbumin fibrils improved by almost 2-fold, as compared to that in an oleogel system.[Citation41] The astaxanthin was loaded in an oil phase composed of candelilla wax and soybean oil, and ovalbumin fibrils formed by acid-heat treatment were employed as the Pickering emulsifier. The improved bioaccessibility in the oleogel-based Pickering emulsion could be attributed to three reasons: (i) better fluidity and dispersion of oleogel-based Pickering emulsion in aqueous-based digestive fluids, as compared to the oleogel that agglomerated into lumps in the fluids and reduced the surface contact area for lipase and lipids, (ii) smaller emulsion droplet size of oleogel-based Pickering emulsion which gives a much larger specific surface area for lipase-lipid attachment, and (iii) disruption of ovalbumin fibrils as a result of proteolysis that promoted rapid lipolysis due to the ease of contact between lipids and lipase. In another work, Xiao and co-workers immobilized kafirin nanoparticles-stabilized Pickering emulsion in an alginate hydrogel matrix to enhance the bioaccessibility of curcumin.[Citation38] Although the alginate hydrogel matrices successfully protected the protein-stabilized Pickering emulsion from harsh processing conditions and the pre-mature release of curcumin during gastric digestion, the bioaccessibility of curcumin decreased with increasing alginate content. A large amount of curcumin resided in the disintegrated gel residues upon intestinal digestion, thereby lowering the bioaccessibility of curcumin.
Polysaccharide-based
Polysaccharide-based colloidal particles from various sources, such as cellulose, chitosan, and starch, have been extensively investigated as Pickering emulsifiers in developing Pickering emulsions as carrier systems for lipophilic micronutrients.[Citation58–60,Citation64,Citation70,Citation118] These polysaccharide-based Pickering emulsifiers are generally preferred due to their natural abundance, biocompatibility, biodegradability, and non-toxicity. The adsorption of polysaccharide-based Pickering emulsifiers at the oil/water interface forms a physical barrier that not just inhibits droplet coalescence, but also delays lipolysis.[Citation59,Citation60,Citation65,Citation73,Citation118] The performance of Pickering emulsion in lipolysis and bioaccessibility can be manipulated by varying the concentration of Pickering emulsifiers and oil fractions used.[Citation59,Citation65,Citation75] For instance, Winuprasith and co-workers investigated the effect of nanofibrillated mangosteen cellulose (NFC) concentration on the lipid digestibility and bioaccessibility of vitamin D3.[Citation65] The authors reported that the lipolysis in Pickering emulsion decreased with increasing NFC concentration (see ), and this can be explained by three mechanisms: (i) increased particle concentration resulted in a lower lipid surface area available for lipolysis, (ii) the NFC formed a protective coating around lipid droplets that inhibits the access of lipase and bile salts to the lipid surface (see ), and (iii) aggregated NFC networks increased the overall viscosity of the emulsion, thereby slowing down the movement of lipase molecules towards the droplet surfaces. It was worth highlighting that the extent of lipolysis in NFC-stabilized Pickering emulsion was lower than that of the whey protein isolate-stabilized emulsion due to the larger droplet surfaces provided by the NFC-stabilized Pickering emulsion, which reduces the accessibility of lipase. Concomitantly, the bioaccessibility of vitamin D3 decreases with increasing NFC concentration due to the inhibition of lipolysis by NFC which causes some of the vitamin D3 to remain in the undigested lipid phase. In a recent work, Li and co-workers demonstrated that the lipolysis and bioaccessibility of β-carotene can be manipulated by varying oil fractions used in Pickering emulsion stabilized by chitosan hydrochloride/carboxymethyl starch nanogels.[Citation75] By maintaining the particle concentration at 1.5% (w/v), the Pickering emulsion with an oil fraction of 0.1 exhibited a lower release of FFA than the Pickering emulsion with an oil fraction of 0.5. The tightly packed droplets at high oil fractions increased the viscosity of the Pickering emulsion, thereby forming a gel-like matrix that inhibits the accessibility of lipases to oil surfaces. As a result, the bioaccessibility of β-carotene in Pickering emulsion with high oil fractions was reduced as compared to that with low oil fractions.
Figure 2. (a) Effect of emulsifier concentration on the release of FFA from NFC-stabilized Pickering emulsion. (b) schematic diagram illustrating the effect of NFC concentration on lipase and bile salts inhibition. Adapted with permission from Winuprasith, Khomein, Mitbumrung, Suphantharika, Nitithamyong and McClement.[Citation65].
![Figure 2. (a) Effect of emulsifier concentration on the release of FFA from NFC-stabilized Pickering emulsion. (b) schematic diagram illustrating the effect of NFC concentration on lipase and bile salts inhibition. Adapted with permission from Winuprasith, Khomein, Mitbumrung, Suphantharika, Nitithamyong and McClement.[Citation65].](/cms/asset/c3cc733f-a601-481c-a958-25f00f1d2c7c/lfri_a_2279585_f0002_oc.jpg)
Recently, an enzymatically modified quinoa starch (EMQS)-based Pickering emulsion gel was fabricated as a delivery system to improve the stability and bioaccessibility of curcumin.[Citation69] The enzymatic modification greatly improved the emulsifying property of quinoa starch, which was hydrophilic by nature. The EMQS Pickering emulsion gel provided better protection for curcumin from UV degradation than Tween 80 emulsion due to the rigid solid particle at the oil/water interface. After an in vitro gastrointestinal digestion, the lipid digestion extent and the bioaccessibility of curcumin of the EMQS Pickering emulsion gel system were lower than that of Tween 80 emulsion but higher than that of bulk oil due to the different droplet sizes of the three systems which affected the interfacial area available for lipase attachment. Besides the particle types, the lipid phase composition can also be varied to improve the stability and bioaccessibility of lipophilic bioactives. For instance, Qi and colleagues developed an oleogel-in-water Pickering emulsion stabilized by cellulose nanocrystals to improve the bioaccessibility of β-carotene.[Citation64] The oleogel was formed by structuring the soybean oil with beeswax. The oleogel-based Pickering emulsion remarkably enhanced the stability and bioaccessibility of β-carotene due to its gel-like nature that formed a physical barrier to isolate oxidative species from reaching the β-carotene as well as to delay lipolysis.
Protein/polysaccharide-based
Proteins and polysaccharides have complementary characteristics that make them ideal candidates for complexation.[Citation119,Citation120] For example, proteins possess amphiphilic properties that allow them to adsorb at the oil/water interface to stabilize an emulsion. However, proteins are prone to pH and thermal denaturation.[Citation121,Citation122] On the other hand, polysaccharides, which are hydrophilic in nature, require modifications to confer them with a higher affinity towards the oil/water interface.[Citation123] The complexation of proteins and polysaccharides enabled a more effective stabilization of Pickering emulsion and provided superior protection to the encapsulated lipophilic micronutrients.[Citation82,Citation88,Citation98,Citation100] For example, the complexation between xanthan gum and whey gluten nanoparticles-stabilized Pickering emulsion improved the creaming stability of the Pickering emulsion and enhanced the protection for encapsulated β-carotene against chemical degradation.[Citation88] This can be attributed to the formation of a thick highly charged biopolymer layer around the oil droplets which provided a steric barrier that inhibited droplet coalescence as well as the diffusion of oxidative species into the droplets. The complexation of xanthan gum and wheat gluten nanoparticles also improved the bioaccessibility of β-carotene in the Pickering emulsion by almost 2-fold, as compared to that without xanthan gum complexation. The presence of xanthan gum around the oil droplets reduced the aggregation of the Pickering emulsion in the gastrointestinal fluid, thereby allowing the lipase molecules to access a bigger surface area of lipids for lipolysis to begin and increasing the solubilization of β-carotene into the micelle phase. Besides complexation, both proteins and polysaccharides can also be simultaneously employed as stabilizers to a Pickering emulsion. For instance, the combination of the spherical zein particles and rod-shaped cellulose nanocrystals yielded Pickering emulsions with enhanced stability, delayed lipolysis, and sustained micronutrient release.[Citation81] Individually, neither the zein particles nor the cellulose nanocrystals can stabilize the Pickering emulsion due to their inappropriate wettability. The mass ratio and addition sequence of both types of particles play an important role in affecting the degree of lipolysis and subsequently, the bioaccessibility of encapsulated β-carotene.
Protein/polysaccharide-based nanoparticles have also been applied as Pickering emulsifiers to form HIPPEs with excellent stability.[Citation89–91,Citation97,Citation102] In the work of Wang et. al, HIPPEs prepared using different mass ratios of ovalbumin and pectin were evaluated for their stability under various environmental stresses as well as their bioaccessibility of encapsulated curcumin.[Citation94] At different mass ratios, the interactions between ovalbumin and pectin differ, thus imparting different emulsification capabilities of the complexes. Besides that, the complexes of different mass ratios also modulated the rheological properties of the HIPPEs, further affecting the stability of emulsions. Their results showed that HIPPEs prepared using a 1:1 mass ratio of ovalbumin and pectin exhibited the most superior stability when subjected to harsh ionic strength and thermal treatments. The smallest droplet size formed at the mass ratio of 1:1 resulted in the highest FFA release and bioaccessibility of curcumin. Besides HIPPEs, protein/polysaccharide-based particles have also been employed as Pickering emulsifiers to stabilize Pickering emulsion gels. Briefly Pickering emulsion gel is a system that combines both the benefits of Pickering emulsions and gels, which have superior stability than Pickering emulsions alone.[Citation78,Citation79,Citation87,Citation95,Citation101] For instance, Lei et. al developed a Pickering emulsion gel by using zein hydrolysate-chitin nanocrystals coacervates as a Pickering emulsifier.[Citation78] The coacervates had better emulsifying properties than the individual particles due to the absorption of hydrophobic zein hydrolysate on the hydrophilic chitin nanocrystals. The aggregation of the coacervates anchored at the oil droplets formed dense interfacial layers that greatly increased the viscoelasticity of the emulsion, hence forming a Pickering emulsion gel. In addition, the thick interfacial layers of the Pickering emulsion gel also reduced the lipid hydrolysis by about 4-fold as compared to chitin nanocrystals-stabilized Pickering emulsion.[Citation78] Interestingly, the bioaccessibility of curcumin was improved by about 1.5-fold, despite the reduction in lipid hydrolysis, as the encapsulated curcumin was well protected from decomposition by the intestinal fluids.
Recently, an interesting work by Yan et al. reported on the formation of a double-crosslinked Pickering emulsion gel using zein and sodium alginate as gelling agents for simultaneous encapsulation of two lipophilic micronutrients, i.e., curcumin and resveratrol.[Citation79] The double-crosslinked Pickering emulsion gel was first prepared by preparation of the self-assembled zein nanoparticles-stabilized Pickering emulsion, followed by the addition of alginate into the continuous aqueous phase of the Pickering emulsion. Subsequently, the single/double-crosslinked Pickering emulsion gels were formed by the addition of transglutaminase (TG, to crosslink with zein) and/or Ca2+ (to crosslink with alginate), as illustrated in . While TG mainly acted on the zein particles that are anchored at the oil/water interfaces, leading to a rigid and tightly bound interfacial coating; Ca2+, on the other hand, crosslinked with alginate molecules through an egg-box mechanism, forming alginate hydrogel structure. Under the double-crosslinking method, the anionic alginate network in the aqueous phase trapped the cationic zein-coated oil droplets within its matrix, creating a 3D structure with uniform microstructures. As a result, the double-crosslinked Pickering emulsion gels provided better protection of the two encapsulated micronutrients, and the bioaccessibilities of lipophilic micronutrients were improved as compared to the single-crosslinked Pickering emulsion ().
Figure 3. (a) Illustration of formation mechanism and possible structures of the single/double-crosslinked pickering emulsion gels. (b) Bioaccessibility of curcumin and resveratrol in the different pickering emulsion gels systems. Adapted with permission from Yan, Liang, Ma, McClements, Liu and Liu.[Citation79]
![Figure 3. (a) Illustration of formation mechanism and possible structures of the single/double-crosslinked pickering emulsion gels. (b) Bioaccessibility of curcumin and resveratrol in the different pickering emulsion gels systems. Adapted with permission from Yan, Liang, Ma, McClements, Liu and Liu.[Citation79]](/cms/asset/95625244-dfd3-4f59-87e4-dc6d9ddd20eb/lfri_a_2279585_f0003_oc.jpg)
Characterization of lipid digestion profile and bioaccessibility
Lipid digestibility and bioaccessibility are key concepts related to the release and absorption of lipophilic micronutrients.[Citation124,Citation125] Examining the lipid digestibility and bioaccessibility of the delivery systems is crucial for understanding the true potential of applied systems in nutrient delivery. To date, most characterization of lipid digestibility and bioaccessibility of Pickering emulsion has been carried out using in vitro method, based on two types of digestion models, namely the static digestion model and dynamic digestion model, which can be further categorized into single-stage or multistage (see ).
Static digestion model
The static digestion model is a popular approach to simulate the sequence of events during digestion in the gastrointestinal tract in determining the lipid digestibility and bioaccessibility of micronutrients. In static digestion models, the physical processes of gastrointestinal digestion, such as shearing, mixing, peristalsis movement, hydration, and changes in physiological conditions over time, are not reproduced.[Citation125] The static digestion models can be divided into single-stage or multi-stage digestion models. In a single-stage static digestion model, just one region of the gastrointestinal tract, i.e., the intestine, where lipid digestion and absorption of lipophilic micronutrients occur, was simulated. Although the single-stage static digestion model is the cheapest, easiest, and quickest option in characterizing the lipid digestibility and bioaccessibility of lipophilic micronutrients, a significant deviation from the actual case scenario exists; as ingested foods will pass through the gastric phase with extreme pH conditions, irrespective of carrier types. Consequently, the effect of gastric conditions on the physicochemical properties of the carrier and the stability of encapsulated micronutrients were not examined. This method is rarely adopted by researchers nowadays due to the shortcomings mentioned earlier. Thus far, only one work has reported on the use of a single-stage digestion model in evaluating the bioaccessibility of Pickering emulsion.[Citation74]
A multi-stage static digestion model that simulates both gastric and intestinal regions, with an optional inclusion of the oral region, gives a better representation of the actual fate of a carrier system in the gastrointestinal tract. The vessel-based pH-stat lipolysis model (see ) is one of the most applied models in determining the lipid digestibility and bioaccessibility of bio-based Pickering emulsions.[Citation124,Citation127,Citation128] The simulated gastric and intestinal digestions were usually carried out in sequential steps. Firstly, the simulated gastric fluid (pH 1–3, with or without pepsin) was prepared. Next, the micronutrient-loaded samples were added to the simulated gastric fluid and incubated at 37°C using a water bath, for 2 h under magnetic stirring to simulate the conditions in the human stomach. After the completion of gastric digestion, an equal amount of simulated intestinal fluid (containing bile salt and lipase) was added to the gastric digesta. The intestinal digestion was then carried out at 37°C for another 2 h. During intestinal digestion, FFA was generated by the lipolysis of emulsified triacylglycerols, which reduced the pH of the medium. During the lipolysis process, the pH of the intestinal medium was continuously monitored and maintained at pH 7 using an automatic pH-stat titration system.[Citation30,Citation65] Alternatively, the pH titration can also be carried out in a separate process, whereby the enzymatic reaction in the vessel was stopped at a pre-determined time point using ethanol solution, and the pH of the reaction mixture was adjusted to pH 7 using an autotitrator and phenolphthalein as an indicator.[Citation24] The pH can be adjusted using NaOH as the titrant, and the molarity of the NaOH usually ranges from 0.05 M to 0.25 M.[Citation34,Citation36,Citation68,Citation70] Finally, the lipid digestibility can be determined by the volume of NaOH used during the digestion process, as follow:
Figure 5. Illustration of a multi-stage static digestion model (i.e., pH-stat lipolysis model). Adapted with permission from Berthelsen, Klitgaard, rades and Müllertz.[Citation126]
![Figure 5. Illustration of a multi-stage static digestion model (i.e., pH-stat lipolysis model). Adapted with permission from Berthelsen, Klitgaard, rades and Müllertz.[Citation126]](/cms/asset/769c1f47-637f-4e5e-af3c-c69525df97e2/lfri_a_2279585_f0005_oc.jpg)
Where VNaoH is the volume (L) of NaOH needed to neutralize the FFA generated, mNaoH is the molarity of the NaOH used (in mol·L−1), Mlipid is the molecular weight of the lipid phase, and wlipid is the total weight of lipid initially present. The FFA release data can be fitted into mathematical models () to determine the release mechanism.[Citation24,Citation129] In addition, the extent of lipolysis, as a function of time, t can be determined as follow[Citation70,Citation130]:
Table 2. Brief description of mathematical models that can be used for fitting release data. Adapted with permission from Sarkar and Mackie.[Citation129]
Where max is the lipid fraction digested by the end of the digestion, k is the rate constant (i.e., μmol FFA released per unit droplet surface area per unit time), M is the lipid molar mass, D is the emulsion droplet size after gastric digestion,
is the density of the lipid.
To access the bioaccessibility of the lipophilic micronutrients, the digesta obtained at the end of the digestion process was subjected to a centrifugation process to separate the micellar layer (i.e., the aqueous phase in the middle of the centrifugation tube) (see ) that contains the solubilized lipophilic micronutrient. The concentration of the solubilized micronutrients can be determined by using high-performance liquid chromatography[Citation31,Citation33,Citation60] or UV-Visible spectrophotometry.[Citation28,Citation34,Citation36,Citation37] In some cases, extraction of the micronutrients from the micellar phase was performed using organic solvents, prior to the concentration determination.[Citation37,Citation60,Citation68] The bioaccessibility can be determined as follow:
Where Cmicelle is the concentration of lipophilic micronutrients in the micelle fraction and Cinitial is the concentration of lipophilic micronutrients in the formulation. In some works, bioaccessibility was defined as the percentage of micronutrients present in the solubilized micelle form compared to the raw digesta,[Citation65,Citation73] as follow:
\Where Cmicelle is the concentration of lipophilic micronutrients in the micelle fraction and Cdigesta is the concentration of lipophilic micronutrients in the raw digesta obtained at the end of the gastrointestinal digestion.
The multi-stage static digestion model allows a quick comparison of the lipid digestibility and bioaccessibility between different formulations under similar experimental conditions. Usually, a constant ratio of formulation to enzymes, a constant ionic strength, and a constant pH at each digestive stage are used to allow elucidation of the effect of different physicochemical properties of the emulsion systems on lipid digestibility and bioaccessibility. McClements and Li reviewed the role of the major components of the simulated intestinal fluid on lipid digestibility as determined using a static digestion model and proposed a standardized pH-stat method for testing the lipid digestibility of emulsion-based systems under fed state conditions ().[Citation127] In fact, a group of researchers from more than 35 countries and different backgrounds (i.e., food science, nutrition, engineering, gastroenterology, and enzymology) has come to a consensus on a harmonized digestion protocol, INFOGEST 2.0 that simulates gastrointestinal digestion for a mechanistic understanding on the impact of food structure on human health.[Citation128] Although the multi-stage static digestion model with standardized protocols can ensure good reproducibility with ease of assessment for each digestion phase, it is incapable of mimicking the complexity of an actual digestion process, such as the peristaltic motion of the gastrointestinal tract, the different gastrointestinal emptying times, biochemical secretions, and the gradual elimination from absorption site. As a result, the static digestion model tends to overestimate the bioaccessibility of a delivery system.[Citation30,Citation60,Citation63,Citation118]
Dynamic digestion model
The TNO Gastro-Intestinal Model (TIM) is a dynamic digestion model developed in the early 1990s by TNO Quality of Life from the Netherlands to meet the industrial demand for a more physiologically relevant simulation of the digestive systems as compared to the contemporary digestion models.[Citation131] TIM enables the simulation of dynamic conditions in the gastrointestinal lumen, such as the gradual secretion of digestive fluids and the absorption of water and nutrients, during the gradual transit of the ingested foods through the tract. Specifically, TIM-1 is the most frequently used dynamic digestion model in determining the bioaccessibility of lipophilic micronutrients in Pickering emulsion systems.[Citation30,Citation60,Citation63,Citation118] TIM-1 is comprised of four successive compartments, representing the stomach, duodenum, jejunum, and ileum, which are connected by peristaltic valve pumps to allow the controlled transfer of chyme from one phase to another (see ). The secretion of digestive enzymes and relevant cofactors, the pH change in each compartment, the peristaltic movements, the physiological transit time, the mixing in each compartment, and the passive absorption of water and nutrients are precisely controlled by computer programs based on real-time human physiological data.[Citation30,Citation131] The temperature of the digestion system is maintained at 37°C using a water-jacketed system to simulate human temperature. To begin the analysis, emulsion samples were pre-mixed with gastric electrolyte solution and gastric start residue prior to feeding into the gastric compartment through a funnel. A complete digestion run using the TIM-1 digestion model usually lasts for 6 h, with the jejunal and ileal filtrates which passed through hollow fiber membranes being collected periodically and analyzed using chromatographic methods to determine the bioaccessibility of micronutrients. The volume of the jejunal and ileal filtrates was also recorded. The periodic bioaccessibility at the specific compartment can then be determined based on the filtrate volume and concentration of micronutrients in the filtrate. Although the dynamic digestion model is effective in reproducing real-life digestion conditions, its application in accessing the bioaccessibility in Pickering emulsion-based systems was uncommon with only a few works being reported to date.[Citation30,Citation60] The high cost of the dynamic digestion model could have hindered its popularization among academic researchers. Furthermore, the complexity of the dynamic digestion model may impose a high chance of data inconsistency.
Figure 6. Schematic representation of TIM-1, equipped with filters to study the bioaccessibility of lipophilic micronutrients. A. gastric compartment; B. pyloric sphincter; C. duodenal compartment; D. peristaltic valve; E. jejunal compartment; F. peristaltic valve; G. ileal compartment; H. ileal-cecal valve; I. gastric secretion; J. duodenal secretion; K. bicarbonate secretion; L. pre-filter; M. filtration system; N. filtrate with bioaccessible fraction; O. hollow fiber system (cross-section); P. pH electrodes; Q. level sensors; R. temperature sensors; S. pressure sensor. Adapted with permission from Minekus.[Citation131]
![Figure 6. Schematic representation of TIM-1, equipped with filters to study the bioaccessibility of lipophilic micronutrients. A. gastric compartment; B. pyloric sphincter; C. duodenal compartment; D. peristaltic valve; E. jejunal compartment; F. peristaltic valve; G. ileal compartment; H. ileal-cecal valve; I. gastric secretion; J. duodenal secretion; K. bicarbonate secretion; L. pre-filter; M. filtration system; N. filtrate with bioaccessible fraction; O. hollow fiber system (cross-section); P. pH electrodes; Q. level sensors; R. temperature sensors; S. pressure sensor. Adapted with permission from Minekus.[Citation131]](/cms/asset/f26ba3e1-8bdb-4370-a7b1-03f1c26cbcd3/lfri_a_2279585_f0006_oc.jpg)
Research gaps and future direction
Characterizing the lipid digestibility and bioaccessibility of bio-based Pickering emulsion is a crucial step to reveal the true potential of the delivery system in delivering lipophilic micronutrients to the targeted absorption site. On some occasions, researchers investigated the in vitro digestive behavior of the Pickering emulsions at different digestion phases, by examining the changes in particle sizes, surface charges, and morphologies of the Pickering emulsions, to provide better insights into the gastrointestinal fate of the Pickering emulsion systems.[Citation34,Citation84,Citation85,Citation90] This helps researchers establish a clear understanding of the relationship between the physicochemical properties and the lipid digestibility and bioaccessibility of Pickering emulsion systems upon gastrointestinal digestion. To ensure the delivered lipophilic micronutrients can exhibit health effects upon absorption by the body, the stability of the lipophilic micronutrients must be well-retained. Nevertheless, previous studies have largely focused on the storage stability of lipophilic micronutrients and the physicochemical stability of the Pickering emulsion systems under various environmental factors, such as ionic strength, pH, light, and temperature.[Citation45,Citation56,Citation85] The number of studies that determined the stability of lipophilic micronutrients upon gastrointestinal digestion remains limited.[Citation45,Citation55,Citation94] Generally, the stability of solubilized lipophilic micronutrients in the micelle phase of the digesta can be evaluated by using in vitro cell cultures.[Citation43,Citation118] For example, Lu and colleagues reported on the anti-carcinogenesis effect of curcumin in the micelle phase of a milled starch particle-stabilized Pickering emulsion on the Caco-2 cells model.[Citation118] Their work showed that the milled starch particle-stabilized Pickering emulsion not only improves the bioaccessibility of curcumin by about 2.5-fold as compared to that in bulk oil but also retains the stability of curcumin upon gastrointestinal digestion. Future works can consider the use of in vivo studies to further elucidate the absorption of delivered lipophilic micronutrients in bio-based Pickering emulsion.
Conclusion
Lipophilic micronutrients with health-enhancing effects are in great demand all over the world. Yet, they are scarcely available and are susceptible to oxidative degradation in their bare forms. In view of that, encapsulating them in an emulsion (i.e., an oil-in-water system) provides protection and enables controlled delivery of these lipophilic micronutrients. Pickering emulsion, a particle-stabilized emulsion, has been extensively researched owing to its surfactant-free characteristics. Bio-based Pickering emulsions stabilized by protein-, polysaccharide-, and protein/polysaccharide-based Pickering emulsifiers are particularly preferred for their food-grade properties. The excellent stability of Pickering emulsions not only improves the stability of encapsulated lipophilic micronutrients but also enhances their delivery to the targeted absorption site. The delivery performances of the bio-based Pickering emulsions can be evaluated by determining their lipid digestibility and bioaccessibility of encapsulated micronutrients. The characterization techniques for lipid digestibility and bioaccessibility have been highlighted in this review, with special emphasis on the in vitro digestion models used. The most generally used in vitro digestion model is the multi-stage static digestion model due to its ease of operation and relatively cheaper cost, as compared to the dynamic digestion model. Future works should consider the use of in vivo studies to validate the absorption of lipophilic micronutrients delivered via bio-based Pickering emulsion. This review can serve as a guide to researchers to understand the latest development in bio-based Pickering emulsion in the delivery of lipophilic micronutrients, as well as the characterization techniques for lipid digestibility and bioaccessibility.
Nomenclature
FFA | = | Free fatty acid |
CHC-CMS | = | Chitosan hydrochloride/carboxymethyl starch complex |
HIPPE | = | High internal phase Pickering emulsion |
NFC | = | Nanofibrillated mangosteen cellulose |
EMQS | = | Enzymatically modified quinoa starch |
TG | = | Transglutaminase |
VNaOH | = | Volume of NaOH |
mNaOH | = | Molarity of NaOH |
Mlipid | = | Molecular weight of the lipid phase |
wlipid | = | Total weight of lipid |
= | Extent of lipolysis | |
= | Lipid fraction digested by the end of the digestion | |
k | = | rate constant |
M | = | Lipid molar mass |
D | = | Emulsion droplet size |
ρ | = | Density of the lipid |
cmicelle | = | Concentration of lipophilic micronutrients in the micelle fraction |
cinitial | = | Concentration of lipophilic micronutrients in the formulation |
TIM | = | TNO Gastro-Intestinal Model |
Acknowledgments
This work was supported by Fundamental Research Grant Scheme (FRGS) from Ministry of Higher Education, Malaysia (FRGS/1/2018/TK05/MUSM/03/1).
Disclosure statement
No potential conflict of interest was reported by the author(s).
Additional information
Funding
References
- Nations, U. World Population Prospects 2022; Department of Economic and Social Affairs: New York, 2022.
- FAO. The State of Food Security and Nutrition in the World 2019: Safeguarding Against Economic Slowdowns and Downturns; Rome: Food & Agriculture Org, 2019.
- Hwalla, N.; El Labban, S.; Bahn, R. A. Nutrition Security is an Integral Component of Food Security. Front. Life Sci. 2016, 9(3), 167–172. DOI: 10.1080/21553769.2016.1209133.
- Anal, A. K.; Shrestha, S.; Sadiq, M. B. Biopolymeric-Based Emulsions and Their Effects During Processing, Digestibility and Bioaccessibility of Bioactive Compounds in Food Systems. Food Hydrocolloids. 2019, 87, 691–702. DOI: 10.1016/j.foodhyd.2018.09.008.
- Araiza-Calahorra, A.; Akhtar, M.; Sarkar, A. Recent Advances in Emulsion-Based Delivery Approaches for Curcumin: From Encapsulation to Bioaccessibility. Trends Food Sci. Technol. 2018, 71, 155–169. DOI: 10.1016/j.tifs.2017.11.009.
- McClements, D. J. Enhanced Delivery of Lipophilic Bioactives Using Emulsions: A Review of Major Factors Affecting Vitamin, Nutraceutical, and Lipid Bioaccessibility. Food Funct. 2018, 9(1), 22–41. DOI: https://doi.org/10.1039/c7fo01515a.
- Lu, W.; Kelly, A. L.; Miao, S. Emulsion-Based Encapsulation and Delivery Systems for Polyphenols. Trends Food Sci. Technol. 2016, 47, 1–9. DOI: 10.1016/j.tifs.2015.10.015.
- McClements, D. J.; Decker, E. A.; Weiss, D. J. Emulsion‐Based Delivery Systems for Lipophilic Bioactive Components. Journal Of Food Science. 2007, 72(8), R109–R124. DOI: 10.1111/j.1750-3841.2007.00507.x.
- Murray, B. S. Pickering Emulsions for Food and Drinks. Curr. Opin. Food Sci. 2019, 27, 57–63. DOI: 10.1016/j.cofs.2019.05.004.
- Berton-Carabin, C. C.; Schroën, K. Pickering Emulsions for Food Applications: Background, Trends, and Challenges. Annual Review of Food Science and Technology. Ann. Rev. Food Sci. Technol. 2015, 6(1), 263–297. DOI: https://doi.org/10.1146/annurev-food-081114-110822.
- Yang, Y.; Fang, Z.; Chen, X.; Zhang, W.; Xie, Y.; Chen, Y.; Liu, Z.; Yuan, W. An Overview of Pickering Emulsions: Solid-Particle Materials, Classification, Morphology, and Applications. Front. Pharmacol. 2017, 8, 287. DOI: 10.3389/fphar.2017.00287.
- Albert, C.; Beladjine, M.; Tsapis, N.; Fattal, E.; Agnely, F.; Huang, N. Pickering Emulsions: Preparation Processes, Key Parameters Governing Their Properties and Potential for Pharmaceutical Applications. J. Controlled Release. 2019, 309, 302–332. DOI: 10.1016/j.jconrel.2019.07.003.
- Mwangi, W. W.; Lim, H. P.; Low, L. E.; Tey, B. T.; Chan, E. S. Food-Grade Pickering Emulsions for Encapsulation and Delivery of Bioactives. Trends Food Sci. Technol. 2020, 100, 320–332. DOI: 10.1016/j.tifs.2020.04.020.
- Lam, S.; Velikov, K. P.; Velev, O. D. Pickering Stabilization of Foams and Emulsions with Particles of Biological Origin. Curr. Opin. Colloid Interface Sci. 2014, 19(5), 490–500. DOI: 10.1016/j.cocis.2014.07.003.
- Low, L. E.; Siva, S. P.; Ho, Y. K.; Chan, E. S.; Tey, B. T. Recent Advances of Characterization Techniques for the Formation, Physical Properties and Stability of Pickering Emulsion. Adv. Coll. Interf. Sci. 2020, 277, 102117. DOI: 10.1016/j.cis.2020.102117.
- Sarkar, A.; Zhang, S.; Holmes, M.; Ettelaie, R. Colloidal Aspects of Digestion of Pickering Emulsions: Experiments and Theoretical Models of Lipid Digestion Kinetics. Adv. Coll. Interf. Sci. 2019, 263, 195–211. DOI: 10.1016/j.cis.2018.10.002.
- Santos, T. P.; Okuro, P. K.; Cunha, R. L. Pickering Emulsions as a Platform for Structures Design: Cutting-Edge Strategies to Engineer Digestibility. Food Hydrocolloids. 2021, 116, 106645. DOI: 10.1016/j.foodhyd.2021.106645.
- Ramsden, W. Separation of Solids in the Surface-Layers of Solutions and ‘Suspensions’(observations on Surface-Membranes, Bubbles, Emulsions, and Mechanical Coagulation).—Preliminary Account. Proc Royal Soc London. 1904, 72(477–486), 156–164. DOI: 10.1098/rspl.1903.0034.
- Pickering, S. U. CXCVI.—Emulsions. J Chem Soc Trans. 1907, 91, 2001–2021. DOI: 10.1039/CT9079102001.
- Mwangi, W. W.; Ho, K.-W.; Tey, B.-T.; Chan, E.-S. Effects of Environmental Factors on the Physical Stability of Pickering-Emulsions Stabilized by Chitosan Particles. Food Hydrocolloids. 2016, 60, 543–550. DOI: 10.1016/j.foodhyd.2016.04.023.
- Ho, K. W.; Ooi, C. W.; Mwangi, W. W.; Leong, W. F.; Tey, B. T.; Chan, E.-S. Comparison of Self-Aggregated Chitosan Particles Prepared with and without Ultrasonication Pretreatment as Pickering Emulsifier. Food Hydrocolloids. 2016, 52, 827–837. DOI: 10.1016/j.foodhyd.2015.08.019.
- Timgren, A.; Rayner, M.; Dejmek, P.; Marku, D.; Sjöö, M. Emulsion Stabilizing Capacity of Intact Starch Granules Modified by Heat Treatment or Octenyl Succinic Anhydride. Food Science & Nutrition. 2013, 1(2), 157–171. DOI: 10.1002/fsn3.17.
- Low, L. E.; Tey, B. T.; Ong, B. H.; Chan, E. S.; Tang, S. Y. Palm Olein-In-Water Pickering Emulsion Stabilized by Fe3O4-Cellulose Nanocrystal Nanocomposites and Their Responses to pH. Carbohydr. Polym. 2017, 155, 391–399. DOI: 10.1016/j.carbpol.2016.08.091.
- Surjit Singh, C. K.; Lim, H.-P.; Tey, B.-T.; Chan, E.-S. Spray-Dried Alginate-Coated Pickering Emulsion Stabilized by Chitosan for Improved Oxidative Stability and in vitro Release Profile. Carbohydr. Polym. 2021, 251, 117110. DOI: 10.1016/j.carbpol.2020.117110.
- Wang, L.-J.; Hu, Y.-Q.; Yin, S.-W.; Yang, X.-Q.; Lai, F.-R.; Wang, S.-Q. Fabrication and Characterization of Antioxidant Pickering Emulsions Stabilized by Zein/Chitosan Complex Particles (ZCPs). J. Agric. Food Chem. 2015, 63(9), 2514–2524. DOI: 10.1021/jf505227a.
- Xiao, J.; Li, C.; Huang, Q. Kafirin Nanoparticle-Stabilized Pickering Emulsions as Oral Delivery Vehicles: Physicochemical Stability and in vitro Digestion Profile. J. Agric. Food Chem. 2015, 63(47), 10263–10270. DOI: 10.1021/acs.jafc.5b04385.
- Kargar, M.; Fayazmanesh, K.; Alavi, M.; Spyropoulos, F.; Norton, I. T. Investigation into the Potential Ability of Pickering Emulsions (Food-Grade Particles) to Enhance the Oxidative Stability of Oil-In-Water Emulsions. J. Coll. Interf. Sci. 2012, 366(1), 209–215. DOI: 10.1016/j.jcis.2011.09.073.
- Tan, H.; Zhao, L.; Tian, S.; Wen, H.; Gou, X.; Ngai, T. Gelatin Particle-Stabilized High-Internal Phase Emulsions for Use in Oral Delivery Systems: Protection Effect and in vitro Digestion Study. J. Agric. Food Chem. 2017, 65(4), 900–907. DOI: 10.1021/acs.jafc.6b04705.
- Abdullah; Weiss, J.; Ahmad, T.; Zhang, <. A.; Zhang, H. A Review of Recent Progress on High Internal-Phase Pickering Emulsions in Food Science. Trends Food Sci. Technol. 2020, 106, 91–103. DOI: 10.1016/j.tifs.2020.10.016.
- Wei, Z.; Zhu, J.; Cheng, Y.; Huang, Q. Ovotransferrin Fibril–Stabilized Pickering Emulsions Improve Protection and Bioaccessibility of Curcumin. Food Res. Int. 2019, 125, 108602. DOI: 10.1016/j.foodres.2019.108602.
- Wei, Z.; Huang, Q. Developing Organogel-Based Pickering Emulsions with Improved Freeze-Thaw Stability and Hesperidin Bioaccessibility. Food Hydrocolloids. 2019, 93, 68–77. DOI: 10.1016/j.foodhyd.2019.01.050.
- Zhou, Q.; Wei, Z.; Xu, Y.; Xue, C. Fibrous and Spherical Aggregates of Ovotransferrin as Stabilizers for Oleogel-Based Pickering Emulsions: Preparation, Characteristics and Curcumin Delivery. Gels. 2022, 8(8), 517. DOI: 10.3390/gels8080517.
- Jiang, F.; Chen, C.; Wang, X.; Huang, W.; Jin, W.; Huang, Q. Effect of Fibril Entanglement on Pickering Emulsions Stabilized by Whey Protein Fibrils for Nobiletin Delivery. Foods. 2022, 11(11), 1626. DOI: 10.3390/foods11111626.
- Hu, Y.; Tan, Y.; McClements, D. J.; Wang, L. Fabrication, Characterization and in vitro Digestive Behavior of Pickering Emulsion Incorporated with Dextrin. Food Chem. 2022, 384, 132528. DOI: 10.1016/j.foodchem.2022.132528.
- Xu, X.; Zhang, Z.; Zhu, J.; Wang, D.; Liu, G.; Liang, L.; Zhang, J.; Liu, X.; Li, Y.; Ren, J., et al. Whey Protein Isolate Nanofibers Prepared by Subcritical Water Stabilized High Internal Phase Pickering Emulsion to Deliver Curcumin. Foods. 2022, 11(11), 1625.
- Cheng, C.; Gao, Y.; Wu, Z.; Miao, J.; Gao, H.; Ma, L.; Zou, L.; Peng, S.; Liu, C.; Liu, W. Gliadin Nanoparticles Pickering Emulgels for β-Carotene Delivery: Effect of Particle Concentration on the Stability and Bioaccessibility. Molecules. 2020, 25(18), 4188. DOI: 10.3390/molecules25184188.
- Liang, L.; Zhu, J.; Zhang, Z.; Liu, Y.; Wen, C.; Liu, X.; Zhang, J.; Li, Y.; Liu, R.; Ren, J.; et al. Pickering Emulsion Stabilized by Tea Seed Cake Protein Nanoparticles as Lutein Carrier. Foods. 2022, 11(12), 1712. DOI: 10.3390/foods11121712.
- Xiao, J.; Shi, C.; Li, Y.; Pan, Y.; Huang, Q. Pickering Emulsions Immobilized within Hydrogel Matrix with Enhanced Resistance Against Harsh Processing Conditions and Sequential Digestion. Food Hydrocolloids. 2017, 62, 35–42. DOI: 10.1016/j.foodhyd.2016.07.025.
- Li, R.; Yuan, G.; Li, D.; Xu, C.; Du, M.; Tan, S.; Liu, Z.; He, Q.; Rong, L.; Li, J. Enhancing the Bioaccessibility of Puerarin Through the Collaboration of High Internal Phase Pickering Emulsions with β-Carotene. Food Funct. 2022, 13(5), 2534–2544. DOI: 10.1039/D1FO03697A.
- Zhang, L.; Zaky, A. A.; Zhou, C.; Chen, Y.; Su, W.; Wang, H.; Abd El-Aty, A. M.; Tan, M. High Internal Phase Pickering Emulsion Stabilized by Sea Bass Protein Microgel Particles: Food 3D Printing Application. Food Hydrocolloids. 2022, 131, 107744. DOI: 10.1016/j.foodhyd.2022.107744.
- Wang, Z.; Gao, Y.; Wei, Z.; Xue, C. Ovalbumin Fibril-Stabilized Oleogel-Based Pickering Emulsions Improve Astaxanthin Bioaccessibility. Food Res. Int. 2022, 161, 111790. DOI: 10.1016/j.foodres.2022.111790.
- Li, Z.; Wang, Y.; Luo, Y. High Internal Phase Pickering Emulsions Stabilized by Egg Yolk Low Density Lipoprotein for Delivery of Curcumin. Colloids And Surfaces B: Biointerfaces. 2022, 211, 112334. DOI: 10.1016/j.colsurfb.2022.112334.
- Tang, X.-M.; Liu, P.-D.; Chen, Z.-J.; Li, X.-Y.; Huang, R.; Liu, G.-D.; Dong, R.-S.; Chen, J. Encapsulation of a Desmodium Intortum Protein Isolate Pickering Emulsion of β-Carotene: Stability, Bioaccesibility and Cytotoxicity. Foods. 2022, 11(7), 936. DOI: 10.3390/foods11070936.
- Zhou, C.; Zhang, L.; Zaky, A. A.; Tie, S.; Cui, G.; Liu, R.; Abd El-Aty, A. M.; Tan, M. High Internal Phase Pickering Emulsion by Spanish Mackerel Proteins-Procyanidins: Application for Stabilizing Astaxanthin and Surimi. Food Hydrocolloids. 2022, 133, 107999. DOI: 10.1016/j.foodhyd.2022.107999.
- Yang, Z.; Yan, J.; Duan, Y.; Dai, L.; Wang, Y.; Sun, Q.; McClements, D. J.; Xu, X. Hydrolyzed Rice Glutelin Nanoparticles as Particulate Emulsifier for Pickering Emulsion: Structure, Interfacial Properties, and Application for Encapsulating Curcumin. Food Hydrocolloids. 2023, 134, 108105. DOI: 10.1016/j.foodhyd.2022.108105.
- Zhang, B.; Wang, Y.; Lu, R. Pickering Emulsion Stabilized by Casein–Caffeic Acid Covalent Nanoparticles to Enhance the Bioavailability of Curcumin in vitro and in vivo. J. Sci. Food Agric. 2023, 103(7), 3579–3591. DOI: https://doi.org/10.1002/jsfa.12447.
- Xu, Y.; Wei, Z.; Xue, C. Pickering Emulsions Stabilized by Zein–Gallic Acid Composite Nanoparticles: Impact of Covalent or Non-Covalent Interactions on Storage Stability, Lipid Oxidation and Digestibility. Food Chem. 2023, 408, 135254. DOI: 10.1016/j.foodchem.2022.135254.
- Ning, F.; Wang, X.; Zheng, H.; Zhang, K.; Bai, C.; Peng, H.; Huang, Q.; Xiong, H. Improving the Bioaccessibility and in vitro Absorption of 5-Demethylnobiletin from Chenpi by Se-Enriched Peanut Protein Nanoparticles-Stabilized Pickering Emulsion. J. Funct. Foods. 2019, 55, 76–85. DOI: 10.1016/j.jff.2019.02.019.
- Ge, S.; Jia, R.; Li, Q.; Liu, W.; Liu, M.; Cai, D.; Zheng, M.; Liu, H.; Liu, J. Pickering Emulsion Stabilized by Zein/Adzuki Bean Seed Coat Polyphenol Nanoparticles to Enhance the Stability and Bioaccessibility of Astaxanthin. J. Funct. Foods. 2022, 88, 104867. DOI: 10.1016/j.jff.2021.104867.
- Xia, T.; Gao, Y.; Liu, Y.; Wei, Z.; Xue, C. Lactoferrin Particles Assembled via Transglutaminase-Induced Crosslinking: Utilization in Oleogel-Based Pickering Emulsions with Improved Curcumin Bioaccessibility. Food Chem. 2022, 374, 131779. DOI: 10.1016/j.foodchem.2021.131779.
- Yi, J.; Gao, L.; Zhong, G.; Fan, Y. Fabrication of High Internal Phase Pickering Emulsions with Calcium-Crosslinked Whey Protein Nanoparticles for β-Carotene Stabilization and Delivery. Food Funct. 2020, 11(1), 768–778. DOI: 10.1039/C9FO02434D.
- Wei, Z.; Cheng, Y.; Zhu, J.; Huang, Q. Genipin-Crosslinked Ovotransferrin Particle-Stabilized Pickering Emulsions as Delivery Vehicles for Hesperidin. Food Hydrocolloids. 2019, 94, 561–573. DOI: 10.1016/j.foodhyd.2019.04.008.
- Geng, M.; Li, L.; Feng, X.; Xu, J.; Huang, Y.; Teng, F.; Li, Y. Encapsulation of β-Carotene in High Internal Phase Pickering Emulsions Stabilized by Soy Protein Isolate – Epigallocatechin-3-Gallate Covalent Composite Microgel Particles. J. Mol. Liq. 2022, 360, 119511. DOI: 10.1016/j.molliq.2022.119511.
- Zou, Y.; Zhong, J.; Pan, R.; Wan, Z.; Guo, J.; Wang, J.; Yin, S.; Yang, X. Zein/Tannic Acid Complex Nanoparticles-Stabilised Emulsion as a Novel Delivery System for Controlled Release of Curcumin. International Journal Of Food Science & Technology. 2017, 52(5), 1221–1228. DOI: 10.1111/ijfs.13380.
- Chen, X.; Chen, Y.; Huang, Y.; Zou, L.; Liu, C.; McClements, D. J.; Liu, W. Hybrid Bionanoparticle-Stabilized Pickering Emulsions for Quercetin Delivery: Effect of Interfacial Composition on Release, Lipolysis, and Bioaccessibility. Acs Appl. Nano Mater. 2019, 2(10), 6462–6472. DOI: 10.1021/acsanm.9b01413.
- Wei, Y.; Tong, Z.; Dai, L.; Wang, D.; Lv, P.; Liu, J.; Mao, L.; Yuan, F.; Gao, Y. Influence of Interfacial Compositions on the Microstructure, Physiochemical Stability, Lipid Digestion and β-Carotene Bioaccessibility of Pickering Emulsions. Food Hydrocolloids. 2020, 104, 105738. DOI: 10.1016/j.foodhyd.2020.105738.
- Wei, Z.; Cheng, Y.; Huang, Q. Heteroprotein Complex Formation of Ovotransferrin and Lysozyme: Fabrication of Food-Grade Particles to Stabilize Pickering Emulsions. Food Hydrocolloids. 2019, 96, 190–200. DOI: 10.1016/j.foodhyd.2019.05.024.
- Torlopov, M. A.; Vaseneva, I. N.; Mikhaylov, V. I.; Martakov, I. S.; Legki, P. V.; Paderin, N. M.; Sitnikov, P. A. Surface, Rheopexy, Digestive Stability and Toxicity of Olive Oil Emulsions Stabilized by Chitin Nanocrystals for Vitamin D3 Delivery. Carbohydr. Polym. 2022, 284, 119162. DOI: 10.1016/j.carbpol.2022.119162.
- Zhou, H.; Tan, Y.; Lv, S.; Liu, J.; Muriel Mundo, J. L.; Bai, L.; Rojas, O. J.; McClements, D. J. Nanochitin-Stabilized Pickering Emulsions: Influence of Nanochitin on Lipid Digestibility and Vitamin Bioaccessibility. Food Hydrocolloids. 2020, 106, 105878. DOI: 10.1016/j.foodhyd.2020.105878.
- Lu, X.; Zhu, J.; Pan, Y.; Huang, Q. Assessment of Dynamic Bioaccessibility of Curcumin Encapsulated in Milled Starch Particle Stabilized Pickering Emulsions Using Tno’s Gastrointestinal Model. Food Funct. 2019, 10(5), 2583–2594. DOI: 10.1039/C8FO02495B.
- Gong, H.; Lin, S.; Ren, H.; Song, X.; Zhao, Q. Pickering Emulsion Stabilised by Double-Modified Starch Particles and Its Delivery Property for Curcumin. Int. J. Food Sci. Tech. 2022, 57(12), 7751–7762. DOI: https://doi.org/10.1111/ijfs.16135.
- Lu, X.; Huang, Q. Stability and in vitro digestion study of curcumin-encapsulated in different milled cellulose particle stabilized Pickering emulsions. Food Funct. 2020, 11(1), 606–616. DOI: https://doi.org/10.1039/C9FO02029B.
- Lu, X.; Zhang, H.; Zheng, T.; Liu, Q.; Zhu, J.; Huang, Q. Evaluation of Oral Bioaccessibility of Aged Citrus Peel Extracts Encapsulated in Different Lipid-Based Systems: A Comparison Study Using Different in vitro Digestion Models. J. Agric. Food Chem. 2020, 68(1), 97–105. DOI: 10.1021/acs.jafc.9b05372.
- Qi, W.; Zhang, Z.; Wu, T. Encapsulation of β-Carotene in Oleogel-In-Water Pickering Emulsion with Improved Stability and Bioaccessibility. Int. J. Biol. Macromol. 2020, 164, 1432–1442. DOI: 10.1016/j.ijbiomac.2020.07.227.
- Winuprasith, T.; Khomein, P.; Mitbumrung, W.; Suphantharika, M.; Nitithamyong, A.; McClements, D. J. Encapsulation of Vitamin D3 in Pickering Emulsions Stabilized by Nanofibrillated Mangosteen Cellulose: Impact on in vitro Digestion and Bioaccessibility. Food Hydrocolloids. 2018, 83, 153–164. DOI: 10.1016/j.foodhyd.2018.04.047.
- Zheng, R.; Zhao, T.; Lin, X.; Chen, Z.; Li, B.; Zhang, Y. Fabrication, Characterization, and Application of Pickering Emulsion Stabilized by Tea (Camellia Sinensis (L.) O. Kuntze) Waste Microcrystalline Cellulose. J. Dispersion Sci. Technol. 2023, 44(11), 1–11. DOI: 10.1080/01932691.2022.2063883.
- Tang, L.; Huang, H. Evaluation of Pineapple Peel Cellulose Nanocrystals/EGCG Complexes for Improving the Stability of Curcumin Emulsion. Cellulose. 2022, 29(11), 6123–6141. DOI: 10.1007/s10570-022-04666-8.
- Patel, A. S.; Balasubramaniam, S. L.; Nayak, B.; Camire, M. E. Lauric Acid Adsorbed Cellulose Nanocrystals Reduced the in vitro Gastrointestinal Digestion of Oil-Water Pickering Emulsions. Food Hydrocolloids. 2023, 134, 108120. DOI: 10.1016/j.foodhyd.2022.108120.
- Zhang, L.; Chen, D.-L.; Wang, X.-F.; Xu, L.; Qian, J.-Y.; He, X.-D. Enzymatically Modified Quinoa Starch Based Pickering Emulsion as Carrier for Curcumin: Rheological Properties, Protection Effect and in vitro Digestion Study. Food Biosci. 2022, 49, 101933. DOI: 10.1016/j.fbio.2022.101933.
- Jo, M.; Ban, C.; Goh, K. K.; Choi, Y. J. Enhancement of the Gut-Retention Time of Resveratrol Using Waxy Maize Starch Nanocrystal-Stabilized and Chitosan-Coated Pickering Emulsions. Food Hydrocolloids. 2021, 112, 106291. DOI: 10.1016/j.foodhyd.2020.106291.
- Shi, Y.; Ye, F.; Zhu, Y.; Miao, M. Development of Dendrimer-Like Glucan-Stabilized Pickering Emulsions Incorporated with β-Carotene. Food Chem. 2022, 385, 132626. DOI: 10.1016/j.foodchem.2022.132626.
- Ribeiro, E. F.; Borreani, J.; Moraga, G.; Nicoletti, V. R.; Quiles, A.; Hernando, I. Digestibility and Bioaccessibility of Pickering Emulsions of Roasted Coffee Oil Stabilized by Chitosan and Chitosan-Sodium Tripolyphosphate Nanoparticles. Food Biophys. 2020, 15(2), 196–205. DOI: 10.1007/s11483-019-09614-x.
- Shah, B. R.; Zhang, C.; Li, Y.; Li, B. Bioaccessibility and Antioxidant Activity of Curcumin After Encapsulated by Nano and Pickering Emulsion Based on Chitosan-Tripolyphosphate Nanoparticles. Food Res. Int. 2016, 89, 399–407. DOI: 10.1016/j.foodres.2016.08.022.
- Han, J.; Chen, F.; Gao, C.; Zhang, Y.; Tang, X. Environmental stability and curcumin release properties of Pickering emulsion stabilized by chitosan/gum Arabic nanoparticles. Int. J. Biol. Macromol. 2020, 157, 202–211. DOI: 10.1016/j.ijbiomac.2020.04.177.
- Li, X.-M.; Li, X.; Wu, Z.; Wang, Y.; Cheng, J.-S.; Wang, T.; Zhang, B. Chitosan Hydrochloride/Carboxymethyl Starch Complex Nanogels Stabilized Pickering Emulsions for Oral Delivery of β-Carotene: Protection Effect and in vitro Digestion Study. Food Chem. 2020, 315, 126288. DOI: 10.1016/j.foodchem.2020.126288.
- Shah, B. R.; Xu, W.; Mráz, J. Formulation and Characterization of Zein/Chitosan Complex Particles Stabilized Pickering Emulsion with the Encapsulation and Delivery of Vitamin D3. J. Sci. Food Agric. 2021, 101(13), 5419–5428. DOI: 10.1002/jsfa.11190.
- Yu, Y.; Liu, Q.; Wang, C.; Zhang, D.; Jiang, B.; Shan, Y.; Fu, F.; Ding, S. Zein/Pullulan Complex Colloidal Particle-Stabilized Pickering Emulsions for Oral Delivery of Polymethoxylated Flavones: Protection Effect and in vitro Digestion. J. Sci. Food Agric. 2022, 102(10), 3952–3963. DOI: 10.1002/jsfa.11742.
- Lei, L.; Chen, Y.-L.; Zhu, C.-H.; Wu, H.-F.; Wan, Z.-L.; Yang, X.-Q.; Yuan, Y. The Novel Pickering Emulsion Gels Stabilized by Zein Hydrolysate-Chitin Nanocrystals Coacervates: Improvement on Stability and Bioaccessibility for Curcumin. Food Res. Int. 2022, 161, 111877. DOI: 10.1016/j.foodres.2022.111877.
- Yan, J.; Liang, X.; Ma, C.; McClements, D. J.; Liu, X.; Liu, F. Design and Characterization of Double-Cross-Linked Emulsion Gels Using Mixed Biopolymers: Zein and Sodium Alginate. Food Hydrocolloids. 2021, 113, 106473. DOI: 10.1016/j.foodhyd.2020.106473.
- Ma, J.-J.; Huang, X.-N.; Yin, S.-W.; Yu, Y.-G.; Yang, X.-Q. Bioavailability of Quercetin in Zein-Based Colloidal Particles-Stabilized Pickering Emulsions Investigated by the in vitro Digestion Coupled with Caco-2 Cell Monolayer Model. Food Chem. 2021, 360, 130152. DOI: 10.1016/j.foodchem.2021.130152.
- Wei, Y.; Liu, Z.; Guo, A.; Mackie, A.; Zhang, L.; Liao, W.; Mao, L.; Yuan, F.; Gao, Y. Zein Colloidal Particles and Cellulose Nanocrystals Synergistic Stabilization of Pickering Emulsions for Delivery of β-Carotene. J. Agric. Food Chem. 2021, 69(41), 12278–12294. DOI: 10.1021/acs.jafc.0c07800.
- Wei, Y.; Zhang, L.; Liao, W.; Mao, L.; Zhang, M.; Guo, X.; Huang, C.; Han, H.; Mackie, A.; Gao, Y. Enhanced Stability and Controlled Gastrointestinal Digestion of β-Carotene Loaded Pickering Emulsions with Particle–Particle Complex Interfaces. Food Funct. 2021, 12(21), 10842–10861. DOI: 10.1039/D1FO01714D.
- Meng, R.; Wu, Z.; Xie, Q.-T.; Zhang, B.; Li, X.-L.; Liu, W.-J.; Tao, H.; Li, P.-J. Zein/Carboxymethyl Dextrin Nanoparticles Stabilized Pickering Emulsions as Delivery Vehicles: Effect of Interfacial Composition on Lipid Oxidation and in vitro Digestion. Food Hydrocolloids. 2020, 108, 106020. DOI: 10.1016/j.foodhyd.2020.106020.
- Ge, R.; Zhu, H.; Zhong, J.; Wang, H.; Tao, N. Storage Stability and in vitro Digestion of Apigenin Encapsulated in Pickering Emulsions Stabilized by Whey Protein Isolate–Chitosan Complexes. Front. Nutrit. 2022, 9. DOI: 10.3389/fnut.2022.997706.
- Araiza-Calahorra, A.; Wang, Y.; Boesch, C.; Zhao, Y.; Sarkar, A. Pickering Emulsions Stabilized by Colloidal Gel Particles Complexed or Conjugated with Biopolymers to Enhance Bioaccessibility and Cellular Uptake of Curcumin. Curr Res Food Sci. 2020, 3, 178–188. DOI: 10.1016/j.crfs.2020.05.001.
- Liu, G.; Li, W.; Qin, X.; Zhong, Q. Pickering Emulsions Stabilized by Amphiphilic Anisotropic Nanofibrils of Glycated Whey Proteins. Food Hydrocolloids. 2020, 101, 105503. DOI: 10.1016/j.foodhyd.2019.105503.
- Lv, P.; Wang, D.; Chen, Y.; Zhu, S.; Zhang, J.; Mao, L.; Gao, Y.; Yuan, F. Pickering Emulsion Gels Stabilized by Novel Complex Particles of High-Pressure-Induced WPI Gel and Chitosan: Fabrication, Characterization and Encapsulation. Food Hydrocolloids. 2020, 108, 105992. DOI: 10.1016/j.foodhyd.2020.105992.
- Fu, D.; Deng, S.; McClements, D. J.; Zhou, L.; Zou, L.; Yi, J.; Liu, C.; Liu, W. Encapsulation of β-Carotene in Wheat Gluten Nanoparticle-Xanthan Gum-Stabilized Pickering Emulsions: Enhancement of Carotenoid Stability and Bioaccessibility. Food Hydrocolloids. 2019, 89, 80–89. DOI: 10.1016/j.foodhyd.2018.10.032.
- Liu, J.; Guo, J.; Zhang, H.; Liao, Y.; Liu, S.; Cheng, D.; Zhang, T.; Xiao, H.; Du, Z. The Fabrication, Characterization, and Application of Chitosan–NaOh Modified Casein Nanoparticles and Their Stabilized Long-Term Stable High Internal Phase Pickering Emulsions. Food Funct. 2022, 13(3), 1408–1420. DOI: 10.1039/D1FO02202D.
- Huang, X.-N.; Zhou, F.-Z.; Yang, T.; Yin, S.-W.; Tang, C.-H.; Yang, X.-Q. Fabrication and Characterization of Pickering High Internal Phase Emulsions (HIPEs) Stabilized by Chitosan-Caseinophosphopeptides Nanocomplexes as Oral Delivery Vehicles. Food Hydrocolloids. 2019, 93, 34–45. DOI: 10.1016/j.foodhyd.2019.02.005.
- Yi, J.; Gan, C.; Wen, Z.; Fan, Y.; Wu, X. Development of Pea Protein and High Methoxyl Pectin Colloidal Particles Stabilized High Internal Phase Pickering Emulsions for β-Carotene Protection and Delivery. Food Hydrocolloids. 2021, 113, 106497. DOI: 10.1016/j.foodhyd.2020.106497.
- Wu, C.; Liu, Z.; Zhi, L.; Jiao, B.; Tian, Y.; Liu, H.; Hu, H.; Ma, X.; Pignitter, M.; Wang, Q. Research Progress of Food-Grade High Internal Phase Pickering Emulsions and Their Application in 3D Printing. Nanomaterials. 2022, 12(17), 2949. DOI: 10.3390/nano12172949.
- Shen, R.; Lin, D.; Liu, Z.; Zhai, H.; Yang, X. Fabrication of Bacterial Cellulose Nanofibers/Soy Protein Isolate Colloidal Particles for the Stabilization of High Internal Phase Pickering Emulsions by Anti-Solvent Precipitation and Their Application in the Delivery of Curcumin. Front Nutr. 2021, 8, 734620. DOI: 10.3389/fnut.2021.734620.
- Wang, L.; Zhang, H.; Li, H.; Zhang, H.; Chi, Y.; Xia, N.; Li, Z.; Jiang, L.; Zhang, X.; Rayan, A. M. Fabrication and Digestive Characteristics of High Internal Phase Pickering Emulsions Stabilized by Ovalbumin-Pectin Complexes for Improving the Stability and Bioaccessibility of Curcumin. Food Chem. 2022, 389, 133055. DOI: 10.1016/j.foodchem.2022.133055.
- Dong, Y.; Wei, Z.; Wang, Y.; Tang, Q.; Xue, C.; Huang, Q. Oleogel-Based Pickering Emulsions Stabilized by Ovotransferrin–Carboxymethyl Chitosan Nanoparticles for Delivery of Curcumin. LWT. 2022, 157, 113121. DOI: 10.1016/j.lwt.2022.113121.
- Fan, Y.; Luo, D.; Yi, J. Resveratrol-Loaded α-Lactalbumin-Chitosan Nanoparticle-Encapsulated High Internal Phase Pickering Emulsion for Curcumin Protection and Its in vitro Digestion Profile. Food Chem.: X. 2022, 15, 100433. DOI: 10.1016/j.fochx.2022.100433.
- Liu, Z.; Hu, M.; Zhang, S.; Jiang, L.; Xie, F.; Li, Y. Oil-In-Water Pickering Emulsion Stabilization with Oppositely Charged Polysaccharide Particles: Chitin Nanocrystals/Fucoidan Complexes. J. Sci. Food Agric. 2021, 101(7), 3003–3012. DOI: 10.1002/jsfa.10934.
- Xu, W.; Sun, H.; Jia, Y.; Jia, Y.; Ning, Y.; Wang, Y.; Jiang, L.; Luo, D.; Shah, B. R. Pickering Emulsions Synergistic Stabilized with Konjac Glucomannan and Xanthan Gum/Lysozyme Nanoparticles: Structure, Protection and Gastrointestinal Digestion. Carbohydr. Polym. 2023, 305, 120507. DOI: 10.1016/j.carbpol.2022.120507.
- Tang, X.-Y.; Wang, Z.-M.; Meng, H.-C.; Lin, J.-W.; Guo, X.-M.; Zhang, T.; Chen, H.-L.; Lei, C.-Y.; Yu, S.-J. Robust W/O/W Emulsion Stabilized by Genipin-Cross-Linked Sugar Beet Pectin-Bovine Serum Albumin Nanoparticles: Co-Encapsulation of Betanin and Curcumin. J. Agric. Food Chem. 2021, 69(4), 1318–1328. DOI: 10.1021/acs.jafc.0c05212.
- Nikbakht Nasrabadi, M.; Sedaghat Doost, A.; Goli, S. A. H.; Van der Meeren, P. Effect of Thymol and Pickering Stabilization on in-Vitro Digestion Fate and Oxidation Stability of Plant-Derived Flaxseed Oil Emulsions. Food Chem. 2020, 311, 125872. DOI: 10.1016/j.foodchem.2019.125872.
- Song, J.; Li, H.; Shang, W.; Wang, H.; Tan, M. Fabrication and Characterization of Pickering Emulsion Gels Stabilized by Gliadin/Starch Complex for the Delivery of Astaxanthin. Food Hydrocolloids. 2023, 137, 108388. DOI: 10.1016/j.foodhyd.2022.108388.
- Ma, L.; Zou, L.; McClements, D. J.; Liu, W. One-Step Preparation of High Internal Phase Emulsions Using Natural Edible Pickering Stabilizers: Gliadin Nanoparticles/Gum Arabic. Food Hydrocolloids. 2020, 100, 105381. DOI: 10.1016/j.foodhyd.2019.105381.
- Wei, Z.; Zhang, H.; Huang, Q. Curcumin-loaded Pickering emulsion stabilized by insoluble complexes involving ovotransferrin–gallic acid conjugates and carboxymethyldextran. Food Funct. 2019, 10(8), 4911–4923. DOI: https://doi.org/10.1039/c9fo01162e.
- Shi, A.; Feng, X.; Wang, Q.; Adhikari, B. Pickering and High Internal Phase Pickering Emulsions Stabilized by Protein-Based Particles: A Review of Synthesis, Application and Prospective. Food Hydrocolloids. 2020, 109, 106117. DOI: 10.1016/j.foodhyd.2020.106117.
- Wei, Z.; Cheng, J.; Huang, Q. Food-Grade Pickering Emulsions Stabilized by Ovotransferrin Fibrils. Food Hydrocolloids. 2019, 94, 592–602. DOI: 10.1016/j.foodhyd.2019.04.005.
- Liu, X.; Huang, Y.-Q.; Chen, X.-W.; Deng, Z.-Y.; Yang, X.-Q. Whole Cereal Protein-Based Pickering Emulsions Prepared by Zein-Gliadin Complex Particles. J. Cereal Sci. 2019, 87, 46–51. DOI: 10.1016/j.jcs.2019.02.004.
- Liu, W.; Gao, H.; McClements, D. J.; Zhou, L.; Wu, J.; Zou, L. Stability, Rheology, and β-Carotene Bioaccessibility of High Internal Phase Emulsion Gels. Food Hydrocolloids. 2019, 88, 210–217. DOI: 10.1016/j.foodhyd.2018.10.012.
- Xu, B.; Liu, C.; Sun, H.; Wang, X.; Huang, F. Highly Surface-Active Chaperonin Nanobarrels for Oil-In-Water Pickering Emulsions and Delivery of Lipophilic Compounds. J. Agric. Food Chem. 2019, 67(36), 10155–10164. DOI: 10.1021/acs.jafc.9b02379.
- Calabrese, V.; Courtenay, J. C.; Edler, K. J.; Scott, J. L. Pickering Emulsions Stabilized by Naturally Derived or Biodegradable Particles. Curr. Opin. Green Sustain. Chem. 2018, 12, 83–90. DOI: 10.1016/j.cogsc.2018.07.002.
- Liu, F.; Tang, C.-H. Soy Glycinin as Food-Grade Pickering Stabilizers: Part. II. Improvement of Emulsification and Interfacial Adsorption by Electrostatic Screening. Food Hydrocolloids. 2016, 60, 620–630. DOI: 10.1016/j.foodhyd.2015.10.024.
- Zheng, W.; Zhang, H.; Wang, J.; Wang, J.; Yan, L.; Liu, C.; Zheng, L. Pickering Emulsion Hydrogel Based on Alginate-Gellan Gum with Carboxymethyl Chitosan as a pH-Responsive Controlled Release Delivery System. Int. J. Biol. Macromol. 2022, 216, 850–859. DOI: 10.1016/j.ijbiomac.2022.07.223.
- Cao, Y.; Yang, L.; Qiao, X.; Xue, C.; Xu, J. Dietary Astaxanthin: An Excellent Carotenoid with Multiple Health Benefits. Crit. Rev. Food Sci. Nutr. 2023, 63(18), 1–27. DOI: 10.1080/10408398.2021.1983766.
- Fakhri, S.; Abbaszadeh, F.; Dargahi, L.; Jorjani, M. Astaxanthin: A Mechanistic Review on Its Biological Activities and Health Benefits. Pharmacol. Res. 2018, 136, 1–20. DOI: 10.1016/j.phrs.2018.08.012.
- Martínez-Delgado, A. A.; Khandual, S.; Villanueva–Rodríguez, S. J. Chemical Stability of Astaxanthin Integrated into a Food Matrix: Effects of Food Processing and Methods for Preservation. Food Chem. 2017, 225, 23–30. DOI: 10.1016/j.foodchem.2016.11.092.
- Yang, L.; Li, F.; Cao, X.; Qiao, X.; Xue, C.; Xu, J. Stability and Bioavailability of Protein Matrix-Encapsulated Astaxanthin Ester Microcapsules. J. Sci. Food Agric. 2022, 102(5), 2144–2152. DOI: 10.1002/jsfa.11556.
- Toro-Vazquez, J. F.; Charó-Alonso, M. A.; Pérez-Martínez, J. D.; Morales-Rueda, J. A. Candelilla Wax as an Organogelator for Vegetable Oils—An Alternative to Develop Trans-Free Products for the Food Industry. In Edible Oleogels; Marangoni, A.G. and Garti, N., Eds.; AOCS Press, 2011; pp. 119–148.
- Manzoor, S.; Masoodi, F. A.; Naqash, F.; Rashid, R. Oleogels: Promising Alternatives to Solid Fats for Food Applications. Food Hydrocolloids Health. 2022, 2, 100058. DOI: 10.1016/j.fhfh.2022.100058.
- Lu, X.; Li, C.; Huang, Q. Combining in vitro Digestion Model with Cell Culture Model: Assessment of Encapsulation and Delivery of Curcumin in Milled Starch Particle Stabilized Pickering Emulsions. Int. J. Biol. Macromol. 2019, 139, 917–924. DOI: 10.1016/j.ijbiomac.2019.08.078.
- Li, X.; de Vries, R. Interfacial Stabilization Using Complexes of Plant Proteins and Polysaccharides. Curr. Opin. Food Sci. 2018, 21, 51–56. DOI: 10.1016/j.cofs.2018.05.012.
- Ribeiro, E.; Morell, P.; Nicoletti, V.; Quiles, A.; Hernando, I. Protein-And Polysaccharide-Based Particles Used for Pickering Emulsion Stabilisation. Food Hydrocolloids. 2021, 119, 106839. DOI: 10.1016/j.foodhyd.2021.106839.
- Wang, M.; Li, Y.; Ma, C.; Zhang, Z.; Guo, L.; Huang, M.; Sun, J. Stability of Native/Thermally Denatured Myofibrillar Protein Particles: Improvement with Decreasing pH. Food Hydrocolloids. 2023, 140, 108628. DOI: 10.1016/j.foodhyd.2023.108628.
- Chen, J.; Zheng, J.; McClements, D. J.; Xiao, H. Tangeretin-Loaded Protein Nanoparticles Fabricated from zein/β-Lactoglobulin: Preparation, Characterization, and Functional Performance. Food Chem. 2014, 158, 466–472. DOI: 10.1016/j.foodchem.2014.03.003.
- Dupont, H.; Maingret, V.; Schmitt, V.; Héroguez, V. New Insights into the Formulation and Polymerization of Pickering Emulsions Stabilized by Natural Organic Particles. Macromolecules. 2021, 54(11), 4945–4970. DOI: 10.1021/acs.macromol.1c00225.
- Tan, Y.; Zhou, H.; McClements, D. J. Application of Static in vitro Digestion Models for Assessing the Bioaccessibility of Hydrophobic Bioactives: A Review. Trends Food Sci. Technol. 2022, 122, 314–327. DOI: 10.1016/j.tifs.2022.02.028.
- Fernández-García, E.; Carvajal-Lérida, I.; Pérez-Gálvez, A. In vitro Bioaccessibility Assessment as a Prediction Tool of Nutritional Efficiency. Nutr. Res. 2009, 29(11), 751–760. DOI: 10.1016/j.nutres.2009.09.016.
- Berthelsen, R.; Klitgaard, M.; Rades, T.; Müllertz, A. In vitro Digestion Models to Evaluate Lipid Based Drug Delivery Systems; Present Status and Current Trends. Advanced Drug Delivery Reviews. 2019, 142, 35–49. DOI: 10.1016/j.addr.2019.06.010.
- McClements, D. J.; Li, Y. Review of in vitro Digestion Models for Rapid Screening of Emulsion-Based Systems. Food Funct. 2010, 1(1), 32–59. DOI: 10.1039/c0fo00111b.
- Brodkorb, A.; Egger, L.; Alminger, M.; Alvito, P.; Assunção, R.; Ballance, S.; Bohn, T.; Bourlieu-Lacanal, C.; Boutrou, R.; Carrière, F., et al. INFOGEST Static in vitro Simulation of Gastrointestinal Food Digestion. Nat. Protoc. 2019, 14(4), 991–1014.
- Sarkar, A.; Mackie, A. R. Engineering Oral Delivery of Hydrophobic Bioactives in Real-World Scenarios. Curr. Opin. Colloid Interface Sci. 2020, 48, 40–52. DOI: 10.1016/j.cocis.2020.03.009.
- McClements, D. J.; Li, Y. Structured Emulsion-Based Delivery Systems: Controlling the Digestion and Release of Lipophilic Food Components. Adv. Coll. Interf. Sci. 2010, 159(2), 213–228. DOI: 10.1016/j.cis.2010.06.010.
- Minekus, M. The TNO Gastro-Intestinal Model (TIM). In The Impact of Food Bioactives on Health: In vitro and ex vivo Models. In The Author(s); Verhoeckx, K., Cotter, P., López-Expósito, I., Kleiveland, C., Lea, T., Mackie, A., Requena, T., Swiatecka, D. and Wichers, H., Eds.; United Kingdom: Springer Copyright, 2015; pp. 37–46.