Abstract
β2-microglobulin (β2M) is highly accumulated by the kidneys of normal rats. The aim of this study was to verify if uninephrectomy can modify the renal uptake of labeled β2M. For this purpose the radioactivity of plasma and those of the remaining kidney, liver and urine have been measured in uninephrectomized rats (NX) and in controls (C) at different times after the injection as i.v. bolus of 131I-β2M. The experiments were performed in 114 Sprague-Dawley male rats. Fifty seven animals underwent right nephrectomy, the other animals being the C. NX and their C were divided in 3 groups, studied 2, 4 and 6 weeks after nephrectomy, respectively. Part of the animals were sacrificed 12 min after the injection of labeled β2M (peak-time, i.e. time of highest kidney accumulation of 131I-β2M in the normal rat) and part 10 min later.
The results demonstrate that:
uninephrectomy increases plasma retention of 131I-β2M
kidney uptake (total and per gram) is always higher in NX
liver uptake (much lower than that of kidney) is not influenced by uninephrectomy
urine excretion of radioactivity is minimal in both NX and C.
INTRODUCTION
Beta2-microglobulin (β2M) is an anionic low mw protein (mw 11,800 pI 5.8) of great interest in nephrology for its amyloidogenic property in long term dialysis patients Citation[1-5]. In the past years the important role of the kidney in the extraction and metabolism of β2M has been elucidated in rat, in dog and in humans Citation[6-9]. Like other low mw proteins, β2M is filltered by the glomeruli (glomerular sieving coefficient 0.94) and almost completely reabsorbed by the proximal tubular cells, where it is metabolized Citation[[10]]. Most probably, β2M is removed from the blood only by the kidney and its plasma concentration increases with the decreasing of renal function Citation[11-13]. Previously we studied in normal rats, body distribution, renal kinetics and kidney uptake of radioiodinated β2M Citation[[7]].
The aim of the present study is to establish if the reduction of renal mass can modify the renal handling of β2M. For this purpose the renal kinetics of 131I-β2M was investigated in uninephrectomized rats (NX) and in controls (C) determining plasma level, kidney content, liver content and urine excretion of 131I-β2M at different intervals after surgery.
In this study human β2M was employed. It has been assumed that rat kidney cannot distinguish human from rat β2M in an acute experimental situation.
METHODS
β2-Microglobulin
β2M (mw 11, 800, pI 5.8) was obtained from the urine of patients with tubular proteinuria and purified by gel filtration and ion-exchange chromatography (Dakopatts, Denmark). It was iodinated with 131I by the chloramine t method Citation[[14]]. The iodination efficiency was approximately 80% and the specific activity was about 20 mCi/mg protein. Radioiodinated β2M was employed within 5 days from the labeling. Prior to each experiment, free iodine was removed by ion-exchange chromatography. After this purification, free iodine was less than 1%.
Animals
Sprague-Dawley male rats were employed (n 114, body weight 220–490 g, mean 327 ± 60 SD). They were allowed free access to tap water and standard rat chow containing 15% of proteins (MIL Morini, Reggio Emilia, Italy).
Experimental Design
Experiments were performed under anesthesia induced by intraperitoneal injection of penthotal sodium (Abbott, Roma, Italy), 50 mg/Kg body weight in 5% aqueous solution. Fifty-seven rats were submitted to right nephrectomy, an equal number being the C. Nephrectomy was performed by laparotomy, ligation of the right renal pedicle and removal of the right kidney. NX were divided into three groups, studied 2, 4 and 6 weeks after nephrectomy, respectively, together with an equal number of C. 131I-β2M was injected as a bolus into a tail vein at a dosage of approximately 25 μCi corresponding to 1 μg of protein. The results of a previous study indicate that in the rat the time of highest kidney radioactivity (peak-time) after bolus injection of 131I-β2M is about 12 min as shown in Citation[[7]]. Part of the animals (NX and C) of each group were sacrificed at the kidney peak-time (12 min after the injection of 131I-β2M) and the remainder 10 min later (that is at the 22nd min after the injection), when kidney radioactivity is decreasing. Sacrifice was performed by opening the chest and rapidly drawing 7–10 mL of blood from the heart. Immediately after sacrifice, left kidney, liver and total urine were removed. Plasma, left kidney and liver were weighed using a precision balance (Cobos 704, Spain). The radioactivity of the injected dose and those of plasma, kidney, liver and urine were measured using the same scintillation counter (Italelettronica, Italy).
Figure 1. Total body scan (left) and time-course curve of kidney radioactivity (right) recorded in a normal rat after i.v. injection of 131I-β2-microglobulin.
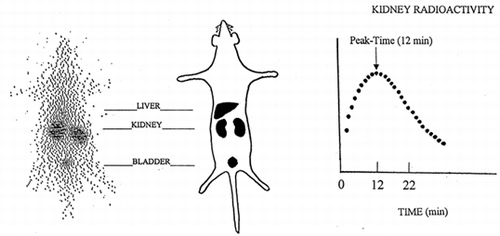
The results are expressed as percent of the injected dose. All the results are presented as mean ± SD. Data were analyzed using Student's unpaired t test. Differences are considered significant if the p value was less than 0.05.
RESULTS
Plasma (1g) — After injection of 131I-β2M plasma radioactivity is higher in NX than in C. Such an increase is particularly evident at the second and fourth week after nephrectomy (peak-time) and at the second week (10 min after peak-time) ().
Figure 2. Plasma radioactivity of 131I-β2-microglobulin (radioactivity per gram of plasma, percent of the injected dose) in uninephrectomized rats (•) and in controls (○) 2, 4 and 6 weeks after nephrectomy. On the left the values at the 12th min after i.v. injection of 131I-β2-microglobulin (peak-time); on the right those at the 22nd min. Mean values ± SD, *p < 0.05, **p < 0.001.
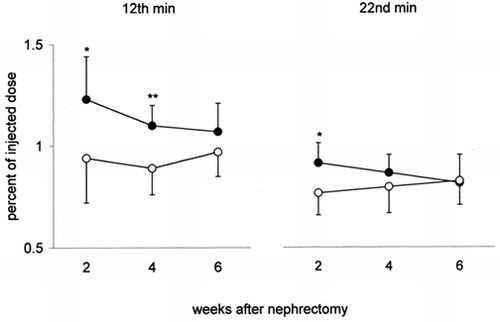
Kidney (total and 1g) — Total kidney uptake of 131I-β2M is markedly higher in NX than in C at any time after nephrectomy, either at the peak-time or 10 min later (). Kidney uptake (per g) of 131I-β2M is also higher in NX than in C at any time after nephrectomy. The difference between NX and C is less relevant than for total kidney but is still statistically significant (). This behavior indicates that in the remaining kidney of NX the increase of kidney uptake of 131I-β2M is higher than the increase of kidney weight due to compensatory hypertrophy. The percent difference between NX and C is higher 10 min after the peak, for both, total and 1 g kidney, suggesting a slower release of β2M by the remaining kidney of NX. Kidney concentration capacity of 131I-β2M is very high: the radioactivity of 1 g of kidney is approximately 17 times higher than that of 1 g of plasma (without significant differences between NX and C).
Figure 3. Kidney uptake of 131I-β2-microglobulin (radioactivity of total left kidney, percent of the injected dose) in uninephrectomized rats (•) and in controls (○) 2, 4 and 6 weeks after nephrectomy. On the left the values at the 12th min after i.v. injection of 131I-β2-microglobulin (peak-time); on the right those at the 22nd min. Mean values ± SD, **p < 0.001.
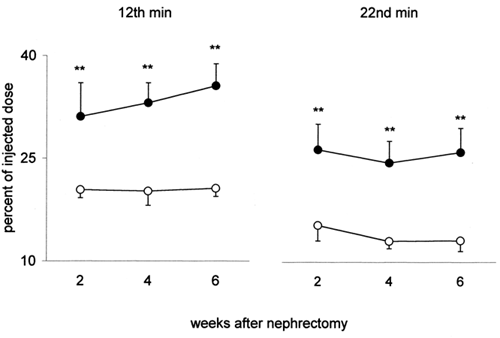
Figure 4. Kidney uptake of 131I-β2-microglobulin (radioactivity per gram of left kidney, percent of the injected dose) in uninephrectomized rats (•) and in controls (○) 2, 4 and 6 weeks after nephrectomy. On the left the values at the 12th min after i.v. injection of 131I-β2-microglobulin (peak-time); on the right those at the 22nd min. Mean values ± SD, *p < 0.05
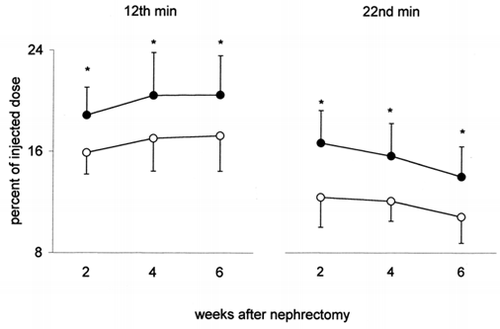
Liver (total and 1g) — Liver uptake of 131I-β2M is much lower than that of the kidney ranging from about 4 to 5.5 percent of the injected dose (total) and from 0.25 to 0.45 (1 g) without difference between NX and C.
Urine (total) — Urine excretion of radioactivity is minimal (approximately 1.20 percent of the injected dose) in both, NX and C.
DISCUSSION
Many low mw proteins of different pI (α1-microglobulin, retinol binding protein, α2U-globulin, α2a-interferon, aprotinin, cytochrome C, lysozyme and β2M) are taken-up and accumulated by the kidney of normal rats Citation[[7]], Citation[15-17].
In man, many low mw proteins seem to be handled by the kidney, as indicated by the increase of their serum levels in renal failure. In particular, this has been demonstrated for β2M, retinol binding protein, lysozyme, complement factor D, α1-microglobulin, cystatin C, tumor associated trypsin inhibitor, prolactin, myoglobin and chromogranin A Citation[[9]], Citation[[11]], Citation[[13]], Citation[18-28]. The key role of the human kidney in the handling of low mw proteins is strongly suggested by the large kidney uptake of aprotinin (a 6,500 dalton polypeptide) Citation[29-30]. Few data are available concerning the effect of the reduction of renal mass on the renal handling of low mw proteins. Our previous results demonstrate that in the rat uninephrectomy is followed by an increased uptake of radioiodinated α1-microglobulin and lysozyme by the remaining kidney Citation[31-32].
The results of the present study show that the remaining kidney of NX accumulates more 131I-β2M than C and retains it for a longer time. In contrast, liver uptake of 131I-β2M is not influenced by uninephrectomy. Probably the increased kidney accumulation (total and per g) of labeled β2M in NX is due to an increased load per nephron. This can be attributed to the higher plasma 131I-β2M (as demonstrated by our results) and to hyperfiltration, both caused by uninephrectomy.
The question arises if the increased kidney accumulation of radioiodinated β2M corresponds to an increase in kidney content of endogenous β2M. The results of a study in progress by this group in NX and in C indicate no difference in renal kinetics of 131I-lysozyme and endogenous lysozyme (unpublished data). Therefore it can be assumed that the renal content of endogenous β2M is also augmented, but whether the increased kidney content of β2M (and that of many other low mw proteins) can damage the kidney is not known. It has been postulated that in different experimental situations and in some human diseases, Bence-Jones proteins, lysozyme, interferons, myoglobin and β2M can induce adverse effects on the kidney Citation[33-43]. Immunohystologically it has been demonstrated in various renal diseases a link between β2M deposition in renal tissue (tubular epithelium and tubular casts) and tubular interstitial injuries with deterioration of renal function Citation[[44]]. There is some evidence to suggest that filtered bioactive proteins such as insulin-like growth factor 1 may play a role in promoting progressive tubulo-interstitial disease Citation[[45]].
The above reported data suggest that the reduction of renal mass (uninephrectomy) could produce tubular hypermetabolism of the many low mw proteins filtered and accumulated in excess by the proximal tubular cells. Hypermetabolism could increase renal ammoniogenesis, which can damage the kidney directly or triggering the release of multiple mediators of tissue injury and promoting fibrosis Citation[46-49]. Amino-acids derived from an increase in the breakdown of reabsorbed peptides may constitute an important nitrogen pool for ammoniogenesis in this situation Citation[[50]].
In conclusion, our findings demonstrate that in the rat the reduction of renal mass increases kidney content of β2M. The consequent renal hypermetabolism of β2M (and probably of other low mw proteins) could play a role in the progression of kidney damage.
ACKNOWLEDGMENT
This work was supported in part by a research fund from the Ministero Universitá e Ricerca Scientifica e Tecnologica, Italy. Dr. Dino Di Pasquale is gratefully acknowledged for his valuable help in the preparation of this paper. The rats employed in this work were kindly provided by Laboratori Guidotti Spa, Pisa, Italy.
REFERENCES
- Goveric P D, Casey T T, Stone W J, et al. Beta-2 Microglobulin is an amyloidogenic protein in man. J Clin Invest 1985; 76: 2425–2429
- Gejyo F, Odani S, Yamada T, et al. β2-microglobulin: a new form of amyloid protein associated with chronic hemodialysis. Kidney International 1986; 30: 385–390
- Flöge J, Granolleras C, Shaldon S, Koch K M. Dialysis-associated amyloidosis and Beta-2-microglobulin. Contrib Nephrol 1988; 61: 27–36
- Campistol J M, Bernard D, Papastoitsis G, et al. Polymerization of normal and intact β2-microglobulin as the amyloidogenic protein in dialysis-amyloidosis. Kidney International 1996; 50: 1262–1267
- Schwalbe S, Holzhauer M, Schaeffer J, et al. β2-microglobulin associated amyloidosis: a vanishing complication of long-term hemodialysis?. Kidney International 1997; 52: 1077–1083
- Bernier G M, Conrad M E. Catabolism of human β2-microglobulin by the rat kidney. Am J Physiol 1969; 217: 1359–1362
- Bianchi C, Donadio C, Tramonti G, et al. High and preferential accumulation in the kidney of anionic and cationic small proteins. Kidney, Proteins and Drugs. Contrib Nephrol, C Bianchi, V Bocci, F A Carone, R Rabkin. Karger, Basel 1990; 83: 39–46
- Hall P W, Chung-Park M, Vacca C V, et al. The renal handling of beta2-microglobulin in the dog. Kidney International 1982; 22: 156–161
- Karlsson F A, Groth T, Sege K, et al. Turnover in humans of β2-microglobulin: the constant chain of HLA-antigens. Eur J Clin Invest 1980; 10: 293–300
- Johnson V, Maak T. Renal tubular handling of proteins and peptides. Textbook of Nephrology, S G Massry, R J Glassock. 2nd edition, Williams and Wilkins, Baltimore 1989; 97–102
- Wibell L, Evrin P E, Berggård I. Serum β2-microglobulin in renal disease. Nephron 1973; 10: 320–331
- Trollfors B, Norrby R. Estimation of glomerular filtration rate by serum creatinine and serum β2-microglobulin. Nephron 1981; 28: 196–199
- Acchiardo S, Kraus A P, Jennings B R. β2-microglobulin levels in patients with renal insuffciency. Amer J Kid Dis 1989; 13: 70–74
- Greenwood F C, Hunter W M, Glover J S. The preparation of 131I-labelled human growth hormone of high specific radioactivity. Biochem J 1963; 89: 114–123
- Bianchi C, Donadio C, Tramonti G, et al. Renal handling of cationic and anionic small proteins: experiments in intact rats. Kidney and Proteins in health and disease. Contrib Nephrol, C Bianchi, V Bocci, F A Carone, R Rabkin. Karger, Basel 1988; 68: 37–44
- Hysing J, Tolleshaug H, Curthoys N P. Reabsorption and intracellular transport of cytochrome C and lysozyme in rat kidney. Acta Physiol Scand 1990; 140: 419–427
- Maack T, Hyung Park C, Camargo M JF. Renal filtration, transport and metabolism of proteins. The kidney: physiology and pathophysiology, D W Seldin, G Giebisch. ed 2, Raven Press, New York 1992; 3005–3038
- Johansson B G, Ravnskov U. The serum level and urinary excretion of α2M, β2M and lysozyme in renal disease. Scand J Urol Nephrol 1972; 6: 249–256
- Ramirez G, O'Neill W M, Bloomer H A, Jubiz W. Abnormalities in the regulation of prolactin in patients with chronic renal failure. J Clin Endocrinol Metab 1977; 45: 658–661
- Hart P M, Feinfeld D A, Briscoe A M, et al. The effect of renal failure and hemodialysis on serum and urine myoglobin. Clinical Nephrology 1982; 18: 141–143
- Sturfelt G, Truedsson L, Thysell H, Björck L. Serum level of complement factor D in systemic lupus erythematosus–an indicator of glomerular filtration rate. Acta Med Scand 1984; 216: 171–177
- Simonsen O, Grubb A, Thysell H. The blood serum concentration of cystatin C (γ-trace) as a measure of the glomerular filtration rate. Scand J Clin Lab Invest 1985; 45: 97–101
- Weber M H, Scholz P, Stibbe W, Scheler F. Alpha-1-mikroglobulin in urin und serum bei proteinurie und niereninsuffzienz. Klin Wochenschr 1985; 63: 711–717
- Kusano E, Suzuki M, Asano Y, et al. Human α1-microglobulin and its relationship to renal function. Nephron 1985; 41: 320–324
- Hsiao R J, Mezger M S, O'Connor D T. Chromogranin A in uremia: progressive retention of immunoreactive fragments. Kidney International 1990; 37: 955–964
- Tramonti G, Donadio C, Ferdeghini M, et al. Serum tumor associated trypsin inhibitor (TATI) and renal function. Scand J Clin Lab Invest 1996; 56: 653–656
- Tramonti G, Ferdeghini M, Donadio C, et al. Serum levels of tumor associated trypsin inhibitor (TATI) and glomerular filtration rate. Renal Failure 1998; 20: 295–302
- Plebani M, Dall'Amico R, Mussap M, et al. Is serum cystatin C a sensitive marker of glomerular filtration rate (GFR)? A preliminary study on renal transplant patients. Renal Failure 1998; 20: 303–309
- Bianchi C, Donadio C, Tramonti G, et al. 99mTc-aprotinin: a new tracer for kidney morphology and function. Eur J Nucl Med 1984; 9: 257–260
- Bianchi C, Donadio C, Tramonti G, et al. 99mTc-aprotinin for the study of renal morphology and tubular function. Uremia Invest 1985–1986; 9: 139–146
- Bianchi C, Donadio C, Tramonti G, et al. L'accumulation rénale de alpha-1-microglobuline radioiodée augmente chez le rat mononéphrectomisé. Néphrologie 1992; 13: 221–225
- Bianchi C, Donadio C, Tramonti G, et al. Increased kidney accumulation of 131I-lysozyme in the uninephrectomized rat. Kidney, Proteins and Drugs. Contrib Nephrol, C Bianchi, V Bocci, F A Carone, R Rabkin. Karger, Basel 1993; 101: 85–91
- Pruzanski W, Platts M E. Serum and urinary proteins, lysozyme (muramidase), and renal dysfunction in mono- and myelomonocytic leukemia. J Clin Invest 1970; 49: 1694–1708
- Cojocel C, Docin N, Baumann K. Early nephrotoxicity at high plasma concentrations of lysozyme in rat. Lab Invest 1982; 46: 149–157
- Selby P, Kohn J, Raymond J, et al. Nephrotic syndrome during treatment with interferon. Br Med J 1985; 290: 1180
- Smolens P, Barnes J L, Stein J H. Effect of chronic administration of different Bence Jones proteins on rat kidney. Kidney International 1986; 30: 874–882
- Metz-Kurschel U, Kurschel E, Niederle N, et al. Urinary enzyme excretion during treatment of malignancies with human recombinant alpha-2-interferon. Nephrol Dial Trasplant 1987; 2: 515–519
- Sanders P W, Herrera G A, Galla H J. Human Bence Jones protein toxicity in rat proximal tubule epithelium in vivo. Kidney International 1987; 32: 851–861
- Sanders P W, Herrera G A, Lott R L, Galla J H. Morphologic alterations of the proximal tubule in light chain-related renal disease. Kidney International 1988; 33: 881–889
- Sanders P W, Herrera G A, Chen A, et al. Differential nephrotoxicity of low molecular weight proteins including Bence Jones proteins in the perfused rat nephron in vivo. J Clin Invest 1988; 82: 2086–2096
- Ault B H, Stapleton F B, Gaber L, et al. Acute renal failure during therapy with recombinant human gamma interferon. N Engl J Med 1988; 319: 1397–1400
- Batuman V. Possible pathogenetic role of low-molecular-weight proteins in Balkan nephropathy. Kidney International 1991; 40(Suppl 34)S89–S92
- Zager R A. Rhabdomyolysis and myohemoglobinuric acute renal failure. Kidney International 1996; 49: 314–326
- Suzuki S, Sato H, Inomata A, et al. Immunohistological localization of beta-2-microglobulin in renal tissue as an indicator of renal dysfunction. Nephron 1992; 60: 181–186
- Hirschberg R, Adler S. Insulin-like growth factor system and the kidney: physiology, pathophysiology, and therapeutic implications. Am J Kid Dis 1998; 31: 901–919
- Nath K A, Hostetter M K, Hostetter T M. Pathophysiology of chronic tubulointerstitial disease in rats. Interaction of dietary acid load, ammonia, and complement. J Clin Invest 1985; 79: 667–675
- Schrier R W, Harris D CH, Chan L, et al. Tubular hypermetabolism as a factor in the progression of chronic renal failure. Amer J Kid Dis 1988; 12: 243–249
- Clark E C, Nath K A, Hostetter M K, Hostetter T H. Role of ammonia in progressive interstitial nephritis. Amer J Kid Dis 1991; 17(Suppl 1)15–19
- El Nahas A M. Mechanism of progression and consequences of nephron reduction. Oxford Textbook of Clinical Nephrology, S Cameron, A Davison, J P Grunfeld, D Kerr, E Ritz. Oxford University Press. 1992; 2: 1191–1227
- Rustom R, Grime S, Costigan M, et al. Oral sodium bicarbonate reduces proximal renal tubular peptide catabolism, ammoniogenesis, and tubular damage in renal patients. Renal Failure 1998; 20: 371–382