Abstract
The effects of sucrose, NaCl, and ascorbic acid on the physical state of wheat dough at sub-zero temperatures were investigated using Differential Scanning Calorimetry (DSC) and Dynamic-Mechanical Analysis (DMA). The DSC thermograms were obtained for annealed samples by scanning from −80 to 10°C at 5°C/min. Added sucrose and NaCl decreased the onset of ice melting of doughs, and they were found at −26°C. Added sucrose and NaCl increased the relative amount of unfrozen water in doughs, while added ascorbic acid had not noticeable effects. DMA measurements were made for annealed samples at a heating rate of 1°C/min from −150 to 10°C. The loss modulus, G″, of DMA showed an α-relaxation (glass transition), two low temperature relaxations (β and γ), and melting of ice in all doughs with added ingredients.
INTRODUCTION
Freezing as an excellent method for preserving food quality is well-established and widely accepted. The frozen bakery industry has multiplied their output all over the world in the last 30 years, much because of the use of frozen doughs has increased in bakeries, supermarkets and restaurants, as it allows easier and more profitable baking. Many factors, however, affect quality of frozen doughs. Probably one of the most important factors affecting frozen dough quality is the physical state of the dough during frozen storage.
Changes in physical state, such as glass transition, are observed from changes in the thermodynamic quantities. There are a number of techniques, which can be used for measuring changes in physical state of frozen food materials. These techniques include calorimetric techniques, dilatometry, NMR, and various thermal analytical techniques. Heating an amorphous glass to above its glass transition temperature results in changes in e.g. free volume, thermal expansion coefficient, specific heat, dielectric constant, viscosity, rheological properties, and proton mobility of water Citation1-2.
Differential Scanning Calorimetry (DSC) is probably the most common thermal analytical technique for measuring state and phase transitions in frozen foods and model systems Citation3-9. However, we showed very clearly in a previous study Citation[10] that DSC, as used in the study, is not sensitive enough to observe state transitions, and the glass transition of complex food systems, such as frozen wheat doughs. Therefore, more sensitive methods of analyses, e.g. Dynamic-Mechanical Analysis (DMA) are needed to observe the glass transition occurring in frozen doughs.
Dynamic-Mechanical Analysis is a technique for measuring mechanical properties of materials, including foods, as they are deformed under varying stress as a function of time, frequency, or temperature. The analysis measures three major properties of the material: 1) the storage modulus, G′, which measures the amount of energy stored in the material per cycle; 2) the loss modulus, G′′, which is proportional to the amount of energy dissipated per cycle; and 3) the loss tan δ, which corresponds to the ratio of energy lost to the energy stored per cycle. The glass transition results in a structural mechanical event related to decreasing viscosity. Therefore, DMA is a very sensitive method for detecting second-order transitions resulting in large changes in viscoelastic properties occurring over the transition temperature range Citation[11]. Moreover, according to Wetton Citation[12] and Rotter and Ishida Citation[13] DMA is about 1000 times more sensitive in observing the glass transition than DSC.
It is very important to understand phase and state transitions, including glass transition, occurring in doughs at sub-zero temperatures and the effects of ingredients on the frozen state properties and transformations. Therefore, the objective of the present study was to investigate the effects of some ingredients, used in bakery products (sucrose, NaCl, and ascorbic acid), on transformations occurring in frozen doughs at sub-zero temperatures using DSC and DMA.
MATERIALS AND METHODS
Flour
Wheat (Torfrida, harvest 1997) was obtained from AVEVE (Landen, Belgium) and milled using a Buhler MLU-202 laboratory mill (Uzwill, Switzerland), according to AACC Method 26–31. Milling yield, protein content (% of dry matter), ash content (% of dry matter) and farinograph water absorption (14% moisture basis) were 69%, 9.2%, 0.54% and 62.0%, respectively. A micro-Kjeldahl procedure, according to AACC Method 46–13 (N × 5.7), was used for protein assessment. Ash content was measured according to AACC Method 08–01. Farinograph water absorption was determined according to AACC Method 54–21, and was based on dough consistency at the 500 BU (Brabender Unit) line.
Sample Preparation
Sucrose (Sigma Chemical Co., Germany), NaCl (Merck, Germany), ascorbic acid (Merck, Germany), and their mixtures were dissolved in distilled water. Wheat flour doughs were mixed 3 min with the solutions to optimum consistency, using a 10 g bowl Micro-Mixer (National Mfg. Co., Lincoln, Nebraska). The amount of added water was the same in all doughs (10 g flour and 5.4 ml water). Sucrose, NaCl, and ascorbic acid contents were 6% (w/w), 1.5% (w/w) and 50 ppm (w/w) of flour weight, respectively. A sheet of 1.5 mm thickness for DMA was prepared from the doughs with a pasta machine (Imperia, Italy).
Differential Scanning Calorimetry (DSC)
The thermal behavior of wheat doughs with added ingredients were determined using a Mettler TA 4000 (Mettler-Toledo AG, Switzerland) thermal analysis system with a DSC-30 low temperature measuring cell, a TC15 TA processor, and STARe Thermal Analysis System Version 3.1 software. The DSC was calibrated using n-pentane (T m = −129.7°C; ΔH m = 116.7 J/g), n-hexane (T m = −94.0°C; ΔH m = 151.8 J/g), mercury (T m = −38.8°C; ΔH m = 11.4 J/g), distilled water (T m = 0.0°C; ΔH m = 334.5 J/g), gallium (T m = 29.8°C; ΔH m = 80.0 J/g), and indium (T m = 156.6°C; ΔH m = 28.5 J/g). An empty aluminum pan was used as a reference sample. Dry nitrogen gas (AGA, 99.9% N2) flow of 50 ml/min was used to minimize water condensation in the measuring cell. Triplicate samples (∼ 10 mg) were prepared in pre-weighed 40 μl aluminum DSC pans (Mettler-Toledo AG, Switzerland) and hermetically sealed. DSC thermograms were obtained for annealed samples at a heating rate of 5°C/min from −80 to 20°C. The T′g and T′m values derived from the DSC data as the onset temperatures for the glass transition and ice melting transitions, respectively. Relative unfrozen water content was determined from the relationship between latent heat of ice melting (ΔH m, J/g solute) and water content (g/g solute). Unfrozen water content, W′g (g H2O/g solute), and solid concentration in unfrozen water, C′g (weight % solute) Citation[14], were calculated using equations Equation1 and Equation2. It should be noticed that used method gives only relative unfrozen water contents, because the latent heats determined and the assumptions used in the calculations differ from absolute thermodynamic values.
Dynamic-Mechanical Analysis (DMA)
The Dynamic-Mechanical Analyzer (DMA 242, Netzsch-Gerätebau GmbH, Selb, Germany) was used with a disc-bending sample holder adapted from MacInnes Citation[15], as described by Laaksonen and Roos Citation[10]. Disc-shaped samples, diameter 30 mm and thickness 1.5 mm, were prepared with a special mold. At least triplicate samples were analyzed with DMA at a heating rate of 1°C/min from −150 to 10°C. Before analysis, the samples were annealed at −30°C for 20 min to obtain maximally freeze-concentrated samples as reported by Laaksonen and Roos Citation[10]. Annealing temperature was selected a few degrees below the onset of ice melting for all doughs with different ingredients. The annealed samples were cooled at 1°C/min to −150°C. During dynamic heating, the samples were analyzed at frequencies of 0.1, 0.5, 1, 2.5, 5, 10, and 20 Hz, and the amplitude was 7.5 μm.
RESULTS AND DISCUSSION
Differential Scanning Calorimetry (DSC)
According to Laaksonen and Roos Citation[10] the endothermic melting peak of ice was found to have onset at −18°C and the relative amount of unfrozen water content was 35.0% of total water of frozen wheat dough (flour+water). Added sucrose and NaCl decreased the onset temperature of ice melting and it was found at −26°C for doughs containing either sucrose or NaCl. Fairly low onset values of ice melting were probably due to rather high amount of maltose in the doughs, as discussed by Laaksonen and Roos Citation[10]. The onset temperatures for ice melting in maximally freeze-concentrated maltose and sucrose solutions are −30 and −34°C, respectively Citation[2], and the sugars may significantly depress the onset temperature for ice melting in frozen foods. Added sucrose and NaCl increased the relative amount of unfrozen water (Figure ), while ascorbic acid was not found to affect the amount of unfrozen water (Table ). However, the unfrozen water data derived from DSC data should be considered only as relative.
Figure 1. DSC thermograms for frozen wheat dough+sucrose, dough+NaCl, and dough+sucrose+NaCl and also their onset of ice melting temperatures, T′m, and relative unfrozen water contents, W′g (g H2O/g solute).
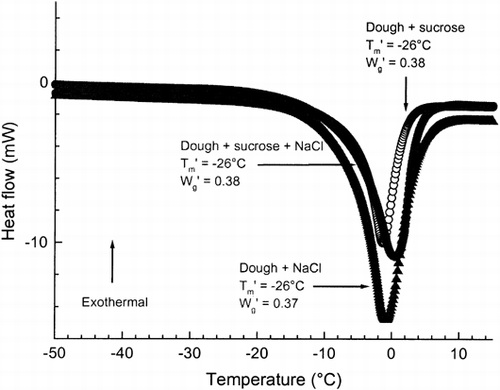
Table 1. The Onset Temperatures of Ice Melting (T′m), Relative Amounts of the Unfrozen Water Content, W′g (g H2O/g solute), and Relative Solid Concentrations in Unfrozen Water, C′g (Weight % Solute) for Different Doughs Measured by DSC
Water in foods is the most important solvent and the depressing effect of solutes, such as sugars and salts, on the freezing temperature of water in foods has been established Citation[2], Citation[14], Citation16-17. Below the freezing temperature the vapor pressure of ice is lower than that of water in the solution. Ice formation in a solution with decreased vapor pressure requires that the vapor pressure of ice becomes lower than that of water in the solution. Thereby, ice formation begins at a lower temperature. During the formation of ice below the freezing temperature, the unfrozen matrix becomes progressively more concentrated. Added solutes increase further the initial concentration of the unfrozen matrix and thereby the relative amount of unfrozen water of concentrated matrix at a constant temperature within the equilibrium freezing temperature range Citation[18].
Dynamic-Mechanical Analysis (DMA)
Effects of Sucrose
Phase and state transitions were studied by observing the storage modulus G′, the loss modulus G′′, and the loss tan δ as a function of temperature and frequency. Figure shows typical changes in the dynamic-mechanical properties of the frozen dough with added sucrose as a function of temperature. A slight peak at −31°C in the G′′ at 0.5, 2.5, 5, and 10 Hz was obtained, which corresponded to the α-relaxation (glass transition). This peak temperature value was taken as the T′g. In our previous study Citation[10], the glass transition temperature (T′g) for maximally freeze-concentrated frozen dough (flour+water) was −34°C, as measured by DMA, while the T′g values were −33 and −25°C at 0.1 and 0.5 Hz, respectively, as measured by DEA. This elevated effect of sucrose on the T′g agreed with the results of Papageorgiou et al. Citation[19] for gellan-sucrose-corn syrup mixtures. There were also opposite results concerning the effects of sucrose on the T′g Citation20-22. However, the onset temperature of the glass transition was lower than the peak temperature of the G″ maximum corresponding more closely to the DSC onset temperature of the T′g of maximally freeze-concentrated sucrose solutions of −46°C Citation[2]. The addition of sugars, in general, increase their relative amount in the unfrozen phase. Therefore, addition of sucrose may also decrease the effect of lower molecular weight solutes shifting the transition to a higher temperature.
Figure 2. The loss modulus (G′′) of frozen wheat dough with added sucrose, as a function of temperature, as measured by DMA at 2.5, 5 and 10 Hz.
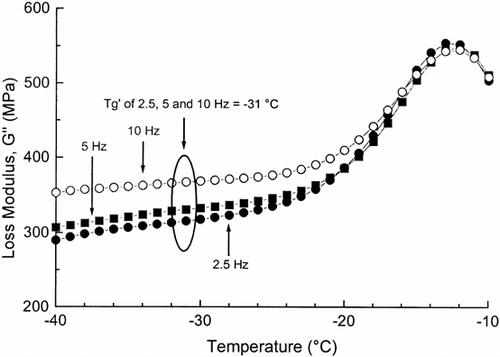
Added sucrose decreased the onset of ice melting by 7°C compared with the onset of ice melting of dough without added ingredients Citation[10] and it was at approximately −25°C (Table ). This agreed well with the results of the DSC study. In the present study, the glass transition, as taken as the G″ peak, was even closer to the onset of the ice melting than we reported earlier Citation[10] and the glass transition almost coincided with the melting of ice (Fig. ). As shown in Figure , two wide low temperature relaxations could be observed; a lower peak occurring from −136 to −100°C and a higher peak occurring from −91 to −70°C (Table ). The higher temperature peak corresponded to the β-relaxation, while the lower temperature peak has been indicated as the γ-relaxation (both relaxations were observed also on the dough without added ingredients Citation[10]). The secondary relaxation region (β and γ) probably resulted from motions within the polymer segments in the glassy state Citation[23]. Molecules of most amorphous polymers and food components contain side-groups capable of undergoing hindered rotations independently of the chain backbone, and the secondary relaxations are often ascribed to such rotations Citation[24]. These relaxations occurred at molecular level and they were probably due to local mode rotations of terminal groups or other side chains of gluten or starch, as discussed by Laaksonen and Roos Citation[10].
Figure 3. The loss modulus (G″) of frozen wheat dough with added sucrose, as a function of whole temperature range, as measured by DMA at 2.5, 5 and 10 Hz.
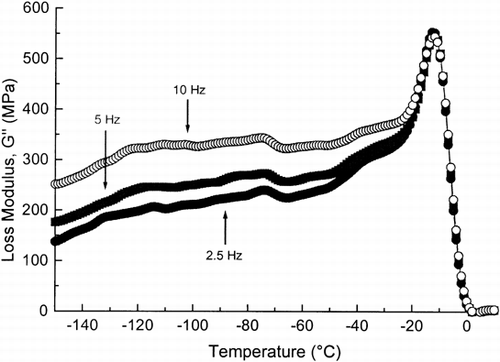
Table 2. The Onset and Endset Temperatures of β- and γ-relaxation, the Glass Transition Temperatures (T′g), and Onset of Ice Melting Temperatures (T′m) of Different Wheat Doughs, as Measured by DMA and T′m of Different Wheat Doughs Measured by DSC
Effects of NaCl
In Figure the G′′ is plotted against temperature for dough with added NaCl. A significant peak was observed at −31°C in the G″ at 0.5, 2.5, 5, and 10 Hz and it corresponded to the α-relaxation (glass transition). The effect of added NaCl for the T′g of dough taken as the temperature value of the G″ peak was equal to the T′g of dough with added sucrose. Thus, added NaCl increased T′g of dough 2°C compared with T′g of dough without added ingredients Citation[10]. The increase in the T g of polymers with addition of salts has been observed by a number of researchers. James et al. Citation[25] have found increases in the T g of poly(propylene oxide) with the addition of cobalt chloride. Cowie and Martin Citation[26] have observed that with the addition of lithium perchlorate and LiT to poly(vinyl methyl ether). These researchers have reported that the elevation in T g levels-off beyond a certain salt concentration. Moreover, Olsen and Koksbang Citation[27] showed that the T g increased dramatically with increasing LiAsF6 concentration for propylene carbonate- and hybrid polymer electrolyte.
Figure 4. The loss modulus (G″) of frozen wheat dough with added NaCl, as a function of temperature, as measured by DMA at 2.5, 5 and 10 Hz.
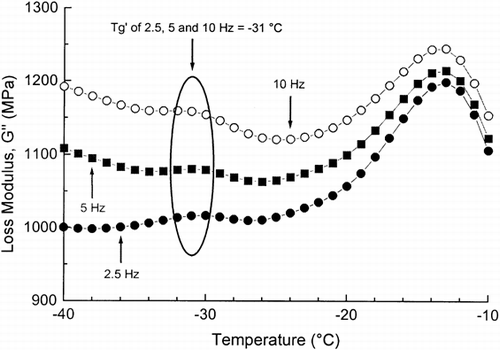
The onset of ice melting was at −26°C, as was reported in the DSC study. Two low temperature relaxations (β and γ) were also observed in doughs with added NaCl. The β-relaxation peak was very wide and it occurred from −102 to −40°C and the γ-relaxation peak was observed from −141 to −108°C (Table ).
Effects of Sucrose-NaCl-Mixture and Ascorbic Acid
Figure shows that added sucrose-NaCl-mixture increased the T′g of dough by 2°C compared with T′g of dough without added ingredients Citation[10] and it was observed at −31°C. The elevated effect of added sucrose-NaCl-mixture on the T′g of dough was equal to that found with added sucrose or NaCl separately, which was expected. The onset temperature of ice melting was found at −26°C, which agreed with our in DSC study. Both low temperature relaxations (β and γ) occurred at decreased temperatures when compared with doughs with added sucrose and NaCl separately (Table ).
Figure 5. The loss modulus (G″) of frozen wheat dough with added sucrose and NaCl, as a function of temperature, as measured by DMA at 2.5, 5 and 10 Hz.
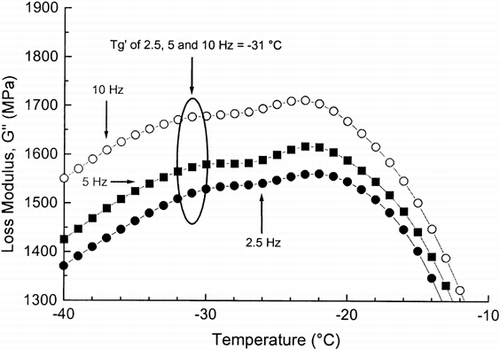
Ascorbic acid has been widely used as an oxidant in the baking industry. It should be increased in frozen dough formulations, as oxidants increase dough strength Citation[28]. This study showed that added ascorbic acid did not have noticeable effects on the physical state on the frozen doughs (Table ).
CONCLUSION
This study confirmed that differential scanning calorimetry (DSC), as used in the present study, is not sensitive enough to observe detailed information about thermal transitions in frozen doughs. Dynamic-Mechanical Analysis (DMA) was more sensitive to observe the phase and state transitions occurring in doughs at sub-zero temperatures. Added sucrose and salt decreased the relative temperature of ice melting and increased the total amount of unfrozen water. Moreover, the addition of sucrose and NaCl did not affect the T′g significantly. It seems that common freezer temperatures do not support the glassy state of freeze-concentrated solids in frozen doughs during storage.
ACKNOWLEDGMENTS
This study has been carried out with financial support from the Commission of the European Communities, Agriculture and Fisheries (FAIR) specific RTD program CT97-3609, Use of Wheat Water-Extractable Arabinoxylans (WEA) to Improve Stability of Frozen Doughs and Quality of Bread. The study does not necessarily reflect the Commission's views and in no way anticipates the Commission's future policy in this area. We are grateful to Dr. William MacInnes, Nestlé Ltd., Lausanne, Switzerland, for providing the details of the design of the DMA disc-bending sample holder. The flour and flour analysis were kindly provided by Professor Jan Delcour, Katholieke Universiteit, Leuven, Belgium.
REFERENCES
- Sperling , L. H. 1992 . Introduction to Physical Polymer Science 594 USA : John Wiley & Sons .
- Roos , Y. H. 1995 . Phase Transitions in Foods 360 New York : Academic Press .
- Roos , Y. H. and Karel , M. 1991 . Phase Transitions of Amorphous Sucrose and Frozen Sucrose Solutions . J. Food Sci. , 56 : 266 – 267 .
- Roos , Y. H. and Karel , M. 1991 . Nonequilibrium Ice Formation in Carbohydrate Solutions . Cryo-Letter , 12 : 367 – 376 .
- Simatos , D. and Blond , G. 1991 . “ DSC Studies and Stability of Frozen Foods ” . In Water Relationships in Foods Edited by: Levine , H. and Slade , L. 139 – 156 . New York : Plenum Press .
- Reid , D. S. , Hsu , J. and Kerr , W. 1993 . “ Calorimetry ” . In The Glassy State in Foods Edited by: Blanshard , J. M.V. and Lillford , P. J. 123 – 132 . Loughborough, , UK : Nottingham University Press .
- Goff , H. D. 1994 . Measuring and Interpreting the Glass Transition in Frozen Foods and Model Systems . Food Res. Int. , 27 : 187 – 189 .
- Goff , H. D. 1995 . The Use of Thermal Analysis in the Development of a Better Understanding of Frozen Food Stability . Pure Appl. Chem. , 67 ( 11 ) : 1801 – 1808 .
- Goff , H. D. and Sahagian , M. E. 1996 . Glass Transition and Aqueous Carbohydrate Solutions and their Relevance to Frozen Food Stability . Thermochimica Acta , 80 ( 2 ) : 449 – 464 .
- Laaksonen , T. J. and Roos , Y. H. 2000 . Thermal, Dynamic-Mechanical, and Dielectric Analysis of Phase and State Transitions of Frozen Wheat Doughs . J. Cereal Sci. , 32 : 281 – 292 .
- Ma , C. Y. , Harwalker , V. R. and Maurice , T. J. 1990 . “ Instrumentation and Techniques of Thermal Analysis in Food Research ” . In Thermal Analysis of Food Edited by: Harwalker , V. R. and Ma , C. Y. 1 – 15 . New York : Elsevier Applied Science .
- Wetton , R. E. 1986 . “ Dynamic Mechanical Thermal Analysis of Polymers and Related Systems ” . In Developments in Polymer Characteristics. 5 Edited by: Dawkins , J. V. 179 – 222 . New York : Elsevier Applied Science .
- Rotter , G. and Ishida , H. 1992 . Dynamic Mechanical Analysis of the Glass Transition. Curve Resolving Applied to Polymers . Macromol. , 25 : 2170 – 2176 .
- Levine , H. and Slade , L. 1988 . Principles of Cryostabilization Technology from Structure/Property Relationships of Water-soluble Food Carbohydrates – A Review . Cryo-Letter , 9 : 21 – 63 .
- MacInnes , W. M. 1993 . “ Dynamic Mechanical Thermal Analysis of Sucrose Solutions ” . In The Glassy State in Foods Edited by: Blanshard , J. M.V. and Lillford , P. J. 223 – 248 . Loughborough, , UK : Nottingham University Press .
- Sahagian , M. E. and Goff , H. D. 1996 . “ Fundamental Aspects of the Freezing Process ” . In Freezing Effects on Food Quality Edited by: Jeremiah , L. E. 1 – 50 . New York : Marcel Dekker .
- Reid , D. S. 1997 . “ Overview of Physical/Chemical Aspects of Freezing ” . In Quality in Frozen Food Edited by: Erickson , M. C. and Hung , Y. C. 10 – 28 . New York : Chapman & Hall .
- Franks , F. 1982 . “ The Properties of Aqueous Solutions at Subzero Temperatures ” . In Water-A comprehensive treatise Edited by: Franks , F. Vol. 7 , 215 – 338 . New York : Plenum Press .
- Papageorgiou , M. , Kasapis , R. K. and Richardson , R. K. 1994 . Glassy State Phenomena in Gellan-Sucrose-Corn Syrup Mixtures . Carbohydr. Polym. , 25 : 101 – 109 .
- Kalichevsky , M. T. , Blanshard , J. M.V. and Tokarczuk , P. F. 1993 . Effect of Water and Sugars on the Glass Transition of Casein and Sodium Caseinate . Int. J. Food Sci. Technol. , 28 : 139 – 151 .
- Cherian , G. , Gennadios , A. , Weller , C. and Chinachoti , P. 1995 . Thermomechanical Behavior of Wheat Gluten Films: Effect of Sucrose, Glycerin, and Sorbitol . Cereal Chem. , 72 ( 1 ) : 1 – 6 .
- Fan , J. , Mitchell , J. R. and Blanshard , J. V.M. 1996 . The Effect of Sugars on the Extrusion of Maize Grits: II. Starch Conversion . Int. J. Food Sci. Technol. , 31 : 67 – 76 .
- Gradin , P. , Howgate , P. G. , Ragnad , S. and Brown , R. A. 1989 . “ Dynamic-Mechanical Properties ” . In Comprehensive Polymer Science: The Synthesis, Characterization, Reactions and Applications of Polymers Edited by: Allen , G. and Bevington , J. C. Vol. 2 , 533 – 569 . Oxford, , England : Pergamon Press .
- McGrum , N. G. , Read , B. E. and Williams , G. 1967 . Anelastic and Dielectric Effects in Polymeric Solids 617 London : John Wiley & Sons .
- James , D. B. , Wetton , R. E. and Brown , D. S. 1979 . Polyether-Metal Salt Complexes: 1. The Elevation of the Glass Transition Temperature of Polyethers by Metal Salts . Polymer , 20 : 187 – 195 .
- Cowie , J. M.G. and Martin , A. C.S. 1987 . Ionic Conductivities and Glass Transition Temperatures in Poly(vinyl methyl ether)-salt Mixtures . Polym. Bull. , 17 : 113 – 117 .
- Olsen , I. I. and Koksbang , R. 1996 . A temperature Study of the Ionic Conductivity of a Hybrid Polymer Electrolyte . J. Electrochem. Soc. , 143 ( 2 ) : 570 – 574 .
- Inoue , Y. and Bushuk , W. 1996 . “ Effects of Freezing, Frozen Storage, and Thawing on Dough and Baked Goods ” . In Freezing Effects on Food Quality Edited by: Jeremiah , L. E. 367 – 400 . New York : Marcel Dekker, Inc. .