Abstract
The melting of chocolate in the mouth is a dynamic process. The time evolution of the perceived flavor and texture of chocolate during fat melting and sugar dissolution has been observed and quantified using time-intensity sensory methodology. Five milk chocolates varying in particle size distribution and rheology were prepared. Additionally, two chocolates varying in particle size were standardized to the same viscosity. Particle size and rheology significantly influenced effort, thickness, chocolate and sweetness attributes, although in some unanticipated ways. Averaging time-intensity responses to produce consensus curves generally yielded the same conclusions as averaging parameters extracted from individual curves. However, the later were amenable to statistical analysis using ANOVA and partial least squares regression. Multivariate analysis was a useful technique for identifying those physical properties most correlated with sensory perception.
INTRODUCTION
Chocolate exhibits the unique property of melting from a solid state at room temperature to a smooth dense suspension in the mouth at body temperature. The melting of chocolate in the mouth is defined by the characteristics of the lipid phase (Beckett, 1994a) and facilitates the perception of its characteristic taste, flavor and textural attributes. The intensity of perceived flavor changes dynamically over time as the chocolate is melted, manipulated and mixed with saliva for swallowing (Lee and Pangborn, 1986).
Traditionally, the sensory attributes of milk chocolate have been measured as single point values using quantitative descriptive methods (Burger, 1992). Attributes that are typical of and contribute to the flavor of milk chocolate have been measured using trained panelists to assess the changes in chocolate quality due to process and composition (Aguilar et al., 1994; 1995). These methods, however, provide only a single facet of information about the sample. Information such as the rate at which an attribute is perceived to its maximum intensity or the duration for which an attribute is perceived can be equally important in forming a basis of differentiation between samples. Such information can be obtained through the use of time-intensity analysis (Noble, 1995).
Time-intensity methodology collects dynamic data consistent with continuous changes in sensory perception resulting in time-intensity curves (Lee and Pangborn, 1986). Time-intensity curves have been used to calculate the maximum intensity, time to reach the maximum intensity and the duration of the stimulus for a given attribute. Advancements in technology and applications of computers in the field of sensory science now allow for extraction of as many as thirteen parameters from one such curve (Guinard et al., 1985; Noble et al., 1991). Noble et al. (1991) noted that individual judges produced unique characteristic curves that could be consistently reproduced, but which varied in shape between judges. Averaging of individual responses to create a consensus curve of intensity values did not yield the same numerical values for the derived time-intensity parameters as were calculated by averaging of the parameters derived from individual curves. Multivariate analysis has been suggested to detect the patterns of differences in the data. Van Buuren (1992) suggested the use of principal component analysis (PCA) for analyzing the time-intensity curves.
For chocolate of the same composition, processed under identical conditions, the particle size distribution of the suspended non-fat solids can play an important role in determining its flow behavior (Fischer, 1994; Mongia and Ziegler, 2000) and sensory quality (Beckett, 1994b). Mean particle size has been one of the distinguishing features of chocolate (Jackson, 1994). Kuster (1984) reported that the sensory characteristics of cocoa liquor are sensed earlier than sugar or milk powder, and presumed that with lower surface area (greater particle size), the sweetness of the sugar could be diminished. It has been hypothesized that the finer the chocolate the sweeter the taste, since small crystals dissolve more rapidly than do larger ones, but according to Niediek (1994) there was no published systematic research on the effect of particle fineness on the flavor of chocolate.
Finer particles tend to give a claggy or pasty chocolate that is harder to manipulate in the mouth (Beckett, 1994b). As a consequence, the perceived intensities and the duration of the perception of flavor and attributes may be affected (Lee et al., 1992; Pangborn et al., 1973). There are conflicting reports about the effect of viscosity on the perception of taste in the literature. While viscosity is known to affect the perception of sweetness in solutions and gel products, it does not affect the perception of chocolate flavor in desserts (Pangborn and Kayasako, 1981). This study was undertaken to elucidate the relationship between particle size distribution and the sensory properties of chocolate.
MATERIALS AND METHODS
Preparation of Chocolate
Non-fat dry milk (Darigold Inc., Seattle, WA), non-fat cocoa powder (Comet Specialty Ingredients Co., Freeport, TX) and sugar (Domino pure cane extra fine 6X granulated sugar, Domino Sugar Corp., NY), were separately ground in a jet mill (Model 0101-C6(S), Jet-O-Mizer, Fluid Energy Aljet, Plumsteadville, PA) to give milled powders with mean diameter over the volume distribution, d4,3, below 20 μm. Milled powders were conditioned at 50°C in a convection oven (Model 1350F, VWR Scientific, Pittsburgh, PA) for at least 12 h and then moved to a desiccator until used. Milled sugar was air classified (Donaldson A-12 Accucut air classifier, Donaldson Co. Inc., Minneapolis, MN) to separate a coarse and a fine fraction. The two sucrose fractions were separately mixed with the other dry particulate ingredients in the ratio given in Table , to give coarse (C100, d4,3 = 17 μm) and fine (C0, d4,3 = 8.5 μm) non-fat chocolate powders. These chocolate powders were blended by weight, in coarse to fine ratios of 25:75 (C25), 50:50 (C50) and 75:25 (C75) to generate five distinct distributions having the same composition by weight.
Table 1. Formulation Used for Preparation of Milk Chocolate
Each powder blend was processed into chocolate by mixing it with cocoa butter (Blommer Chocolate Co., Union City, CA) and anhydrous milk fat (Grassland Dairy Products, Inc., Greenwood, WI) (approximate total batch weight of 750 g), followed by conching at 65°C for 4 h in a Hobart mixer (Model N-50, The Hobart Mfg., Co., Troy, OH) equipped with a jacketed mixing bowl. After 4 h, soy lecithin (W.A. Cleary Products Corp., Somerset, NJ) was added and mixing continued for 30 min. Chocolates with 100% fine fraction (C0) and 100% coarse fraction (C100) were divided into two parts. One part was diluted with fat (cocoa butter and milk fat mixture) to lower the apparent viscosity to that of C50, yielding samples C0D (final composition 28.32% fat) and C100D (final composition 27.98% fat). The samples were tempered and molded into circular discs (28 mm diameter and 5 mm thickness).
Sensory Analysis
Sensory characteristics of the milk chocolates were evaluated using two separate evaluation methods: time-intensity (TI) procedures using software by Compusense® (Guelph, Ontario, Canada) and texture descriptive analysis (Meilgaard et al., 1991). A trained panel of ten graduate students from the Department of Food Science performed the evaluations in partitioned booths in the Sensory Evaluation Laboratory, Dept. of Food Science, Penn State University.
Commercial milk and dark chocolate products that were melted, re-tempered and molded into uniform size discs were used for training the panel. Panelists were trained to identify and scale (Table ) the chocolate and sweetness flavor attributes and the effort, coarseness and thickness textural characteristics during 7 training sessions. These attributes were chosen based on preliminary studies. During training the panelists were introduced to the evaluation procedures, and the descriptors defined and demonstrated with references. During the last three training sessions the panelists practiced TI evaluations for sweetness, chocolate and effort and scaling of coarseness and thickness of melt on a 15-point scale.
Table 2. Definitions of the Attributes, Standards, and Scaling used to Describe the Sensory Characteristics of Milk Chocolates
Samples (discs 28 mm in diameter and 5 mm thick) were cut in half and served at room temperature (20–22°C) under white fluorescent lighting. For TI evaluations of sweetness and chocolate, the panelists were instructed to place one piece (half of a disc, ∼ 1.8 g) between their molars and chew once before bringing the sample to the top of the tongue. The evaluation of attribute intensity was initiated as soon as the sample reached the top of the tongue by melting the sample between the tongue and hard palate with gentle rubbing. For TI evaluation of effort panelists placed the piece of chocolate on the top of their tongue, pressed it against the hard palate, and began their evaluation immediately. The intensity rating of all attributes was recorded at 0.5 s intervals for 100 s or until the sample was swallowed. There was a three minute waiting period between samples during which the panelists were instructed to rinse several times with carbon-filtered, room temperature water.
For the descriptive analysis evaluation of coarseness the panelists melted the sample by moving the piece of chocolate against the hard palate and observed the size of the particles. For thickness the panelists melted the sample as described for coarseness and then observed the viscosity of the melted sample. Responses were recorded on a paper ballot using a 15-cm line scale.
Twenty one samples (7 treatments X 3 replicates) were evaluated in a random order by ten judges in seven sessions (3 samples/session). The TI and descriptive evaluations were carried out as separate evaluations during each session. The panelists received a total incentive of $60.00 for participating in the study.
Statistical Analysis
Parameters extracted from time-intensity curves for each of the attributes were analyzed by ANOVA using the GLM procedure (Minitab statistical software, Minitab Inc., State College, PA) (α = 0.05) for the contributions of panelist, replicate, sample and their interactions to the variance in the data.
Principal component analysis and partial least-squares regression methods were applied to examine relationships between the samples using multivariate analysis software (Simca-S for Windows, Umetrics, Inc., Kinnelon, NJ). Principal component analysis was performed for the time-intensity curve parameters to assess the spatial arrangement of the seven samples relative to each other on the basis of their mean sensory evaluation scores. The principal component data matrices for the time-intensity attributes were correlated to that of the physical properties (Mongia and Ziegler, 2000) to establish relationships between instrumental and sensory analyses.
RESULTS AND DISCUSSION
A significant panelist*replicate interaction term for the analysis of variance suggested a lack of consistency for scoring between replicates. To identify the panelists that were not consistent between the replicates, the data for the time-intensity parameters and the descriptive parameters (coarseness and thickness) were separated for each panelist. The consistency of the panelists in scaling the time-intensity attributes was assessed by examining the analysis of variance for each of the ten parameters from the time-intensity curves with respect to the replicate, sample and their interaction. The results identified one panelist as showing significant differences (p < 0.05) in six out of ten parameters due to replicates. The data from this panelist was excluded from any further statistical analysis. The panelist* replicate interaction terms were found not significant (p > 0.05) when the analysis of variance was repeated for the data from the remaining nine panelists. The ten time-intensity parameters (Table ) for each of the three attributes and the scores of descriptive terms were separately analyzed for significance at α = 0.05. Tables and show the means (from data pooled over panelists and replicates) for the time-intensity parameters of effort, chocolate and sweetness attributes evaluated for the seven samples used in the study.
Table 3. Definitions of the Parameters Derived from the Time-Intensity Curves
Table 4. Time-Intensity Parameters for the Effort (to Melt, Manipulate, and Swallow the Sample) Attribute
Table 5. Time-Intensity Parameters for the Chocolate Attribute
Table 6. Time-Intensity Parameters for the Sweetness Attribute
Particle size significantly influenced the texture of chocolate as measured by the effort to melt, manipulate and swallow the sample (p < 0.05) with respect to seven time-intensity curve parameters (time to maximum intensity, maximum intensity, duration, area under curve, increase angle, increase area, and initial delay). “Consensus” time-intensity curves created by simply averaging all responses at each time interval for the effort attribute are shown for samples of constant composition (C0, C25, C50, C75 and C100) in Fig. . These curves reveal trends consistent with the tabulated values (Table ), but would not yield the same absolute value of the parameters. This discrepancy has been a source of concern and is a subject of ongoing research in the field of sensory sciences. For the purpose of this study the curves are presented for visual comparison among samples.
Figure 1. Average time-intensity curves for the effort attribute for samples with d4,3 of C0 = 8.5, C25 = 10.9, C50 = 12.7, C75 = 14.2, and C100 = 17.0 μm.
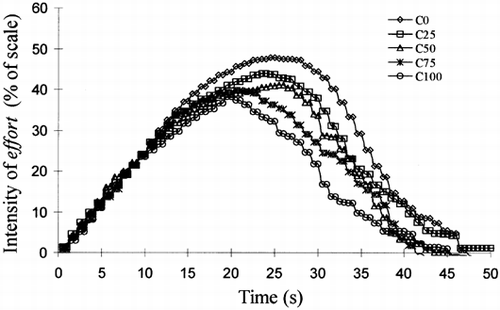
Consistent with Beckett (1994b) that a higher percentage of fine particles leads to a pasty or claggy chocolate, the data indicate that as mean particle size of nonfat solids in the chocolate decreases (from 17.0 μm in C100 to 8.5 μm in C0) the effort required to consume the sample increases. As evident in Fig. , coarser chocolates cleared the palate more quickly. The viscosity of commercial chocolates is standardized after conching by the addition of cocoa butter. To investigate the potential effect of this practice on sensory properties, the finest and coarsest samples (C0 and C100) were diluted with additional fat to produce C0D and C100D with apparent viscosities at 17 s− 1 not significantly different (p > 0.05) than that of C50. The effect of this dilution was to increase the rate at which effort was initially perceived (IangEF) compared to all undiluted samples, while the parameters from the descending portion of the curve were very similar to those of C50 (Fig. ). One could expect an increase in the amount of fat to influence the effort required to melt the sample, which occurs early in the evaluation, while viscosity influences the effort required to manipulate and swallow the bolus. Hence an effect of composition on the ascending portion of the curve, but an effect of rheology in the descending portion.
Figure 2. Average time-intensity curves for the effort attribute for samples (C0D and Cl00D) after the addition of fat to standardize their viscosity compared to the original samples (C0 and C100).
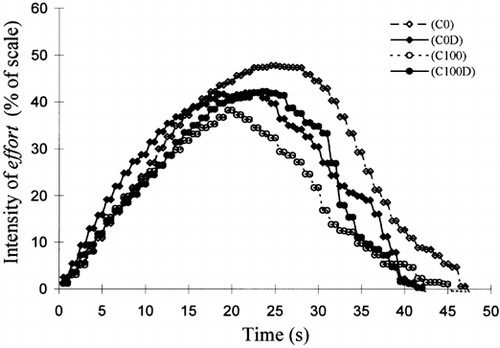
For chocolate only the duration (DurCH) and the initial intensity (InICH) were found to vary significantly between treatments (Table ). Average time-intensity curves (Fig. ) for chocolate reveal that the samples can be differentiated in the decreasing phase of the curves. DurCH correlates with DurEF (r = 0.86) and the differences in DurCH between treatments seem to represent the residence time in the mouth (Matuszewska and Barylko-Pikilna, 1995). Since the particle size and distribution of the non-fat cocoa powder, as well as, the concentration of cocoa solids was consistent for the undiluted samples, it is not at all surprising that the maximum intensity (ImaxCH) was unaffected (p > 0.05). This is one example where time-intensity methodology was able to differentiate between samples when simple quantitative descriptive analysis may have failed to do so. The dilution of the samples with fat to lower their viscosity influenced the shape of the time-intensity curves for chocolate in the same manner as the effort.
Figure 3. Average time-intensity curves for the chocolate attribute for samples with d4,3 of C0 = 8.5, C25 = 10.9, C50 = 12.7, C75 = 14.2, and C100 = 17.0 μm.
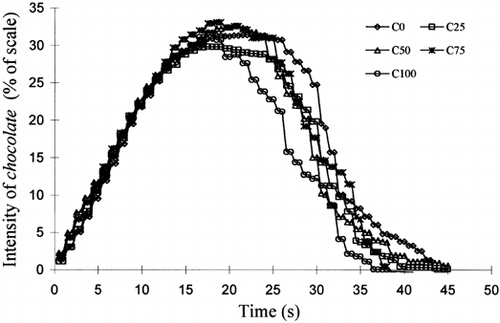
The sweetness attribute for the seven treatments could be differentiated with respect to the time to maximum intensity, duration and the increase angle (Table ). The average time-intensity curves for sweetness of the undiluted treatments (C0, C25, C50, C75 and C100) are presented in Fig. . The perception of sweetness increased faster, peaked sooner and lasted for a shorter time as the coarse fraction of particles, i.e., mean particle size, in the sample increased. Unlike chocolate, differences appeared in rate (IAngSW) and timing (TImaxSW) of the perception of sweetness. It is noteworthy that those samples with smaller mean particle sizes (C0 and C25) did not exhibit an increased sweetness intensity as has been speculated (Kuster, 1984). Higher viscosity can cause lessened perception of sweet taste (Pangborn and Kayasako, 1981), and a reduced, although not significant, ImaxSW was observed for the more viscous samples (C0 and C25). Pangborn and Kayasako (1981) showed that chocolate flavor release was not modified by viscosity in chocolate desserts. Unlike sucrose, which is perceived as the basic taste sweet, cocoa particles are largely insoluble and chocolate flavor has a large aromatic component, potentially explaining the difference between sweetness and chocolate in response to viscosity. The duration of the perception of the chocolate flavor was less than the time required to clear the palate as shown by the effort curves. This indicates that the chocolate perception was lost before the sample was swallowed, whereas the sweetness perception persisted until the end.
Figure 4. Average time-intensity curves for the sweetness attribute for samples with d4,3 of C0 = 8.5, C25 = l0.9, C50 = 12.7, C75 = 14.2, and C100 = 17.0 μm.
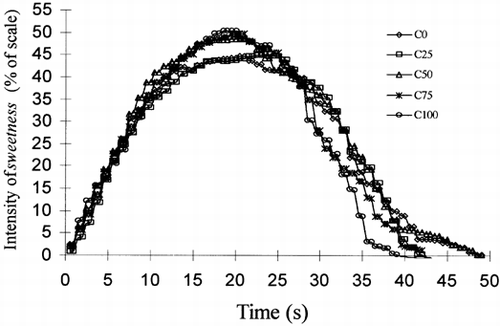
The standardization of viscosity with additional fat had the same general effect on sweetness that it had on the other attributes, i.e., it influenced the ascending portion of the curve (e.g., IAngSW), but unlike the results for effort and chocolate, the diluted C0 and C100 samples did not simply converge toward the TI curve for C50 (Fig. ). While the behavior of the coarse sample, C100, changed little, the maximum intensity of the fine sample, C0, appeared to increase dramatically. However, the information contained in the average curves did not agree well with the data as presented in Table , in which ImaxSW, while higher for C0D vis-à-vis C0, did not vary significantly. The results imply that the perception of sweetness will be greater at smaller particle size provided the viscosity is the same or lower, otherwise the intensity may be suppressed.
Figure 5. Average time-intensity curves for the sweetness attribute for samples (C0D and C100D) after the addition of fat to standardize their viscosity compared to the original samples (C0 and C100).
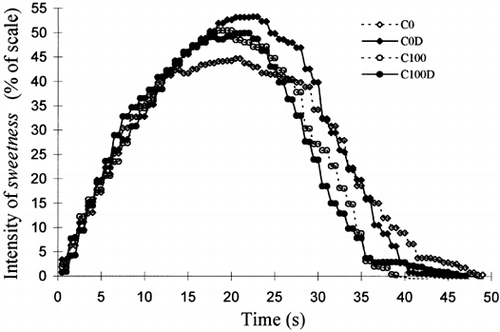
Preliminary tests showed that the perception of coarseness and thickness very quickly reached a constant maximum that persisted for the duration of the time-intensity evaluation. To reduce panelist fatigue and allow panelists to concentrate on the attributes that changed more dramatically over time, coarseness and thickness were evaluated separately using descriptive analysis methodology. According to Rostagno (1969) gritty texture can be detected in chocolates with particles larger than 35 μm, and he defined a coarse chocolate as one with at least 20% of the particles larger than 22 μm. While the coarsest chocolate prepared for this study had about 2% of particles by volume greater than 43 μm and 40% by volume greater than 24 μm, no significant differences (p > 0.05) in coarseness were detected. Although coarseness did correlate (r = 0.83) with d0.9 (Table ). (The 90th percentile volume diameter, d0.9, obtained from laser light scattering approximates the fineness measured by micrometer as a common quality control test for grinding operations.) Particle size significantly influenced the perceived thickness, which displayed a strong correlation (r = 0.97) to Casson yield value (Table ).
Table 7. Correlation Table for Physical Parameters and the Coarseness and the Thickness Scores
Principal component analysis for time-intensity parameters from all attributes resulted in three significant components (eigen value > 1) that explained approximately 74% of the variance in the data., The three components accounted for 39, 19 and 16% of the variance in the data, respectively. The five samples with the same composition were clearly differentiated across the 1st principal component. Not surprisingly, the time-intensity parameters with the greatest effect on principal component 1 were those that were significantly different by ANOVA (Tables and ). A partial least squares regression of the sensory attributes with the physical parameters of the samples (Mongia, 1997) shows the samples to be approximately linearly distributed along the diagonal axis of the two principal components (Fig. ). The physical parameters most highly correlated with sensory properties were the Casson yield value and the mean diameter, d4,3.
CONCLUSIONS
Time-intensity methodology was established as an more effective means of discriminating between samples of chocolate that varied in the size of the nonfat particulate solids than simple descriptive profiling. While the results confirmed some expectations, e.g., effort, other results seemed to be at odds with the published literature, e.g., sweetness and coarseness. The Casson yield value correlated most strongly with sensory attributes as might be expected given the apparently low shear rates in the mouth during evaluation, Both principal component analysis and partial least squares regression can be used to draw meaningful information from the time-intensity data. The ascending portion of the TI profile may be manipulated through changes in the lipid phase, while sensory perception is influence by non-fat particles once the sample is molten through their affect on the rheological properties. This provides a sound theoretical basis for the standardization of the sensory properties of chocolate products.
Acknowledgments
REFERENCES
- Aguilar , C. A. , Hollender , R. and Ziegler , G. R. 1994 . Sensory Characteristics of Milk Chocolate with Lactose from Spray-dried Milk Powder . Journal of Food Science , 59 ( 6 ) : 1239 – 1243 .
- Aguilar , C. A. , Dimick , P. S. , Hollender , R. and Ziegler , G. R. 1995 . Flavor Modification of Milk Chocolate by Conching in a Twin-screw, Co-rotating, Continuous Mixer . Journal of Sensory Studies , 10 : 369 – 380 .
- Beckett , S. T. 1994a . Control of Particle Size Reduction during Chocolate Grinding . Manufacture of Confectionary , 74 ( 5 ) : 90 – 97 .
- Beckett , S. T. 1994a . “ Traditional Chocolate Making ” . In Industrial Chocolate Manufacture and Use Edited by: Beckett , S. T. 1 – 7 . New York, NY : Chapman and Hall .
- Burger , J. 1992 . Sensory Evaluation Techniques . Manuf. Conf. , 72 ( 10 ) : 56 – 60 .
- Fischer , B. J. 1994 . Particle Size Distribution Effects on Rheology of Molten Dark Chocolate , MS Thesis University Park, PA : The Pennsylvania State University .
- Guinard , J. X. , Pangborn , R. M. and Lewis , M. J. 1985 . Computerized Procedure for Time-Intensity Measurement . Journal of Food Science , 50 : 543 – 546 .
- Jackson , K. 1994 . “ Recipes ” . In Industrial Chocolate Manufacture and Use Edited by: Beckett , S. T. 258 – 280 . New York : Chapman and Hall .
- Kuster , W. 1984 . Liquor Grinding . Manuf. Conf. , 64 ( 8 ) : 47 – 55 .
- Lee , W. E. III and Pangborn , R. M. 1986 . Time-Intensity: The Temporal Aspects of Sensory Perception . Food Technology , 40 ( 11 ) : 71 – 78 . 82
- Lee , W. E. III , Takahashi , K. and Pruitt , J. S. 1992 . Temporal Aspects of Oral Processing of Viscous Solutions . Food Technology , 46 ( 11 ) : 110 – 112 . 106, 108
- Matuszewska , I. and Barylko-Pikilna , N. 1995 . The Effect of Sample Exposure Time on the Time-Intensity Response to NaCl Solutions . Food Quality and Preference , 6 : 43 – 48 .
- Meilgaard , M. , Civille , G. V. and Carr , B. T. 1991 . Sensory Evaluation Techniques , 2nd ed. Boca Raton, FL : CRC Press .
- Mongia , G. 1997 . Particle Size Distribution Affects the Rheology and Sensory Attributes of Milk Chocolate , Ph.D. Thesis University Park, PA : The Pennsylvania State University .
- Mongia , G. and Ziegler , G. R. in press . The Role of Particle Size Distribution of Suspended Solids in Defining the Flow Properties of Milk Chocolate . International Journal of Food Properties 2000 ,
- Niediek , E. A. 1994 . “ Particle Size Reduction ” . In Industrial Chocolate Manufacture and Use Edited by: Beckett , S. T. 83 – 101 . New York, NY : Chapman and Hall .
- Noble , A. C. 1995 . Application of Time-Intensity Procedures for the Evaluation of Taste and Mouthfeel . Am. J. Enol. Vitic. , 46 ( 1 ) : 128 – 133 .
- Noble , A. C. , Matysiak , N. L. and Bonans , S. 1991 . Factors Affecting the Time-Intensity Parameters of Sweetness . Food Technology , 45 : 121 – 124, 126 .
- Pangborn , R. M. and Kayasako , A. 1981 . Time-course of Viscosity, Sweetness and Flavor in Chocolate Desserts . Journal of Texture Studies , 12 : 141 – 150 .
- Pangborn , R. M. , Trabue , I. M. and Szczesniak , A. S. 1973 . Effect of Hydrocolloids on Oral Viscosity and Basic Taste Intensities . Journal of Texture Studies , 4 : 224 – 241 .
- Rostagno , W. 1969 . Chocolate Particle Size and its Organoleptic Influence . Manuf. Conf. , 49 ( 5 ) : 81 – 85 .
- van Buuren , S. 1992 . Analyzing Time-Intensity Responses in Sensory Evaluation . Food Technology , 46 ( 2 ) : 101 – 104 .