Abstract
Changes in tenderness, unit proteolytic activity, protein denaturation and fatty acid content of trout (Onchorhyncus mykiss) cooked in microwave oven for different time-power combinations were studied and the relationship between protein denaturation and textural changes was investigated. Trout was cooked in the microwave oven at 20, 40 and 60% powers for 10, 20, 30, 40 s. As a control uncooked fish was used. Proteolysis was controlled by using microwave energy. Texture degradation due to proteolytic enzymes was reduced as microwave power increased. As cooking time and/or power of the microwave oven increased, proteolytic activity decreased which was a sign of the increase in enzyme inactivation. A correlation was found between the variation of texture and proteolytic activity (r = 0.973). It was observed that proteolytic enzymes were effective especially on myosin. Most of the fatty acids were not destroyed during microwave cooking. The optimum cooking conditions in microwave oven were determined as 60% power for 20 s of cooking time.
INTRODUCTION
Fish is a good source of polyunsaturated fatty acids (PUFA), mainly of the n − 3 family which is important to reduce the risk of cardiovascular diseases Citation[1]. The decrease in shear force value after cooking could be attributed to two possible mechanisms: thermal dissociation and the enzyme hydrolysis of muscle proteins Citation[2]. Proteolysis which results in undesirable flavor and texture alterations, can take place during post-mortem storage and processing Citation[3]. Excessive softening of the muscle tissue during cooking is a big problem. This problem is due to the high levels of endogeneous proteinases, i.e., cathepsins and heat stable alkaline proteinase Citation[4]. Cathepsins B, H and L are highly active proteases found in fish. Cathepsin L showed the highest activity at 55°C. Cathepsin B and H have an optimum activity at 20°C Citation[5]. Heat stable alkaline protease was considered to be the cause of textural degradation occurring when fish paste was processed at 55–75°C Citation[6]. Beef plasma proteins, egg white and other food grade enzyme inhibitors have been used to inhibit proteolytic activity in fish products (e.g. surimi) Citation7-8. However, these enzyme inhibitors used commercially have some negative effects such as high cost, off-odor, off-color and labelling concerns Citation[9]. Thermal inactivation of the enzyme can be an alternative method to minimize proteolysis, but inactivation should be quickly achieved since these enzymes may be activated during cooking depending on their optimum temperature. In conventional cooking methods, texture degradation may be observed since the enzymes are active for longer heating times. Ohmic heating with a rapid heating rate was found to be an effective method for maximizing the gel functionality of Pacific whiting surimi Citation[9].
Microwave technology has gained a lot of interest in recent years. The major mechanisms of microwave heating of foods are dipolar rotation and ionic conduction. Heat is generated by the rotation of polar molecules or the movement of ions to keep pace with the alternating field Citation[10]. It is possible to reach high temperatures in a short time using microwaves. Therefore, the nutritional components are less damaged during microwave processing Citation[11].
The objective of this research was to determine the optimum power and time during microwave cooking of trout by considering the proteolytic activity, texture and protein (actin and myosin) degradation. At the same time, the effect of microwave processing on fatty acid composition of fish will be studied.
MATERIALS AND METHODS
Trouts (Onchorhyncus mykiss) containing 74.0% moisture, 3.4% fat, 19.6% protein and 2.8% ash were used in the experiments. They were headed, gutted and de-boned manually as soon as they were caught off. The fish fillets were cut into two pieces separating tail and breast portions. The tail portion was discarded, breast portion was further cut into two equal pieces and each having an initial weight of 40 ± 2 g was processed in microwave oven separately. The fish were stored in ice until processing which was within 1 h post-catch. Fish samples were processed in microwave oven using different powers (20%, 40%, 60%) and times (10 s, 20 s, 30 s, 40 s). The power of the microwave oven was determined by IMPI method as 635 W Citation[10]. After processing, fish samples were placed in plastic bags and stored in the deep-freeze at −25°C for up to one month prior to use. For the texture measurements, fish samples were analyzed immediately without freezing. For the other analysis, frozen fish were thawed at 10 ± 1°C in the refrigerator. All the experiments were repeated at least three times at each experimental condition.
Texture Analysis
Texture measurements were done using a Universal Testing Machine (Lloyd Instruments LR30K, UK) with 2.5 cm diameter flat surface probe. Sample having a diameter of 2.5 cm was cut from the center portion of the fish breast by using a manually operated cutting device manufactured for this purpose. The thickness was measured accurately by using a micrometer (Mitutuyo, Japan). The samples were subjected to 25% compression with 5 N load cell and a cross-head speed of 55 mm/min. The maximum force required for 25% compression of its original thickness was reported in Newton (N).
Proteolytic Activity
The amount of protein was determined by using Kjeldahl method Citation[12]. Proteolytic activity was determined using the method of Chang-Lee et al. Citation[7] with some modifications. Casein solution was chosen as a substrate. One unit of proteolytic activity was defined as one micromole of tyrosine released per mg of protein in 2 h of incubation.
Analysis of Fish Proteins (Actin and Myosin) by Differential Scanning Calorimetry (DSC)
DSC studies were performed in a Dupont 9900 Differential Scanning Calorimeter (Dupont, Wilmington, DE) according to the method of Vissessanguan et al. Citation[13]. Samples taken from the central portion were accurately weighed to 16 ± 3 mg in aluminium hermetic DSC pans (TA Instrument, USA). All the samples were scanned at 10°C/min over the range of 30–130°C. An empty hermetic pan was used as a reference. At least three determinations were made and the most representative thermogram with the average onset and transition temperatures was reported. The apparent transition heat (ΔH) was determined from the peak area and expressed in W/g sample.
Fatty Acid Analysis
Fish samples were analysed for the fatty acids (C12, C14, C14:1tr, C14:1cis, C15, C16, C16:1 tr, C16:1cis, C16:4, C17, C18, C18:1tr, C18:1cis, C18:2tr, C18:2cis, C18:3tr, C18:3cis, C20, C20:1, C20:2, C20:3, C20:4, C22, C22:1, C22:2, C22:3, C22:5, C22:6) using the method of Castrillon et al. Citation[14]. Commercial fatty acid methyl esters (FAME) were obtained from Sigma and a standard curve was established for each FAME.
The method is generally made of four steps: Extraction, saponification, methylation and analysis of FAME in GC. Lipid fraction was obtained by Soxhlet extraction of previously dried (in a forced convection oven at 105°C overnight) and grinded sample with petroleum ether for 6 h. After hydrolysis of triacylglycerides with 0.5 N methanolic NaOH and methylation of fatty acids using BF3-methanol reagent, FAME were analyzed by Hewlett Packard HP6890 GC (Hewlett Packard Co., Palo Alto, CA) equipped with a flame ionization detector. CP-Sil 88 capiler column (50 m × 0.25 mm ID × 0.20 μm film thickness (Chrompak, Middelburg, The Netherlands)) was used. The carrier gas was Helium, both the injection and detector temperature were 250°C, column temperature was 177°C, and injection volume was 0.5 μL. The results were given in weight percentages of total fatty acid methyl esters.
Statistical Analysis
Data were analyzed by using two-way Analysis of Variance (p ≤ 0.05). If significant difference was found between processing times and/or powers, Duncan's multiple comparison test was used for comparison. Three replications were used for each analysis.
RESULTS AND DISCUSSION
Figure shows the variation of proteolytic activity of trout during microwave cooking at different powers. As the cooking time and/or microwave power increased, unit proteolytic activity decreased. During processing, 60% power, especially the first 10 s, was determined to be the most effective condition to inactivate the proteolytic enzymes. Unit proteolytic enzyme activity was halved within the first 10 s of processing at 60% power. At 20% and 40% powers, the enzyme concentration remained almost constant during the first 20 s, but it showed a significant decrease thereafter. Power 60% was found to be significantly different than the other powers in decreasing the proteolytic activity. No significant difference was found between 20% and 40% powers.
Figure 1. The variation of proteolytic activity of trout during microwave cooking at different powers (⋄ 20%a, ▪ 40%a, ▴ 60%b). Solid lines represent the model. Powers with different letters are significantly different (p ≤ 0.05).
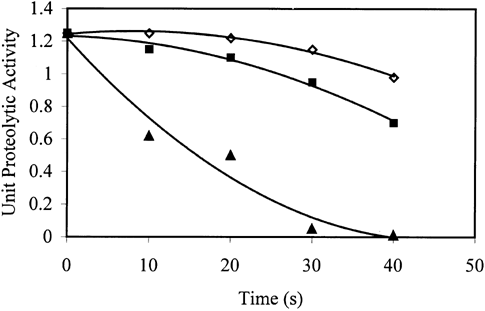
Softening in texture due to proteolysis was minimized as the power of microwave increased (Figure ). As cooking time increased, texture values increased slightly when 20% and 40% power of microwave was used. The effect of 20% power on texture was found to be significantly different than the other two powers. At 60% power, there was a decrease in texture values after a sharp increase. Cooking of trout at 60% power for 10 s caused almost three-fold increase in the force required for compression compared to unprocessed trout. The softening observed after 10 s may be explained by the dominance of the effect of cooking as compared to proteolytic enzyme inactivation. A burnt and destroyed structure was observed visually for the trouts processed at 60% power especially for 30 s and 40 s of processing during the experiments.
Figure 2. The variation of texture of trout during microwave cooking at different powers (⋄ 20%b, ▪ 40%a,▴ 60%a). Solid lines represent the model. Powers with different letters are significantly different (p ≤ 0.05).
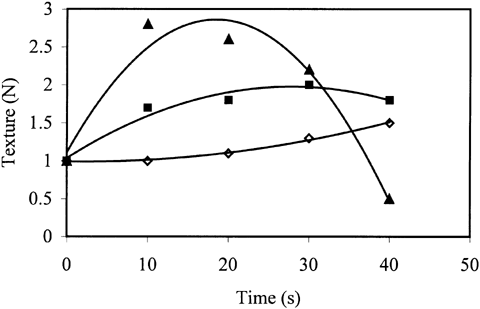
Both proteolytic activity and texture obeyed second order polynomial equation with the coefficient of determination (r 2) greater than 0.95. Figures and showed that the texture and the proteolytic activity of fish processed at 20% and 40% powers were negatively correlated (r = 0.97). This was an expected result since the reduction in proteolytic activity due to enzyme inactivation improves the texture. The sudden increase in texture and decrease in proteolytic activity in the first 10 s of processing at 60% microwave power strengthen this correlation. It was not possible to observe that correlation for 60% power after 10 s since the texture increase due to decrease in proteolytic activity is masked by the effect of thermal dissociation of muscle proteins.
DSC thermograms were analyzed and the results were tabulated in Table . The first peak of the thermogram of fish tissue corresponds to myosin Citation[15]. At 20% power, myosin peak was not detected up to processing time of 40 s. After this time a small myosin peak appeared. Actin peak was observed during all processing times but its peak area decreased due to denaturation. These results showed that the proteolytic enzymes were effective especially on myosin and the processing at 20% power was not enough to prevent proteolysis. As sample graphs, the thermograms obtained for the trout cooked using 40% microwave power for different times were given (Figures . As can be seen in these graphs, myosin peak was observed for 30 s and 40 s of processing. This showed that processing at 40% power up to 30 s was not sufficient for enzyme inactivation. As time increased, the myosin peak area, which is the energy required for denaturation of proteins increased gradually. Since the increase in peak area shows the decrease in enzyme concentration, it can be understood that the proteolytic enzyme inactivation was successfully achieved. When 60% power was used, both myosin and actin peaks were observed. However, in the case of 40 s of processing too many peaks were obtained and it was difficult to guess their corresponding proteins. This may be due to burning of the tissue and adverse effects of high temperature.
Table 1. Analysis of DSC Thermograms
When texture, proteolytic activity and DSC results are compared, a relationship arises among the decrease in texture, increase in proteolytic activity and the denaturation of myosin (lower the texture value, higher the unit proteolytic activity, smaller the myosin peak area).
The stability of the most fatty acids was observed during microwave cooking (Table ). Since cooking is achieved in a very short time in microwave oven, less damage to nutritional components was an expected result. However, according to statistical analysis, the concentration of C16, C16:4, C18:2 trans, C18:2 cis, C18:3 trans, C20:4 and C22:5 changed slightly as processing time increased (p ≤ 0.05). This shows that different fatty acids may undergo distinct changes during microwave cooking.
Table 2. Fatty Acid Ester Composition (in Weight Percentages of Total Fatty Acid Methyl Esters) of Trout Processed in Microwave Oven for Different Powers and Times
The results show that microwave cooking is advantageous with respect to the nutritional quality of fish. Considering both proteolytic activity and texture results, processing at 60% power for 20 s can be selected as the optimum condition.
CONCLUSIONS
It was possible to prevent proteolysis using microwave energy. As the microwave power and/or processing time increased, inactivation of proteolytic enzymes increased. A correlation was found between the proteolytic activity and texture. The proteolytic enzymes were found to be effective especially on myosin. The optimum cooking conditions of trout in microwave oven was determined to be 60% power and 20 s of time. In addition, microwave cooking did not have any adverse effect on fatty acid content of trout.
ACKNOWLEDGMENT
This research was supported by the Middle East Technical University (AFP-98-03-14-01).
REFERENCES
- Hearn , T. L. , Sgoutas , S. A. , Sgoutas , D. S. and Hearn , J. A. 1987 . Stability of Polyunsaturated Fatty Acids after Microwave Cooking of Fish . Journal of Food Science , 52 ( 5 ) : 1430 – 1431 .
- Deng , J. C. 1981 . Effect of Temperatures on Fish Alkaline Protease, Protein Interaction and Texture Quality . Journal of Food Science , 46 : 62 – 64 .
- Asghar , A. and Bhatti , A. R. 1987 . Endogenous Proteolytic Enzymes in Skeletal Muscle: Their Siginificance in Muscle Physiology and during Postmortem Aging Events in Carcasses . Advances in Food Research , 31 : 343 – 451 .
- An , H. , Peters , M. Y. and Seymour , T. A. 1996 . Roles of Endogenous Enzymes in Surimi Gelation . Trends in Food Science and Technology , 7 : 321 – 327 .
- An , H. , Weerasinghe , V. , Seymour , T. A. and Morrissey , M. T. 1994 . Cathepsin Degradation of Pacific Whiting Surimi Proteins . Journal of Food Science , 59 ( 5 ) : 1013 – 1017 . 1033
- Boye , S. W. and Lanier , T. C. 1988 . Effects of Heat-stable Alkaline Protease Activity of Atlantic Menhaden (Brevoorti tyrannus) on Surimi Gels . Journal of Food Science , 53 ( 5 ) : 1340 – 1342 . 1398
- Chang-Lee , M. V. , Pacheo-Aguilar , R. , Crawford , D. L. and Lampila , L. 1989 . Proteolytic Activity of Surimi from Pacific Whitining (Merluccius Productus) and Heat Set-gel Texture . Journal of Food Science , 54 ( 5 ) : 1116 – 1119 . 1124
- Morrissey , M. T. , Wu , J. W. , Lin , D. and An , H. 1993 . Protease Inhibitor Effects on Tortion Measurements and Autolysis of Pacific Whiting Surimi . Journal of Food Science , 58 : 1050 – 1054 .
- Yongsawatdigul , J. , Park , J. W. , Kolbe , E. , Abu Dagga , Y. and Morrisssey , M. T. 1995 . Ohmic Heating Maximizes Gel Functionality of Pacific Whiting Surimi . Journal of Food Science , 60 ( 1 ) : 10 – 14 .
- Buffler , C. 1993 . Microwave Cooking and Processing: Engineering Fundamentals for the Food Scientist 6 – 7 . New York : AVI Book . 157–158
- Decearau , R. V. and Peterson , R. 1986 . Microwave Processing and Engineering 18 – 21 . Chichester : Allis Horwood .
- 1975 . “ AOAC ” . In Official Methods of Analysis , 12th 15 – 16 . Washington, DC : Assoc. of Official Analytical Chemists .
- Visessanguan , W. and An , H. 2000 . Effects of Proteolysis and Mechanism of Gel Weakening in Heat-induced Gelation of Fish Myosin . Journal of Agricultural and Food Chemistry , 48 ( 4 ) : 1024 – 1032 .
- Castrillon , A. M. , Alvarez-Pontes , E. , Arias , M. T.G. and Navarro , P. 1996 . Influence of Frozen Storage and Defrosting on the Chemical and Nutritional Quality of Sardine (Clupea pilchardus) . Journal of the Science of Food and Agriculture , 70 : 29 – 34 .
- Bertola , N. C. , Bevilacqua , A. E. and Zaritzky , N. E. 1994 . Heat Treatment Effect on Texture Changes and Thermal Denaturation of Proteins in Beef Muscle . Journal of Food Processing and Preservation , 18 : 31 – 46 .