ABSTRACT
Heat transfer coefficient data in food processing were retrieved from literature and classified per process and material. Most of the data were available in the form of empirical equations using dimensionless numbers. All available empirical equations were transformed in the form of Heat Transfer Factor vs. Reynolds Number (jH=aRen). In the case when more than one equation reported for the same process and material, a new similar equation was fitted to consolidate the existing literature equations. It is expected that the resulting equations are more representative and predict more accurately the heat transfer coefficients. Average equations for each process are also proposed.
INTRODUCTION
The interface heat transfer coefficient is important in the design of food processes and processing equipment, and in the control of food packaging and storage. Heat transfer coefficients are essential in thermal processing, drying, cooling/freezing and storage operations. The surface heat transfer coefficient (h) is defined in the Newton's law of cooling:
Most of the data were available in the form of empirical equations using dimensionless numbers. According to the most common equation the heat transfer factor (jH) was a function of the Reynolds number as follows:
DATA
The data analyzed in the present paper are mainly come from the following journals:
Drying Technology, 1983–1999 | |||||
Journal of Food Science, 1981–1999 | |||||
International Journal for Food Science and Technology, 1988–1999 | |||||
Journal of Food Engineering, 1983–1999 | |||||
Transactions of the ASAE, 1975–1999 | |||||
International Journal of Food Properties, 1998–2000 |
Table 1. Number of Available Equations for Each Food Process
Table 2. Number of Available Equations for Each Food Material
REGRESSION ANALYSIS
In order to homogenize and compare the literature data by Eq. Equation2 was selected and all the available equations were transformed into Eq. Equation2. Since analytical transformation using mathematical operations does not exist a numerical transformation was used. The main steps of the numerical transformation are summarized as follow:
1. | Generate a grid of N3 values for the factors characteristic dimension of system geometry d, fluid velocity u and temperature T. | ||||
2. | Calculate the fluid properties at the grid points (e.g., density, viscosity, thermal conductivity and heat capacity etc.) and the corresponding values of Reynolds and Prandtl numbers. | ||||
3. | Calculate the heat transfer coefficient using the -equation available in the literature e.g., if the Nu number is available versus Re, h=St ρuCp if the ST number is available versus Re and so on. | ||||
4. | Calculate the corresponding jH factor using Eq. Equation4. | ||||
5. | Fit Eq. Equation2 to the available values of jH, versus Re. |
RESULTS AND DISCUSSION
The results are classified per process and material and presented in Table . All the equations are presented in Fig. to determine the range of variation of the jH and Re. The range of variation per process is also sketched in Fig. . The above results are presented analytically for each process in Figs . The effect of food material is obvious in these diagrams. The results of fitting the equation to all data for each process is summarized in Table and in Fig. .
Figure 1. Heat transfer factor (jH) vs. Reynolds Number (Re) for all the examined processes and materials.
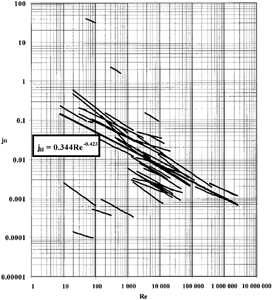
Figure 2. Ranges of variation of the heat transfer factor (jH) vs. Reynolds Number (Re) for all the examined processes.
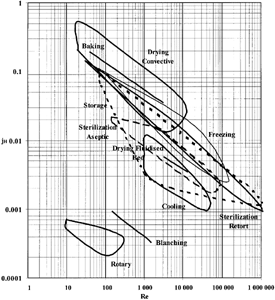
Figure 3. Heat transfer factor (jH) vs. Reynolds Number (Re) for cooling process and various materials.
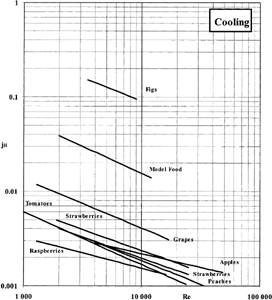
Figure 4. Heat transfer factor (jH) vs. Reynolds Number (Re) for convective drying process and various materials.
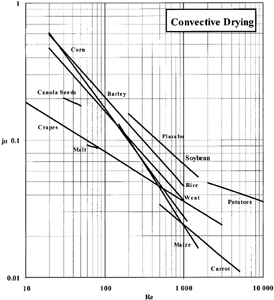
Figure 5. Heat transfer factor (jH) vs. Reynolds Number (Re) for rotary drying process and various materials.
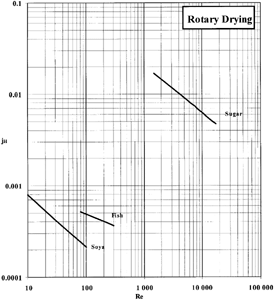
Figure 6. Heat transfer factor (jH) vs. Reynolds Number (Re) for freezing process and various materials.
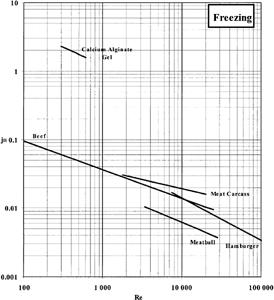
Figure 7. Heat transfer factor (jH) vs. Reynolds Number (Re) for storage process and various materials.
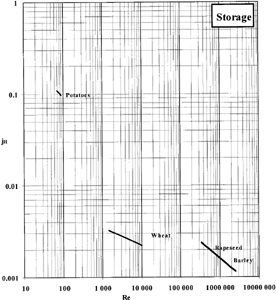
Figure 8. Heat transfer factor (jH) vs. Reynolds Number (Re) for sterilization aseptic process and various materials.
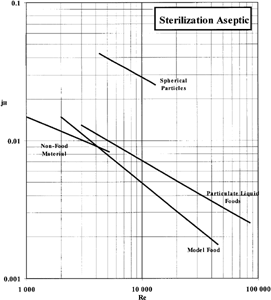
Figure 9. Heat transfer factor (jH) vs. Reynolds Number (Re) for sterilization retort process and various materials.
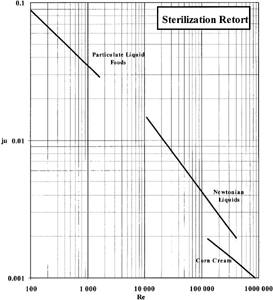
Figure 10. Estimated equations of heat transfer factor (jH) vs. Reynolds Number (Re) for all the examined processes.
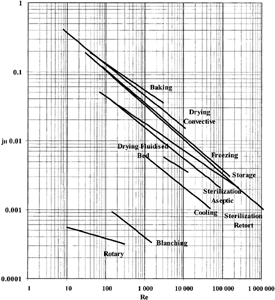
Table 3. Parameters of the Equation jH=aRen for Each Process and Each Material
Table 4. Parameters of the Equation jH=aRen for Each Process
The total error between the actual and calculated values for the equations jH=aRen, is the sum of the following errors:
The error of the initial equation (e.g., Nu=aRem Prn or h=St ρuCp which have been taken from the literature, | |||||
The numerical error due to the transformation from the initial equation to jH=aRen. | |||||
The variation among the different literature sources |
CONCLUSION
Heat transfer coefficient values for process design can be obtained easily from the proposed equations and graphs. The range of variation of this uncertain coefficient can also be obtained in order to carry out valuable process sensitivity analysis. Estimation can be done, using the proposed equations when experimental data is missing.
Acknowledgments
REFERENCES
- Rahman , M.S. 1995 . Food Properties Handbook New York : CRC Press .
- Zogzas , N.P. , Krokida , M.K. , Michailidis , P.A. and Maroulis , Z.B. 2000 . Literature Data of Heat Transfer Coefficients in Food Processing . Journal of Food Properties , Submitted
- Baik , O.D. , Grabowski , S. , Trigui , M. , Marcotte , M. and Castaigne , F. 1999 . Heat Transfer Coefficients on Cakes Baked in a Tunnel Type Industrial Oven . Journal of Food Science , 64 ( 4 ) : 688 – 694 .
- Zhang , Q. and Cavalieri , R. 1991 . Thermal Model for Steam Blanching of Green Beans and Determination of Surface Heat Transfer Coefficient . Transactions of the ASAE , 34 ( 1 ) : 182 – 186 .
- Fikiin , A.G. , Fikiin , K.A. and Triphonov , S.D. 1999 . Equivalent Thermophysical Properties and Surface Heat Transfer Coefficient of Fruit Layers in Trays During Cooling . Journal of Food Engineering , 40 ( 1–2 ) : 7 – 13 .
- Dincer , I. 1995 . Transient Heat Transfer Analysis in Air Cooling of Individual Spherical Products . Journal of Food Engineering , 26 ( 4 ) : 453 – 467 .
- Alvarez , G. and Flick , D. 1999 . Analysis of Heterogeneous Cooling of Agricultural Products Inside Bins . Journal of Food Engineering , 39 ( 3 ) : 239 – 245 .
- Dincer , I. 1997 . New Effective Nusselt-Reynolds Correlations for Food-cooling Applications . Journal of Food Engineering , 31 ( 1 ) : 59 – 67 .
- Sokhansanj , S. 1987 . Improved Heat and Mass Transfer Models to Predict Grain Quality . Drying Technology , 5 ( 4 ) : 511 – 525 .
- Lang , W. , Sokhansanj , S. and Rohani , S. 1996 . Dynamic Shrinkage and Variable Parameters in Bakker-Arkema's Mathematical Simulation of Wheat and Canola Drying . Drying Technology , 12 ( 7 ) : 1687 – 1708 .
- Mulet , A. , Berna , A. and Rosselo , C. 1989 . Drying of Carrots . I. Drying Models Drying Technology , 7 ( 3 ) : 537 – 557 .
- Fortes , M. and Okos , M. 1981 . Non-equilibrium Thermodynamics Approach to Heat and Mass Transfer in Corn Kernels . Transactions of the ASAE , : 761 – 769 .
- Torrez , N. , Gustafsson , M. , Schreil , A. and Martinez , J. 1998 . Modelling and Simulation of Crossflow Moving Bed Grain Dryers . Drying Technology , 16 ( 9&10 ) : 1999 – 2015 .
- Ghiaus , A.G. , Margaris , D.P. and Papanikas , D.G. 1997 . Mathematical Modeling of the Convective Drying of Fruits and Vegetables . Journal of Food Science , 62 ( 6 ) : 1154 – 1157 .
- Vagenas , G. , Marinos-Kouris , D. and Saravacos , G. 1990 . An Analysis of Mass Transfer in Air-drying of Foods . Drying Technology , 8 ( 2 ) : 323 – 342 .
- Mourad , M. , Hemati , M. , Steinmetz , D. and Laguerie , C. 1997 . How to Use Fluidization to Obtain Drying Kinetics Coupled with Quality Evolution . Drying Technology , 15 ( 9 ) : 2195 – 2209 .
- Lopez , A. , Virseda , P. , Martinez , G. and Llorka , M. 1997 . Deep Layer Malt Drying Modelling . Drying Technology , 15 ( 5 ) : 1499 – 1526 .
- Taranto , O. , Rocha , S. and Raghavan , G. 1997 . Convective Heat Transfer During Coating of Particles in Two-dimensional Spouted Beds . Drying Technology , 15 ( 6–8 ) : 1909 – 1918 .
- Wang , N. and Brennan , J.G. 1995 . Mathematical Model of Simultaneous Heat and Moisture Transfer During Drying of Potato . Journal of Food Engineering , 24 ( 1 ) : 47 – 60 .
- Dixon , J. , Wells , G. and Esmay , M. 1978 . Convective Heat Transfer Coefficient for Poultry Excreta . Transactions of the ASAE , : 534 – 536 .
- Shene , C. , Cubillos , F. , Perez , R. and Alvarez , P. 1996 . Modelling and Simulation of a Direct Contact Rotary Dryer . Drying Technology , 14 ( 10 ) : 2419 – 2433 .
- Alvarez , P. and Shene , C. 1994 . Experimental Determination of Volumetric Heat Transfer Coefficient in a Rotary Dryer . Drying Technology , 12 ( 7 ) : 1605 – 1627 .
- Wang , F. , Cameron , I. , Lister , J. and Douglas , P. 1993 . A Distributed Parameter Approach to the Dynamics of Rotary Drying Processes . Drying Technology , 11 ( 7 ) : 1641 – 1656 .
- Heldman , D.R. 1980 . “ Predicting of Food Product Freezing Rates ” . In Food Process Engineering Vol. 1 , Applied Science Publishers Ltd. .
- Flores , E.S. and Mascheroni , R.H. 1988 . Determination of Heat Transfer Coefficients for Continuous Belt Freezers . Journal of Food Science , 53 ( 6 ) : 1872 – 1876 .
- Tocci , A.M. and Mascheroni , R.H. 1995 . Heat and Mass Transfer Coefficients During the Refrigeration, Freezing and Storage of Meats, Meat Products and Analogues . Journal of Food Engineering , 26 ( 2 ) : 147 – 160 .
- Alagusundaram , K. , Jayas , D. , White , N. and Muir , W. 1990 . Three-dimensional, Finite Element, Heat Transfer Model of Temperature Distribution in Grain Storage Bins . Transactions of the ASAE , 33 ( 2 ) : 577 – 584 .
- Xu , Y. and Burfoot , D. 1999 . Simulating the Bulk Storage of Foodstuffs . Journal of Food Engineering , 39 ( 1 ) : 23 – 29 .
- Chang , C. , Converse , H. and Steele , J. 1993 . Modelling of Temperature of Grain During Storage with Aeration . Transactions of the ASAE , 36 ( 2 ) : 509 – 519 .
- Balasubramaniam , V.M. and Sastry , S.K. 1994 . Convective Heat Transfer at Particle-liquid Interface in Continuous Tube Flow at Elevated Fluid Temperatures . Journal of Food Science , 59 ( 3 ) : 675 – 681 .
- Sastry , S.K. , Lima , M. , Brim , J. , Brunn , T. and Heskitt , B.F. 1990 . Liquid to Particle Heat Transfer During Continuous Tube Flow: Influence of Flow Rate and Particle to Tube Diameter Ratio . Journal of Food Process Engineering , 13 ( 3 ) : 239
- Zuritz , C.A. , McCoy , S.C. and Sastry , S.K. 1990 . Convective Heat Transfer Coefficients for Irregular Particles Immersed in Non-Newtonian Fluid During Tube Flow . Journal of Food Engineering , 11 : 159 – 174 .
- Kramers , H. 1946 . Heat Transfer from Spheres to Flowing Media . Physica , 12 : 61
- Ranz , W.E. and Marshall , W.R. 1952 . Evaporation for Drops . I. Che. Eng. Progr. , 48 : 141
- Whitaker , S. 1972 . Forced Convection Heat Transfer Calculations for Flow in Pipes, Past Flat Plates, Single Cyclinders, Single Spheres, and for Flow in Packed Beds and Tube Bundles . AICheE J. , 18 : 361
- Mankad , S. , Nixon , K.M. and Fryer , P.J. 1997 . Measurments of Particle-liquid Heat Transfer in Systems of Varied Solids Fraction . Journal of Food Engineering , 31 ( 1 ) : 9 – 33 .
- Sannervik , J. , Bolmstedt , U. and Tragardh , C. 1996 . Heat Transfer in Tubular Heat Exchangers for Particulate Containing Liquid Foods . Journal of Food Engineering , 29 ( 1 ) : 63 – 74 .
- Astrom , A. and Bark , G. 1994 . Heat Transfer Between Fluid and Particles in Aseptic Processing . Journal of Food Engineering , 21 ( 1 ) : 97 – 125 .
- Anantheswaran , R.C. and Rao , M.A. 1985 . Heat Transfer to Model Newtonian Liquid Foods in Cans During End-over-end Rotation . Journal of Food Engineering , 4 : 1 – 19 .
- Sablani , S.S. , Ramaswamy , H.S. and Mujumdar , A.S. 1997 . Dimensionless Correlations for Convective Heat Transfer to Liquid and Particles in Cans Subjected to End-over-end Rotation . Journal of Food Engineering , 34 ( 4 ) : 453 – 472 .
- Zaman , S. , Rotstein , E. and Valentas , K.J. 1991 . Can Material Influence on the Performance of Rotating Cookers . Journal of Food Sciences , 56 ( 6 ) : 1718 – 1724 .
- Mallikarjunan , P. and Mittal , G.S. 1994 . Heat and Mass Transfer During Beef Carcass Chilling-modelling and Simulation . Journal of Food Engineering , 23 ( 3 ) : 277 – 292 .
- Shu-De , Q. and Fang-Zhen , G. 1993 . The Experimental Study of Rotary-stream Fluidized Bed Drying . Drying Technology , 11 ( 1 ) : 209 – 219 .