ABSTRACT
Crystallization of lactose in a co-lyophilized amorphous polysaccharide matrix was investigated under various hydration conditions to test the possible relation between the ability of the polymer to raise the glass transition temperature (Tg) of the lactose-pullulan blend, relative to pure lactose, and the crystallization kinetics. Both calorimetric (DSC) non-isothermal measurements and x-ray diffraction analysis of samples stored at a constant temperature revealed a marked retardation in lactose crystallization in the presence of pullulan; i.e., the rate constant values, as determined by the Avrami analysis, declined and the ‘half-time’ (t1/2c) for lactose crystallization increased with decreasing ratio of lactose/pullulan. The inhibitory action of pullulan on crystallization of lactose could not be solely attributed to Tg-related effects on molecular mobility of the composite systems. At a pullulan weight fraction range of 0.25–0.33 (w/w of total solids) the influence of the polymeric additive on Tg was marginal over the entire water content range examined, although crystallization was delayed as compared with pure lactose. Modeling of the temperature-dependence of t1/2c for the combined lactose-pullulan systems with the Williams-Landel-Ferry (WLF) equation was feasible only when the coefficients C1 and C2 were allowed to vary instead of assuming their ‘universal’ values.
INTRODUCTION
Sugars are present in countless food products, ranging from naturally occurring foods (e.g., honey and maple syrup, fruit juices and various dehydrated powders), to formulated foods such as jams, jellies and a large variety of beverages and confections. In these food items, sugars largely contribute to their organoleptic and textural attributes. for the latter, the physical state of the sugar plays a very important role in determining the quality of the product.Citation[1] For example, a stable amorphous state is desirable in ungrained confections (hard candies, toffee, jellies, etc.). If the glassy sugar in these products is not maintained during storage, the development of ‘graining’ (i.e., sugar crystallization) is considered as a serious defect. Similarly, the growth of lactose crystals in ice cream and in other frozen dairy deserts brings about an unpleasant sandy mouthfeel.Citation[2] Furthermore, lactose crystallization may adversely affect other changes in dairy powders, like stickiness, collapse or distortion, caking and flow behavior, nonenzymatic browning, and loss of volatiles.Citation3-4 The amorphous state of certain small molecular weight carbohydrates, e.g., trehalose, is also important in the stabilization of biomaterials (e.g., enzymes) at low moisture.Citation5-6 Prevention of crystallization in all these products is thus desirable and it can be accomplished by proper selection of storage conditions and/or formulation with nucleation-inhibiting ingredients. On the other hand, the quality of some food products relies on the presence of a crystalline phase to impart the desired texture; e.g., the presence of fine crystals in a fondant icing is critical to retain its shape in a baked good or in a filled-type candy. Since the texture of confectionary products is dependent on the number and size of the crystals formed, the nucleation and growth kinetics during product manufacture will play a critical role in the development of the final quality and textural stability of grain confections (fondant, fudge, grained nougats, etc.) and panned products.
Crystallization of carbohydrates in amorphous foods formed by freeze-drying, spray-drying, extrusion cooking, and rapid cooling from the melt, is a time-dependent process that is largely governed by the physical state of the material.Citation7-8 At relatively low temperatures, amorphous (glassy) solids are thermodynamically metastable materials of higher energy, with high viscosity and low molecular mobility. Despite a possible strong tendency to crystallize, molecules in the glassy state are not able to change their spatial arrangements to form a highly ordered crystalline state (equilibrium state) of lower free energy due to diffusional constraints; i.e., translational molecular motions are strongly inhibited in the glass. However, as the temperature is raised to above the glass transition (Tg) and/or the material is plasticized by water (e.g., via moisture sorption at high relative humidity environments), the viscosity is dramatically lowered leading to enhanced diffusion and translational mobility. This in turn fosters nucleation and crystal growth. It has been even suggested that the temperature difference between storage temperature and the glass transition temperature (ΔT=T−Tg) may control the rate of crystallization of amorphous carbohydrates.Citation7-10 Roos and KarelCitation11-13 have fitted the Williams-Landel-Ferry (WLF) equationCitation[14] to crystallization data of amorphous lactose and sucrose obtained from isothermal DSC measurements. Using induction times for crystallization as a measure of crystallization rate, the WLF equation seemed to fit reasonably well the data, implying that the crystallization rate is related to viscosity and to the relaxation times of mechanical properties above Tg; however, the experimental values for ΔT (T−Tg) were rather narrow, ranging within 24–44°C and 36–54°C for sucrose and lactose, respectively. Some studies have further suggested that using additives that raise the overall Tg above that of the pure sugar may reduce molecular mobility and thereby inhibit crystallization. Nevertheless, the work of Shamblin, Huang and ZografiCitation[15] showed that polymeric additives (polyvinylpyrrolidone and a cross-linked polysucrose) at levels as low as 1–10 Kg/100 Kg solids, which affected very little the Tg of the composite system, brought about significant inhibition of sucrose crystallization. This finding may suggest that other factors, beyond the changes in molecular mobility (related to Tg), may contribute to the effects such additives have on the crystallization kinetics. According to these authors as well as the observations of Iglesias and Chirife,Citation[16] the delayed crystallization of sucrose in the presence of polysaccharides could be related to decreased nucleation; i.e., inhibition of the diffusion of sugar molecules to form nuclei. Jouppila, Kansikas and RoosCitation[17] have recently reported an asymmetrical parabolic relationship between the extent of crystallization (level-off intensity values of characteristic peaks of the X-ray diffraction patterns) of lactose in skim milk powder and T−Tg.
To date, very few studies have looked at the effect of amorphous macromolecular matrices on the crystallization behavior of small molecular weight carbohydrates.Citation[12], Citation15-16 The objective of the present study was to examine the crystallization behavior of lactose in the presence of a co-lyophilized polysaccharide matrix (pullulan) that remains amorphous on humidification, and to test the applicability of the WLF formalism to describe lactose crystallization kinetics in these systems over a much broader range of ΔT. Co-lyophilized pullulan-lactose mixtures of varying polymer/sugar ratios were humidified at different levels of moisture and the crystallization behavior of lactose was monitored either by non-isothermal calorimetry or isothermally by X-ray powder diffraction.
MATERIALS AND METHODS
Preparation of Amorphous Systems
Amorphous samples were prepared by freeze drying of 15 Kg/100 Kg aqueous solutions of lactose or mixtures of lactose and pullulan. a-Lactose (anhydrous, grade II) was a product of Sigma Chem. Co. (St. Louis, MO, USA), whereas pullulan was a food grade preparation (PI-20) from Hayashibara Biochemical Lab Inc (Okayama, Japan). All other chemicals were of analytical grade and purchased from Merck KGaA (Darmstadt, Germany). The carbohydrates were dissolved in distilled water by heating under stirring at 70°C and small aliquots were placed in cylindrical polypropylene containers which were rapidly frozen and maintained at −25°C overnight. The samples were subsequently freeze-dried (P<0.1 mbar) using a LyoVac GT2 dryer (Leybold-Heraeus Gmbh & Co, Cologne, Germany).
Dehydrated samples were stored at various relative humidity (RH) environments in vacuum desiccators over saturated salt solutions at 25°C. The salts were LiCl, CH3COOK, MgCl2·6H2O, K2CO3, Mg(NO3)2·6H2O, NaNO2, NaCl, and KCl which correspond to RH levels (25°C) of 11, 23, 33, 43, 53, 64, 75, and 84%. In some cases, and for quick hydration, samples have been exposed to 100% RH, whereas for measurements at very low moisture content the dehydrated materials were kept over P2O5.
Differential Scanning Calorimetry
Differential scanning calorimetry (DSC) (PL DSC-Gold, Polymer Labs Ltd., Epsom, UK) was used to determine the glass transition (Tg, midpoint temperature of endothermic baseline shift) and crystallization peak temperature (Tc) of amorphous samples at different moisture contents. Small freeze-dried samples (∼8–10 mg) were placed in aluminum DSC pans and stored at various relative humidities in vacuum desiccators at room temperature as described above to acquire different moisture contents; at least duplicate samples were tested for every relative humidity environment. The pans were then hermetically sealed and analyzed by DSC. Temperature and heat flow calibration of the DSC instrument were carried out as described elsewhere,Citation[18] while the samples were scanned twice over the glass transition region zone at 5°C min−1 under a continuous nitrogen flushing (20 mL/min) to avoid condensation of moisture. The moisture content of the various systems was determined using similarly conditioned samples (in triplicate) by vacuum oven drying (70°C until constant weight). The experimental data (Tg values) derived from the DSC scans were fitted to the Gordon-Taylor (G-T) model:Citation[19]
Isothermal Crystallization of Lactose
Crystallization kinetics was monitored by XRD measurements. Dried samples (∼700 mg) in small plastic vials were placed and stored in vacuum desiccators over saturated salt solutions at 25°C. The salts were Mg(NO3)2·6H2O, NaNO2, NaCl, and KCl; respective aw levels of 0.53, 0.64, 0.75, and 0.84. At least three replicate samples were removed from the vacuum dessicators at several intervals. The samples were rapidly frozen and lyophilized, as described above, and then powdered using a mortar and pestle. At least two duplicate powdered samples for every sampling interval were subjected to WAXRD analysis at 25°C using a Philips diffractometer (PW 1830 generator, equipped with a graphite-crystal monochromator and a vertical PW 1820 goniometer). The operating conditions were: copper K α radiation (λ=0.15418 nm) in reflection mode, voltage 40 kV, amperage 30 mA, sampling interval time 0.4 s, and a diffraction angle (2θ) range from 7 to 40° (step size 0.02°). The progress of lactose crystallization during storage was followed from the increasing intensities of peaks in the XRD patterns at 19 and 20° after subtraction of the background noise. Kinetic modeling of lactose crystallization data was carried out using the Avrami equation:Citation[22]
RESULTS AND DISCUSSION
Non-Isothermal Lactose Crystallization
Figure shows typical DSC traces for pure amorphous lactose and lactose/pullulan (2:1) mixtures at specified low levels of moisture content. The main features of these diagrams were the progressive decline of the transition temperatures (Tg and Tc) and the reduction of the exothermic crystallization effect with increasing moisture content. These trends are summarized in Fig. . It was also evident that the Tc for lactose crystallization in the presence of the amorphous polysaccharide was significantly higher (∼50°C at equivalent moisture contents) than that of pure lactose. Moreover, the crystallization exotherms were broader in the case of the combined lactose-pullulan systems. The latter observations imply a significant inhibition of lactose crystallization in the composite specimens. The decline in Tg and Tc with increasing moisture (Fig. and Table ) can be viewed in terms of increased molecular mobility caused by water plasticization, as predicted by the free volume theory.Citation[4] Citation[6] Citation[7] Water and other plasticizing solutes decrease the glass transition temperatures of amorphous materials, leading to enhanced free volume and mobility, and thereby to decreased viscosity. As a result, changes in physical structure or phase/state transformations (structural collapse, crystallization, etc.) occur with increased rates. On the other hand, the inhibitory effect of pullulan on lactose crystallization could be related to changes in Tg (polymeric diluent) and/or specific effects related to mass transfer limitations of the sugar molecules or steric hindrance effects which are known to affect the extent and rate of nucleation.Citation[1] The observed delay of lactose crystallization by pullulan is in agreement with the results of previous studies on sucrose crystallization in the presence of polymeric additives such as starch,Citation[12] carboxymethylcellulose, guar gum, and sodium alginate,Citation[16] and polyvinylpyrrolidone.Citation[15] Similar to the present findings, the non-isothermal DSC data of Jouppila and RoosCitation[20] have shown a delay of ∼50°C in the onset crystallization temperature (Tc) of lactose in dehydrated milk compared to pure lactose for powdered systems stored for 24 h at RH 44%. This delay was attributed to the milk protein and other components present in the milk solids.
Figure 1. Dynamic DSC thermal scans for amorphous lactose and lactose/pullulan blends conditioned at several specified moisture content levels.
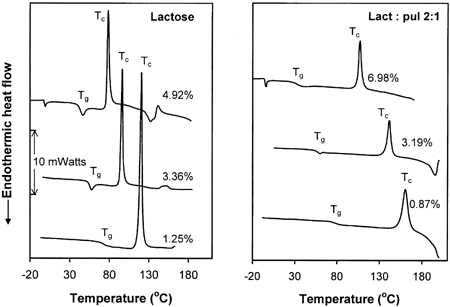
Figure 2. Effect of moisture content on apparent crystallization enthalpy and crystallization temperature (peak of exotherm) derived from dynamic DSC experiments.
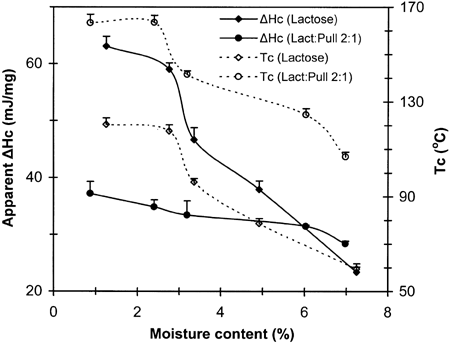
Table 1. Apparent Crystallization (Tc) and Glass Transitions Temperatures (Tg) of Freeze-Dried Lactose and Lactose-Pullulan Systems at Specified Moisture Contents as Derived from the DSC Thermal Curves
Lactose Crystallization Kinetics
Since the rates of lactose crystallization were substantially reduced in the presence of pullulan, isothermal calorimetry, which has been often used in crystallization of low molecular weight carbohydratesCitation10-12, Citation[23] and synthetic polymers,Citation[24] Citation[25] was not convenient to monitor crystallization kinetics in the humidified pullulan-lactose amorphous systems. Instead, the WAXRD technique was employed to obtain a reliable set of data points. Figures give some representative XRD patterns that were obtained for samples of lactose and lactose/pullulan (3:1) stored at specified time-RH protocols. Lactose crystallization is complicated first by the fact that there are two anomeric forms, α- and β-lactose. At room temperature the equilibrium solution contains about 38% of the α-anomer and 62% of the β-anomer, but it is the former, which owing to its far lower solubility, crystallizes under normal conditions. Amorphous lactose may also crystallize into several crystal forms (polymorphism) depending on crystallization conditions. These include α-lactose monohydrate, stable and unstable anhydrous α-lactose, anhydrous β- lactose, and mixed anhydrous of α- and β-lactose in molar ratios of 5:3 and 4:1; the latter forms do not constitute a mechanical admixture of the α- and β-forms of lactose, but rather compound crystals of the two anomers.Citation[26] All crystalline polymorphs have been identified using XRD analysisCitation27-30 and calorimetry.Citation31-33 Although a number of factors are expected to be important determinants of the crystal habit (e.g., crystallization temperature, pH, lactose concentration, moisture content, presence of nucleation-modulating compounds, mutarotation equilibria and factors affecting the ratio of the various anomeric forms), yet there is no clear understanding of the crystal (trans)formation process in lactose containing products.Citation[1] Nevertheless, amorphous lactose in milk powders has been shown to crystallize as α-lactose monohydrate, as an anhydrous mixture of α- and β-lactose in a molar ratio of 5:3, and as anhydrous β-lactose,Citation[28] Citation[34] Citation[35] depending on the RH and temperature during storage. Jouppila, Kansikas, and RoosCitation[17] Citation[36] have recently commented on the complexity of XRD patterns of crystallized lactose and lactose containing products in view of the XRD patterns of relatively pure polymorphs of lactose crystals.Citation[27] Citation[29] Citation[30] Quantitative analysis of the various crystalline forms in a specimen is thus a fairly difficult task since for most of the diffraction peaks shown in Figs. and there is a contribution from more than one type of lactose crystal. For example, three different types of lactose crystalline polymorphs contribute to the intensity of the peaks at 2θ 20° and 19°Citation[17] Citation[36] i.e., α-lactose monohydrate, anhydrous mixture of α- and β-lactose in a molar ratio of 5:3 and unstable anhydrous α-lactose for the diffraction peak at 20°, and α-lactose monohydrate, anhydrous mixture of α- and β-lactose in a molar ratio of 4:1 and stable anhydrous α-lactose for the peak at 19°. On crystallization, amorphous lactose exhibited the largest number of diffraction peaks (Fig. ). The strong peaks at 10.5° and 21° are indicative of anhydrous β-lactose crystals. This form was only found in samples of lactose crystallized at 54% RH and above as well as in the lactose/pullulan (3:1) system at 75% RH and above. On the other hand, the presence of α-lactose monohydrate, anhydrous mixture of α- and β-lactose in a molar ratio of 5:3 was evident in all samples of lactose and lactose/pullulan mixtures. Moreover, the peak at the diffraction angle of 12.8° was suggested to represent anhydrous crystals with α- and β-lactose in a molar ratio of 4:1 since it was present with peaks at diffraction angles of 13.7° and 27.4°.Citation[36] In other studies, spray dried lactose was found to crystallize as a mixture of α-lactose monohydrate and anhydrous β-lactose, when stored at above 50% RH at 25°,Citation[28] whereas freeze-dried lactose stored at 44.4% RH crystallized mainly as a mixture of three crystal forms (α-lactose monohydrate, anhydrous α- and β-lactose in a molar ratio of 5:3, and anhydrous β-lactose). Lactose in freeze-dried skim milk powder was found to crystallize largely as an anhydrous mixed crystal of α- and β-lactose in a molar ratio of 5:3.Citation[17] Differencin crystal type distributions for samples of similar composition among various reports are generally attributed to the diversity of the early nucleation process where mutarotation phenomena, RH (moisture content) and temperature conditions, and the presence of other co-solutes could influence the crystallization habit of lactose.Citation[1] Citation[17] Citation[36]
Figure 3. X-ray diffraction patterns for freeze-dried lactose stored at specified water activity environments (aw) for different time periods.
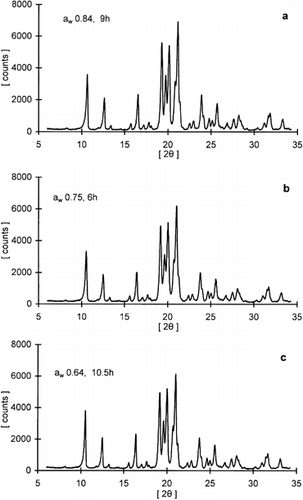
Figure 4. X-ray diffraction patterns for freeze-dried lactose/pullulan blend (3:1 w/w) stored at specified water activity environments (aw) for different time periods.
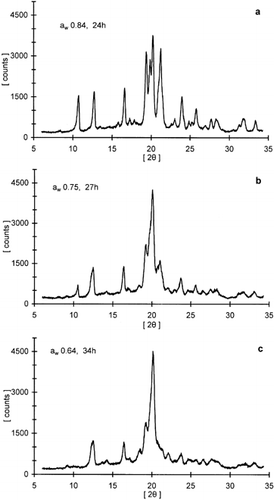
The extent of lactose crystallization (peak intensity) for all samples increased with time and then leveled off under various storage conditions. Both RH and sample composition seemed to influence the kinetics of this process. The intensity of peaks at 19° and 20° were chosen as indicators to monitor the crystallization kinetics because they were well defined diffraction lines present in all samples, reflecting the contribution of the most abundant crystal types, α-lactose monohydrate and the anhydrous crystals of α- and β-lactose in a molar ratio of 5:3. Leveling-off values of peak intensities, indicating the extent of crystallization, were different for samples stored at different RH conditions as well as among samples of varying ratio of actose/pullulan (Table ). In general, the leveling-off values decreased with decreasing ratio of lactose/pullulan. The time-dependence of the XRD crystallization data was also modeled with the Avrami equation. A representative curve for lactose crystallization is shown in Fig. along with the respective Avrami plot of the data. The crystallization kinetic parameters (leveling-off values, rate constants, Avrami exponent and half-time for crystallization) for the various systems examined are compiled in Table . Although the theoretical utility of the Avrami model in analysis of crystallization kinetics of food constituents has been argued,Citation[7] Citation[9] this equation provides a convenient tool to compare crystallization data under different storage and constituent formulation protocols. In general, the Avrami model fitted reasonably well the data at all RH environments tested in the present study. The calculated rate constants showed a progressive increase with increasing RH (or aw) as has been also shown for lactose crystallization in skim milk powder stored under similar conditions.Citation[17] Furthermore, for most RH environments examined there was evidence that the crystallization rate decreases with increasing proportion of the polysaccharide in the composite lactose-pullulan samples. These results further supported the DSC data of the inhibitory role of the hydrocolloid additive in the crystallization process.
Figure 5. Kinetics of lactose crystallization (X-ray peak intensities at diffraction angle 19°) and the respective Avrami plot of the crystallization data for the lactose/pullulan blend (3:1 w/w).
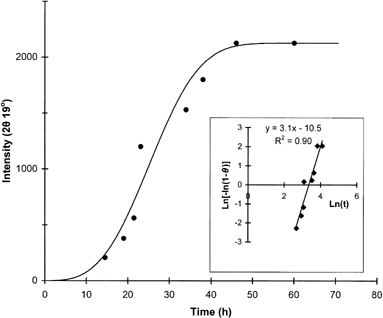
Table 2. Leveling-off Values for Extent of Crystallization (Peak Intensities at Specified Diffraction Angles) of Different Freeze-Dried Samples Stored at Various Water Activity Environments, and Calculated Rate Constants, ‘Half-time’ for Crystallization and Exponent Values from the Avrami Plots
Crystallization and Physical State
Crystallization kinetics of amorphous sugars have been claimed to be governed by the glass transition and the melting temperatures of the sugar. Direct support for this notion has come from the work of Roos and Karel.Citation11-13, Citation[37] In the context of polymer blends, one might expect a substantial elevation of Tg when lactose is co-lyophilized with a polymeric carbohydrate such as pullulan. This has prompted us to examine the antiplasticing effect of pullulan at the weight concentrations used in the present study. The calorimetrically determined Tg values of the lactose-pullulan blends (Fig. ) were used to construct the G-T plots of these materials as shown in Fig. . The fitting parameters (Tg dry solids, K and r2) of these plots are summarized in Table . At weight concentrations of pullulan between 25–33 Kg/100 Kg solids there was very little effect of the polysaccharide on the Tg of the blend, whereas at 50% (w/w) concentration there was a substantial elevation in the respective Tg values. Clearly, as seen in Fig. , there is a significant deviation from ideality (i.e., ideal mixing of free volumes from each component separately),Citation[19] implying that the free-volume additivity is not valid at intermediate compositions of the blends. Such deviations are expected from systems exhibiting a tendency towards demixing (thermodynamic incompatibility); however, there was no evidence in the DSC thermal profiles of the lactose-pullulan blends for a two-phase separated system. Nevertheless, the important observation here is that although pullulan had an almost negligible influence on the Tg value of the blend, it did exert significant inhibiting effects on lactose crystallization (Fig. and Table ). Shamblin, Huang and ZografiCitation[15] have reached to similar conclusions for crystallization of freeze-dried sucrose in the presence of relatively small amounts (1–10 Kg/100 Kg solids) of polymeric additives. Therefore, the inhibition of lactose crystallization in co-lyophilized amorphous polymeric matrix cannot be solely explained in the context of molecular mobility that is governed by the Tg of the system. Other factors more associated with the molecular environment of the lactose molecules in the composite matrix (thermodynamic and geometric factors) seemed to be more important in controlling nucleation.Citation[1] Citation[15] Michaels and van KreveldCitation[38] have also demonstrated microscopically the inhibition of individual crystal growth of lactose in supersaturated solutions by very low concentrations (10 ppm) of gelatin. According to these authors, the inhibiting action of the hydrocolloid was non-specific and most likely results from the adsorption of the gelatin onto the lactose crystal surfaces.
Figure 7. Relationships between water weight fraction and glass transition temperature (Tg) according to the Gordon-Taylor model.
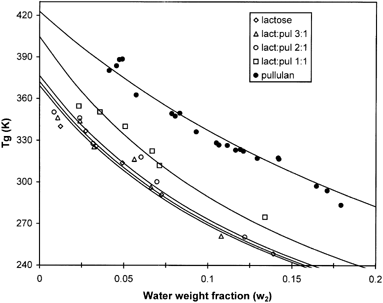
Table 3. Estimated Values of Tg dry solids from the G-T Plots and Coefficients of Determination Using the Specified Values for K
Figure shows the WLF prediction of the half-time for lactose crystallization, as calculated from the Avrami plots (Fig. , Table ). Although the half-time to crystallization of lactose decreased with increasing ΔT, the experimental data largely deviated from the theoretical line within the ΔT=30−100°C range examined (Fig. ), assuming the universal constants of the WLF equation (C1 =−17.44, C2=51.6). Instead, a fairly good fit of the WLF model (r2>0.90, p<0.05) was obtained when the Tg was used as a reference temperature and the values of the coefficients were optimized (C1=−8.95 and C2=10.6). In this context, Roos and KarelCitation[12] have shown that the WLF equation with the universal constants fitted fairly well the temperature dependence of time for crystallization of pure lactose over a much narrow ΔT (36 to 54°C) range. However, for a variety of polymers and amorphous sugars, PelegCitation[39] has concluded that the magnitude of C1 and C2 may vary considerably from the universal constants, depending on the material, the measured property, and the selected reference temperature. The fit of the WLF model to crystallization kinetics implies that the rate of crystallization is related to viscosity and to the relaxation times of mechanical properties above Tg. However, the very little influence seen on Tg elevation in the mixtures of lactose-pullulan (Fig. , solids ratios of 2:1 and 3:1 vs. pure lactose), strongly points to other contributing factors to the inhibiting action of pullulan, such as the effects from accumulation of the polysaccharide at the surfaces of individual aggregated sugar particles; i.e., mass transfer limitations to bulk molecular motion or steric hindrance effects.
CONCLUSIONS
Crystallization of lactose in a water-plasticized amorphous polysaccharide (pullulan) matrix has been investigated under non-isothermal (DSC) or isothermal storage conditions (WAXRD). With both crystallization protocols a significant inhibition of lactose crystallization was observed in the presence of the polymeric additive. In the context of the plasticizing action of water and the anticipated anti-plasticizing effect of pullulan in the sugar-polymer blends, there was very little influence on the effective Tg of the blend, compared to pure lactose, for a weight fraction of pullulan within the range 25–33 Kg/100 Kg solids. It was therefore concluded that in addition to Tg-related changes in molecular mobility, other mechanisms could be operative in controlling the rate and extent of nucleation and crystal growth. One possible factor could be that the high molecular weight carbohydrate exerts a significant effect on the mass transfer rate of lactose molecules, reducing the occurrence of nucleation as well as the positioning of sugar molecules to the growing crystal interface.
ACKNOWLEDGMENTS
This work has been carried out in part with the financial support from the Commission of the European Communities, Agriculture and Fisheries (FAIR) specific RTD programme CT96-1085 “Enhancement of Quality of Food and Related Systems by Control of Molecular Mobility”. It does not necessarily reflect its views and in no way anticipates the Commission's future policy in this area.
REFERENCES
- Hartel , R.W. and Shastry , A.V. 1991 . Sugar Crystallization in Food Products . Crit. Rev. Food Sci. Nutr. , 30 : 49 – 112 .
- White , G.W. and Cakebread , S.H. 1966 . The Glassy State in Certain Sugar-containing Food Products . J. Food Technol. , 1 : 73 – 82 .
- Saltmarch , M. , Vagnini-Ferrari , M. and Labuza , T.P. 1981 . Theoretical Basis and Application of Kinetics to Browning in Spray-dried Whey Food Systems . Prog. Food Nutr. Sci. , 5 : 331 – 344 .
- Chirife , J. and Karel , M. 1974 . Effect of Structure Disrupting Treatments on Volatile Release from Freeze Dried Maltose . J. Food Technol. , 9 : 13 – 20 .
- Green , J.L. and Angell , C.A. 1989 . Phase Relations and Vitrification in Saccharide-water Solutions and the Trehalose Anomaly . J. Phys. Chem. , 93 : 2880 – 2882 .
- Cardona , S. , Schebor , C. , Buera , M.P. , Karel , M. and Chirife , J. 1997 . Thermal Stability of Invertase in Reduced-moisture Amorphous Matrices in Relation to Glassy State and Trehalose Crystallization . J. Food Sci. , 62 : 105 – 112 .
- Slade , L. and Levine , H. 1991 . Beyond Water Activity: Recent Advances Based on an Alternative Approach to the Assessment of Food Quality and Safety . Crit. Rev. Food Sci. Nutr. , 30 : 115 – 360 .
- Roos , Y.H. 1995 . Phase Transitions in Foods San Diego, California, , USA : Academic Press .
- Slade , L. and Levine , H. 1987 . “ Recent Advances in Starch Retrogradation ” . In Industrial Polysaccharides: The Impact of Biotechnology and Advanced Methodologies Edited by: Stivala , S.S. , Crescenzi , V. and Dea , I.C.M. 387 – 430 . New York : Gordon and Breach Science Publishers .
- Kedward , C.J. , MacNaughtan , W. , Blanshard , J.M.V. and Mitchell , J.R. 1998 . Crystallization Kinetics of Lactose and Sucrose Based on Isothermal Differential Scanning Calorimetry . J. Food Sci. , 63 : 192 – 197 .
- Roos , Y. and Karel , M. 1990 . Differential Scanning Calorimetry Study of Phase Transitions Affecting the Quality of Dehydrated Materials . Biotechnol. Prog. , 6 : 159 – 163 .
- Roos , Y. and Karel , M. 1991 . Plasticizing Effect of Water on Thermal Behavior and Crystallization of Amorphous Food Models . J. Food Sci. , 56 : 38 – 43 .
- Roos , Y. and Karel , M. 1992 . Crystallization of Amorphous Lactose . J. Food Sci. , 57 : 775 – 777 .
- Williams , M.L. , Landel , R.F. and Ferry , J.D. 1955 . The Temperature Dependence of Relaxation Mechanisms in Amorphous Polymers and other Glass-forming Liquids . J. Am. Chem. Soc. , 77 : 3701 – 3707 .
- Shamblin , S.L. , Huang , E.Y. and Zografi , G. 1996 . The Effects of Co-lyophilized Polymeric Additives on the Glass Transition Temperature and Crystallization of Amorphous Sucrose . J. Thermal Anal. , 47 : 1567 – 1579 .
- Iglesias , H.A. and Chirife , J. 1978 . Delayed Crystallization of Amorphous Sucrose in Humidified Freeze Dried Model Systems . J. Food Technol. , 13 : 137 – 144 .
- Jouppila , K. , Kansikas , J. and Roos , Y.H. 1997 . Glass Transition, Water Plasticization, and Lactose Crystallization in Skim Milk Powder . J. Dairy Sci. , 80 : 3152 – 3160 .
- Biliaderis , C.G. , Lazaridou , A. and Arvanitoyannis , I. 1999 . Glass Transition and Physical Properties of Polyol-plasticised Pullulan-starch Blends at Low Moisture . Carbohydr. Polym. , 40 : 29 – 47 .
- Gordon , M. and Taylor , J.S. 1952 . Ideal Copolymers and the Second-order Transitions of Synthetic Rubbers . I. Non-crystalline Copolymers. J. Appl. Chem. , 2 : 493 – 500 .
- Jouppila , K. and Roos , Y.H. 1994 . Glass Transitions and Crystallization of Milk Powders . J. Dairy Sci. , 77 : 2907 – 2915 .
- Sugisaki , M. , Suga , H. and Seki , S. 1968 . Calorimetric Study of the Glassy State IV . Heat Capacities of Glassy Water and Cubic Ice. Bull. Chem. Soc. Japan , 41 : 2591 – 2599 .
- Avrami , M. 1939 . Kinetics of Phase Change . I. General Theory. J. Chem. Phys. , 7 : 1103 – 1112 .
- Arvanitoyannis , I. and Blanshard , J.M.V. 1994 . Rates of Crystallization of Dried Lactose-sucrose Mixtures . J. Food Sci. , 59 : 197 – 205 .
- Chan , T.W. and Isayev , A.I. 1994 . Quiescent Polymer Crystallization: Modeling and Measurements . Polymer Engineering and Science , 34 : 461 – 471 .
- Park , L.S. and Lee , D.C. 1995 . Synthesis of Copoly(Ethylene Terephthalate/Imide) and Crystallization Kinetics . Polymer Engineering and Science , 35 : 1629 – 1635 .
- Morrissey , P.A. 1985 . “ Lactose: Chemical and Physicochemical Properties ” . In Developments in Dairy Chemistry-3, Lactose and Minor Constituents Edited by: Fox , P.F. 1 – 34 . New York : Elsevier .
- Buma , T.J. and Wiegers , G.A. 1967 . X-ray Powder Patterns of Lactose and Unit Cell Dimensions of β-lactose . Neth. Milk Dairy J. , 21 : 208 – 213 .
- Bushill , J.H. , Wright , W.B. , Fuller , C.H.F. and Bell , A.V. 1965 . The Crystallization of Lactose with Particular Reference to its Occurrence in Milk Powder . J. Sci. Food Agric. , 16 : 622 – 628 .
- Simpson , T.D. , Parrish , F.W. and Nelson , M.L. 1982 . Crystalline Forms of Lactose Produced in Acidic Alcoholic Media . J. Food Sci. , 47 : 1948 – 1951 . 1954
- Fries , D.C. , Rao , S.T. and Sundaralingam , M. 1971 . Structural Chemistry of Carbohydrates. III. Crystal and Molecular Structure of 4-O-β-D-galactopyranosyl-α-D-glucopyranose Monohydrate (α-lactose Monohydrate) . Acta Crystallogr. , B27 : 994 – 1005 .
- Ross , K.D. 1978 . Rapid Determination of α-lactose in Whey Powders by Differential Scanning Calorimetry . J. Dairy Sci. , 61 : 255 – 259 .
- Lerk , C.F. , Buma , T.J. and Andreae , A.C. 1980 . The Effect of Mechanical Treatment on the Properties of Lactose as Observed by Differential Scanning Calorimetry . Neth. Milk Dairy J. , 34 : 69 – 73 .
- Lerk , C.F. , Andreae , A.C. , de Boer , A.H. , de Hoog , P. , Kussendrager , K. and van Leverink , J. 1984 . Alterations of α-lactose During Differential Scanning Calorimetry . J. Pharm. Sci. , 73 : 856 – 857 .
- Saito , Z. 1985 . Particle Structure in Spray-dried Whole Milk and in Instant Skim Milk Powder as Related to Lactose Crystallization . Food Microstruct. , 4 : 333 – 340 .
- Wursch , P. , Rosset , J. , Kollreutter , B. and Klein , A. 1984 . Crystallization of β-lactose Under Elevated Storage Temperature in Spray-dried Milk Powder . Milchwissenschaft , 39 : 579 – 582 .
- Jouppila , K. , Kansikas , J. and Roos , Y.H. 1998 . Crystallization and X-ray Diffraction of Crystals Formed in Water-plasticized Amorphous Lactose . Biotechnol. Prog. , 14 : 347 – 350 .
- Roos , Y. and Karel , M. 1991 . Water and Molecular Weight Effects on Glass Transitions in Amorphous Carbohydrates and Carbohydrate Solutions . J. Food Sci. , 56 : 1676 – 1681 .
- Michaels , A.S. and van Kreveld , A. 1966 . Influences of Additives on Growth Rates in Lactose Crystals . Neth. Milk Dairy J. , 20 : 163 – 181 .
- Peleg , M. 1992 . On the use of the WLF Model in Polymers and Foods . Crit. Rev. Food Sci. Nutr. , 32 : 59 – 66 .