ABSTRACT
Effect of barrel temperature (80°–100°C) and amylose content (28.6 gkg−1, 22.3 gkg−1 and 5.0 gkg−1) of rice upon extrusion cooking on macromolecular profile of starch was studied by gel permeation chromatography (GPC) of the rice flour on Sepharose CL-2B. Starch in all rice samples was separated into two main fractions, viz. Fraction-I, a high molecular weight, was excluded by gel, amylopectin, and Fraction-II, a low molecular weight, that entered the gel, amylose. Extrusion cooking of rice led to the degradation of high molecular weight fraction of the starch, the extent of degradation increasing with increasing severity of extrusion conditions. The absorption maxima (λ max) of iodine complex of the fraction-I showed an increase after extrusion cooking and this increase was more in the non-waxy variety of rice than in waxy.
INTRODUCTION
Extrusion cooking involves conversion of shear and frictional energy to heat, which is used to cook the feed material.Citation[1] A large variation occurs in product viscosity due to heat and pressure in the extruder. The changes in molecular and crystalline properties of starchy foods during extrusion cooking are highly dependent upon the conditions of extrusion and post-extrusion drying. Processes involving high pressure/temperature and high shear have been known to cause partial or complete destruction of crystalline structure of starch, and considerable macro-molecular degradation, as shown in the case of puffing and popping of rice,Citation2-3 drum drying and extrusion cooking of wheat.Citation4-6 The type and severity of thermal processing employed, therefore, imparts specific properties to the respective starch-based products. Changes in the two components of starch viz., amylose and amylopectin (which are present in different proportion in the native starch granules specific to the source materials and the cultivar) are reflected in changes in the functional properties exhibited by the end product.Citation7-8
An important variable in controlling extrusion operation parameters and product properties is the extruder barrel temperature. Pan et al. Citation[9] have reported that the power consumption of the extruder and the shear force were more affected by the barrel temperature than by the screw speed. They also showed that the variety (rice having amylose content between 0.1% and 26%) plays an important role in determining the type and properties of extruded products. Cai et al.Citation[10] studied the degradation of wheat starch at different barrel temperatures. Wen et al. Citation[11] have conducted detailed studies on starch fragmentation of corn meal under 15 different extrusion variables including barrel temperature. The effect of barrel temperature on the macromolecular and functional properties of corn starch has also been studied by many researchers.Citation12-13
In comparison to corn and wheat, very little work has been reported on extrusion of rice. Granular and molecular structures of rice starch differ from those of corn and wheat, so the properties of rice extrudate are expected to be different from those of corn and wheat extrudates. The process of extrusion generally involves both thermal treatment and mechanical shearing in which tissue damage is likely to occur, altering the product's qualities further. The present study was carried to elucidate the macromolecular changes in starch during extrusion of rice as a function of barrel temperature in relation to the amylose/amylopectin content of rice, and to understand the macromolecular properties of the extrudates.
MATERIALS AND METHODS
Rice
A high amylose paddy cultivar, IR64, an intermediate amylose paddy cultivar, Pojobora, and a very low amylose (i.e., waxy) variety cultivar, Agonibora, were procured from Assam Agricultural Research Institute, Titabar, Assam, India. All these three paddy cultivars were milled to about 8% degree of polish at pilot plant rice mill at CFTRI using rubber roll sheller for dehusking and huller for polishing (debraning). The milled rice was ground to flour in a hammer mill. The flour was passed through a 24 mesh British Standard (BS) sieve and used for extrusion cooking. Analysis for the proximate composition parameters of rice powder was done as per AOAC methodsCitation[14] and the results are shown in Table .
Table 1. Proximate Composition of Raw Rice Flour
Extruder and Extrusion Cooking
A co-rotating, twin-screw extruder (Model No. ZSK 30, Werner & Pfleiderer Co., Ramsey, NJ, USA) with a barrel bore of 30.85 mm and screw diameter 30.7 mm was employed with a die having a cylindrical aperture of 5 mm diameter. The extruder was provided with a digital display for Torque (T,%) developed during extrusion. The length-to-diameter (L/D) ratio of the extruder was 30.8:1. The extruder screw consisted of forward pitch screw element, a reverse pitch screw element (near the outlet), and also five kneading blocks.
The temperature of the extruder barrel was maintained at 80°, 100° or 120°C throughout the last three zones of the barrel, whereas the first two zones (feed end) were maintained at 50°C. The feed rate was maintained at 15 kgh−1 using a volumetric co-rotating twin-screw feeder. The screw speed and moisture content of the feed were maintained constant at 400 rpm, and 20.0±0.2% (dry basis, d.b.) respectively. All extrusion trials were repeated at least once.
The extrudates were dried in a tray drier at 40°C for 2 to 4 h. Moisture content of the dried samples lay in the range of 6.1–6.4% (d.b.). The extrudates were then converted to flour by grinding first in a Buhler Laboratory Disc Grinder (type MLI 204, Buhler-Miag, Italy) and then in a Fritsch Pulverisette − 14 (Fritsch GmbH, Germany) to pass through a 100 mesh (BS) sieve.
Defatting
Rice flour used for gel permeation chromatography was first defatted by refluxing with 85% methanol in a Soxhlet apparatus for 18–20 h, as described by Sowbhagya and Bhattacharya.Citation[15]
Gel Permeation Chromatography (GPC) of Starch
Gel permeation chromatography of starch was carried out essentially as per the method of Chinnaswamy and BhattacharyaCitation[2] using flour itself, rather than isolated starch, as isolation of starch from the processed sample was extremely difficult.
About 100 mg defatted flour was taken in a 100 mL conical flask. One millilitre distilled alcohol and 10 mL of 1N NaOH were added to it and left overnight. The mixture was then heated under nitrogen atmosphere for 10 min on a boiling water bath with occasional mixing. After cooling, the dispersion was neutralized with the 1N hydrochloric acid using phenol phthalein as indicator. The solution was filtered through a G-4 sintered glass filter. When perfectly dispersed, the solution became clear, though rather opalescent. The carbohydrate content of the dispersion was measured by phenol-sulphuric acid reagent method.Citation[16] The flour dispersion containing exactly 10 mg (d.b.) carbohydrate was fractionated by ascending chromatography on a Sepharose CL-2B gel (Pharmacia Fine Chemicals, Sweden) column (1.6×70 cm) operating with the peristaltic pump at a flow rate of 15 mLh−1, using distilled water containing 0.02% sodium azide as eluent. Three millilitre fractions were collected. Mean values for duplicate runs are reported.
The void volume (Vo) and the total volume (Vt) of the gel column were determined using isolated starch from waxy rice and glucose respectively by noting their elution volumes. The molecular weight of the peaks of GPC fractions was determined from the elution volume, read on a curve prepared by plotting the elution volumes of dextran standards (T20 to T2000, Pharmacia Fine Chemicals, Sweden) of different molecular weights.
Carbohydrate content of the each 3 mL sub-fraction was estimated by phenol-sulphuric acid reagent method.Citation[16] To an aliquot (0.5 mL) of each subfraction, 0.5 mL distilled water and 1 mL of 5% phenol (w/v) were added. Five millilitre concentrated sulphuric acid was added rapidly on to the liquid. The solution was mixed thoroughly using a cyclo-mixer and cooled. The absorbance of the characteristic yellow-orange colour developed was measured at 490 nm against a reagent blank in a Shimadzu UV-1601 spectrophotometer substituting distilled water for the sample. The carbohydrate content was determined using standard glucose (Sigma Chemical Co, USA) solution. The glucose equivalent obtained was multiplied with the factor 0.9 to express as anhydroglucose or starch.Citation[17] An overall mass balance was calculated to ensure that the recovery of carbohydrate from the column was reasonable.
To the aliquot (2.5 mL) of each subfraction, remaining after estimation of carbohydrate, 0.2 mL of 0.2% iodine (2 g of I2 and 20 g of KI per litre) was added for determination of total amylose (amylose equivalent). The colour developed was read in a Shimadzu UV-1601 spectrophotometer at 630 nm against iodine blank. The amylose equivalent was calculated against the absorbance of a standard potato amylose (ICN Biochemicals, USA).
The absorption maximum (λ max) of the iodine-polysaccharide complex was also determined by scanning the solution between 400 to 800 nm using a Shimadzu UV-1601 spectrophotometer.
RESULTS AND DISCUSSION
Gel Permeation Chromatography (GPC) Profile of Starch
Upon fractionation on Sepharose CL-2B gel column, starch in all rice samples was separated into two main fractions, a high molecular weight fraction eluting at the void volume (Fraction-I) and the other, a relatively lower molecular weight one, that entered the gel and was eluted over a wide range (Fraction-II). Fraction-I, the high molecular weight void volume fraction, is generally considered as the branched component of starch i.e. amylopectin, and Fraction-II, mainly the linear component i.e., amylose.Citation[2], Citation18-19 The recovery of carbohydrates in the chromatographed fractions ranged between 73.5% and 96.8% with a mean of 85.1%. Figs. show typical chromatograms of raw and extrudate products from three different varieties. Table shows data on the proportion of carbohydrate in Fraction-I, and Fraction-II and the molecular weight of peak of Fraction-II.
Figure 1. Typical GPC profiles for raw and extruded rice flour at different barrel temperatures from IR64 variety. Vo=Void volume; Vt=Total volume, in this and subsequent figures.
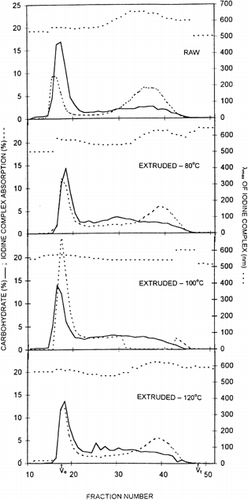
Figure 2. Typical GPC profiles for raw and extruded rice flour at different barrel temperatures from Pojobora variety.
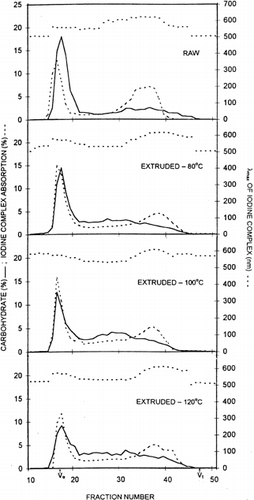
Figure 3. Typical GPC profiles for raw and extruded rice flour at different barrel temperatures from Agonibora variety.
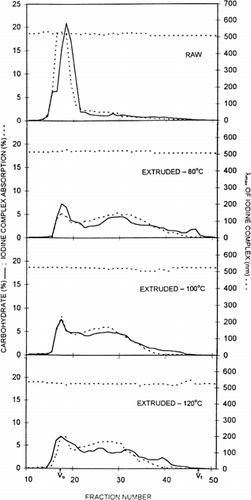
Table 2. Proportion of Carbohydrate Content (Starch) in Different Fractions (Fr-I, Fr-II) upon Fractionation over Sepharose CL-2B and Average Molecular Weight (M¯w) of Peak of Fr-II of Raw and Extruded Products from Three Rice Cultivars
Fraction-I represented 61.6%, 66.4% and 85.4% of the material eluted from the column for the unprocessed raw rice flour from IR64, Pojobora and Agonibora cultivars, respectively. The most obvious result in the chromatograms of all the extruded products was the reduction in the amount of void-volume-carbohydrate-fraction (Fraction-I) relative to unprocessed (raw) rice flour. Depending on the barrel temperature and the rice varieties, only 40.4% to 55.3% of the sample was excluded by the gel in comparison to 61.6 to 85.4% for the unprocessed rice flour (Table ). The present values are in corroboration with the results reported by Colonna and MercierCitation[20] for extruded manioc starch, and Davidson et al.Citation[21] for wheat starch. It is apparent therefore, that a significant degradation of starch occurred during extrusion processing. The degraded products entered the gel and were eluted in Fraction-II, thereby increasing the proportion of carbohydrates in the second fraction. Further as the molecular weight of theses degraded products was higher than those originally present in the Fraction-II and as the quantities also increased, the peak of the Fraction-II appeared at a lower Kav, thereby showing a shift of peak of Fraction-II from an original lower molecular weight region to a higher molecular weight side upon extrusion.
It could also been seen from Table , that the carbohydrate content in Fraction-I of the total starch decreased in case of all the varieties with consequent increase in Fraction-II to 49.8%, 55% and 59.6%, respectively. Further, among the three varieties, the reduction of Fraction-I was maximum (45% points) in the case of waxy rice variety followed by low amylose rice (21% points) for pojobora and the least (11% points) in the high amylose variety (IR64). Since the degraded products of high molecular weight component were eluted along with the low molecular weight products as one broad peak, it could not be ascertained whether the amylose (linear fraction, Fraction-II) also underwent degradation as a result of extrusion.
The extent of molecular degradation of starch in rice flour depends not only on the severity of extrusion but also on the variety of rice. As noted above, starch from waxy rice variety was degraded to the maximum extent as compared to that from high amylose variety under similar extrusion cooking conditions. Overall, it appears that the high molecular weight branched molecules (Fraction-I) were more prone to degradation than the linear ones (Fraction-II). This may be due to the large molecular size of Fraction-I, with many branch points which renders it vulnerable to degradation under severe conditions of shear and thermal forces within the extruder.
Starch from waxy variety, Agonibora was degraded to the maximum extent at lower temperature (80°C), in case of nonwaxy varieties maximum degradation was observed at 100°C extrusion. The degradation was slightly less beyond these temperatures in the respective cases. Similar observations have been reported by Wen et al.Citation[11] in case of corn meal extrusion. Maximum fragmentation was exhibited at 100°C with 20% feed moisture and high screw speed (300 rpm).
Further, it could also be noted from Table that the peak of the Fraction-II of all extruded rice samples were eluted at a slightly lesser elution volume (i.e., lower Kav) than that of unprocessed rice flour, indicating a shift of peak of this fraction towards a higher-molecular-weight region. The tendency of this shift increased with increasing barrel temperature and also with decreasing amylose content in rice variety. The average molecular weight of peak of Fraction-II also increased with the increasing barrel temperature. This indicated that the degraded products of Fraction-II had a larger molecular size in comparison to the original present in the unprocessed raw rice, as explained earlier.
Iodine Binding Nature of GPC Fractions
Table presents data on the distribution of iodine absorbance in GPC fractions of total starch, and absorption maxima (λ max) of Fraction-I and II of raw and extruded rice products from the three varieties studied. The distribution of iodine absorption of the two starch fractions separated on Sepharose CL-2B, showed a progressive increase in Fraction-I in case of IR64 and Pojobora varieties. Thus, about 33% and 42% of total iodine absorption of Fraction-I in raw rice in these varieties increased to about 65% and 52%, respectively in the extruded samples. The proportion of iodine absorption in Fraction-II consequently decreased and was accompanied by a continuous decrease in the λ max of Fraction-II peak. The λ max of raw rice of these varieties thus decreased from 656 nm–621 nm to 612 nm–608 nm in the extruded products respectively. However, the λ max for Fraction-II of Agonibora remained approximately constant at 522 nm before and after extrusion.
Table 3. Distribution of Iodine Absorbance in GPC Fraction s of Total Starch and Absorption Maxima of Fr-II of Raw and Extruded Rice Products
The λ max of iodine complex of starches of the Fraction-I of all the three varieties showed an increase after extrusion cooking (Table ). This increase was more in nonwaxy variety than waxy. Raw (unprocessed) rice of IR64 and Pojobora varieties had λ max of 561 nm and 557 nm, respectively which increased to 577 nm and 575 nm after extrusion, respectively. The λ max of Fraction-I of Agonibora (waxy), on the other hand, showed a lesser increase, from 520 nm for raw rice to 530 nm for the extruded samples.
It could be seen from the results presented in Table that the λ max of Fraction-I increased after extrusion while that of Fraction-II decreased. Similar to the observations made by Chinnaswamy et al.Citation[12] with regard to the extrusion of corn starch, it could be speculated that the population of less branched amylopectins might have increased in Fraction-I after extrusion, thus increasing λ max. Fraction-I may have gradation in the branched structures from less branched to the highly branched. The highly branched ones undergo preferential degradation due to steric hindrances yielding to high shear and temperature, while the less branched ones withstand these stresses better. The lower λ max of the degraded molecules of Fraction-I, that entered the gel, caused a decrease in the λ max of Fraction-II by increasing the proportion of branched molecules in the resultant fraction.
CONCLUSION
Starch in rice was degraded to lower molecular weight components during extrusion cooking, as evidenced by GPC studies. Upon fractionation of raw and extruded rice flour, differing in amylose content, on Sepharose CL-2B gel column, the content of carbohydrate in void volume (high molecular weight) fraction decreased, with a proportionate increase in the lower molecular weight carbohydrate, Fraction-II. Among the different rice varieties, at constant screw speed, feed moisture and feed rate, molecular degradation was maximum in waxy rice and also it got degraded maximally at a comparatively lower (80°C) temperature than the high and intermediate rice varieties, which showed maximum degradation at 100°C. These findings could serve as useful guidelines for production of better quality extruded rice products by selecting appropriate variety of rice cultivar and optimum extrusion system parameters.
Acknowledgments
REFERENCES
- Hauck , B.W. 1981 . Control of Process Variables in Extrusion Cooking . Cereal Foods World , 26 : 170 – 173 .
- Chinnaswamy , R. and Bhattacharya , K.R. 1986 . Characteristics of Gel Chromatographic Fractions of Starch in Relation to Rice and Expanded Rice-products Qualities . Staerke , 38 : 51 – 57 .
- Murugesan , G. and Bhattacharya , K.R. 1989 . The Nature of Starch in Popped Rice . Carbohydrate Polymers , 10 : 215 – 225 .
- Colonna , P. , Doublier , J.L. , Melcion , J.P. , Monredon , F.De. and Mercier , C. 1984 . Extrusion Cooking and Drum Drying of Wheat Starch. I. Physical and Macromolecular Modifications . Cereal Chemistry , 61 : 538 – 543 .
- Schweizer , T.F and Reimann , S. 1986 . Influence of Drum-drying and Twin-screw Extrusion Cooking on Wheat Carbohydrates. I. A Comparison Between Wheat Starch and Flours of Different Extraction . J. Cereal Science , 4 : 193 – 203 .
- Abdel-Aal , E.-S.M. , Sosulski , F.W. , Adel , A. , Shehata , Y. , Youssef , M.M. and Ibave , J.L. 1992 . Effect of Extrusion Cooking on the Physical and Functional Properties of Wheat, Rice and Fababean Blends . Lebensm. Wiss. u. Technol. , 25 : 21 – 25 .
- Jane , J.-L. and Chen , J.-F. 1992 . Effect of Amylose Molecular Size and Amylopectin Branch Chain Length on Paste Properties of Starch . Cereal Chemistry , 69 : 60 – 65 .
- Mujoo , R. and Ali , S.Z. 1999 . Molecular Degradation of Rice Starch During Processing of Flakes . J. Science of Food and Agriculture , 79 : 941 – 949 .
- Pan , B.S. , Kong , M.S. and Chew , H.H. 1992 . “ Twin-screw Extrusion for Expanded Rice Products: Processing Parameters and Formulation of Extrudate Properties ” . In Food Extrusion Science and Technology Edited by: Kokini , J.L. , Ho , C. T. and Karwe , M. V. 693 – 709 . NY : Marcel Dekker .
- Cai , W. , Diosady , L.L. and Rubin , L.J. 1995 . Degradation of Wheat Starch in a Twin-screw Extruder . J. Food Engineering , 26 : 289 – 300 .
- Wen , L.F. , Rodis , P. and Wasserman , B.P. 1990 . Starch Fragmentation and Protein Insolubilization During Twin-screw Extrusion of Corn Meal . Cereal Chemistry , 67 : 268 – 275 .
- Chinnaswamy , R. , Hanna , M.A. and Zobel , H.F. 1989 . Microstructural, Physiochemical, and Macromolecular Changes in Extrusion-cooked and Retrograded Corn Starch . Cereal Foods World , 34 : 415 – 422 .
- Chinnaswamy , R. and Hanna , M.A. 1990 . Macromolecular and Functional Properties of Native and Extrusion Cooked Corn Ctarch . Cereal Chemistry , 67 : 490 – 499 .
- 1984 . “ AOAC. Official Methods of Analysis ” . 14th edition Arlington, VA : Association of Official Analytical Chemists .
- Sowbhagya , C.M. and Bhattacharya , K.R. 1971 . A Simplified Colorimetric Method for Determination of Amylose Content in Rice . Staerke , 23 : 53 – 56 .
- Dubois , M. , Gilles , K.A. , Hamilton , J.K. , Rebers , P.A. and Smith , F. 1956 . Colorimetric Method for Determination of Sugars and Related Substances . Analytical Chemistry , 28 : 350 – 356 .
- Lyne , F.A. 1976 . “ Determination of Starch in Various Products ” . In Examination and Analysis of Starch Products Edited by: Radley , J.A. 167 – 188 . London : Applied Science Publishers .
- Biliaderis , C.G. , Grant , D.R. and Vose , J.R. 1979 . Molecular Weight Distribution of Legume Starches by Gel Chromatography . Cereal Chemistry , 56 : 475 – 480 .
- Biliaderis , C.G. , Grant , D.R. and Vose , J.R. 1981 . Structural Characterization of Legume Starches. I. Studies on Amylose, Amylopectin and Beta-limit Dextrins . Cereal Chemistry , 58 : 496 – 502 .
- Colonna , P. and Mercier , C. 1983 . Macromolecular Modifications of Manioc Starch Components by Extrusion Cooking with or without Lipids . Carbohydrate Polymers , 3 : 87 – 107 .
- Davidson , V.J. , Paton , D. , Diosady , L. and Rubin , L.J. 1984 . A Model for Mechanical Degradation of Wheat Starch in a Single-screw Extruder . J. Food Science , 49 : 1154 – 1157 .
- Reddy , K.R. , Ali , S.Z. and Bhattacharya , K.R. 1993 . The Fine Structure of Amylopectin and its Relation to the Texture of Cooked Rice . Carbohydrate Polymers , 22 : 267 – 275 .