ABSTRACT
Starch gels containing mixtures of sago+wheat of different protein content at different levels of sago substitutes were prepared as follows: sago+high protein wheat (HPW), sago+medium protein wheat (MPW) and sago+slow protein wheat (LPW). The rheological profile analysis (RPA) related to texture was carried out for sago-wheat mixtures at different percentages of sago substitutions of 10,15, 20, 30, 40 and 50% of sago. The hardnes for control sago gel increased gradually with the increment of concentration of gel and the value tends to be higher than control wheat gels at 20% concentration. The gel becomes too hard for control sago when the concentration exceeded 30%. In RPA study, gel did not exhibit any significant differences in hardness, cohesiveness and adhesiveness with sago portion in HPW, MPW and LPW flour mixtures at different percentages. In retrogradation study, the hardness was found to decrease in comparison to the values for fresh samples.
INTRODUCTION
Sago starch (Metroxylene sago) derived from the pith of several kinds of palm trees native to East Indies, growing extensively in the peat swamps of Malaysia, Papua New Guinea and Indonesia. It is also cultivated in Japan and South America. In major wheat-growing areas, wheat starch is produced almost as a by-product because of the high price commended by dried and vital gluten. It shares with wheat flour obvious uses in the food industries as a thickener and gelling agent. In South-East Asian countries sago starch is cheaper then wheat starch and produces an opaque paste similar in clarity to that of wheat starch, has medium paste strength under mechanical shear and heating. So sago starch is used as a substitute of wheat starch in sago-wheat flour mixture at different ratio.
According to Ingrid et al.,Citation[1] few substances can be present in a food system without affecting starch. Understanding these effects is important for better process control and for improving the texture and other quality attributes of starch-based foods. Therefore, selection and use of starches in food products for consistent production quality and for innovative new products require good understanding of starch properties and their interaction with other food components, as well as their behavior during processing and storing.
Texture is one of the important sensory acceptability factors for foods.Citation[2] Many studies have understood the importance of texture of foods.Citation3-4 During the course of consumption, a food product undergoes several changes. Changes taking place during mastication,Citation[2] including mixing with saliva, communication and temperature change. Thus, to understand texture of foods, one needs to understand the perceptory processes employed during texture evaluation and also the apparatus employed to relate these.
The basic texture of a wide variety of formulated foods stems from the gelling properties of starch (polysaccharides), and proteins, which are increasingly used to create and modify textural properties in foods. During the processing, manufacture and consumption of food, these gelled systems are subjected to large deformations, which may cause the food either to deform irreversibly or to fracture. Suppliers and users to characterize gelled systems have used single point measurements such as “gel strength”. However, these single point measurements, often based on rupture tests, are not representative of the overall mechanical behaviour of gel.
In recent decades, much work has been done on the development of mechanical tests that tried to imitate the sensory evaluation of food texture.Citation[5] Among these instrumental rheological profile analysis (RPA) related to texture has been applied as a useful method for a wide range of foods. Rheological profile analysis (RPA) related to texture is widely used in basic research and new product development in foods.Citation6-7 The origin of RPA related to texture was Szczesniak's classification of textural characteristics,Citation[8] the key principle of which was to serve as a bridge between the instrumental and sensory evaluation of texture. The use of General Foods (GF) Texturometer by Szczesniak's research team was the major breakthrough in instrumental rheological profile analysisCitation[7] related to texture. This equipment which was a modification of the original MIT Denture Tenderometer was designed to simulate the masticating action of the human mouth and can be described as a unit composed of a plate supported by a flexible arm attached to a strain gauge, and a plunger which acted upon the food sample, usually a bite-size piece, compressing it twice in a reciprocating motion that imitated the action of the jaw. The curve generated by the GF Texturometer is plot of force as a function of time. SzeczesniakCitation[9] bases interpretation of the Texturometer curves on the earlier classification of mechanical parameters of texture. The curve provided seven rheological parameters, five measured and two calculated from the measured ones. These parameters were named hardness, cohesiveness, elasticity, adhesiveness, brittleness, chewiness, gumminess and viscosity.Citation[6] According to these authors, brittleness and adhesiveness are never in counted in the same food productCitation[10] evaluated selected foods for GF TPA parameters, reporting that correlation was good between sensory and instrumental evaluations. Cohesiveness and elasticity values can be obtained from Texturometer curves, but these parameters were not included in the early organoleptic evaluations because they were very difficult to perceive as such. Studies the RPA of gel for the mixture of sago and wheat at different levels of sago substitution are the subject of this paper because not much work has been done on sago-wheat mixed flour gel to diversify the application of sago in wheat based food products.
MATERIALS
High protein wheat (HPW) flour (bread flour), medium protein wheat (MPW) flour (rose flour) and low protein wheat (LPW) flour (super soft flour) samples were bought from local superstore. Sago starch was supplied from Craun Research SDN. BHD., Kuching, Sarawak, Malaysia.
METHODS
Determination of Moisture Content
Moisture content of wheat flours and sago was determined as AACC methods (Method no. 44–15).Citation[11]
Determination of Protein, Fat, and Amylose Content
Protein content of wheat and sago was determined by micro-Kjeldahl distillation method. Soxhlet extraction method was used to determine fat content of wheat and sago (AOAC, 12). Petroleum ether (b.p. 40–60°C) was used for fat extraction. The amylose content of sago and wheat was measured by using the methods of Williams et al.Citation[13]
Mixed Flour Gel Preparation
Mixed flours and control flours gel were prepared for each ratio at 20% concentration (dry weight basis). Samples were prepared approximately 3.5% (w/w), and were heated in a boiling water bath at 100°C for about one hour with gentle stirring, and then cooled down to room temperature (25±1°C).
Remainder flour was added to the suspension with a constant stirring. then it was heated again in the water bath for two hours. Paraffin oil was applied on the surface of samples to reduce dehydration. After boiling, the gels were stored at 4°C for 16 to 18 h before rheological measurement.
RHEOLOGICAL ANALYSIS
Rheological Profile Analysis (RPA)
Rheological properties of mixed flour gels type were determined by Texture Analyzer-XT2, version 1.05 (Stable Micro Systems, Goldaming, Surrey, UK). A-D Exchanger, which linked to microcomputer, transmitted signal voltan. Cylindrical gel (1 cm diameter, 1 cm height) was compressed by a cylindrical probe (1.5′′ diameter) to 85% deformation. The parameter setting and operation of the instrument were accomplished through a PC with Texture Expert software version 1.0. The force and probe calibration procedure for the system were followed before actual tests. The text mode was set to ‘texture profile in compression’. The pre-test, test and post-test speeds were set to 5, 5 and 5 mm/sec, respectively. Each test was repeated 5 times at room temperature (25±1°C).
RESULTS AND DISCUSSION
Composition
The moisture content of wheat (HPW, MPW and LPW) flour and sago was found to be 12.9% for HPW flour, 12.9% for MPW flour, 12.8% for LPW flour, and 13% for sago. The results for protein, fat and amylose content of wheat and sago are shown in the Table . The ratios of mixtures of sago and wheat have been shown in the Table .
Table 1. Chemical Composition of Samples Used in the Experiment (Dry Basis)
Table 2. Wheat and Sago Mixtures Were Determined by Following Ratios
Rheological Profile Analysis (RPA)
Szczeniak's proposed rheological parameters like hardness, cohesiveness, adhesiveness, springiness and stickiness are most important in RPA related to texture.Citation[9] Figure shows typical force-diagram curve for RPA of the sago gel at 20% concentration. The results are shown in Figs. .
Figure 4. Hardness of gels prepared from wheat-sago mixtures at different percentages of sago (retrogradation, gels stored for 4 days).
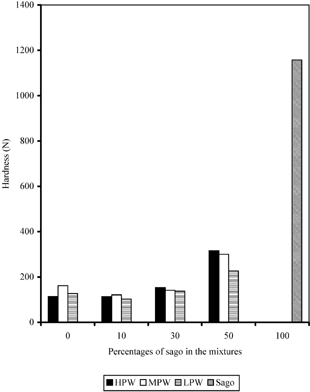
The hardness for 15% control sago gel and for control wheat flour gel was observed to be almost the same (Fig. ). The hardness of sago gel increased rapidly at 20% and then gradually up to 30%. For control HPW, MPW and LPW flours, the hardness was increased slightly with gel concentration up to 20%. For 25% gel, the values of hardness remain almost the same value with 20%, but further increase could be observed for three types of control wheat flour at 30% (Fig. ). The hardness of HPW flour gel tends to increase with sago portion up to 10%, and then gradually decreased with sago portion to 40%, followed by a slight increase with sago portion 50%. For LPW and MPW, the same trends in the hardness have been observed (Fig. ).
For retrogradation study the hardness of the same sample was measured after 4 days, the samples were kept at 4±0.5°C temperature. The results are shown in Fig. . The experiment was conducted for three portion of sago (10%, 30% and 50%) and also for control flour. Therefore, the hardness was found to decrease compared to the hardness of fresh samples.
The hardness for the gel mixtures containing fixed concentration (20%) of flour in the gels and varying portion of sago is shown in Fig. . For control wheat the hardness of LPW and HPW flour was the same, for MPW flour it is higher than others. In fact, it has been observed that for all percentages of sago in the mixtures, the hardness of MPW is always higher than the other two types of mixtures; the reason for this is not clear to us. This could be attributed to interaction between amylose-protein interactions. BiliaderisCitation[14] has shown that the modulus of amylose gels is less temperature sensitive than amylopectin gels. The amylose gelation process when compared to amylopectin gelation is much more rapid.Citation15-16 Sago starch contain more amylose than wheat (Table ), resulting in the dilution of protein content in wheat flour by sago, consequently increase amylose in the mixtures according to the ratio of sago. So, these statements are supporting our experimental results in Figs. and .
Retrogradation is an important functional attribute in starch-based foods. It involves the reassociation of starch polymers after they have undergone gelatinisation, resulting in gel firmingCitation[17] during storage. After heating starch slurry to 90–95°C, amylose reassociates rapidly during cooling, while amylopectin slowly recrystalize during the storage.Citation[18] Although numerous investigations have been undertaken on this phenomenon, the exact mechanism of retrogradation, particularly at the molecular level, is not clear. Retrogradation is a complex process and depends on many factors such as type of starch, starch concentration, cooking and cooling regimes, pH, and the presence of solutes such as lipids, salts, sugars, and other biopolymers; for example, proteins and polysaccharides.Citation[19]
The value for springiness of all the gel mixtures was found to be very small therefore, can be neglected. The result of the RPA for cohesiveness is shown in Fig. . The value of cohesiveness of MPW flour mixtures for all sago ratios was found to be higher than the values of LPW and HPW, flour mixtures. The trend of cohesiveness for all sago ratios were found to increase with sago portion up to 20%, followed by a gradual decrease with sago portion up to 50%. At the ratio 20% of sago, the MPW flour mixtures have the maximum cohesiveness value (Fig. ) followed by HPW and LPW flour mixtures. The cohesiveness of control sago was lower than the cohesiveness of control wheat and mixtures.
The results of adhesiveness are shown in Fig. . For all types of mixtures, adhesiveness increased with increment of sago portion up to 20% followed by a gradual decrease with sago portion up to 50%, except for the HPW flour mixture at 50%, here the value increased rapidly (Fig. ). For control MPW flour and for 10% sago in the mixtures, the adhesiveness of MPW was higher than HPW and LPW. However, for 20% and 50% of sago in the mixtures, HPW flour has higher adhesive value than the other mixtures, except for the 40 sago. It was noted that at 10% sago in the mixture, the MPW had the highest RPA adhesiveness and the adhesiveness of control sago was negligible.
Adhesiveness is work necessary to overcome the attractive forces between the surface of the food and the surface of the other materials with which the food comes in contact. Figure shows that HPW flour with the ratio of 50% sago exhibited the highest adhesiveness among all types of flour. This statement indicate that internal bonding for constructing or forming the gel body would be stronger than other gels, while LPW flour mixture gel with the ratio of 10% sago has the weakest internal bonding.
There are two categories of starch granule protein distinguished according to their ease of extraction: the surface and the integral proteins.Citation20-23 Surface proteins in wheat starch granules have a low MW, ranging from 5–30 kD with the 30 kD protein as the main component. All proteins in wheat starch left after removal of the surface proteins are classified as integral protein. They have a higher MW, ranging from 30–140 kD.Citation[20], Citation[23] Since the MW of protein of surface starch granules is lower than integral protein therefore in gelation characteristic was found in the order HPW>MPW>LPW mixture that supports our experiments for RPA related to texture studies of gel in Figs. and . According to OatesCitation[22] and Prentice et al.,Citation[24] some of these integral proteins may be associated with the amylopectin, in particular, remnants of starch granules can be observed after all polysaccharide order has been lost by heating. These heat resistant “ghosts” contain mostly amylopectin and are enriched with protein, suggesting that some proteins may be associated with amylopectin.Citation[24]
Starch-protein interactions have also been discussed in relation to wheat endosperm hardness.Citation25-26 Research has indicated that the physical differences between hard and soft endosperm may lie in the adhesive strength between the starch granule and the surrounding protein matrix.Citation[27] Glenn and SaundersCitation[28] have reported that both starch-protein adhesion and distribution of air spaces influence endosperm cohesiveness and hardness. Greenwell and SchofieldCitation[29] have isolated a wheat protein at 15,000 daltons (friabilin) that appears to play a role in controlling wheat endosperm texture, and is generally used as a marker for endosperm texture.Citation[30] Surface proteins are likely to be contaminants from the endosperm storage proteins, although Lowy et al.Citation[31] identified a family of protein associated with the wheat starch granule that are different from the endosperm storage proteins. Some integral starch granule proteins are enzymes involved in starch biosynthesis, which believed to have become trapped in the growing starch granule. These latter proteins may be involved in cross-linking starch molecules to help maintain granular integrity, at least during deposition and mobilization.Citation[22]
CONCLUSION
Gel hardness and cohessiveness increased, and adhessiveness decreased with addition of sago. In mixtures MPW flour shows an anomalous characteristic in our experiments. For retrogradation study, the hardness was found to decrease in comparison to the values of fresh samples. Data from this work can be used for development of bakery products, e.g., bread, cakes, biscuits and crackers.
ACKNOWLEDGMENT
This work was supported by the RM-7 IRPA grant from the Malaysian Government.
REFERENCES
- Ingrid , A.M. , Appelqvist , Martine , R.M. and Debet. 1997 . Starch-biopolymer Interactions – A Review . Food Reviews International , 13 ( 2 ) : 163 – 224 .
- Bourne , M.C. 1982 . Food Texture and Viscosity New York : Academic Press .
- Szczesniak , A.S. and Kahn , E.L. 1971 . Effect of Mode of Rehydration on Textural Parameters of Precooked Freeze-dried Sliced Beef . Journal of Texture Studies , 2 : 280 – 295 .
- Szczesniak , A.S. 1972 . Classification of Textural Characteristics . Journal of Texture Studies , 3 : 206 – 217 .
- Peleg , M. 1983 . Application of Nonlinear Phenomenological Rheological Models to Solid Food . Journal of Texture Studies , 13 : 1 – 11 .
- Friedman , H.H. , Whitney , J.E. and Szczesniak , A.S. 1963 . The Texturometer – A New Instrument for Objective Texture Measurement . Journal of Food Science , 28 : 390 – 396 .
- Szczesniak , A.S. 1973 . “ International Methods of Texture Measurements ” . In Texture Measurements of Foods Edited by: Kramer , A. and Szczesniak , A.S. 71 – 108 . Dordrecht, , Holland : D. Reidel Publishing Co. .
- Szczesniak , A.S. 1983 . Classification of Textural Characteristics . Journal of Food Science , 28 : 385
- Szczesniak , A.S. 1963 . Objective Measurement of Food Texture . Journal of Food Science , 28 : 285 – 289 .
- Szczesniak , A.S. , Brandt , M.A. and Friedman , H.H. 1963 . Development of Standard Rating Scales for Mechanical Parameters of Texture and Correlation Between The Objective and The Sensory Methods of Texture Evaluation . Journal of Food Science , 28 : 397 – 403 .
- AACC. Approved Methods of the American Association of Cereal Chemists. American Association of Cereal Chemists, St. Paul, Minnesota 1984
- AOAC. Official Methods of Analysis 15/e. Association of Analytical Chemists: Washington, D.C. 1990
- Williams , P.C. , Kuzina , F.D. and Hlynka , I. 1970 . A rapid Colorimetric Procedure for Estimating The Amylose Content of Starches and Flours . Cereal Chemistry , 47 ( 4 ) : 411 – 421 .
- Biliaderis , C.G. 1991 . “ Non-equilibrium Phase Transitions of Aqueous Starch Systems ” . In Water Relationships in Foods Edited by: Levine , H. and Slade , L. 251 – 273 . New York : Plenum Publ. Crop. .
- Clark , A.H. , Gidley , M.J. , Richardson , R.K. and Ross-Murphy , S.B. 1989 . Rheological Studies of Aqueous Amylose Gels: The Effects of Chain Length and Concentration on Gel Modulus . Macromolecules , 22 : 346 – 351 .
- Gidley , M.J. and Bulpin , P.V. 1989 . Molecular Mechanism Underlying Amylose Aggregation and Gelation . Macromolecule , 22 : 341 – 346 .
- Collison , R. 1968 . “ Starch Retrogradation ” . In Starch and Its Derivatives Edited by: Radley , J.A. 168 – 202 . London : Chapman and Hall .
- Russell , P.L. 1987 . The Ageing of Gels From Starches of Different Amylose/Amylopectin Content Studied by Differential Scanning Calorimetry . Journal of Cereal Science , 6 : 147 – 158 .
- Matsunaga , A. and Kainuma , K. 1986 . Studies on The Retrogradation of Starch in The Starchy Foods. Part 3. Effect of The Addition of The Sucrose, Fatty Acid Esters on The Retrogradation of Corn Starch . Journal of the Japanese Society of Starch Science , 30 ( 1 ) : 106 – 113 .
- Schofield , J.D. and Greenwell , P. 1987 . “ Wheat Starch Granule Proteins and Their Technological Significance ” . In Cereal in a European Context Edited by: Morton , I.D. 407 – 420 . England : Ellis Horwood . VCH, F.R.G.
- Goldner , W.R. and Boyer , C.D. 1989 . Starch Granule-bound Proteins and Poly-Peptides: The Influence of The Waxy Mutation . Storke/Starch , 41 : 250 – 254 .
- Oates , C.G. 1990 . “ Evidence for Protein Crosslinks in Mung-bean Starch ” . In Gums and Stabilisers for the Food Industry 5 Edited by: Phllips , G.O. , Wedlock , D.J. and Williams , P.A. 203 – 206 . Oxford : IRL press: Oxford University Press .
- Rahman , S. , Kosar-Hashemi , B. , Samuel , M.S. , Hill , A. , Abbott , D. , Skerritt , J.H. , Preiss , J. , Appels , R. and Morell , M.K. 1995 . The Major Proteins of Wheat Endosperm Starch Granules . Australian Journal of Plant Physiology , 22 : 793 – 803 .
- Prentice , R.D.M. , Stark , J.R. and Gidley , M.J. 1992 . Granule Residues and “Ghosts” Remaining After Heating A-type Barley Starch Granules in Water . Carbohydrate Research , 227 : 121 – 130 .
- Glenn , G.M. and Johnston , R.K. 1992 . Mechanical Properties of Starch, Protein and Endosperm and Their Relationship to Hardness in Wheat . Food Structure , 11 ( 3 ) : 187 – 199 .
- Morris , C.F. , Greenblatt , G.A. , Bettge , A.D. and Malkawi , H.I. 1994 . Isolation and Characterization of Multiple Forms of Friabilin . Journal of Cereal Science , 20 : 167 – 174 .
- Anjum , F.M. and Walker , C.E. 1991 . Review on The Significance of Starch and Protein to Wheat Kernel Hardness . Journal of the Science of Food and Agriculture , 56 : 1 – 13 .
- Glenn , G.M. and Saunders , R.M. 1990 . Physical and Structural Properties of Wheat Endosperm Associated with Grain Texture . Cereal Chemistry , 67 ( 2 ) : 176 – 182 .
- Greenwell , P. and Schofield , J.D. 1986 . A Starch Granule Protein Associated with Endosperm Softness in Wheat . Cereal Chemistry , 63 : 379
- Bettege , A.D. , Morris , C.F. and Greenblatt , G.A. 1995 . Assessing Genotypic Softness in Single Wheat Kernel using Starch Granule-associated Friabilin as A Biochemical Marker . Euphytica , 86 : 65 – 72 .
- Lowy , G.D.A. , Sargeant , J.G. and Schofield , J.D. 1981 . Wheat Starch Granule Protein: The Isolation and Characterisation of a Salt-extractable Protein From Starch Granules . Journal of the Science of Food and Agriculture , 32 ( 4 ) : 371 – 377 .