ABSTRACT
Mathematical models of pizza baking by forced and natural convection heating methods were developed describing heat and mass transfer phenomena. The models were solved using finite difference technique and a simulation language. Moisture and temperature histories were collected at different baking conditions. The models and experimental data were used to determine mass transfer properties—moisture diffusivities of crust, tomato paste and cheese, and moisture transfer coefficient. Heat transfer coefficient was measured by minimizing the internal heat resistance.
Abbreviations:
C, | = |
Non dimensional moisture content; |
c p , | = |
Specific heat (J/(kg.K)); |
D m , | = |
Moisture diffusivity m2/s); |
h rc , | = |
Combined convection/radiation surface heat transfer coefficient (W/(m2 K)); |
h r , | = |
Radiation heat transfer coefficient (W/(m2.K)); |
H s , | = |
Product surface humidity ratio (kg water/kg dry air); |
H a , | = |
Air humidity ratio (kg water/kg dry air); K, Thermal conductivity (W/(m.K)); |
K mc , | = |
Surface mass transfer coefficient (kg water/(m2.s)); |
L, | = |
Layer thickness (m); |
L v , | = |
Latent heat of vaporisation (J/kg); |
m, | = |
Moisture content (% dry basis); |
S, | = |
Product surface; |
t, | = |
Time(s); |
T, | = |
Temperature ( °C); |
x, | = |
Linear distance (m); |
α, | = |
Thermal diffusivity (m2/s); |
Δx, | = |
Thickness increment (m); |
ρ, | = |
Density (kg/m3); |
0, | = |
Non dimensional temperature; |
ψ, | = |
Non dimensional distance |
Subscripts: | = | |
abs, | = |
Absolute temperature (K); |
a, | = |
Ambient air in oven; |
B, | = |
Bottom node; |
c, | = |
Cheese layer; |
t, | = |
Tomato paste layer; |
p, | = |
Pizza crust; |
S, | = |
Surface; |
0, | = |
Initial condition; |
1–9, | = |
Node numbers; |
i, | = |
Node increment |
INTRODUCTION
Pizza is a most favourite fast food in North America after hamburger. Common baking processes use forced air or natural convection with radiation. These processes are characterised by low humidity and high processing temperatures, which typically resulted in crust formation on the pizza surface. Forced convection baking is typically performed on foods in ovens with induced air circulation Citation[1]. The heat is transferred to the product by surrounding air. The variables influencing the process are temperature, humidity and velocity of the air. Heat radiation implies a non-contact method by means of an electromagnetic heating, e.g. infrared radiation. Heating a moist material involves water diffusion, evaporation and transfer of water from the product to the air. Latent heat is needed to evaporate the water. Close to the product surface, a thin layer of slowly moving air defines the boundary layer, which is of great importance in heat and water transport.
Little work has been published on moisture transfer properties and the modelling of pizza baking process. However, a number of papers were published on the baking of breads, biscuits and cookies. The baking industry in North America has over $30 billion annual sales Citation[2]. Recent advances in baking technology have been mainly in the areas of equipment refinements and more sophisticated control systems. Sablani et al. Citation[3] reviewed mathematical studies on heat and moisture transport during the baking process for biscuit, bread and cake. Limited results are available on the kinetics of physico-chemical changes in the baked products. Fahlout et al. Citation[4] described heat and mass transfer models of indirect fired baking tunnel oven. Good agreement was obtained between the experimental results and model predictions. Further, Zanoni et al. Citation[5] developed and experimentally validated a mathematical model describing heat and mass transfer during bread baking process. The validation showed that the model satisfactorily simulated the process. The process models are useful tools in process development, optimisation and automation.
The models for bread baking are for soft-baked products and thus are unsuitable for hard crust products such as pizza baking. Therefore, the objectives of this work were to model the coupled heat and mass transfer phenomena within a pizza during different baking processes, and to determine heat and moisture transfer properties.
PROCESS MODELLING
Heat was transferred by forced convection and radiation from the heating media (air) to the pizza surface, followed by conduction towards its geometric centre. Meanwhile, moisture diffuses outwards the pizza surface, where it is vaporised. The following assumptions were taken: the pizza was homogeneous, isotropic, and an infinite slab; initial temperature and moisture in pizza layers were uniform; ambient temperature and relative humidity (RH) were step functions of time; vaporisation of the water was restricted to the pizza's upper surface only; negligible changes in pizza dimensions during baking; properties were assumed constant during the baking; negligible effect of crust formation on physical properties; and fat transport was neglected.
One dimensional finite difference framework through an infinite slab of thickness L, constituted of 11 nodes: 5 in the crust (L p ), one in the tomato paste (L t ), one in the cheese (L c ) Fig. , one at the bottom (B), one at the surface (S), one at the interface crust-tomato and one at the interface tomato-cheese. The crust was 1 cm thick, the tomato layer 0.2 cm, and cheese layer 0.2 cm, i.e. L=L p +L t +L c =1.4 cm. Based on above assumptions, mathematical models characterising simultaneous one dimensional heat and mass transfer in a pizza during forced convection baking, and natural convection baking could be represented as from x=0 to L Equation3:
The symbols are defined in the beginning. Initial and boundary conditions were: At t=0, m p =m p0 in the crust, m t =m t0 in the tomato paste, and m c =m c0 in the cheese. T=T 0 in the crust, tomato paste and cheese. In scenario 1, at the bottom, the heat transfer was by conduction. At the top surface (cheese layer), the heat transfer was by convection and radiation, and evaporation of diffused water as illustrated in Fig. Thus at the bottom: T=T B when t>0, and at the surface (T B was equal to ambient temperature) Equation5:
The heat transfer at the interface between the crust and the tomato paste:
The heat transfer at the interface between the tomato and the cheese layer:
Figure 2. Pizza crust, tomato paste and cheese layers with the locations of nodes for finite difference equations.
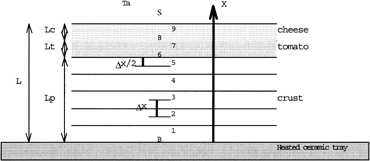
Moisture transfer at the top surface:
The moisture transfer at the interface between the crust and the tomato paste:
The moisture transfer at the interface between the tomato paste and the cheese layer:
Non Dimensional Form
A non-dimensional form of the model except for t was developed by taking the following as dimensionless variables Equation6:
Equation Equation2 provided:
Equation Equation1 provided:
Initial and boundary conditions become:
At the pizza bottom:
At the top layer:
At the interface node 6:
At the interface node 8, Eq. Equation5 provided:
At the bottom,
At the surface of cheese layer, Eq. Equation6 became:
At the interface node 6, Eq. Equation7 provided:
At the interface node 8, Eq. Equation8 provided:
Finite Difference Model
Node 9: cheese layer (c)
Node 7: tomato paste layer (t)
Node 5: surface of the pizza crust layer (p)
Nodes 4 to 2: pizza crust (p)
Node 1: pizza crust in contact with the heated tray (p)
Equation Equation17 provided:
Therefore
From equation Equation14:
From equation Equation18:
From Eqs. Equation15-16, Equation19 and Equation20:
In scenario 2, at the surface, heat transfer was by radiation and natural convection. Only Eq. Equation32 was required to be modified:
The finite difference equations of the models were solved using a simulation language ISIM Citation[7] on a microcomputer. Thermal conductivity and diffusivity for the crust were taken from the equations given by Metel et al. Citation[8] and Zanoni et al. Citation[5]. For tomato paste, thermal conductivity was given by Rahman Citation[9] as 0.546 W/(m K), and for Mozzarella cheese, a value of 0.380 W/(m K). Other properties are given in Table .
MATERIAL AND METHODS
Baking experiments were conducted to obtain temperature and moisture histories during forced and natural convection bakings for mass transfer properties determination. Pizza crusts were obtained (Famosa make, Molinaro's Fine Italian Foods Limited, Mississauga, Canada). They were of 30 cm diameter and 1 cm thickness, and ingredients used were: enriched white flour (flour, niacin, reduced iron, thiamin, riboflavin), water, vegetable oil, salt, whey powder, yeast, sugar, calcium propionate (used to preserve freshness) and L-cystein. These were kept in a refrigerator at 5°C up to 2 weeks before using. The tomato paste was in 156 mL cans (Hunt-Wesson, Tilbury, Ontario, Canada), and ingredients used were: tomato paste, water, tomatoes and salt. It was spread on the crust in 2 mm thick layer. Mozzarella cheese was in 2 mm thick slices (Sunfresh Limited, Toronto, Canada), and ingredients used were: milk solids, bacterial culture, salt, rennet and/or microbial enzyme and calcium chloride. The cheese was kept refrigerated at 5°C up to 2 weeks before using. A sharp cutter was used to cut the crust at a diameter of 17 cm. A gauge (a ring of 15 cm diameter) of 2 mm height, was used to spread the tomato paste. A circular ring was used to cut two joined cheese slices into a disk shaped slice of 15 cm. A cork borer of 0.5 cm diameter was used to make 50 random holes in the cheese slice to simulate grated cheese.
An oven (38×21×32 cm, model 7094, Toastmaster, Missouri, USA) was used, and allowed to control the desired baking: “CONVECTION BAKE” for forced convection baking and “BAKE” for natural convection baking. A temperature control dial allowed temperature selection. A flat ceramic plate (Fox Run Craftsmen, Toronto, Canada) was placed at the pizza bottom in the oven for uniform conduction heat transfer. A data acquisition system consisted of a datalogger, a CPU module and a serial interface (Labmate make, model 901, Sciemetrics Instruments Inc., Barrie, Ontario, Canada) for temperature recording at 20 s intervals. Temperature measurements at midpoint location of crust were taken with copper-constantan thermocouples (0.5 mm diameter junction, Kapton Insulated, Duowrap Parallel Duplex wire, Thermo-Electric Canada Ltd., Brampton, Canada). Each thermocouple was encased in 10 cm long Teflon tubing to reinforce them during placement in the pizza. A thermocouple alignment stand was constructed to ensure accurate placement of thermocouples within the pizza. A thermocouple was also used to monitor heating medium (air) temperature. A pizza was considered baked after 12 min.
The crust moisture content was measured indirectly by determining the total solids as AOAC #935.36 (10). Six moisture measurements were taken, i.e. after every 2 min during 12 min baking time. Two samples were taken using a cylindrical borer (4 cm diameter) at the pizza centre. Total solid in the tomato paste was determined by a microwave oven drying (10, section 42.1.09). For cheese moisture content measurement (10, # 926.08), first the sample was finely shredded into a food chopper and thoroughly mixed (10, #955.30), then dried in a vacuum oven at 13.3 kPa absolute pressure, 100°C. Details are given by AOAC Citation[10]. The moisture contents of cheese and tomato paste during baking were difficult to measure due to the problem in separating properly these materials from the pizza.
Surface heat transfer coefficients (h rc and h r ) were determined using an aluminium plate (shape and size similar to the pizza) with a thermocouple placed at the plate centre and half thickness Citation[11]. The D m values for the crust, tomato paste and cheese, and K mc were determined by minimising the root mean square of deviations (RMS) between experimental and predicted crust moisture and temperature histories. For RMS minimisation, Hooks and Jeeve algorithm was used Citation[6]. Two variables (temperature and moisture contents) varied with time, hence number of datasets were enough to determine 4 parameters. The relative humidity of air in the oven was measured with a portable digital hygrometer and thermometer (Thermo-Hygro make, Cole Palmer, Concord, Ontario, Canada), then humidity and saturation humidity ratios were determined. A velocity sensor (Davis make, Cole Palmer, Concord, Ontario, Canada) was used to measure the air velocity in the oven near the pizza surface. The three baking temperatures used were: 218°C, 204°C and 191°C. Experiments were replicated three times.
RESULTS AND DISCUSSION
Table summarises surface heat transfer coefficients (h rc ) for the pizza baking. It varies from 780 to 800 W/(m2 K) for forced convection+radiation, and from 675 to 780 W/(m2 K) for natural convection+radiation bakings. As expected, it is not so larger for forced convection compared to natural convection. This may be due to higher contribution of radiation heating compared to convective heating. The h rc increased when ambient temperature was increased from 204 to 218°C. This was mainly due to increase in air thermal conductivity with temperature. Prandtl number is generally constant at these temperatures and there is slightly reduction in Reynolds number due to increase in kinematic viscosity. Table gives the values of various properties and model parameters used in the simulation program to calculate mass transfer properties. These were taken from published results as indicated above.
Table 1. Heat Transfer Coefficients at Different Processing Conditions During Pizza Baking
Table 2. Summary of Parameters Used in the Simulation for Two Banking Processes
Table summarises the values of moisture transfer coefficient and moisture diffusivities obtained after minimising RMS values. The variations among the coefficients are due to different experimental conditions used. The RMS values provided the accuracy of the properties determined; the properties appeared to be reasonable, and described correctly the process. The mass transfer coefficient varied from 1.07 to 1.28 kg/(m2 s) for forced convection+radiation, and from 1.00 to 1.21 kg/(m2 s) for natural convection+radiation bakings. The differences are small but significant in both bakings. Moisture diffusivities of these products are not reported in the literature Citation[12]. Moisture diffusivity of cheese has not changed due to temperature or baking mode change. Since there were small holes in the cheese layer to represent the use of shredded cheese layer on the commercially prepared pizza, more water was lost from higher moisture content tomato paste than cheese. Therefore cheese moisture diffusivity is reasonable for pizza baking process. However, it can not be used in other processes.
Table 3. Moisture Diffusivities and Surface Mass Transfer Coefficient During Pizza Baking
Moisture diffusivity of pizza crust and tomato paste increased exponentially with the increase in baking temperature. The values can be represented by the Arrhenius relationship:
For pizza crust, forced convection+radiation baking | |||||
D mp=7.0582 (10−8) exp (−1890.68/T abs) | |||||
For pizza crust, natural convection+radiation baking | |||||
D mp=1.4596 (10−9) exp (−420.34/T abs) | |||||
For tomato paste, forced convection+radiation baking | |||||
D mt=9.9646 (10−10) exp (−605.93/T abs) | |||||
For tomato paste, natural convection+radiation baking | |||||
D mt=1.7738 (10−10) exp (−1212.71/T abs) |
Figs. and give the plots for the temperature changes at crust centre under different process conditions for two types of bakings. The ambient temperature was not steady during the baking processes. The rate of product temperature change increased rapidly at the start of baking due to the high ambient temperature. No heat transfer lag seemed to occur, and rate of temperature change gradually decreased as the baking progressed. When the product temperatures were above wet bulb temperatures, the rate of change declined slightly yet continued to rise towards the ambient temperature. The final temperatures recorded were 101–112°C for the crust, 102–120°C for the tomato paste and 112–127°C for the cheese at three baking temperatures. The final internal temperature of the crust was higher than 100°C for all the baking conditions. This may be due to boiling drying mode Citation[4]. The model satisfactorily simulated temperature histories of crust at various baking conditions.
Figure 3. Observed and predicted temperature histories of pizza crust at different oven temperatures during forced convection baking.
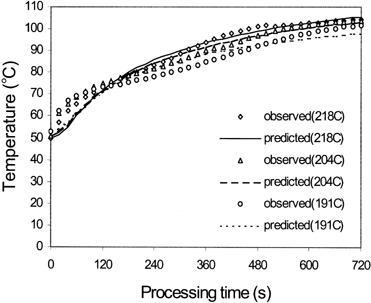
Figure 4. Observed and predicted temperature histories of pizza crust at different oven temperatures during natural convection baking.
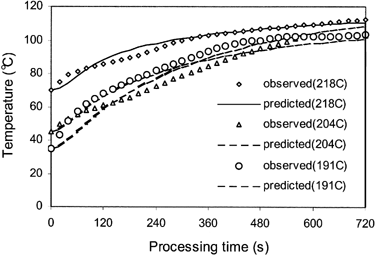
Natural convection baking (Fig. ) appeared to be gentle than the forced convection baking (Fig. ). Small differences in temperatures were observed between the layers. The rates of temperature change appeared to be lower in natural convection as well. Deviations could also be explained by variation in ambient conditions such as oven temperature, assumed constant in the simulation.
The observed and predicted crust moisture content histories are given in Fig. for forced convection heating. Similar results were obtained for natural convection baking. The crust is the most important quality criterion providing crustiness, tenderness and softness to a bite. Zanoni et al. Citation[5] assimilated bread baking to a drying operation. During both forced and natural convection bakings, the moisture content after 12 min was slightly higher than at time t=0, except for natural convection baking at 204°C. This may be due to higher tomato paste initial moisture content (373% d.b.) compared to the initial moisture of the crust (47 to 56% d.b.). Therefore, during the baking, tomato paste moisture diffused into the crust, increasing its moisture content. The crust moisture content increased at slow rate until 6 min. From 6 to 10 min, the rate of moisture increase was slightly faster, increasing the moisture content significantly. The diffusion from the tomato paste to the crust was then enhanced by the temperature increase in both layers. After 10 min, the diffusion from the crust to the air through tomato paste and cheese was more compared to the diffusion inter-layers. Baking temperature has a significant effect on the water loss of the crust. The simulated moisture content of crust is in good agreement with experiments. The natural and forced convection influenced heat and mass transfer coefficients, and thus affected temperature and moisture content histories.
Figure 5. Observed and predicted moisture histories of pizza crust at different oven temperatures during forced convection baking.
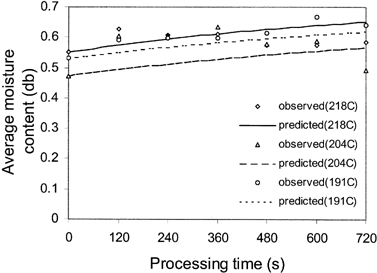
Baking by natural convection is the process used by professional “Pizzaïolo”, in a restaurant for example. Though at an industrial scale, forced convection baking would be preferred because it is faster and more efficient. More work is needed on the effect of process conditions on the pizza quality.
CONCLUSIONS
Heat transfer coefficients were determined for pizza during two baking types. The comparison between experimental and simulated moisture and temperature histories provided mass transfer properties (mass transfer coefficient and moisture diffusivities of crust, tomato paste and cheese) during two types of pizza baking processes. Heat and mass transfer properties accuracy is better as at 218°C baking, the temperatures were predicted within ±1.8°C and moisture content within ±0.042 db. For all 6 baking conditions, moisture content of the crust was predicted within ±0.052 db and temperature within ±4.8°C.
Acknowledgments
The research was supported by Natural Sciences and Engineering Research Council of Canada. The assistance provided in the simulation and experimental work by Ms. Robin Williams and Dr. J. Zhang is acknowledged and appreciated.
REFERENCES
- Hallstrom , B. , Skjoldebrand , C. and Tagardh , C. 1988 . Heat Transfer and food Products 263 New Tork : Elsevier .
- 1999 . EPRI. Advances and New Technology–Baked Food Industry . Food Industry Currents , 3 ( 2 ) : 1 – 8 .
- Sablani , S.S. , Marcotte> , M. , Baik , O.D. and Castaigne , F. 1998 . Modelling of Simultaneous Heat and Water Transport in the Baking Process . Food Sci. & Technol. , 31 : 201 – 209 .
- Fahloul , D. , Trystram> , G. , Duquenoy , A. and Barbotteau , I. 1994 . Modelling Heat and Mass Transfer in Band Oven Biscuit Baking . Food Sci. & Technol. , 27 : 119 – 124 .
- Zanoni , B. , Pieriucci , S. and Peri , C. 1994 . Study of the Bread Making Process-II. Mathematical Modelling . J. Food Engg. , 23 : 321 – 336 .
- Mittal , G.S. and Blaisdell , J.L. 1982 . Moisture Mobility in Frankfurter During Thermal Processing Analysis of Moisture Profile . J. Food Process & Preservation , 6 : 111 – 126 .
- Dunn , I.J. , Heinzle , J. and Prenosil , J.E. 1992 . Biological Reaction Engineering 438 New Tork : VCH .
- Metel , S.N. , Mikrukov , V.V. and Kasparov , M.N. 1986 . Thermophysical Characteristics of Yeast Dough. Izvestiga Vysshikh Uchebnnykh Zavedenii . Pishschevaya Teknologiya , 4 : 107 – 108 .
- Rahman , S. 1995 . Food Properties Handbook 500 Boca Raton : CRC Press .
- AOAC. Official Methods of Analysis; Association of Analytic Chemists: Washington, DC, 1996; 762.
- Rizvi , S.S.H. and Mittal , G.S. 1992 . Experimental Methods in Food Engineering 289 New York : Van Nostrand Rheinhold .
- Mittal , G.S. 1999 . Mass Diffusivity of Food Products . Food Review Int , 15 ( 1 ) : 19 – 66 .