Abstract
Green coffee was subjected to thermal study of the reactions evolved during the roasting process. Specific heat, heats of reactions, pressure drop, and permeability of the packed coffee powder were obtained from the measurements. The sample was dried, therefore the thermograms showed no evidence of occluded water present in the coffee. The results showed predominant endothermic reaction in the range of 100 and 245°C mainly due to the formation of phenolic and nitrogen containing heterocyclic compounds. The reactions at temperatures above 245°C exhibited a double trough exotherm related to fracturing of the matrix and formation of cracks as a result of the decomposition of the lignin. The largest pressure build up and consequently lowest permeability was observed in this temperature range. The decomposition reactions were completed at 430°C.
Introduction
Over 100 volatile products are evolved during roasting of green coffee beans in a range of complex reactions and reformations. The distinguished aroma and flavor of the coffee is a thermochemical process. When heated, the beans are undergoing pyrolysis and decomposition of the polymeric lignocellulose matrix forming typical volatile products. Some of the gaseous products contain volatile organic compounds, nitrous oxides, and the oxides of carbon.
During roasting the beans significantly alter their physical properties increasing the volume up to almost a double of the original size, losing between 15 and 25% of the weight and continuously changing the color. Essentially, the desired aroma and beverage flavor of the brew is determined by the degree of the roasting, which depends on the final temperature of the beans and roasting time. The degree of roasting is usually monitored through visual means by the ground bean light reflectance.Citation[1] However, visual determination of the degree of roasting has been argued by Dutra et al.Citation[2] as beans having different aromas could have the same reflectance, depending on the chemical composition. Gas analysis techniques, such as gas chromatography have been proposed to aid in the studying of the roasting degree and flavor of the coffee beans. Similarly, a mass spectroscopyCitation[3] and 1H NMRCitation[4] have also been applied in the study of the volatile products developed during roasting.
A range of designs of coffee roasters are currently in use some consisting of rotating cylinder with internal baffles for mixing and tumbling beans, fluidized bed roasters and roasters using spouted bed technology.Citation[5] In general, the coffee beans are heated for a period of approximately 5–15 min during which the temperature of the roasted coffee should not exceed the maximum of 250°C at the end of the process. New designs of the roasters have mainly been applied to shorten the roasting time. Only recently, the polluting effect of the volatile emissions during the roasting has been considered as a driving point in the considerations for designs of the new roasters.Citation[6]
While there is a considerable data provided for the gas composition measurements and its relation with the roasting degree, the information on the thermal reactions evolved during the roasting process is scarce. This work is concerned with the thermal investigation of the coffee beans, measurement of the heats of reactions during roasting and specific heat of the beans. As the information available for the specific heat of the coffee beans is limited to temperatures below 150°C,Citation[6] Citation[7] which is considerably lower than the temperatures achieved in the conventional roasting, the necessity for these type of measurements arouse when optimization of the roasting process was required. Furthermore, the data on the thermal properties of coffee beans are essential for the future designs and operations of the new roasters. The reactions developed during the roasting could, on the other hand, provide an additional input in determination of the roasting degree.
Materials and Methods
Sample
Green Arabica coffee beans were selected for this study. The samples were finely grinded and dried under vacuum at 80°C for the period of 2 h. Moisture is one of the major constituents in the coffee beans, however its presence is highly variable and dependent on the ambient temperature conditions. To ensure that no overlap occurs in the reactions between the moisture evaporation and the decomposition of the stronger chemical bonds in the coffee, it was decided to remove the occluded water from the material. Grinded coffee beans weighing 2 g were packed inside a silica tube as shown in Fig. .
Thermal Analysis
A novel computer aided thermal analysis technique was employed for the purpose of this study. The technique has been detailed elsewhere,Citation[8] and here it is only briefly described. The sample was packed to a density of 755 kg/m3 inside a 10.6 mm ID quarts sample tube and insulated on the sides with alumina ceramics. The quarts glass tube was then covered with carbon soot on the outer surface to ensure uniform emissivity is achieved throughout the glass. The glass tube with the packed sample was inserted into the center of a graphite heating element, as shown in Fig. . The system was placed inside an infrared furnace and both the heating element and the sample were kept under inert atmosphere with separate flows of argon gas. Using the flow of the carrier gas, a positive pressure was maintained across the sample to ensure that the gases generated by the decomposition process were promptly removed from the heated zone.
Three chromel–alumel thermocouples were used during the measurements, one embedded inside the graphite tube and used to control the heating rate of the furnace at 20°C/min and two thermocouples placed on the surface and in the center of the sample. The heating was carried out until the graphite temperature reached 500°C, which was enough for completion of the decomposition of the coffee. The temperature logging was achieved at 1 Hz logging rate and the data were stored in a computer.
The thermal analysis in this work was performed with the stored temperature data by a numerical approximation of the heat conduction Eq. (Equation1) based on the inverse thermal modeling technique:
The specific heat estimated by Eq. (Equation3) has apparent values, which means that the heats evolved during decomposition (ΔH) of the heated sample are included in the specific heat data (Cp = Cp * + ΔH/ΔT). The thermal analysis technique was previously tested on a range of calorimetric calibration materials and the accuracy of the method was found to be approximately in the order of ±2%.Citation[8]
Pressure Drop Measurements
Pressure drop measurements were also carried out with an absolute pressure cell connected on the inlet of the sample tube. Pressure drop is an additional indicator of the thermal changes that are occurring in the heated sample material which are occurring either due to the physical changes in the volume or pressure development during volatile release. The permeability η of the packed coffee powder was estimated with the measured data according to the Darcy's rule expressed by the Eq. (Equation4):
Results
Figure shows the time temperature history of the heated coffee powder from this measurement. When the temperature of the coffee exceeds 220–250°C generally over-roasting occurs. In a conventional roasting process it is unlikely the coffee beans would exceed these temperatures, however the current work aimed to depict the reactions during the entire decomposition range and the heating was performed until the decomposition was entirely completed. The result on Fig. shows that while the surrounding graphite heating element was heated at a constant heating rate, the coffee established deviations during the temperature rise due to the decomposition and chemical reactions. The changes in the temperature rise affected the surface heat flux Q, which was estimated according to Eq. (Equation2) and is shown in Fig. . The surface heat flux achieved the maximum of 1.8 kW/m2 at 200°C decreasing rapidly to 0.75 kW/m2 at approximately 400°C.
The apparent specific heat was estimated numerically from the surface heat flux and measured temperatures. The specific heat, shown in Fig. , was around 1400 J/kgK at room temperature with a steady rise up until 100°C after which a sharp increase in the specific heat was evident. An endothermic reaction having a peak at 120°C was apparent as a contributor to the increase in the specific heat. This event was followed by two clear exothermic troughs at 340 and 400°C. The reactions were found to be completed at 430°C with a steady specific heat at approximately 1450 J/kgK for temperatures beyond this point.
Figure 3 (a) Calculated transient surface heat flux of the roasted coffee beans. (b) Apparent specific heat of coffee bean powder.
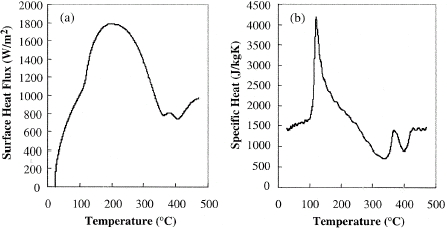
To estimate the heats of reactions a straight line baseline connecting the start and the end point of the reactions in the specific heat data was assumed. The reactions, shown in Fig. , were extracted by subtracting the apparent specific heat data from the assumed straight line baseline. Clearly, three regions were determined where the first endothermic reaction was in the range of 100–245°C. The integral underneath determines the amount of heat consumed for the reaction to occur and was estimated at 100 kJ/kg. The second region was exothermic and ranged between 245 and 370°C, followed by the second exothermic reaction which was completed at 430°C. The estimated heats were −60 kJ/kg for the first exothermic reaction and −20 kJ/kg for the second.
Figure 4 Rate of change in heat of reactions with respect to temperature determined by subtracting the specific heat from the baseline.
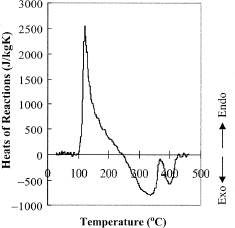
Measured heats of reactions were used to determine the activation energies of the reactions. The activation energies and kinetics of degradation of food have been a subject of interest for a long period and were recently applied in determination of color change and degradation of various foods.Citation[10] Citation[11] In the current work, similar approach was adopted where the thermal changes in coffee degradation were assumed to occur with a series of first order kinetics mechanisms. The change in heat of reactions was at first integrated in respect with time and plotted cumulatively as a degree of reaction. The degree was set to 0 before the reaction was found to commence and 1 when it finished. A first order reaction kinetics was assumed with a rate constant varying with the temperature according to the Arrhenius relationship:
The activation energy E for each of the reaction regions can be estimated from the heats of reactions data by taking the logarithm of the Eq. (Equation5):
Finally, the left side of Eq. (Equation6) was plotted against 1/T, as shown in Fig. . A linear trendline was fitted through each curve and for the three reaction regions the estimated activation energy was 70 kJ/mol for the endothermic region and 90 and 315 kJ/mol respectively for the two exothermic reactions. Summary of the temperature regions of decomposition of the selected coffee sample with the corresponding heats and activation energies are summarized in Table .
Table 1 Temperature region of thermal decomposition of green coffee with corresponding heats of decomposition and activation energies
Figure 5 Calculation procedure for activation energies based on the measured rate of change in heat of reaction.
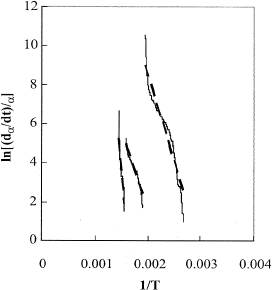
Liberation of the volatiles and changes in the volume of the particles consequently alter the pressure across the bed of the packed coffee particles. In this work the pressure drop across the sample was measured with an absolute pressure cell and is shown in Fig. . The pressure drop was caused by the resistance to the flow of the gas flown across the bed. The maximum pressure of 10 kPa was achieved at 300°C. The permeability of the coffee was calculated according to Eq. (Equation4) and is plotted against the temperature in Fig. . The permeability was at 10−11.5 m2 at room temperature and the minimum degree of the permeability was at around 10−13 m2 at 300°C.
Discussion
The reactions developed during the roasting process are clearly consequent to the chemical reactions and thermal decomposition of the compounds present in the green coffee. The product volatiles consist of a vast multiplicity of compounds. The major constituents of the green coffee beans are polysaccharides, lipids, and proteins. Free amino acids, chlorogenic acids, trigonelline, and sugars are also found in the green coffee, which are considered as an essential source of the coffee aroma.Citation[12] In the conventional roasting, the green coffee beans are heated under air at constant temperature. Although the surrounding of the coffee beans is air, the conditions are pyrolytic due to the large amounts of liberated carbon dioxide. The carbon dioxide is evolved as a product of decarboxylation of the organic acids and prevents from oxidative degradation of the formed volatiles. This is of significant importance as it provides protection to the flavors developed during the roasting.Citation[3] The separation methods for investigating the coffee aroma were detailed by SilwarCitation[13] and the chemical background of the coffee flavor was extensively reviewed by Grosch.Citation[14] It was confirmed that the key aroma compound of the coffee is 2-furfurylthiol. Other contributors are 4-hydroxy-2,5-dimethyl-2(2H)-furanone, 2-ethyl-3,5-dimethyl-pyrazine, 2-3-methylbutanal, diacetyl, guaiacol, and other volatiles.
There have been reports of four regions of decomposition of the green coffee beans: dehydration, hydrolysis, desmolysis, and catalysis.Citation[15] The endothermic dehydration reaction depends on the amount of free moisture present in the beans. The increase of specific heat due to the moisture content and temperature has been well documented for various cases of binary aqueous solutions using calorimetric techniques.Citation[16] In the case of green coffee, this reaction is also well known and was excluded from the results shown in the current work by drying the beans prior to the measurements to avoid overlap with the following decomposition reaction.
The decomposition started at 100°C with a significant endothermic reaction apparent throughout the temperature range where roasting is normally performed. The same reaction was reported elsewhereCitation[2] by observing a drop in the temperature during the roasting process as a consequence of the endothermic energy consumption of the pyrolytic reactions. When calculating the activation energy, this reaction was assumed to be of a single order. However, it comprises of complex matrix of structural bond rupture reactions and chemical reformations, so the reaction is more than likely of a multiple order. Major contributor to the thermal endothermicity is formation of phenols. The chlorogenic acid (CGA) commonly found in the green coffee firstly decomposes to caffeic and chianaic acids,Citation[17] which further form phenolic compounds such as phenol, dihydroxybenzenes, guaiacol, 4-vinylguaiacol, and 4-ethylguaiacol.Citation[3] Depending on the type of the green coffee, it could contain between 6 and 10% CGA on a dry matter basis. Decomposition of CGA occurs with 8–10% being lost for every 1% loss of dry matter.Citation[18] The CGA decomposition is not finalized during the conventional roasting as substantial amounts are still commonly found in the brews and commercial coffee powders.
The second reaction which occurred during the same temperature region is the formation of nitrogen containing heterocyclic compounds such as indol and caffeine. Nitrous oxides are found in the off gas measurements causing environmental concerns. Caffeine, which evolves in a liquid state can be dragged off by the water vapour,Citation[2] however a certain quantity is retained by absorption. Water, formed from chemical decomposition and secondary reactions, is also absorbed by the porous coffee beans.
The thermal decomposition reaction was found to shift towards an exotherm at 245°C. There was a substantial resistance to the flow of the gas resulting in the pressure build up across the heated bed. This is consequent to the release of high molecular weight liquid phase products. Oils and flavor related substances are unstable and deteriorate at this point. The lignin, which is a highly polymerized aromatic, also decomposes at higher temperatures resulting in a severe damage to the cell walls of the matrix.Citation[19] Darker roast and cracks in the beans occur due to the fracturing of the matrix. Therefore, roasting at temperatures where reactions shift towards the exotherm should be avoided. The exothermicity is clearly marking the over-roasting temperature region. Some catalytic effect of the minerals on the pyrolytic reactions is also expected to occur at larger temperatures.Citation[15]
Conclusions
From the current work the following conclusions have been driven out:
• | The specific heat of green coffee was found to be around 1400 J/kgK at room temperature. | ||||
• | The thermal decomposition of dried green coffee was endothermic in the temperature range of 100–245°C with a peak at 120°C. This reaction was estimated at 100 kJ/kg having activation energy of 70 kJ/mol. | ||||
• | The endothermicity was related to the formation of phenols by decomposition of chlorogenic acid and to the formation of nitrogen containing heterocyclic compounds. | ||||
• | In the temperature range of 245 and 430°C, the decomposition was exothermic undergoing two reaction modes with heats estimated at −60 and −20 kJ/kg and with activation energies of approximately 90 and 315 kJ/mol. This region was related to the formation of higher molecular weight liquid phase products resulting in extensive pressure build-up and decrease in the permeability of the coffee during roasting. This region was an indicator of the over-roasting temperature range. |
Acknowledgments
The authors would like to acknowledge the input from Breckenridge Coffee Roasters, USA for the initiation and support of this research.
References
- Purdon , M.P. and McCamey , D.A. 1987 . Use of a 5-caffeoylquinic acid/caffeine ratio to monitor the coffee roasting process . Journal of Food Science , 52 ( 6 ) : 1680 – 1683 .
- Dutra , E.R. , Oliveira , L.S. , Franca , A.S. , Ferraz , V.P. and Afonso , R.J.C.F. 2001 . A preliminary study on the feasibility of using the composition of coffee roasting exhaust gas for the determination of the degree of roast . Journal of Food Engineering , 47 ( 3 ) : 241 – 246 .
- Zimmermann , R. , Dorfner , R. and Kettrup , A. 1999 . Direct analysis of products from plant material pyrolysis . Journal of Analytical and Applied Pyrolysis , 49 ( 1–2 ) : 257 – 266 .
- Bosco , M. , Toffanin , R. , De Palo , D. , Zatti , L. and Segre , A. 1999 . High-reslolution 1H NMR investigation of coffee . Journal of Science of Food and Agriculture , 79 ( 6 ) : 869 – 878 .
- Nagaraju , V.D. , Murthy , C.T. , Ramalakshmi , K. and Srinavasa Rao , P.N. 1997 . Studies of coffee beans in a spouted bed . Journal of Food Engineering , 31 ( 2 ) : 263 – 270 .
- Singh , P.C. , Singh , R.K. , Bhamidipati , S. , Singh , S.N. and Barone , P. 1996 . Thermophysical properties of fresh and roasted coffee powders . Journal of Food Process Engineering , 20 ( 1 ) : 31 – 50 .
- Telis-Romero , J. , Gabas , A.L. , Polizelli , M.A. and Telis , V.R.N. 2000 . Temperature and water content influence on thermophysical properties of coffee extract . International Journal of Food Properties , 3 ( 3 ) : 375 – 384 .
- Strezov , V. , Lucas , J.A. and Strezov , L. 2003 . Computer aided thermal analysis . Journal of Thermal Analysis and Calorimetry , 72 ( 3 ) : 907 – 918 .
- Beck , J.V. , Blackwell , B. and St Clair , C.R. 1985 . Inverse Heat Conduction—Ill Posed Problems New York : John Willey .
- Ahmed , J. , Shivhare , U.S. and Kaur , M. 2002 . Thermal color degradation kinetics of mango puree . International Journal of Food Properties , 5 ( 2 ) : 359 – 366 .
- Vélez-Ruiz , J. and Sosa-Morales , M. 2003 . Evaluation of physical properties of dough of donuts during deep-fat frying at different temperatures . International Journal of Food Properties , 6 ( 2 ) : 341 – 353 .
- De Maria , C.A.B. , Trugo , L.C. , Moreira , R.F.A. and Werneck , C.C. 1994 . Composition of green coffee fractions and their contribution to the volatile profile formed during roasting . Food Chemistry , 50 ( 2 ) : 141 – 145 .
- Silwar , R. 1986 . Analytical techniques for the investigation of coffee aroma . TrAC Trends in Analytical Chemistry , 5 ( 3 ) : 78 – 81 .
- Grosch , W. 1998 . Flavour of coffee . Nahrung , 42 ( 2 ) : 344 – 350 .
- Coste , R. 1992 . Coffee, the Plant and the Product 231 – 234 . London : The MacMillan Press .
- Darros-Barbosa , R. , Balaban , M.O. and Teixeira , A.A. 2003 . Temperature and concentration dependence of heat capacity of model aqueous solutions . International Journal of Food Properties , 6 ( 2 ) : 239 – 258 .
- Baltes , W. 1985 . Application of pyrolytic methods in food chemistry . Journal of Analytical and Applied Pyrolysis , 8 : 533 – 545 .
- Clifford , M.N. 1999 . Chlorogenic acids and other cinnamates—nature, occurrence and dietary burden . Journal of the Science of Food and Agriculture , 79 ( 3 ) : 362 – 372 .
- Staub , C. 1995 . “ Basic chemical reactions occurring in the roasting process ” . In SCAA Roast Color Classification System Reno : Agtron Inc. .