Abstract
Actomyosin isolated from fish varied from other vertebrate actomyosin. Ca2+ATPase activity of fish actomyosin showed the maximum activity at pH 9.0. The enzyme activity decreased with increase in temperature. Polyhydric alcohols, such as sorbitol and mannitol, inhibited the Ca2+ATPase activity of fish actomyosin. Mannitol was more potent inhibitor of the activity than sorbitol. An amount of 0.2M of mannitol and 0.3M of sorbitol in solution completely inhibited the Ca2+ATPase activity. The reduction in the intrinsic fluorescence intensity of actomyosin was insignificant in presence of these polyols. The inhibition of actomyosin Ca2+ATPase activity is associated with reduction in the free SH content. Secondary structure of actomyosin recorded a marginal increase in the α-helicity in presence of these polyols. These polyols reduced the nucleotide degrading property of actomyosin without alteration in the structure of molecules.
INTRODUCTION
Myosin is the most abundant molecular motor, which plays both a structural and enzymatic role in both muscle contraction and intracellular motility.[Citation1] Myosin molecule is a highly asymmetric hexamer. Each myosin molecule consists of two globular head regions and a rodlike tail portion that is a coiled of α-helices. The properties of myosin depend on the source of origin. Even though the structure of fish myosin is similar to other mammalian myosin, it is more unstable than the mammalian. Studies on the Mg2+ ATPase of actomyosin and Ca2+ ATPase of myofibrils suggest that the stability of fish myosin is species specific and associated with the temperature of its habitat.[Citation2] In myosin, the chemical step occurs at a point different in the contractile cycle from the energy transduction event.[Citation3] Fish myosin are generally unstable and easily and rapidly form aggregates with concomitant decrease of ATPase activity.[Citation4] Heat-induced formation of myosin oligomers precedes inactivation of myosin ATPase.[Citation5] The nucleotide-binding site is located in a pocket at the interface of the 25kD N-terminal and the central 50kD tryptic fragment.[Citation3]
Sugars and sugar alcohols are used as cryoprotective agents for fish myosin during storage. Sugars added to myosin filament suspensions or myofibrils form hydrogen bonds with some amino acids residues, including charged amino acids of myosin rods, via hydroxyl groups of sugars, and sorbitol was able to disassemble the myosin filaments. The osmotic nature of sugar solutions, is one of the several polyldydric qualities of alcohols. This quality does not only affects the function of sugar alcohols as food, but it also participates in numerous intracellular events and has medical applications. Because of their polyol nature, some sugar alcohols with the right configuration (e.g., D-mannitol) can act as free radical scavengers in biological and experimental systems. Sorbitol promoted solubilization of myofibrillar proteins at physiological concentrations of NaCl. Here we are elucidating one of the lesser known property of sugar alcohol to myosin: the inhibition of calcium activated ATPase activity of actomyosin. ATPase activity of fish actomyosin has been characterized as a function of pH and temperatures. The nature of inactivation has been followed by kinetic studies of the enzyme and structural changes of actomyosin in presence of two prominent sugar alcohols, such as sorbitol and mannitol.
MATERIALS AND METHODS
Preparation of Actomyosin
Actomyosin from a pelagic, planktivorus, shoaling fish, the oil sardine (Sardinella longiceps), was prepared according to a modified procedure of Margossian and Lowey.[Citation6] The procedure has been adapted to the step of actomyosin in the above procedure, which is meant for rabbit skeletal myosin. Also we have used a buffer system of potassium phosphate and potassium chloride and used a potassium phosphate buffer instead of EDTA solution to remove the low molecular weight proteins. The detailed procedure is described below. Fresh oil sardines were brought to the lab in iced conditions and used for actomyosin preparation. Separated meat was washed with potassium phosphate buffer (pH 6.5; 0.15M) to remove all the low molecular weight proteins. The actomyosin was isolated from washed meat using the potassium phosphate buffer (pH 6.5; 0.15M) containing 0.3M KCl, 1×10−3M ATP; 2.5×10−2M EDTA and 5×10−3M MgCl2.
The slurry was then centrifuged at 10,000×g for 25 minutes. The supernatant was diluted with distilled water three times and kept for 3 hours to accomplish complete precipitation and again centrifuged. The pellets were dissolved in a potassium phosphate buffer (pH 6.5; 0.15M) containing 1.0M KCl and kept for 30 minutes. The solubilized proteins were diluted 10 times, and the pellets were collected after centrifuging at 7000 ×g for 15 min. All the steps in extraction were carried out at 4°C. The isolated actomyosin was kept under refrigerated conditions and used for experiments within 48 hours of isolation.
Ca2+ ATPase Activity
Actomyosin was solubilized in respective buffers containing 0.5M KCl (glycine-NaOH pH 9.0 and Tris-HCl pH 7.0) in the ratio of 1:10. The insoluble fraction was removed by centrifuging the solution at 7000 ¥ g for 15 minutes in an inverter high speed refrigerated centrifuge (Kuboto 6930, Kuboto corporation, Tokyo). Protein content of the supernatant was estimated by the method of Bradford.[Citation7] ATPase activity of the actomyosin was determined using the method of Perry [Citation8] at different temperatures and pH. Different concentrations of mannitol and sorbitol were taken in the reaction mixture (0.2 ml of actomyosin solution, 0.2 ml of 0.1M CaCl2. The solution was made up to 2ml with buffers). Reaction started with the addition of 0.05ml of 50 mM ATP and incubated for 15 minutes in a shaking water bath (Grant OLS 200,Grant Instruments [Cambridge] Ltd, England) and stopped by the addition of 1 ml of 15% TCA. Inorganic phosphorous released during the reaction was estimated in the filtrate using the method of Taussky and Shorr.[Citation9] Quartz double distilled water was used throughout the study.
Intrinsic Fluorescence Emission Spectra
Intrinsic fluorescence emission spectra of solubilized actomyosin in presence of various concentrations of sorbitol and mannitol was recorded for an emission range of 300‐400nm after exciting with 280nm using a specrtofluorimeter (Shimadzu RF-500, Japan). Emission spectra recorded after 10s of excitation of the proteins. Excitation and emission slits were kept at 5nm. The protein concentration was adjusted to an absorbance of 0.1 at 280 nm (approximate protein concentration of 0.09-0.12 mg/ml) before the experiment. The fluorescence spectra was recorded at a constant temperature of 25˚C (with the help of a circulating water bath, which is connected to instrument).
ANS Hydrophobicity
Hydrophobicity of fish actomyosin in the presence of various concentrations of sorbitol and mannitol was determined with the help of the fluorescent dye ANS according to the method of Kato and Nakai.[Citation10] A stock solution of ANS (8×10−3 M) was prepared in the respective buffers at 25˚C. To 4 ml of protein solutions of concentration in the range of 0.001 to 0.025%, 20µL of ANS solution was added, and the emission intensity recorded at wave length of 470nm after exciting at 390nm. Fluorescence intensity was recorded 30sec after excitation. The temperature of the solutions was kept constant with the help of a circulation water bath attached to the instrument. A plot of the fluorescence intensity versus the protein concentration was recorded, and the initial slope of the plot (S0) was taken as hydophobic index of protein (Kato and Nakai).[Citation10]
Circular Dichroic Spectra
The secondary structural change in fish actomyosin was determined by a circular dichroic spectra using a Jasco 810C spectropolarimeter (Jasco, Tokyo, Japan). Solubilized actomyosin in presence of different concentrations of sorbitol and mannitol was used for the determination of CD spectra. The far UV CD spectra of proteins were recorded in presence of sorbitol and mannitol using quartz cuvettes of 0.1cm path length with protein solutions of concentration 0.0010-0.0016 M. Dry nitrogen gas was purged into the instrument before and during use. Mean residue ellipticity values were calculated using a value of 115 for the mean residue weight. The secondary structural analysis was done with the help of programme in the instrument.[Citation11]
Free Thiol Groups Estimation
The free SH content of fish actomyosin was determined by the method of Beveridge et al.[Citation12] using Ellman's reagent. Samples were incubated for 15 min at 25˚C with 1mM of Ellaman's reagent in respective buffers. Absorbance was recorded at 412nm with appropriate blanks. The total number of thiol groups was calculated using the molar extinction coefficient of 13500M−1 cm−1 at 412nm for the thiobenzoate that was stochiometrically released by the reaction of Ellman's reagent with the sulfhydryl groups.
RESULTS AND DISCUSSION
The Ca2+ activated ATPase activity of actomyosin as a function of pH in the range of 5.5 to 10.0 is given in . The data indicates two pH values in which there is optimum activity: pH 7.0 and pH 9.0. However, the activity at pH 9.0 is nearly twice the activity at pH 7.0. The results in the literature are very similar to this in terms of overall bimodal peak of the profile. Myosin ATPase activity shows maximum activity at pH 9.0; it shows one additional peak at neutral pH in presence of actin.[Citation8] The rate of ATP hydrolysis varies at different pH values, as shown in . In the alkaline pH, the slope of the curve, i.e., the increment in activity with increase in time, is more than the activity at neutral pH. In both cases, the activity reached maximum values after 15 min. There is a drastic reduction in the activity due to increase in temperature (, ). Activity of ATPase decreased with an increase in temperature. It reached near zero values at a temperature of 45ºC. The rate of reduction of activity due to temperature followed a linear equation. Conformational changes in the myosin head during heating might have brought down the activity of the enzyme. Heat-induced denaturation of fish myosin has been reported with three transitions occurring at 36.9ºC, 53.2ºC and 71.8ºC.[Citation13] The loss of activity, therefore, at higher temperatures is probably due to the aggregation of myosin molecules. Changes in the conformation of myosin at a temperature around 40ºC might have been responsible for the loss of Ca2+ activated ATPase activity of myosin.[Citation14] Aggregations of tail portion of the myosin molecules are also a major possibility at this temperature resulting in loss of activity.[Citation15] Thermal stability of myosin is low compared to actomyosin and thermal activation of Mg2+ ATPase activity is dependant on the tail portion of myosin. Actomyosin ATPase is affected by LC2 removal, but large aggregates may lower the activity as a result of the steric hindrance effect.
Table 1 Ca2+ ATPase activity of fish actomyosin in presence of sorbitol at different pH values of 7.0 and 9.0 as a function of temperature. (Activity expressed as µM Pi/mg protein/min)
Table 2 Ca2+ ATPase activity of fish actomyosin in presence of sorbitol at different pH values of 7.0 and 9.0 as a function of temperature. (Activity expressed as µM Pi/mg protein/min)
Figure 1 Ca2+ activated ATPase activity of fish actomyosin as a function of different pH conditions.
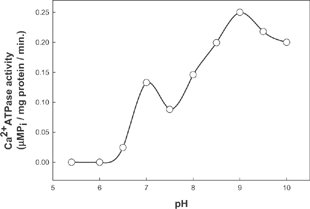
Figure 2 Time kinetics of Ca2+ activated ATPase activity of fish actomyosin at pH 9.0 and pH 7.0

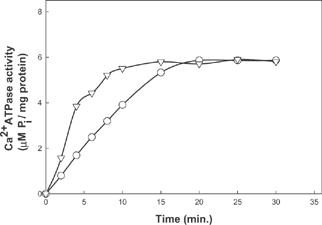
Ca2+ATPase activity of fish actomyosin increased with increase in substrate concentration (). The activity profile shows different patterns with increase in substrate concentration at different pH values. Activity reached the maximum value at a concentration of 600µM at pH 9.0. A further increase beyond 1000µM resulted in a decreased activity. The activity profile shows a steady increase even after 1000 µM at pH 7.0 (). ATPase activity reached a maximum value in the presence of 1000µM ATP. The difference in activity and ATP requirement perhaps have contributed to the change in characteristic variation of myosin from various sources.
Figure 3 Ca2+ ATPase activity of fish actomyosin at different pH conditions as function of substrate concentration

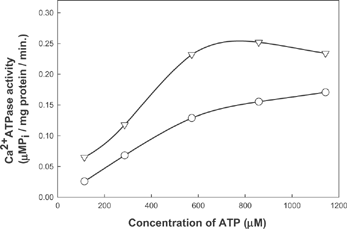
Activity of Ca2+ activated ATPase decreased in presence of both sorbitol and mannitol (,). The effect of mannitol on the activity was more pronounced than that of sorbitol. Mannitol showed higher inhibitory effect than sorbitol at both of these pH values. Double reciprocal plots of Ca2+ATPase activity indicates a change in Km value with respect to an increase in inhibitor concentration (). This may indicate uncompetitive nature of inhibition by polyhydric alcohols. The nature of inhibition was evident both for sorbitol and mannitol at the pH values where the activity was monitored. The inhibitory effect is seen explicitly in the case of actomyosin from rabbit muscle and ATPase from porcine cerebral cortex also (Unpublished data).
Figure 4 Double reciprocal plot of inhibition of actomyosin ATPase by A) sorbitol B) mannitol at pH 7.0



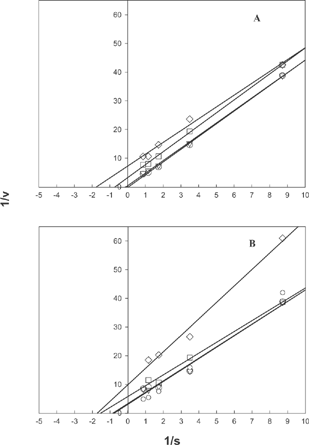
The nucleotide binding site of myosin is located in a pocket at the interface of the 24kD N-terminal and the central 50-kD tryptic fragments; the nucleotide lies parallel to the plane of a seven stranded β-sheet, where the α-and β-phosphates interact with the P-loop from the 50-kDa segment.[Citation3] There are two active SH groups present in the two α-helices lying under the nucleotide binding pocket that are more reactive than other 11 SH groups [Citation1]. The free SH content of fish actomyosin decreased with increased concentration of polyhydric alcohols (). Chaussepied et al.[Citation16] located the inter thiol cross-link joining the 50 and 20 kD domains of skeletal S1 heavy chain. The loss of Ca2+ATPase activity accompanying formation of the intramolecular bridge suggested the involvement of thiol SH2 Residue 697 of 20kD fragment.[Citation17] It was suggested that destruction of sulfhydryl groups located in subfragment-1 of myosin could cause loss of Ca2+ATPase activity. There appears to be a close relationship between Ca2+ATPase activity and sulfhydryl content in fresh beef heart surimi but not for frozen stored surimi.[Citation18]
Table 3 Free SH content of fish actomyosin in presence of different concentrations of co-solvents at pH 7.0 (Tris-HCl buffer) (Free SH content expressed as µM SH/ g of protein)
Intrinsic fluorescence emission spectra of fish actomyosin in presence of sorbitol and mannitol in the concentration range of 0-10% (w/v) are shown in A, B. In both the cases, one can see marginal decrease in fluorescence intensity and in case of mannitol (B) there is almost no change in intensity of the peak or the nature of the spectra. However, in both cases, the fluorescence emission maximum is 336nm. This indicates that more tryptophan residues are in the hydrophobic environment, perhaps in the interior of proteins, than in the bulk solvent. Even if comparatively much less is exposed to the bulk system, there is no change. Neither red nor blue shifts occurred in fluorescence emission maximum in presence of different concentrations of sorbitol and mannitol. It is fair enough to conclude that these solvents did not significantly alter the structures in the protein, as judged by the fluorescence spectra. This is a fully established and sensitive probe used in protein chemistry for understanding the structural stability of tryptophanyl peptides and proteins using these fluorescent emission spectra.
Figure 5 Intrinsic fluorescence spectra of fish actomyosin at pH 7.0 in presence of A) sorbitol; B) mannitol





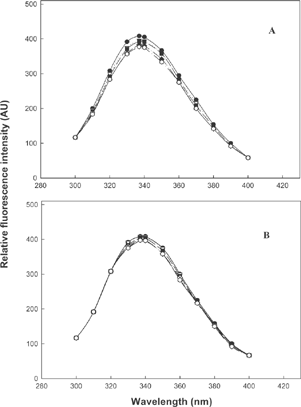
The hydrophobicity of actomyosin decreased with increase in concentrations of polyhydric alcohols (). The exposure of hydrophobic residues might have been hindered by an interaction of OH groups from the co-solvent molecules. The hydrophobic proteins orient more readily than less hydrophobic ones at the surface, with their polar groups directed toward the aqueous phase and their hydrophobic groups towards the nonaqueous phase to lower the surface free energy.[Citation10] Unfolding of myosin molecule at extremes of pH and subsequent refolding might have increased the hydrophobicity.[Citation19]
Table 4 Hydrophobic index of fish actomyosin in presence of different concentrations of co-solvents at pH 7.0 (Tris-HCl buffer)
The far UV CD spectra of actomyosin in presence of co-solvent molecules are shown in . The secondary structural analysis did not show much difference due to the presence of co-solvents. The myosin rod is almost 100% helical, and the globular head is about 50% helical.[Citation20] Helical content of myosin molecule increased by only a few percent (5%) by the presence of 5% mannitol, which completely inhibited the ATPase activity; hence, the loss of ATPase activity does not necessarily imply that the myosin head has been unfolded. It only indicated that the myosin head has undergone some conformational change.[Citation21] The increased helicity might be due to the exposure of α- helix, which extends from Asp327 to Ile340 and forms the top of nucleotide binding pocket [Citation1] in the presence of co-solvents in solutions. The α- helicity of actomyosin decreased during setting, which induces gelation of myosin molecule by the unfolding of long helical tail portion.[Citation22] It has been reported that cold-water fish myosin lost the active sites of ATPase with the unfolding of secondary structure during preparation.[Citation23]
CONCLUSION
Actomyosin from fish varied in its characteristics from other vertebrate actomyosin. The Ca2+ATPase activity of fish actomyosin was less stable to temperature and substrate concentration than other vertebrate actomyosin. That both polyhydric alcohols sorbitol and mannitol induced inhibition of Ca2+ATPase is of importance in dealing with postmortem muscle systems where ATP plays a protective role. The retention of structural integrity of actomyosin, even after the inhibition of Ca2+ATPase activity, opens up a new vista of muscle protection without altering the required properties for further processing. The retardation of nucleotide degradation by these compounds helps to extend the quality of fish products in general during processing and storage.
ACKNOWLEDGEMENT
The award of Senior Research Fellowship to Sijo Mathew by Council of Scientific and Industrial Research, New Delhi during the course of this study is gratefully acknowledged.
REFERENCES
- Rayment , I. , Rypniewski , W.R. , Scmidt-Base , K. , Smith , R. , Tomchik , D.R. , Benning , M. M. , Winkelmann , D. A. , Wesenberg , G. and Holden , H. M. 1993 . Three- Dimensional structure of myosin subfragment-1: A molecular motor . Science , 261 : 50 – 58 . [PUBMED] [INFOTRIEVE]
- Hashimoto , A. , Kobayashi , A. and Arai , K. 1982 . Thermostability of fish myofibrillar Ca2+ATPase and adaptation to temperature . Nippon Suisan Gakkaishi. , 48 : 671 – 684 .
- Rayment , I. 1996 . The structural basis of the myosin ATPase activity . J. Biol Chem. , 271 ( 27 ) : 15850 – 15853 . [PUBMED] [INFOTRIEVE]
- Ochiai , Y. and Chow , C.J. 2000 . “ Myosin ATPase. ” . In Sea Food Enzymes Utilization and Influence on Post Harvest Sea Food Quality , Edited by: Haard , N.F. and Simpson , B.K. 69 – 89 . New York : Marcel Dekker .
- Kimura , I. , Arai , K. , Takahashi , K. and Watanabe , S. 1980 . Earliest event in the heat denaturation of myosin . J. Biochem (Tokyo) , 88 : 1703 – 1713 .
- Margossian , S.M. and Lowey , S. 1982 . “ Preparation of myosin and its subfragments from rabbit skeletal muscle ” . In Methods Enzymology , Vol. 85 , 55 – 71 . New York : Academic Press .
- Bradford , M.M. 1976 . A rapid and sensitive method for the quantitation of microgram quantities of protein utilizing the principle of protein-dye binding . Anal. Biochem. , 72 : 248 – 254 . [PUBMED] [INFOTRIEVE]
- Perry , S.V. 1955 . Myosine adenosine tri phosphatase . Methods in enzymology , 2 : 582 – 588 .
- Taussky , H.H. and Shorr , E. A . 1953 . microcalorimetric method for determination of inorganic phosphorous . J. Biol. Chem. , 202 : 675 – 685 . [PUBMED] [INFOTRIEVE]
- Kato , A. and Nakai , S. 1980 . Hydrophobicity determined by a fluorescence probe method and its correlation with surface properties of proteins . Biochim Biophys. Acta. , 624 : 13 – 20 . [PUBMED] [INFOTRIEVE]
- Yang , J.T. , Wu , C.C. and Martinez , H.M. 1986 . Calculation of protein conformation from circular dichroism . Methods Enzymology , 130 : 208 – 269 .
- Beveridge , T. , Toma , S.J. and Nakai , S. 1974 . Determination of SH and SS groups in some food proteins using Ellman's reagent . J. Food Sci. , 39 : 49 – 51 .
- Park , J.W. and Lanier , T.C. 1987 . Combined effect of phosphates and sugar or polyol on protein stabilization of fish myofibrils . J. Food Sci. , 52 ( 6 ) : 1509 – 1513 .
- Benjakul , S. , Viesessanguan , W. , Ishizaki , S. and Tanaka , M. 2001 . Difference in gelation characteristics of natural actomyosin from two species of big eye snapper, Priacanthus tayenus and Priacanthus macracanthus . J. Food Sci. , 66 ( 9 ) : 1311 – 1318 .
- Sano , T. , Noguchi , S.F. , Matsumotto , J.J. and Tuschiya , T. 1990 . Thermal gelation characteristics of myosin subfragements . J. Food Sci. , 55 : 55 – 58 .
- Chaussepied , P. , Mornet , D. , Barman , E. , Travers , F. and Kassab , R. 1986 . Nucleotide trapping at the ATPase site of the myosin subfragment 1 by a new interthiol cross linking . Proc Natl. Acd. Sci. USA. , 83 : 2037 – 2041 .
- Chaussepied , P. , Morales , M.F. and Kassab , R. 1988 . The myosin SH2-50-kilodalton fragment cross-link: location and consequences . Biochemistry , 27 : 1778 – 1785 . [PUBMED] [INFOTRIEVE] [CROSSREF]
- Wang , B. , Xiong , Y.L. and Srinivasan , S. 1997 . Chemical stability of antioxidant washed beef heart surimi during frozen storage . J. Food Sci. , 62 ( 5 ) : 946 – 948 .
- Kristinsson , H.G. and Hultin , H.O. 2003 . Changes in the conformation and subunit assembly of cod myosin at low and high pH and after subsequent refolding . J. Agric. Food Chem. , 51 ( 24 ) : 7187 – 7196 . [PUBMED] [INFOTRIEVE] [CROSSREF]
- Harrington , W.F. and Rodgers , M.E. 1984 . Myosin . Ann. Rev. Biochem. , 53 : 35 – 73 . [PUBMED] [INFOTRIEVE] [CROSSREF]
- Chan , J.K. , Gill , T.A. , Thompson , J.W. and Singer , D.S. 1995 . Herring surimi during low temperature setting, physicochemical and textural properties . J. Food Sci. , 60 ( 6 ) : 1248 – 1253 .
- Ogawa , M. , Kanamaru , J. , Miyashita , H. , Tamiya , T. and Tsuchiya , T. 1995 . Alpha-Helical structure of fish actomyosin: changes during setting . J Food Sci. , 60 ( 2 ) : 297 – 299 .
- Ogawa , M. , Tamiya , T. and Tsuchiya , T. 1996 . Alpha helical structure of fish actomyosin changes during storage . J.Agric. Food Chem. , 44 : 2944 – 2945 . [CROSSREF]