Abstract
The rapid development in nanomaterials has brought great opportunities to cancer theranostics, which aims to combine diagnostics and therapy for cancer treatment and thereby improve the healthcare of patients. In this review we focus on the recent progress of several cancer theranostic strategies using mesoporous silica nanoparticles and carbon-based nanomaterials. Silicon and carbon are both group IV elements; they have been the most abundant and significant non-metallic substances in human life. Their intrinsic physical/chemical properties are of critical importance in the fabrication of multifunctional drug delivery systems. Responsive nanocarriers constructed using these nanomaterials have been promising in cancer-specific theranostics during the past decade. In all cases, either a controlled texture or the chemical functionalization is coupled with adaptive properties, such as pH-, light-, redox- and magnetic field- triggered responses. Several studies in cells and mice models have implied their underlying therapeutic efficacy; however, detailed and long-term in vivo clinical evaluations are certainly required to make these bench-made materials compatible in real bedside circumstances.
1. Introduction
Cancers do not induce any significant symptoms in the early stages of the disease. The primary emphasis for asymptomatic cancers is to focus on screening and early detection. Traditional clinical diagnostics such as x-ray computed tomography (CT) and magnetic resonance imaging (MRI) are not capable of providing sufficient spatial resolution of the faint changes in the morphology, which occurs in most asymptomatic cancers. In addition, the concentration of relevant biomarkers secreted by cancer cells is usually low. This results in further difficulties in clinical diagnostics and the following therapeutics for most of the common techniques with poor sensitivity.
Traditional chemotherapeutic agents do not discriminate between rapidly dividing normal cells and tumor cells. Their poor selectivity leads to systemic side effects, such as multidrug resistance (MDR) [Citation1]. This has been one of the critical challenges to providing effective chemotherapy to cancer patients. With the advent of nanotechnology, research into cancer treatments has taken on a new dimension, called theranostics, which combines diagnostics and therapeutics to improve the management of healthcare in clinics. In this regime, the development of molecular diagnostic tools and targeted therapeutics is inter-connected, aiming at smart drug release. To circumvent the limitations associated with non-selective chemotherapeutics, researchers have developed different stimuli-responsive drug delivery systems (DDS). The use of micro and nanomaterials such as metals and non-metals, polymers, liposomes, micelles and nanoclusters has been developed in many studies [Citation2–Citation7] for smart drug release. Stimuli-responsive systems for the transport and delivery of materials to a given location at a specific time are highly valuable. These drug carriers are systems that sense the changes in their environment as a stimulus and make the desired response. Nanoscale materials have the potential to radically change cancer therapy and to dramatically improve the efficacy of therapeutic approaches. Consequently, many innovative DDS have been developed to improve their sensitivity and specificity for the enhanced and targeted transport of therapeutic drugs to tumors.
A delicate, smart DDS typically consists of three key components. (i) Specific ligands to enhance tumor selectivity: tumor targeting is usually achieved by immobilizing the ligands, which recognize the cancer-specific receptors, to ensure the cellular uptake effectively. (ii) Stimuli-responsive mechanisms for drug release: drug unloading is usually designed to be triggered by a specific mechanism, such as the pH or the redox potential, which is known to be distinct between a tumor and normal tissues. (iii) Optical labeling or other detectable tracers: this allows the direct visualization of the delivery. The strategy may enable the optimization of drug efficacy with minimizing side effects and assist in improving the drug formulation and development process. The development of such multifunctional stimuli-responsive nanostructured DDS requires the design of nanocarriers, which are biocompatible, composed of non-toxic components, able to be triggered by stimuli and capable of targeting the desired cell or tissue, considering the enormous complexity of the human body. Of all these, the ability to release the drugs in a time- and site-controllable way is one of the most desired features for a drug delivery carrier. The stimuli to trigger the systems could either be internal or external. Depending on the cellular homeostasis, the internal stimuli could be pH, redox status or enzymatic activity. External stimuli such as light, ultrasound or an electromagnetic field could alternatively be used to achieve the time- and site-specific delivery of drugs. With increasing interest in such targeted DDS, significant in vitro experiments have been performed during the last decade.
In this review, we present an overview of several theranostic strategies developed for varied cancer treatments using inorganic silica and carbon-based nanomaterials (figure ). Silicon and carbon are the two most abundant elements on the earth, making them among the most significant non-metallic substances in close acquaintance with human life: carbon is the basis for the chemistry of life; silicon is the major component of electronics and has also been used in a broad range of practical inventions. Their similarity may be explained by examining the electronic configuration of carbon (1s2 2s2 2p2) and silicon (1s2 2s2 2p6 3s2 3p2 (3d)). Both carbon and silicon are group IV elements, having a valence of 4. In other words, the number of bonding electrons is the same in the corresponding subshells, such that the single bond formations of these two elements are very similar in nature. Both carbon and silicon have a broader hardness. While diamond is the hardest substance graphite, however, is a form of carbon and is one of the softest elements known. On the other hand, silicon carbide is of a similar hardness to diamond, whereas mica is the silicon version of carbon's graphite. Above all, these materials provide the advantages of low cost and high biocompatibility, making them ideally suitable for medical diagnosis and therapeutics [Citation8].
Figure 1. The MSNs and carbon-based nanocarriers as versatile platforms for stimuli-responsive drug delivery in cancer theranostics. The time- and space- controlled drug release has been achieved by the employment of light, redox potential, pH gradient and magnetic field. These nanocarriers can be functionalized by (i) biocompatible polymers such as PEG for better blood circulation; (ii) gold nanoparticles or QDs as optical detection probes; (iii) FA, cell penetrating peptides or antibodies as cancer-specific ligands; and finally, (iv) DNA plasmids or small interference RNA (siRNA) for gene therapy.
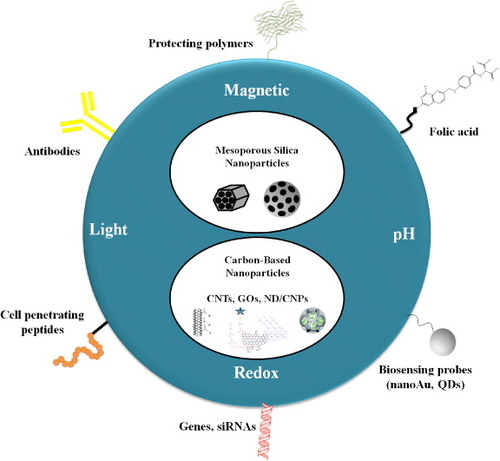
Despite these similarities, the differences in the chemical properties of their compounds are more striking than the similarities. Carbon makes multiple bonds with other carbon molecules, forming alkanes such as CH4 and C2H6. These compounds are analogous to the hydrides of silicon, silanes, which have the chemical formulae SiH4, Si2H6 and so on. However, due to the presence of an available d orbital, silicon may achieve a covalency of six (e.g. SiF62−), whereas carbon forms stable double bonds with other atoms. Furthermore, because of the larger size and lower electronegativity of silicon atoms compared to carbon atoms, the silanes react vigorously with oxygen and water containing hydroxide ions. By comparison, the alkanes are rather unreactive compounds. No reaction occurs when alkanes are simply mixed with oxygen at room temperature. As a matter of fact, alkanes do not react with water molecules. In addition, while there are relatively few carbonate salts, the silicates (the [SiO4]4− tetrahedron-shaped anionic group) can be joined in many different ways, each with a characteristic network of silicon and oxygen atoms. Thus, silicone polymers combine properties of organic compounds as well as those of inorganic compounds, their great stability being especially noteworthy. This brief consideration of the physical/chemical properties of carbon and silica-based materials is of critical importance to evaluate the feasibility of implementing them for the fabrication of multifunctional nanosystems. This review is going to focus on the syntheses and developmental studies of these novel multifunctional materials employed in cancer theranostics.
2. Silica-based nanomaterials
During recent decades, solid silica nanoparticles (SiNPs) and mesoporous silica nanoparticles (MSNs) have been primarily studied and characterized extensively for use in diverse applications. So far, many synthesis methodologies, such as sol–gel synthesis and water in the oil microemulsion technique, have been proposed for synthesizing silica nanoparticles with tunable particle diameters and morphology. Essentially, the choice of synthetic method depends on the requirement of the desired application. For example, larger particles are often necessary for optical applications, whereas smaller particles (< 50 nm) are desirable for cellular uptake [Citation9]. However, smaller particles on the nanoscale tend to grow and aggregate into large particles. In most cases, to synthesize nanoparticles with a specific size and morphology, the microemulsion technique is the method of choice, since it provides adept control of the nucleation and growth of particles within the water core of inverse micelles. By adjusting the concentration of the precursors, the type of surfactant and the molar ratio of water/surfactant, it is possible to tune the size and morphology of the resultant particles by the microemulsion method.
Although SiNPs have been widely applied in DDS and as optical contrast agents for imaging, the functionalization of SiNPs was often limited by their surface. On the other hand, MSNs exhibit a higher surface area and a tunable pore volume, allowing for a higher loading capacity of therapeutic drugs. Recently, silica materials for the enzyme immobilization have been extensively explored. The comparative study of SiNPs and hollow silica nanoparticles for enzyme immobilization demonstrated that the protein loading capacity within hollow silica nanospheres could reach up to more than twice the amount of that on the SiNPs [Citation10]. Silica materials with a hollow structure and tunable pore sizes could adapt to diverse chemical conjugations and facilitate the immobilization of therapeutic proteins or drugs. As a result, MSNs have become prevalent nanoplatforms to design smart DDS for biomedical applications.
A variety of methods have been established to prepare the MSNs. Stöber et al, in the late 1960s, were the first to report the synthesis of monodispersed and spherical silica nanoparticles by introducing a high concentration of surfactant to the traditional sol–gel method [Citation11]. The Mobil Composition of Matter No. 41 (MCM-41), fabricated by Mobil's researchers, was one of the most well-known MSN structures. Although the silica wall is amorphous, the interior of the material possesses an extremely ordered framework with uniform mesopores [Citation12–Citation14]. It was proposed that the cationic surfactant molecules self-organize into a hexagonal structure as a template (figure ) and the silica precursors co-condense with the cylindrical micelles to form MSNs of porosity of 2–50 nm [Citation14, Citation15]. Some studies have further found that the pore structure of MSNs could be determined by controlling the relative amounts of silica source and surfactant molecules in the reaction [Citation16]. The synthesis of smaller sized MSNs (< 100 nm) has been developed by dilution of the surfactant [Citation17] or using a double surfactant system [Citation18]. Following that, a significant number of studies have demonstrated successful control of the particle size, morphology and surface functionalization of the MSN for diverse applications [Citation19–Citation23]. Both novel optical devices and diffusion-controllable drug delivery can be fabricated and achieved by orientation confined one-dimensional mesochannels in films or two-dimensional (2D) mesochannel alignments within MSNs [Citation24, Citation25]. In the following section, we have provided an overview of the MSN-based stimuli-responsive nanosystems. They include various triggers such as the pH, redox gradient, light irradiation and magnetic field, which have been applied to release drugs in cancer theranostics.
Figure 2. MCM-41 type MSNs were synthesized via self-assembly of silica and surfactant species. The cationic surfactants could self-assemble with negative-charged silica precursors to form ordered hexagonal mesoporous nanostructures. Surfactants can be removed via calcination.
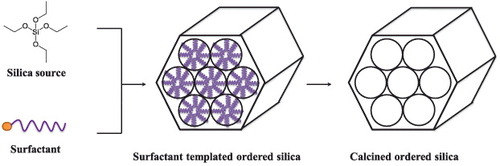
2.1. MSNs in cancer diagnostics and therapy
2.1.1. Light-responsive MSNs.
Light has specific physical characteristics, which can be localized in time and space. As a result, it has been used as a trigger to release encapsulated molecules in light-driven micro and nanosystems. Many researchers have successfully incorporated light-sensitive molecules into a MSN framework to fabricate light-responsive DDS (as shown in figure ). The light-responsive modulation of the MSN state can be reversible or irreversible, which usually depends on how the chromophores link to the silica nanoparticles. Well-known photochromic molecules, such as azobenzene, spiropyran and salicylideneaniline, could be used to tune the properties within nanocarriers, by undergoing a reversible isomerization upon irradiation. The isomerization of the photochromic component in a nanocarrier can usually be followed by a thermal or visible reisomerization process. UV-light in the range of 300–400 nm is generally used for the light-stimulation, while visible light (λ > 400 nm) is typically used for the initiation of the reisomerization process.
Figure 3. Schematic representation of the light-responsive MSNs. The metal core or shell of MSNs can effectively absorb NIR light and convert it to heat. With functionalization of thermal-responsive polymers on the surface of MSNs, drugs could be released upon remote NIR irradiation. Therapeutic drugs can also be immobilized on MSNs via photocleavable linkers. Upon irradiation at corresponding wavelengths, the linkers were cleaved and the cargos were unloaded from the porous structure of MSNs. Additionally, azobenzene derivatives, with their novel characteristics of the cis trans conformation switch by external light, could thereby be applied as ‘gatekeeper’ molecules to regulate the drug release from MSN-based nanocarriers.
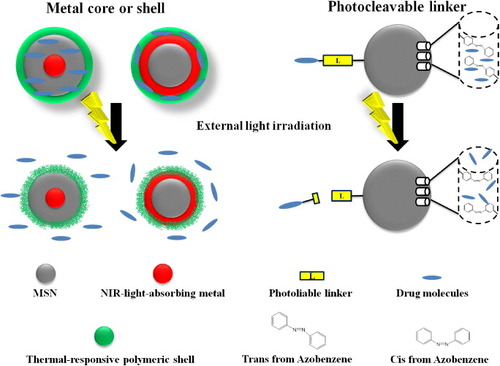
The azobenzene derivatives can be reversibly isomerized between a planar trans form and non-planar cis form in aqueous environments [Citation26–Citation28] under UV or visible light irradiation. In particular, the trans/cis isomerization of these molecules has been the widest applied photo-activated mechanism to modulate the MSN state. An irreversible light-responsive nanosystem can generally be found in silica nanoparticles having functionalized photocleavable components instead of photochromic ones. Upon irradiation, the light-responsive moiety would be cleaved into a more polar species through an irreversible transformation. The chromophore o-nitrobenzyl ester (ONB) [Citation29], featuring an irreversible light-responsive behavior, has been extensively applied in the UV-light initiated photocleavage reaction. In addition, under certain conditions, near-infrared (NIR)-light can also be used for the cleavage of ONB-groups as well. The reversible or irreversible cross-linking reactions based on coumarin dimerization have also been applied to the synthesis of light-responsive MSNs [Citation30]. Various photoresponsive linkers, such as S-coordinated Ru(bpy)2(PPh3)-moieties (absorption at visible light) [Citation31], thioundecyl-tetraethylene-glycol-ester-o-nitrobenzylethyl dimethyl ammonium bromide (TUNA) (absorption at UV region) [Citation32] and 7-amino-coumarin derivative (CD) (absorption at visible or NIR) [Citation33], have been functionalized in MSNs as light-driven release systems. Upon the irradiation of these functionalized MSNs at corresponding wavelengths, the linkers were cleaved and the loaded cargos were released from the pores of the MSNs.
It could be noted that UV or blue light has been frequently applied in light-responsive strategies. Nevertheless, they are less suitable for in vivo therapeutic applications, since UV light tends to trigger unwanted responses, including cellular apoptosis. In addition, the rapid intensity attenuation of the short-wavelength light in tissues further limits their applications in biological systems [Citation34]. Therefore, the irradiation with a wavelength below 700 nm, due to the insufficient penetrability (less than a few micrometers deep), was often limited to skin or external layers of organs. For this reason, UV-responsive MSNs were usually only applied to epidermis or mucosa treatments. To improve the light penetration in tissue, NIR-triggered smart materials have recently drawn considerable attention, facilitating a better resolution in depth (more than a few micrometers) [Citation35]. Such light-responsive systems are primarily composed of metal nanoparticles as the core, which could efficiently absorb the NIR light and convert it to heat for photothermal therapy [Citation36]. Combined with a thermal-responsive polymeric shell or coordinated bonds, drugs could be released with increased temperature upon remote NIR light irradiation [Citation37, Citation38]. It is worth mentioning that the photothermal stability of the metal core becomes important for the successful implementation of these strategies. Both the loading capacity of drugs and the intensity of the NIR irradiation may influence the efficiency of NIR responsive drug delivery, which has been the pivotal factor for the chemo-photothermal therapy.
2.1.2. pH-responsive MSNs.
Recently, some studies [Citation39, Citation40] have incorporated pH-responsive materials in MSNs for endosomal drug delivery, taking advantages of the pH difference between the extracellular tumor milieu (pH 6.6–7.0) and the intracellular pH 5.9–6.0. A schematic representation of the pH-responsive MSNs-based DDS is demonstrated in figure . In order to release drugs under the acidic conditions, such as at pH 5.0–6.0, of late endosomes [Citation41], designing a nanocomposite that can be taken up by cells via endocytosis is the major prerequisite for successful drug delivery. MSNs containing ordered 2D hexagonal arrays of uniform cylindrical pores of diameter ∼ 2 nm are large enough to load molecules, including anticancer drugs or biological dyes. Macrocyclic organic molecules, such as the β-cyclodextrin ring, have been applied as a ‘stopper’ to hinder the cylindrical pores of MSNs. The noncovalent binding interaction between β-cyclodextrin caps and the aromatic amine stalks efficiently blocked the nanopore and trapped the cargo molecules. Lowering the pH could lead to protonation of the amine stalks and the discharge of β-cyclodextrin. The cargo molecules could then unload from the nanopores [Citation42, Citation43].
Figure 4. Schematic representation of the pH-responsive MSNs. Four major molecular designs are shown in the figure: (i) β-cyclodextrin and aromatic amine stalks could serve as effective ‘blockers’ to prevent leakage of drug molecules from nanocarriers. Under acidic pH, the amine stalks were protonated and the discharge of the β-cyclodextrin ring led to on-site drug release. (ii) Nanoparticles were modified on the surface of MSNs via acid-liable linkers to trap drug molecules in the silica mesoporous structures. Cleavage of these acid-sensitive linkers under acidic condition led to drug release. (Alternatively, direct modification of drug molecules with acid-liable linkers can also be achieved.) (iii) Many metal-containing compounds, especially transition metals, consist of coordination complexes. Drug molecules were conjugated with ligands or complexing agents, which enabled strong binding with the metal ion. The coordination bonding of the metal ion and its ligand is sensitive to the external pH and thereby the drug molecules were released. (iv) The dissoluble ZnO QDs could be conjugated on the MSNs to serve as the nanolids. The ZnO QDs were stable and insoluble under neutral pH and rapidly dissolved at a pH below 5.5. As a result, drug molecules can be released from MSNs due to the intracellular pH gradient.
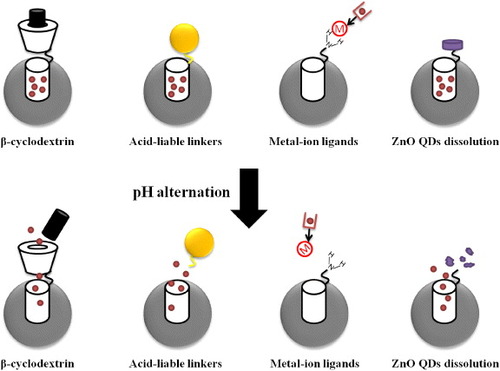
Furthermore, more delicate pH-responsive MSNs have been developed, in which the ‘gatekeeper’ molecules were covalently bonded inside or at the nanopore entries, for controlled drug release. Several research groups have taken great efforts to explore MSNs functionalized with pH-dependent acid-liable linkers. In principle, the drugs were covalently linked to the nanoporous structure of MSNs via acid-sensitive linkers, such as hydrazone and acetal [Citation44–Citation46]. The drugs can be released, followed by the cleavage of hydrazone linkers under acidic conditions. In addition, alternative designs using polyalcohols have also been applied to modify the surface of MSNs, whereas the metal nanoparticles such as superparamagnetic Fe3O4 nanoparticles or gold nanoparticles [Citation47, Citation48] acting as the gatekeepers were modified with boronic acids. It is a reversible process that boronic acids react rapidly with dialcohols or polyalcohols to form boronate esters at neutral pH. Under acidic pH, the esters are again hydrolyzed.
Recently, a novel pH-responsive MSNs DDS based on the interaction of coordinated metal ions and the corresponding ligands has reported [Citation49]. It is based on the fact that both metal ions and protons (H+) are Lewis acids and both are likely to compete for ligands (Lewis base). Here, the coordination bonding of a metal ion and its ligand is sensitive to external pH. Similarly, the modification of chitosan polymeric film on the surface of MSNs has been another smart design for pH-responsive carriers [Citation50–Citation52]. At high pH, chitosan undergoes shrinkage induced by the deprotonated amino groups. By decreasing the pH approximately to the isoelectric point of chitosan, it is possible to reprotonate the amino groups again. The electrostatic repulsion between chitosan and the MSN matrix enabled the cargo release. Here, the delivery of cargo molecules, either in several portions or at various time periods, could easily be controlled by switching the environmental pH.
Moreover, dissoluble ZnO quantum dots (QDs) ‘nanolids’ functionalized MSN conjugates have also shown their potential as pH-responsive nanocarriers. The ZnO QDs exhibit great stability at neutral pH, but rapidly dissolve when the pH is below 5.5. As a result, the trapped molecules can be released from MSNs due to the intracellular pH gradient [Citation53]. The ZnO QD nanolids may prevent the cytotoxic drugs from misallocated release. In addition, they seem to exhibit a synergistic antitumor effect in carcinoma. However, their cytotoxicity towards normal cells or tissues has not been analyzed so far, which still remains a critical issue.
2.1.3. Redox-responsive MSNs.
The redox potential gradient existing between the extracellular and intracellular environment is a widely exploited physiological stimulus for subcellular therapeutics delivery. It has been reported that the concentration of glutathione (GSH) in cytosol is about 2–10 mM. It is much higher than its level in the extracellular milieu (2–20 μM) and is also far more abundant than most of the other redox active compounds [Citation54]. The glutathione disulfide (GSSH) is maintained at a reduced status by enzymes such as GSH reductase [Citation55]; the intracellular GSH level has been tightly regulated by NADH/NAD+, NADPH/NADP+ and the thioredoxinred/thioredoxinox level. A reduced environment also appeared in the endocytosis pathway. The excess level of cysteine in lysosome favors the reduction of disulfide bonds in the co-presence of a specific enzyme, gamma interferon-inducible lysosomal thiol-reductase (GILT) [Citation56]. In cancer cells, the intrinsic increase in the generation of reactive oxygen species often induces redox adaptation in response to the sustained oxidative stress, leading to the up-regulation of antioxidant molecules, such as GSH [Citation57].
To achieve therapeutic selectivity in cancer theranostics, in recent years the design of novel redox-responsive drug nanocarriers, which specifically respond to the redox gradient in the intracellular milieu of carcinoma, has received great interest (figure ). Most of the current MSN-based GSH-mediated controlled release systems share a setup similar to pH-responsive DDS. Cap or gatekeeper molecules, such as collagen [Citation58], polyethylene glycol (PEG) [Citation59] or cyclodextrin [Citation60, Citation61], were stably functionalized on the surface of MSNs using disulfide bond linkers. This prevented the drugs leakage during the delivery routes and ensured that the drugs were released only under reducing conditions. Anticancer drugs, such as doxorubicin (DOX), or dye molecules could also be covalently linked to the inner channels of MSNs via disulfide bonds, to minimize the potential degradation triggered by the chaotic milieu of the biological system [Citation62, Citation63]. Additionally, organic polymers have also been applied as a surface coating to trap the cargo molecules in the cylindrical pores of the MSNs [Citation64, Citation65].
Figure 5. Schematic representation of the redox-responsive MSNs as a novel DDS in cancer cells. In the system, cargo molecules were often tethered in the cylindrical pores of MSNs via disulfide bond linkage or trapped by disulfide cross-linked polymers to prevent drugs leakage during the delivery routes. The cancer specific ligands enabled effective tumor targeting. Upon redox potential stimuli in the cancer cells, the cargo molecules then could be released.
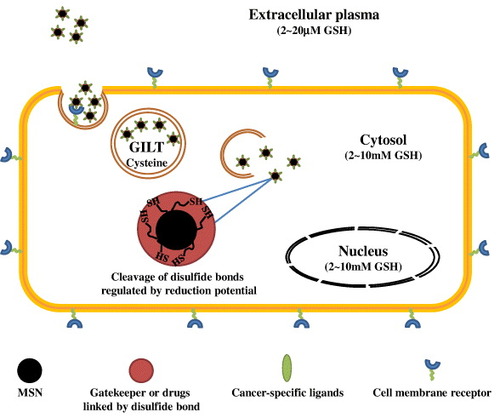
2.1.4. Magnetic-responsive MSNs.
In addition to the light, pH and redox potential, a magnetic field could also serve as an external stimulus to trigger the release of molecules from MSNs. Magnetic nanostructures are of importance in biomedical applications, not only for bioimaging but also for theranostics. MRI is the most representative clinical diagnostic modality, characterized by non-invasive, non-ionizing, real-time and cost-effective features. For soft tissue imaging, researchers commonly employ Gd3+ molecules as chemical contrast agents. In addition to Gd3+, magnetic nanoparticles (mNPs) of elements such as Fe, Ni, Co, Mn, Cr, as well as their chemical compounds are also used in MRI. However, these mNPs alone are usually not target-specific and may circulate throughout the body fluid and enter the large volumes of the blood flow system. The combination of mNPs and MSNs constitutes a promising alternative for drug targeting, as mNPs can carry a drug either attached on their surface or entrapped in a surface coating, which maximizes its drug loading capacity. Exploiting the magnetic effect, the drug could be magnetically guided to the target organ for specific release. Due to the merits of magnetic nanostructures in inducing a hyperthermic effect under an oscillating magnetic field and the contrast enhancement for MRI [Citation66, Citation67], the conjugation of mNPs within mesoporous silica nanostructures holds great promise for hyperthermia therapy.
Typically, two types of MRI contrast agents, namely, T1 and T2, are used (figure ). For T1 MRI tracking methods, ‘positive’ contrast agents are usually used [Citation68]. A metal–chelator complex of gadolinium such as Gd-DTPA has been applied as the preferred technique for MRI and has entered the clinical arena [Citation69, Citation70]. Alternatively, MRI contrast could be enhanced by using manganese oxide nanoparticles as the T1 relaxation agent. Recently, manganese has been used in direct labeling of cells in vitro and for imaging the tumors in vivo [Citation71, Citation72]. Here, to alter the transverse (T2) relaxation times of water protons, the researchers usually employ the T2 agents. In the past decade, T2 MRI has been created by using ‘negative’ contrast agents; this mode provides dark, negative signals in the images when signal intensity increases. Superparamagnetic iron oxide (SPIOs)-capped MSN [Citation73] appeared to be ideal T2 MRI contrast agents for monitoring cells and animal tumors, owing to their high sensitivity, excellent biocompatibility and simple detection [Citation74, Citation75]. The improved stability of the mNPs–MSNs hybrid materials and the potential synergism in local heat production shall enable a better clinical efficacy [Citation76–Citation78]. Significant advances derived from in vitro experiments have been attained; however, in vivo tests have rarely been performed. Much research endeavor is still required to permit the transit from bench to bedside.
Figure 6. Schematic representation of magnetic field-responsive MSNs. A metal–chelator complex of gadolinium such as Gd-DTPA and manganese oxide nanoparticles have been applied as a preferred T1 contrast agent for MRI, while SPIOs and MNPs were commonly introduced as T2 contrast agents which provided dark, negative images as the intensity of the T2 signal increased.
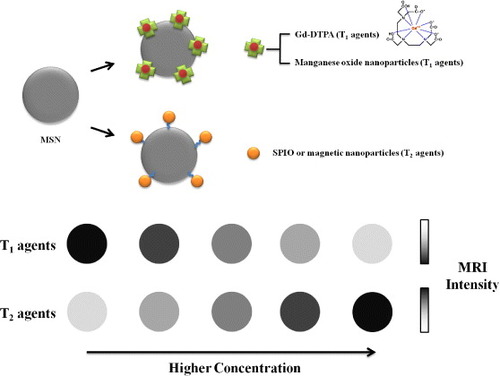
Table provides a brief overview of the various stimuli-responsive MSN-based DDS applied in cancer theranostics. As evidenced from the extensive data, MSN-based DDS have progressed significantly in the past decade. Besides, functionalized MSNs have emerged as promising materials for imaging and drug delivery in cancer theranostics. Here, the cargo molecules can either be loaded into the nanoporous structure of MSN or covalently modified on the surface of MSNs by diverse linkers. By varying the conjugated linkers or nanoparticles, different types of stimuli-responsive nanocarriers, including irradiation, pH, redox and magnetic-driven systems, can be flexibly fabricated. However, silicone polymers have the inherent limitation of having poor optical properties. This often demands laborious chemical modifications to create the required characteristics, especially for non-invasive theranostics.
Table 1. Various stimuli-responsive MSNs-based DDS applied in cancer theranostics.
In the following sections, we will provide an outline of the recent advances in carbon-based nanomaterials. Lately, several interdisciplinary research teams are beginning to focus on carbon-based materials for cancer theranostics [Citation79–Citation83]. The increased interest in these carbon-based nanomaterials is owing to their inherent physical and chemical properties, including NIR emission and a high surface area-to-volume ratio. Additionally, the low toxicity, high biocompatibility and cost-effectiveness, make them ideal candidates for biotheranostics [Citation84–Citation89].
3. Carbon-based nanomaterials
Among the numerous types of nanomaterials developed in recent years, research on nanocarbons such as carbon nanotubes, graphene, fullerene, nanodiamond and carbon nanoparticles (CNPs) has emerged as a booming area, especially for the development of delivery vehicles for imaging agents and drugs. Nanodiamond was first studied in the 1960s in Russia and recently began to be highlighted in medical applications [Citation79]. In 1985, fullerene was discovered as a new class of carbon molecules [Citation90]. The soccer-shaped fullerene possesses an extended π–conjugation system and is composed of sixty carbon atoms. Upon functionalization, it becomes water-soluble and enables applications in biological systems.
The carbon nanotube (CNT), an allotrope of carbon with a cylindrical structure, was first discovered by Iijima in 1991 [Citation91]. It was commonly prepared by three major techniques: laser ablation, thermal or plasma enhanced chemical vapor deposition and electric arc discharge [Citation92]. Depending of the number of cylindrical grapheme layers, CNTs can be mainly categorized into two groups: single-walled carbon nanotubes (SWNTs) and multi-walled carbon nanotubes (MWNTs). Graphene is a novel 2D carbon material, which has gained significant interest since scientists isolated a graphene sheet from graphite crystal in 2004 [Citation93]. Since then, graphene and graphene oxide (GO) were employed in a variety of applications in the biomedical field [Citation94, Citation95]. Yet another form of carbon, CNPs, has recently been discovered. CNPs were separated and purified from an arc-discharge CNT by electrophoresis [Citation96]. Following the development of novel sensors and fluorescent molecule probes based on CNPs, research on CNPs has become the prevailing direction in bioimaging and diagnostics [Citation97, Citation98].
Firstly, we will review the current status of CNT-based nanosystems in cancer theranostics, especially various diagnostic strategies and stimuli-responsive DDS, which exhibited designs similar to MSN-based nanoconstructs. One of the most important properties of CNTs is their intrinsic photoluminescence at the NIR region. In addition, some studies have revealed their capability to penetrate cell membranes, which provides a route for the delivery of cargoes into the cytoplasm and, in many cases, to the nucleus of cells [Citation99]. The high aspect ratio (i.e. length divided by width) of CNTs presents a large surface area for surface modification with various functionalities. Moreover, similar to MSNs, cargoes can be attached to the surfaces (inner or outer) or even packaged within the core of the tubes [Citation100]. As inspiring as these studies have been, the application of CNTs for improving the treatment of cancerous malignancies may not be just an illusion anymore.
3.1. CNTs in cancer diagnostics
Early detection and monitoring of cancer by techniques like photoacoustic imaging may greatly improve the life quality of cancer patients. Conventionally, photoacoustic imaging of cancer at early stages of the disease demands an effective contrast agent, which can convert the light effectively to ultrasound waves. The detection at the NIR or longer wavelength region (700–1100 nm) is highly preferred over the conventional UV–VIS window in biological systems, as NIR absorption in tissue is rather weak. Additionally, low background signals in the longer wavelength region enables the cells to be imaged at deeper tissue sections [Citation101]. In general, cancer cells present in the blood stream are very few in number, making it extremely difficult to distinguish them from the blood cells in the absence of any tumor-specific ligands. Under such conditions, the exogenous use of contrast agents with tumor-specific ligands may facilitate the recognition and detection of the cancer cells. Shi et al [Citation102] had synthesized multifunctional MWNTs for cell targeting and imaging. Both tumor-specific ligands (folic acid (FA)) and the imaging probes (fluorescein isothiocyanate (FITC)) attached to the surface of acid-treated MWNTs by a one-step dendrimer-mediated poly(amidoamine) reaction. Flow cytometry and confocal microscopic results have shown that the MWNT-nanocomposites can specifically target the cancer cells overexpressing folate receptors and can be visualized via FITC labels.
Similarly, Welsher et al [Citation103] reported PEG-functionalized SWNTs with a selective Rituxan antibody, which is capable of recognizing CD20 on B cells. The PEG coating of SWNTs enhanced the stability of the nanocomposite and its biodistribution. Most important of all, the intrinsic NIR fluorescence of SWNTs could serve as optical tracers for bioimaging, without the necessity to label additional fluorescence tags. The data revealed the feasibility of observing interactions between various types of functionalized SWNTs and the living cells, with low interference in auto-fluorescence. Another similar design, which features surface modification of SWNTs with indocyanine green (SWNT-ICG) dye, demonstrated a photoacoustic agent with ultrahigh sensitivity [Citation104]. The immobilization of Arg-Gly-Asp (RGD) peptides on SWNT-ICG enabled the recognition of the cell adhesion molecule, αvβ3 integrins. The in vivo mice experiments indicated that the signal intensity was 300-fold higher in the new contrast agent than that in the case of those already reported. Moreover, this nanoplatform can be applied to detect approximately 20-fold fewer cancer cells than previous SWNT-related studies. Guo et al [Citation105] was the first group to use conjugated QDs to increase luminescence during in vivo mice imaging. The QDs and the anti-cancer drug were chemically coupled on the SWNTs by a novel plasma polymerization approach that deposited ultrathin polymer films. Inductively coupled plasma-mass spectrometry (ICP-MS) analysis has shown the accumulation of CNT-QD in the liver, kidney, stomach and intestine of the mice. The data also suggested that the strong luminescence of QD enables it to serve as a photoacoustic contrast agent for in vivo imaging.
For the early detection of cancer, CNTs doped with superparamagnetic Gd3+ ion clusters (gadonanotubes) have been used as an alternative contrast agent [Citation106]. The Gd3+ ion cluster exhibits unparalleled high relaxivity and pH dependence. Under appropriate conditions (1.5 T, 37 °C, pH = 6.4), the r1 relaxivity of a gadonanotube is 40-fold greater than any current Gd3+ ion-based clinical agent. This pH-sensitive probe might be developed as a clinical agent against cancers, where the pH at malignant lesions can drop to seven or below. Additional CNT-based imaging systems are summarized in table . As already shown in these studies, CNTs exhibit excellent chemical/physical properties and photoacoustic contrast. These encouraging results provide a new direction for early cancer diagnostics. Moreover, diverse novel designs provide an opportunity to overcome traditional limitations in cancer therapy in the future.
Table 2. CNT-based imaging/diagnostic systems in cancer theranostics.
3.2. CNTs in cancer therapy
Unlike traditional cancer therapies such as surgery, chemotherapy or radiotherapy, CNTs serve as smart nanomaterials for the development of novel DDS in cancer theranostics. There have been at least three types of CNT-based cancer treatments, including small molecule drug delivery, photothermal therapy and gene delivery, in which the removal of cancer cells could be achieved via different stimuli-responsive designs similar to MSN-based nanosystems. Here, we have summarized the important results demonstrated by the current studies and the potential applications of CNT-based nanomaterials in clinics.
3.2.1. Chemotherapy.
The genesis of applying CNTs as novel drug carriers originated owing to their high surface area-to-volume ratio and the hydrophobic surface. Many biomolecules, such as oligonucleotides, small interference RNAs (siRNAs), drugs and fluorescent dyes with aromatic ring structures, can easily be immobilized onto CNTs by physical adsorption. Moreover, the acid-mediated oxidation of CNTs contributes to the high density of carboxyl group on the sidewalls of CNTs. As a result, ligands or molecules with amino groups can also be tethered on the CNTs via the conventional 1-ethyl-3-(3-dimethylaminopropyl)carbodiimide/N-hydroxysuccinimide (EDC/NHS) crosslinking method to enhance the targeting selectivity. Additionally, the ability of CNTs to cross the cell membrane by endocytosis further implies their potential to serve as multifunctional DDS.
Table outlines the recent developments in CNT-based DDS, especially with a focus on cancer theranostics. Heister et al [Citation107] demonstrated the functionalization of SWNTs with a monoclonal antibody as the tumor targeting ligand, the anti-cancer drug ‘doxorubicin’ and a fluorescent tag, to construct the triple-function nanocarrier. The results have validated the successful delivery of anti-cancer drugs to human cancer cells via this SWNT-based nanocarrier. The study indicated that the SWNTs were retained in the cytoplasm, while the drugs released from the SWNTs were translocated to the nuclei. Similarly, Chen et al [Citation108] designed and synthesized biotin-modified SWNTs as a novel multi-functional DDS. In this study, biotin was used as the tumor-targeting module and the drug release was regulated through the cleavage of the disulfide bond (figure ). It occurred at the tumor region where the high concentration of endogenous thiols, such as GSH or thioredoxin, was found.
Figure 7. Schematic of a tumor-targeted DDS that uses biotin-linker-taxoid functionalized SWNT nanoconjugates. A link to tumor-targeting modules as well as pro-drug modules has been demonstrated. The tumor-recognition modules were designed by the attachment of biotin and a spacer to the nanotube surface. The conjugation of pro-drug modules of an anticancer agent (taxoid with a cleavable S-S linker) were achieved, which could be activated to its cytotoxic form inside the cancer cells upon internalization and in situ drug release. Adapted with permission from Chen et al [Citation108]. Copyright© 2008, American Chemical Society.
![Figure 7. Schematic of a tumor-targeted DDS that uses biotin-linker-taxoid functionalized SWNT nanoconjugates. A link to tumor-targeting modules as well as pro-drug modules has been demonstrated. The tumor-recognition modules were designed by the attachment of biotin and a spacer to the nanotube surface. The conjugation of pro-drug modules of an anticancer agent (taxoid with a cleavable S-S linker) were achieved, which could be activated to its cytotoxic form inside the cancer cells upon internalization and in situ drug release. Adapted with permission from Chen et al [Citation108]. Copyright© 2008, American Chemical Society.](/cms/asset/a9493223-c067-4976-bdb4-8379980e18a2/tsta_a_11661384_f0007_oc.jpg)
Table 3. CNT-based nanocomposites in cancer theranostics: applications in chemo-, photothermal- and gene therapy.
Likewise, Dhar et al [Citation109] demonstrated the use of PEG to enhance the solubility of SWNTs in water. Additionally, the PEG coating improved the biocompatibility of the material, solving the problem concerning the short retention period of low-molecular-weight Pt(IV) drugs in the blood circulation. In addition, a folate derivative was conjugated on the SWNTs to incorporate the targeting ability to cancer cells having high folate receptor overexpression. The supramolecular pH-sensitive DDS, in which the PEG-coated water soluble SWNTs again served as the carrier, was developed by Liu et al [Citation110]. The cancer drugs and fluorescence tags were adsorbed via π–π stacking. In the study, the authors also found that the release rate of molecules from SWNTs depended on the diameter of the nanotube. Finally, the challenge in the K562 human leukemia cells having MDR has been currently resolved. The anti-P-glycoprotein antibody functionalized SWNTs with loaded DOX were synthesized by Li et al [Citation111]. The results suggested that the prepared SWNT-based nanocarriers specifically and effectively targeted the drug resistant-K562 cells, leading to high cytotoxicity and the suppression of their proliferation.
3.2.2. Photothermal therapy.
As mentioned earlier in this review, conventional cancer treatments are often harmful and systematically damage both the cancer cells and normal tissues. Therefore, targeted-thermal ablation of cancer cells has emerged as a practical alternative to conventional cancer therapies. At temperatures over 40 °C, photothermal therapy induces protein denaturation or membrane damage, resulting in cell death [Citation112]. CNTs enable the absorption of NIR light (wavelength of 700–1100 nm) and convert into heat, which provides the potential for targeted thermal therapy [Citation113, Citation114]. The heat generated via the enhanced vibrational modes of the carbon lattice originates from the excitation of the optical transitions followed by the relaxation [Citation115]. The inherent physicochemical properties of CNTs facilitate the use this material for a less invasive ablation technique in photothermal therapy.
Robinson et al [Citation116] proposed the intravenous injection of SWNTs for in vivo tumor imaging and photothermal therapy. The SWNTs absorbed light at 808 nm and emitted photoluminescence at 1000–1400 nm. The distribution of SWNTs in tumors can also be identified using Raman spectra with high spatial resolution. Under laser exposure, the tumor in mice was completely eliminated without any side effects. Alternative research [Citation117] employed the silica-supported bimetallic cobalt–molybdate catalyst SWNTs (CoMoCAT), which had a uniform size distribution of 0.81 nm and a narrow NIR absorption at 980 nm. The folate-conjugated SWNTs (FA-SWNT) specifically targeted cancer cells having an overexpression of folate receptors, both in vitro and in vivo.
Ghosh et al [Citation112] designed the DNA-encased MWNTs to enhance the heat emission for photothermal therapy, which resulted in a three-fold reduction in the concentration required to achieve an increase in temperature of 10 °C. The reason may be owing to less DNA-MWNTs aggregation rather than non-DNA MWNTs. The results clearly demonstrated that the intra-tumor injection of a limited quantity of DNA-MWNTs could be sufficient enough to eradicate xenograft tumors in mice. Furthermore, the gold-plated carbon nanotube (GNT) developed by Kim et al [Citation82] has been applied as a photoacoustic contrast agent and for photothermal therapy (figure ). The deposited gold film facilitated the conjugation of the antibodies at the surface of CNTs. The data indicated that the GNT exhibited minimal toxicity and a two-order enhancement in NIR contrast. Recently, Klingeler et al [Citation118] synthesized magnetic-responsive MWNT for hyperthermia. The ferromagnetically filled CNTs allowed the non-invasive elevation of temperature in vivo, after employing the magnetic field. In addition, the synergic effect with the loaded anti-cancer drug was an additional merit. So far, numerous groups have developed different strategies for photothermal therapy, using CNTs. Further studies on CNT-based theranostic nanoplatforms can be referred to in table . Although these reports provide us with some hints to optimize the CNT-based material, detailed in vivo studies are still rather limited.
Figure 8. Schematics of GNT-assisted photothermal (PT) and photoacoustic (PA) theranostics. (a) The synthesis of GNTs and the targeted delivery. (b) The principle of targeting endothelial LYVE-1 receptors with antibody–GNT complex (left panel) and PA (top right) and PT (bottom right) detection modules. GNTs conjugated with an antibody specific to the LYVE-1 receptor (anti-LYVE-1 antibody) were delivered to the lymphatic vessels and exposed to a laser pulse using an integrated intravital microscope. Laser-induced PA/PT effects at a relatively low laser energy were used for non-invasive diagnostics. For therapeutic purposes, such as ablating individual cells locally, the increased laser energy accompanied by microbubble formation could be applied. Adapted with permission from Kim et al [Citation82]. Copyright© 2009, Rights Managed by Nature Publishing Group.
![Figure 8. Schematics of GNT-assisted photothermal (PT) and photoacoustic (PA) theranostics. (a) The synthesis of GNTs and the targeted delivery. (b) The principle of targeting endothelial LYVE-1 receptors with antibody–GNT complex (left panel) and PA (top right) and PT (bottom right) detection modules. GNTs conjugated with an antibody specific to the LYVE-1 receptor (anti-LYVE-1 antibody) were delivered to the lymphatic vessels and exposed to a laser pulse using an integrated intravital microscope. Laser-induced PA/PT effects at a relatively low laser energy were used for non-invasive diagnostics. For therapeutic purposes, such as ablating individual cells locally, the increased laser energy accompanied by microbubble formation could be applied. Adapted with permission from Kim et al [Citation82]. Copyright© 2009, Rights Managed by Nature Publishing Group.](/cms/asset/0d0f646b-671b-4c97-8852-0ca51bb0f404/tsta_a_11661384_f0008_oc.jpg)
3.2.3. Gene therapy.
Gene therapy, which directly delivers the oligonucleotides or nucleic acids to cancer cells and inhibits them at the gene level, is a promising strategy, alternative to common radiotherapy or chemotherapy [Citation119, Citation120]. In current studies, most effective gene delivery systems have been achieved by viral vectors [Citation121]. However, virions pose serious cytotoxic side effects, such as inflammation, immunogenic and oncogenic responses. Concerned about these unfavorable factors, researchers strived to develop non-viral carriers, for instance, liposomes, cell-penetrating peptides and cationic lipid polymers [Citation119, Citation122, Citation123]. Nevertheless, the transfection efficiency of non-viral systems is often lower than that of viral vectors due to their poor intracellular uptake and susceptibility to proteases degradation. To overcome the abovementioned bottleneck, CNTs, with their simple-functionalized surface, great membrane penetration and low immunogenicity, have recently been identified as a potential alternative.
According to the study by Herrero et al [Citation124], a series of dendron derivatives with positively charged tetra-alkyl ammonium functionalized on MWNT could serve as a siRNA nanocarrier. The positively charged surface improves both the MWNTs solubility and the membrane penetration. By using fluorescence tags, further study suggested that the single-chain dendron derivatives exhibit the best siRNA delivery efficiency; it has also shown better performance than the conventional liposome-based gene carriers. Another study [Citation86] focused on the development of MWNT-based nanocarriers with functionalized antisense therapeutic oligodeoxynucleotides (ASODNs) and CdTe QDs, prepared via a simple electrostatic-driven layer-by-layer (LbL) strategy (figure ). Here, the QDs served as optical tracers to enable the visualization of the intracellular delivery. The results demonstrated that the ASODNs/CdTe/MWNT nanocomposite could efficiently be used for nucleus translocation and showed good anti-cancer activity.
Figure 9. A CNTs-based gene delivery system fabricated by LbL assembling. The antisense oligodeoxynucleotides (ASODNs) as a therapeutic gene could easily be immobilized on the acid-treated CNTs via the coating of positively charged polymers at the interfaces. Additionally, fluorescent probes such as CdTe QDs could be labeled in ASODNs to track the uptake of materials. Reprinted (adapted) with permission from Jia et al [Citation86]. Copyright© 2007, American Chemical Society.
![Figure 9. A CNTs-based gene delivery system fabricated by LbL assembling. The antisense oligodeoxynucleotides (ASODNs) as a therapeutic gene could easily be immobilized on the acid-treated CNTs via the coating of positively charged polymers at the interfaces. Additionally, fluorescent probes such as CdTe QDs could be labeled in ASODNs to track the uptake of materials. Reprinted (adapted) with permission from Jia et al [Citation86]. Copyright© 2007, American Chemical Society.](/cms/asset/f2096dee-ac47-49ae-b0cc-a07f4c181d1d/tsta_a_11661384_f0009_oc.jpg)
3.3. Other carbon-based nanomaterials in cancer theranostics
Besides CNTs, the other fullerene family also possesses some unique physicochemical properties, which can be adapted to develop novel DDS for cancer theranostics. In the last section of this review, we will provide a brief outline of the current progress in nanodiamond, graphene-derivatives and CNPs. Although clinical applications of these nanomaterials are still in their infancy and relatively few studies have been reported in the literature, the results derived so far indicate the possibilities for significant breakthroughs in the near future.
Graphene is a typical 2D carbon-based nanomaterial with characteristic sp2 hybridized orbitals and an absorption spectra similar to CNTs. Compared to the sophisticated experimental techniques required for the synthesis of CNTs, graphene could be economically fabricated on a large scale from graphite. Consequently, the research on graphene-based DDS for photothermal or gene therapies has recently taken a great leap [Citation125, Citation126]. Markovic et al [Citation127] compared the photothermal activity of graphene nanoparticles and CNTs; they demonstrated that polyvinylpyrrolidone-modified graphene nanoparticles generate more heat than DNA or sodium-dodecylbenzenesulfonate-solubilized CNTs, to destroy U251 human glioma cells in vitro. Similarly, another group studied the delivery of graphene sheets in vivo [Citation81]. In this study, PEG with functionalized amino groups was coated on the nanographene sheet (NGS) to enable the labeling of fluorescent dyes. The half-life of PEG-coated NGS in the blood circulation system can reach up to ∼ 1.5 h. The strong NIR absorption of functionalized-NGS facilitated the efficacy of photothermal therapy and tumors were ablated effectively in mice. By employing a tumor-specific ligand on NGS, a long retention time in tumor cells and the kidney system (i.e., an enhanced permeability and retention effect) has been observed. No obvious side effects were observed either by histology staining or blood analysis in mice.
Similarly, Robinson et al [Citation128] reported the use of a reduced graphene oxide (nano-rGO) single layer of size 20 nm for photothermal therapy. As demonstrated in figure , the modification of amphiphilic, PEGylated polymers and RGD peptides ensured the stability of the DDS and also the selective uptake of U87 mg cancer cells. The results have shown a six-fold higher NIR absorption than non-reduced GO after light stimulation. This once again implies its potential as an efficient photothermal agent. In addition to these materials, several other stimuli-responsive GO-based DDS nanoplatforms have also been reported. For instance, a magnetically-driven superparamagnetic Fe3O4 nanoparticle-GO hybrid synthesized by the chemical precipitation method has been employed in cancer therapy [Citation129]. The authors chemically modified the FA on the hybrid to target HeLa cells. The anti-tumor drug, DOX, was loaded onto the surface of the GO hybrid via π–π stacking, with a loading capacity as high as 0.387 mg mg−1. The resulting multi-functionalized DDS not only responded to the magnetic field, but also depended strongly on the pH values for drug unloading.
Figure 10. The nanosized, reduced graphene oxide (nano-rGO) sheets with high NIR light absorbance and biocompatibility for potential photothermal therapy. The figure illustrates that the attachment of a targeting peptide bearing the RGD motif to nano-rGO afforded selective cellular uptake in cancer cells via αvβ3 integrin receptors recognition. In this nanosystem, stability was further improved by the noncovalent functionalization of amphiphilic PEGylated polymers on the nano-rGO sheets. Adapted with permission from Robinson et al [Citation128]. Copyright© 2011, American Chemical Society.
![Figure 10. The nanosized, reduced graphene oxide (nano-rGO) sheets with high NIR light absorbance and biocompatibility for potential photothermal therapy. The figure illustrates that the attachment of a targeting peptide bearing the RGD motif to nano-rGO afforded selective cellular uptake in cancer cells via αvβ3 integrin receptors recognition. In this nanosystem, stability was further improved by the noncovalent functionalization of amphiphilic PEGylated polymers on the nano-rGO sheets. Adapted with permission from Robinson et al [Citation128]. Copyright© 2011, American Chemical Society.](/cms/asset/44aa8c9b-1dc3-4acf-836e-cfffc95b0ad3/tsta_a_11661384_f0010_oc.jpg)
Quantum-sized (1–10 nm) fluorescent CNPs [Citation97, Citation96–Citation132] are another promising carbon-based nanomaterial for cancer therapy. For both in vitro and in vivo clinical applications, fluorescent CNPs have demonstrated the advantages of environment-friendliness, diminutive toxicity and low cost over semiconductor QDs. Owing to these unique features, they hold the promise for applications such as drug delivery, biosensing and bioimaging. One known example is nanocrystalline diamond (ND) with its inherent diamond-lattice structure. Due to its superior chemical, physical and biological properties, ND has been developed as a multifunctional platform for cancer identification and therapeutics [Citation79]. The small particle size makes it trouble-free to enter the cells by diffusion or endocytosis. Similar to the chemical modifications of CNTs, most of the strategies can be applied in ND to create specific targeting ligand or biocompatible polymers.
Huang et al [Citation133] have demonstrated efficient ND-driven DOX delivery regulated by Cl−. Similarly, Chen et al [Citation134] demonstrated the cluster-mediated interactions of water-dispersible ND with several hydrophobic drugs to enhance their dispersion in water with preserved functionality, thereby enabling novel treatment paradigms that were previously unrealized. In that study, common anti-cancer drugs such as Purvalanol A and 4-hydrooxytamoxifen (4-OHT), which were respectively used in hepatocarinoma and breast cancer therapy, were considered for the investigations. The results have shown that ND-mediated DDS improved the therapeutic efficacy of examined cancers. Likewise, Zhang et al [Citation135] developed a low molecular weight (Mw) polyethyleneimine (PEI) functionalized ND-based nanocarrier and different plasmid DNAs, such as the GFP vector that can be electrostatically adsorbed on the surface of the carrier. The study demonstrated the low cytotoxicity and high transfection efficiency of PEI/NDs compared to the PEI system. The other ND-based system [Citation84] was developed using the LbL deposition technique via electrostatic interaction. As the first step, positively charged polylysine was deposited onto a glass slide, followed by the deposition of negatively charged ND. Finally, the positively charged drugs were immobilized. This DDS successfully suppressed the release of inflammatory mediators and exhibited high biocompatibility in the biological system. The same group [Citation87] proposed a DOX containing a parylene-ND-based hybrid to establish a flexible, robust and slow drug release nanoplatform. The microfilm is capable of slowly releasing the drug for at least 1 month at the rather localized malignant region.
Nevertheless, the synthesis of ND, which involves detonation reactions, is difficult implement on an industrial scale. That said, amorphous CNPs recently became the popular alternative. Amorphous CNPs could be prepared by methods such as the electroxidation of graphite [Citation136], the treatment of carbon precursors with HNO3 [Citation137] and the hydrothermal decomposition of organic compounds [Citation138]. Liu et al [Citation97] obtained amorphous CNTs by treating the carbon precursor with HNO3, followed by refluxing, centrifugation, neutralization and dialysis, to purify the end products. Alternatively, Xu et al [Citation96] employed electrophoresis to separately enrich different lengths of CNTs and fluorescent nanoparticles. Fluorescent CNPs prepared by Cao et al [Citation80] were synthesized using poly(propionyl-ethylenimine-co-ethylenimine) (PPEI-EI) as the surface passivation agent. Due to the small size (sub-10 nm) of CNPs, the nanoparticles easily diffused into the cytoplasma of human breast cancer cells MCF-7 and enabled fluorescent imaging.
Additional examples, such as structurally ordered mesoporous carbon nanoparticles (MCN) with a high surface area and pore morphology, have also been reported [Citation88]. The authors employed MCN loaded with a membrane impermeable fluorescence dye (Fura-2), to investigate the delivery of the MCN (as shown in figure ). Using this system, they successfully observed its uptake by HeLa cells with negligible cytotoxicity. In addition, fullerene with inherent π-conjugation, which absorbs visible light and generates reactive oxygen species after illumination, can also be applied for photodynamic therapy. The study indicated that both Type I (photogeneration of superoxide) and Type II (photogeneration of singlet oxygen) processes occurred in the system. The functionalized fullerenes could kill the mouse cancer cell lines (J774, LLC and CT 26) under light exposure and may serve as a novel nanomaterial to substitute the conventional photosensitizers currently used in clinics [Citation139].
Figure 11. A structurally ordered MCN synthesized using a MCM-48 type MSN as the template. This MCN material could serve as a transmembrane delivery vehicle for the intracellular release of a cell membrane impermeable fluorescence dye, Fura-2, inside human cervical cancer cells (HeLa). Adapted with permission from Kim et al [Citation88]. Copyright© 2008, American Chemical Society.
![Figure 11. A structurally ordered MCN synthesized using a MCM-48 type MSN as the template. This MCN material could serve as a transmembrane delivery vehicle for the intracellular release of a cell membrane impermeable fluorescence dye, Fura-2, inside human cervical cancer cells (HeLa). Adapted with permission from Kim et al [Citation88]. Copyright© 2008, American Chemical Society.](/cms/asset/09fb8922-258f-4a89-90c1-bef7993dd741/tsta_a_11661384_f0011_oc.jpg)
4. Future perspectives
As more knowledge has been gained in disease mechanisms, for instance, the ever-evolving understanding of the cancer microenvironment and the new treatments resulting from discoveries of bioactive molecules and gene therapies, nowadays the researchers can develop more effective DDS for cancer management [Citation140–Citation143]. However, the current DDS exhibited several issues that scientists are still attempting to address. The improvement in theranostics of malignancies is of great necessity. For example, many drug potencies and therapeutic effects are limited or otherwise reduced because of the partial degradation that occurs before they reach a desired target in the body; in most cases once ingested, the release of medications has traditionally been diffusion-controlled and time-dependent drug release delivers treatment continuously, rather than providing relief from symptoms and protection from adverse events. Moreover, injectable medications could be made less expensively and administered more easily if they could simply be dosed orally. However, such an idea cannot be fulfilled until methods are developed to effectively direct drugs through specific organs such as the stomach, where medication can be destroyed in the low pH acidic environment. The mechanisms with which to administer drugs are of critical importance when designing drug carriers. The formulations that control the rate and period of drug delivery and target specific areas of the body for treatment have become the well- accepted trend. While it is required to develop materials which are resistant to pH fluctuation or proteases degradation during drug delivery, to administer drugs for treating cancer patients, the investigation of physiological stimuli-responsive biocompatible/ biodegradable materials has been a distinctly new way of thinking about conventional DDS.
It is important to target the affected regions of the body with specific pharmaceutical compounds; these drugs can be liberated in a controlled manner over time. It could be achieved by only using versatile new delivery platforms that (i) provide specific target recognition on cancer cells, (ii) substantially enhance the delivery of cytotoxic agents to cancer cells with each target recognition event and (iii) selectively guide within compartments of the cells (e.g. the lysosomes or the nucleus). Evidently, to prepare such smart multifunctional pharmaceutical nanocarriers, chemical moieties providing certain required individual properties have to be simultaneously assembled either on the surface or within the structure of the same nanoconstructs. The next generation of theranostic modalities will likely have enhanced surface characteristics, making them more biocompatible, water-soluble or colloidal, displaying reduced toxicity and high differential uptake efficiencies. Moreover, these individual moieties have to function in a certain coordinated way to provide a desired combination of the useful properties. The development of multi-functional nanoscale systems for combined sensing, imaging and therapy are continuing to be active subjects of pharmaceutical research.
The non-metallic nanomaterials-based DDS described here obviously represent little more than the tip of the iceberg of the current developments in cancer theranostics. Although the applications of nanotechnology to clinics appear to have attracted more attention recently, the basic nanotechnology approaches for medical application could actually date back several decades. Liposomes were observed in 1965 by Bangham et al, who investigated their lipid membrane–enclosed structures [Citation144]. Since then, the field has progressed dramatically and applications have been established in several areas, such as drug and gene delivery. Beside the silica- and carbon-based nanoconstructs reviewed here, the other common DDS in this non-metallic category also included the natural or synthetic polymeric nanoparticles and engineered viral nanoparticles [Citation145], etc. The earliest DDS, first introduced in the 1970s, was based on polymers of lactic acid [Citation146]. The first liposome DDS to gain FDA approval in 1995 was Doxil (DOX liposomes) to treat the AIDS associated Kaposi's sarcoma. Abraxane, a paclitaxel/albumin decorated liposome based drug, is another drug formulation which was FDA approved in 2005 as a second line treatment for breast cancer patients [Citation147]. In addition, poly-lactic-co-glycolic acid (PLGA), an FDA approved synthetic polymer, has been utilized in the development of theranostic devices [Citation148, Citation149], owing to its biodegradability and biocompatibility. The degradation process itself involves the breakdown of polymers into small molecules, such as lactic and glycolic acids. These acids eventually enter the Kreb's cycle and become CO2 and H2O, which can be easily expelled by the body. The PEG-modified pharmaceutical nanocarriers have been the most frequent platforms for the improvement of in vivo longevity, which were firstly suggested two decades ago [Citation150, Citation151] and even until now the PEG-based derivatives are still ideal protecting polymers in many studies [Citation59, Citation119, Citation152].
Although there have been several successful breakthroughs in the development of DDS, most have not widely entered the markets and been implemented in the clinics due to the problems which intrinsically limit the manufacture and development of the DDS. The stability, sterilization issues, low drug entrapment, the production of a large batch size and the short circulation half-lives are existing concerns. Chemical instability may be caused by hydrolysis and oxidation. This happened frequently not only in lipid molecules but also in natural polymers, such as gelatin hydrogels. On the other hand, the physical instability may result in the difficulty of precise size control, drug leakage and the fusion of vesicles to form irregular aggregates, which often occurred in liposome-based nanocarriers; the in vivo performance of the drug formulation can therefore influence the therapeutic index. In addition, for gene therapy [Citation153], many clinical trials rely on retroviruses or adenoviruses to deliver the desired gene. The viral gene delivery system may be an excellent platform, which has been developed for the delivery of nucleic acid based therapeutics, such as gene vectors, anti-sense, aptamers and RNAi; it shows a high transfection efficiency compared to most of the well-known DDS, such as liposome- or PEI-based cationic polymeric nanocarriers [Citation154]. They have been applied in a number of FDA-approved clinical trials, such as the SCID-X1 trial [Citation155]. However, the severe concerns of viral-based nanocarriers, including oncogenic effects and immunogenicity, remain unsolved [Citation156].
As discussed above, functionalized MSNs and carbon-based nanocarriers have proven to be promising materials for imaging and drug delivery in cancer theranostics. In these systems, cargo molecules can either be loaded into the nanoporous structure or covalently modified on the surface of these nanocarriers by diverse linkers. In any case, the controlled diameter, porosity, texture or chemical composition is coupled with adaptive properties such as the pH, ionic strength, thermal, light or magnetic-stimulation for molecular-recognition. Given this functionality, these nanocarriers can serve as customizable, rather stable, targeted drug delivery vehicles, capable of carrying large doses of chemotherapeutic or molecular agents into malignant cells, while sparing healthy cells. Current investigations, as already summarized in this review, have proved quite promising, with results indicating that the drug delivery rate, bio/chemical sensing and various stimuli triggering can all be synthetically and precisely controlled. This is expected to greatly reduce or even eliminate the undesirable side effects that limit the efficacy of current cancer therapies.
The Nanomedicine is currently in its early phase of sharp growth and still a considerable number of years away from maturity [Citation157]. Despite the rapid advancements in the field of cancer nanotechnology, there is still a huge gap to transfer the current bench-made nanocarriers to real bedside circumstances. The successful clinical translation of any diagnostic or therapeutic nanocarrier requires an optimization of the parameters including: a variation in the composition of the carrier system, drug loading efficiency, surface hydrophilicity, surface charge, particle size, density of possible ligands for targeting, etc., resulting in a large number of potential variables for optimization, which is impractical to achieve using a low throughput approach. Furthermore, one of the existing challenges similar to most of the other existing DDS in the field has been the time-consuming and laborious synthetic procedures of these nanomaterials, which questions the repeatability of the process in the industrial scale. Implementation of high throughput screening approaches may efficiently solve this problem to screen multiple characteristics simultaneously with the goal of identifying formulations with the desired physical and biochemical properties for each specific application. The selection of cationic polymeric systems suitable for gene or RNAi delivery is an example [Citation158] and can serve as a useful strategy when developing other new silica- or carbon-based nanocomposites in the future.
To apply either MSNs or carbon-based materials in nanomedicine, the same consideration of their safety and cytotoxicity issues as examined in the conventional DDS is required. Detailed experimental results establishing their in vitro biocompatibility, biodistribution, degradation and clearance of these materials in vivo, is of vital importance [Citation159, Citation160]. Many features can be included as potential toxic triggers, such as surface area and size distribution, chemical composition, surface structure, solubility, shape and aggregation. The existing silanol groups in silica nanoparticles enable a variety of chemical modifications in DDS. However, some studies also reveal the latent risk of silanol in hemolysis [Citation161, Citation162]. Carbon-based materials are the rising stars in cancer theranostics due to their superior inherent optical properties, making them readily suitable for several theranostic modalities such as NIR fluorescence, photoacoustic, photothermal and photodynamic therapies [Citation95, Citation163]. In addition, for gene therapy, carbon-based materials have been reported for their intrinsic property of high permeability to the cells and may serve as great alternatives to viral- or PEI-based gene delivery systems [Citation154, Citation164], which are often criticized for their adverse biological responses and the toxicity at high Mw. Nevertheless, unlike most well-established DDS, the toxicological evaluations of carbon-based nanomaterials in current reported studies were actually often performed in a rather simple cell line model and under a short-term period; a detailed understanding of the potential metabolic pathways will be required. Also, in vivo analysis of these systems is relatively limited, with almost no detailed clinical trials. Although these nanomaterials have shown great potential for biomedical applications, our current understanding of the toxicological effects in vivo is, again, still very limited [Citation45, Citation71, Citation75, Citation81, Citation85, Citation105, Citation116]. As a result, detailed exploration of the pharmacological and inherent toxicological properties shall provide a feasible pathway to reach the final clinical needs.
Based on the knowledge we have acquired, we anticipate that the data reported so far, regarding the biological effects of these nanomaterials both in vitro and in vivo, needs to be improved further to achieve realistic clinical needs. The chemical versatility of these non-metallic nanomaterials shall afford these nanocarriers with clinically applicable properties such as better blood circulation, tumor-specificity, biodegradability and clearance from the animal/human body. We foresee their potential in future theranostics.
Acknowledgments
National Science Council of Taiwan (grants no. NSC 101–2113-M-009–006-MY2) and the Ministry of Education, Taiwan (‘Aim for the Top University Plan’ of National Chiao Tung University) supported this work. Y-C C and X-C H contributed equally to this work.
References
- DeanMFojoTBatesS 2005 Tumour stem cells and drug resistance Nature Rev. Cancer 5 275 284 275–84 10.1038/nrc1590
- PaciottiG FKingstonD G ITamarkinL 2006 Colloidal gold nanoparticles: a novel nanoparticle platform for developing multifunctional tumor-targeted drug delivery vectors Drug Dev. Res. 67 47 54 47–54 10.1002/ddr.20066
- SlowingI ITrewynB GGiriSLinV S Y 2007 Mesoporous silica nanoparticles for drug delivery and biosensing applications Adv. Funct. Mater. 17 1225 1236 1225–36 10.1002/adfm.200601191
- NasonklaNBeyERenJAiHKhemtongCGuthiJ SChinS FSherryA DBoothmanD AGaoJ 2006 Multifunctional polymeric micelles as cancer-targeted, MRI-ultrasensitive drug delivery systems Nano Lett. 6 2427 2430 2427–30 10.1021/nl061412u
- SoppimathK STanD CYangY Y 2005 pH-triggered thermally responsive polymer core–shell nanoparticles for drug delivery Adv. Mater. 17 318 323 318–23 10.1002/adma.200401057
- DromiSFrenkelVLukATraughberBAngstadtMBurMPoffJXieJLibuttiS KLiK CWoodB J 2007 Pulsed-high intensity focused ultrasound and low temperature-sensitive liposomes for enhanced targeted drug delivery and antitumor effect Clin. Cancer Res. 13 2722 2727 2722–7 10.1158/1078-0432.CCR-06-2443
- YangHKaoW J 2007 Synthesis and characterization of nanoscale dendritic RGD clusters for potential applications in tissue engineering and drug delivery Int. J. Nanomed. 1 89 99 89–99 10.2147/nano.2007.2.1.89
- ArigaKJiQMcShaneM JLvovY MVinuAHillJ P 2011 Inorganic nanoarchitectonics for biological applications Chem. Mater. 24 728 737 728–37 10.1021/cm202281m
- UboldiCGiudettiGBroggiFGillilandDPontiJRossiF 2012 Amorphous silica nanoparticles do not induce cytotoxicity, cell transformation or genotoxicity in Balb/3T3 mouse fibroblasts Mutat. Res. 745 11 20 11–20 10.1016/j.mrgentox.2011.10.010
- XiaoQ GTaoXZouH KChenJ F 2008 Comparative study of solid silica nanoparticles and hollow silica nanoparticles for the immobilization of lysozyme Chem. Eng. J. 137 38 44 38–44 10.1016/j.cej.2007.09.012
- StöberWFinkABohnE 1968 Controlled growth of monodisperse silica spheres in the micron size range J. Colloid Interface Sci. 26 62 69 62–9 10.1016/0021-9797(68)90272-5
- GrünMLauerIUngerK K 1997 The synthesis of micrometer-and submicrometer-size spheres of ordered mesoporous oxide MCM-41 Adv. Mater. 9 254 257 254–7 10.1002/adma.19970090317
- BeckJ Set al 1992 A new family of mesoporous molecular-sieves prepared with liquid-crystal templats J. Am. Chem. Soc. 114 10834 10843 10834–43 10.1021/ja00053a020
- KresgeC TLeonowiczM ERothW JVartuliJ CBeckJ S 1992 Ordered mesoporous molecular-sieves synthesized by a liquid-crystal template mechanism Nature 359 710 712 710–2 10.1038/359710a0
- HuoQMargoleseD IStuckyG D 1996 Surfactant control of phases in the synthesis of mesoporous silica-based materials Chem. Mater. 8 1147 1160 1147–60 10.1021/cm960137h
- VartuliJSchmittKKresgeCRothWLeonowiczMMcCullenSHellringSBeckJSchlenkerJ 1994 Effect of surfactant/silica molar ratios on the formation of mesoporous molecular sieves: inorganic mimicry of surfactant liquid-crystal phases and mechanistic implications Chem. Mater. 6 2317 2326 2317–26 10.1021/cM0048a018
- CaiQLuoZ-SPangW-QFanY-WChenX-HCuiF-Z 2001 Dilute solution routes to various controllable morphologies of MCM-41 silica with a basic medium Chem. Mater. 13 258 263 258–63 10.1021/cm990661z
- SuzukiKIkariKImaiH 2004 Synthesis of silica nanoparticles having a well-ordered mesostructure using a double surfactant system J. Am. Chem. Soc. 126 462 463 462–3 10.1021/ja038250d
- SlowingI IVivero-EscotoJ LTrewynB GLinV S Y 2010 Mesoporous silica nanoparticles: structural design and applications J. Mater. Chem. 20 7924 7937 7924–37 10.1039/c0jm00554a
- AmbrogioM WThomasC RZhaoY-LZinkJ IStoddartJ F 2011 Mechanized silica nanoparticles: a new frontier in theranostic nanomedicine Acc. Chem. Res. 44 903 913 903–13 10.1021/ar200018x
- ArigaKVinuAYamauchiYJiQHillJ P 2012 Nanoarchitectonics for mesoporous materials Bull. Chem. Soc. Japan 85 1 32 1–32 10.1246/bcsj.20110162
- LiZBarnesJ CBosoyAStoddartJ FZinkJ I 2012 Mesoporous silica nanoparticles in biomedical applications Chem. Soc. Rev. 41 2590 2605 2590–605 10.1039/c1cs15246g
- TarnDAshleyC EXueMCarnesE CZinkJ IBrinkerC J 2013 Mesoporous silica nanoparticle nanocarriers: biofunctionality and biocompatibility Acc. Chem. Res. 46 792 801 792–801 10.1021/ar3000986
- WuK C WJiangXYamauchiY 2011 New trend on mesoporous films: precise controls of one-dimensional (1D) mesochannels toward innovative applications J. Mater. Chem. 21 8934 8939 8934–9 10.1039/c1jm10548e
- WuK C WYamauchiY 2012 Controlling physical features of mesoporous silica nanoparticles (MSNs) for emerging applications J. Mater. Chem. 22 1251 1256 1251–6 10.1039/c1jm13811a
- FerrisD PZhaoY-LKhashabN MKhatibH AStoddartJ FZinkJ I 2009 Light-operated mechanized nanoparticles J. Am. Chem. Soc. 131 1686 1688 1686–8 10.1021/ja807798g
- WangYLiBZhangLSongHZhangL 2012 Targeted delivery system based on magnetic mesoporous silica nanocomposites with light-controlled release character ACS App. Mater. Interfaces 5 11 15 11–5 10.1021/am302492e
- YuanQZhangYChenTLuDZhaoZZhangXLiZYanC-HTanW 2012 Photon-manipulated drug release from a mesoporous nanocontainer controlled by azobenzene-modified nucleic acid ACS Nano 6 6337 6344 6337–44 10.1021/nn3018365
- ParkCLeeKKimC 2009 Photoresponsive cyclodextrin-covered nanocontainers and their sol–gel transition induced by molecular recognition Angew. Chem. Int. Edn. Engl. 48 1275 1278 1275–8 10.1002/anie.200803880
- MalN KFujiwaraMTanakaY 2003 Photocontrolled reversible release of guest molecules from coumarin-modified mesoporous silica Nature 421 350 353 350–3 10.1038/nature01362
- KnezevicN ZTrewynB GLinV S Y 2011 Functionalized mesoporous silica nanoparticle-based visible light responsive controlled release delivery system Chem. Commun. 47 2817 2819 2817–9 10.1039/c0cc04424e
- Vivero-EscotoJ LSlowingI IWuC-WLinV S Y 2009 Photoinduced intracellular controlled release drug delivery in human cells by gold-capped mesoporous silica nanosphere J. Am. Chem. Soc. 131 3462 3463 3462–3 10.1021/ja900025f
- LinQHuangQLiCBaoCLiuZLiFZhuL 2010 Anticancer drug release from a mesoporous silica based nanophotocage regulated by either a one- or two-photon process J. Am. Chem. Soc. 132 10645 10647 10645–7 10.1021/ja103415t
- SchwarzAStänderSBerneburgMBöhmMKulmsDvan SteegHGrosse-HeitmeyerKKrutmannJSchwarzT 2001 Interleukin-12 suppresses ultraviolet radiation-induced apoptosis by inducing DNA repair Nature Cell Biol. 4 26 31 26–31 10.1038/ncb717
- JuzenasPJuzenieneAKaalhusOIaniVMoanJ 2002 Noninvasive fluorescence excitation spectroscopy during application of 5-aminolevulinic acid in vivo Photochem. Photobiol. Sci. 1 745 748 745–8 10.1039/b203459j
- LiuHChenDLiLLiuTTanLWuXTangF 2011 Multifunctional gold nanoshells on silica nanorattles: a platform for the combination of photothermal therapy and chemotherapy with low systemic toxicity Angew. Chem. 123 921 925 921–5 10.1002/ange.201002820
- FangWYangJGongJZhengN 2012 Photo-and pH-triggered release of anticancer drugs from mesoporous silica-coated Pd@ Ag nanoparticles Adv. Funct. Mater. 22 842 848 842–8 10.1002/adfm.201101960
- TangHShenSGuoJChangBJiangXYangW 2012 Gold nanorods@mSiO2 with a smart polymer shell responsive to heat/near-infrared light for chemo-photothermal therapy J. Mater. Chem. 22 16095 16103 16095–103 10.1039/c2jm32599c
- HelmlingerGYuanFDellianMJainR K 1997 Interstitial pH and pO2 gradients in solid tumors in vivo: high-resolution measurements reveal a lack of correlation Nature Med. 3 177 182 177–82 10.1038/nm0297-177
- SilvaA SYunesJ AGilliesR JGatenbyR A 2009 The potential role of systemic buffers in reducing intratumoral extracellular pH and acid-mediated invasion Cancer Res. 69 2677 2684 2677–84 10.1158/0008-5472.CAN-08-2394
- MaxfieldF RMcGrawT E 2004 Endocytic recycling Nature Rev. Mol. Cell Biol. 5 121 132 121–32 10.1038/nrm1315
- ZhaoY-LLiZKabehieSBotrosY YStoddartJ FZinkJ I 2010 pH-operated nanopistons on the surfaces of mesoporous silica nanoparticles J. Am. Chem. Soc. 132 13016 13025 13016–25 10.1021/ja105371u
- MengHXueMXiaTZhaoY-LTamanoiFStoddartJ FZinkJ INelA E 2010 Autonomous in vitro anticancer drug release from mesoporous silica nanoparticles by pH-sensitive nanovalves J. Am. Chem. Soc. 132 12690 12697 12690–7 10.1021/ja104501a
- LinC-HChengS-HLiaoW-NWeiP-RSungP-JWengC-FLeeC-H 2012 Mesoporous silica nanoparticles for the improved anticancer efficacy of cis-platin Int. J. Pharm. 429 138 147 138–47 10.1016/j.ijpharm.2012.03.026
- LeeC HChengS HHuangISourisJ SYangC SMouC YLoL W 2010 Intracellular pH-responsive mesoporous silica nanoparticles for the controlled release of anticancer chemotherapeutics Angew. Chem. 122 8390 8395 8390–5 10.1002/ange.201002639
- LiuRZhangYZhaoXAgarwalAMuellerL JFengP 2010 pH-responsive nanogated ensemble based on gold-capped mesoporous silica through an acid-labile acetal linker J. Am. Chem. Soc. 132 1500 1501 1500–1 10.1021/ja907838s
- GanQLuXYuanYQianJZhouHLuXShiJLiuC 2011 A magnetic, reversible pH-responsive nanogated ensemble based on Fe3O4 nanoparticles-capped mesoporous silica Biomaterials 32 1932 1942 1932–42 10.1016/j.biomaterials.2010.11.020
- AznarEMarcosM DMartiánez-MaánñezR NSancenónFSotoJAmoroásPGuillemC 2009 pH-and photo-switched release of guest molecules from mesoporous silica supports J. Am. Chem. Soc. 131 6833 6843 6833–43 10.1021/ja810011p
- GaoCZhengHXingLShuMCheS 2010 Designable coordination bonding in mesopores as a pH-responsive release system Chem. Mater. 22 5437 5444 5437–44 10.1021/cm100667u
- PopatALiuJLuG Q MQiaoS Z 2012 A pH-responsive drug delivery system based on chitosan coated mesoporous silica nanoparticles J. Mater. Chem. 22 11173 11178 11173–8 10.1039/c2jm30501a
- ChenFZhuY 2012 Chitosan enclosed mesoporous silica nanoparticles as drug nano-carriers: sensitive response to the narrow pH range Micropor. Mesopor. Mater. 150 83 89 83–9 10.1016/j.micromeso.2011.07.023
- TangHGuoJSunYChangBRenQYangW 2011 Facile synthesis of pH sensitive polymer-coated mesoporous silica nanoparticles and their application in drug delivery Int. J. Pharm. 421 388 396 388–96 10.1016/j.ijpharm.2011.10.013
- MuhammadFGuoMQiWSunFWangAGuoYZhuG 2011 pH-Triggered controlled drug release from mesoporous silica nanoparticles via intracelluar dissolution of ZnO nanolids J. Am. Chem. Soc. 133 8778 8781 8778–81 10.1021/ja200328s
- SchaferF QBuettnerG R 2001 Redox environment of the cell as viewed through the redox state of the glutathione disulfide/glutathione couple Free Radi. Biol. Med. 30 1191 1212 1191–212 10.1016/S0891-5849(01)00480-4
- WuGFangY-ZYangSLuptonJ RTurnerN D 2004 Glutathione metabolism and its implications for health J. Nutr. 134 489 492 489–92
- ArunachalamBPhanU TGeuzeH JCresswellP 2000 Enzymatic reduction of disulfide bonds in lysosomes: characterization of a gamma-interferon-inducible lysosomal thiol reductase (GILT) Proc. Nat. Acad. Sci. 97 745 750 745–50 10.1073/pnas.97.2.745
- TrachoothamDAlexandreJHuangP 2009 Targeting cancer cells by ROS-mediated mechanisms: a radical therapeutic approach? Nature reviews. Drug Disc. 8 579 591 579–91 10.1038/nrd2803
- LuoZCaiKHuYZhaoLLiuPDuanLYangW 2011 Mesoporous silica nanoparticles end-capped with collagen: redox-responsive nanoreservoirs for targeted drug delivery Angew. Chem. Int. Edn. 50 640 643 640–3 10.1002/anie.201005061
- CuiYDongHCaiXWangDLiY 2012 Mesoporous silica nanoparticles capped with disulfide-linked peg gatekeepers for glutathione-mediated controlled release ACS Appl. Mater. Interfaces 4 3177 3183 3177–83 10.1021/am3005225
- KimHKimSParkCLeeHParkH JKimC 2010 Glutathione-induced intracellular release of guests from mesoporous silica nanocontainers with cyclodextrin gatekeepers Adv. Mater. 22 4280 4283 4280–3 10.1002/adma.201001417
- ZhangQLiuFNguyenK TMaXWangXXingBZhaoY 2012 Multifunctional mesoporous silica nanoparticles for cancer-targeted and controlled drug delivery Adv. Funct. Mater. 22 5144 5156 5144–56 10.1002/adfm.201201316
- SauerA MSchlossbauerARuthardtNCaudaVBeinTBraäuchleC 2010 Role of endosomal escape for disulfide-based drug delivery from colloidal mesoporous silica evaluated by live-cell imaging Nano Lett. 10 3684 3691 3684–91 10.1021/nl102180s
- ZongSWangZChenHYangJCuiY 2013 Surface enhanced Raman scattering traceable and glutathione responsive nanocarrier for the intracellular drug delivery Anal. Chem. 85 2223 2230 2223–30 10.1021/ac303028v
- ZhuC-LSongX-YZhouW-HYangH-HWenY-HWangX-R 2009 An efficient cell-targeting and intracellular controlled-release drug delivery system based on MSN-PEM-aptamer conjugates J. Mater. Chem. 19 7765 7770 7765–70 10.1039/b907978e
- WanXWangDLiuS 2010 Fluorescent pH-sensing organic/inorganic hybrid mesoporous silica nanoparticles with tunable redox-responsive release capability Langmuir 26 15574 15579 15574–9 10.1021/la102148x
- DavisJ JHuangW-YDaviesG-L 2012 Location-tuned relaxivity in Gd-doped mesoporous silica nanoparticles J. Mater. Chem. 22 22848 22850 22848–50 10.1039/c2jm35116a
- CarniatoFTeiLCossiMMarcheseLBottaM 2010 A chemical strategy for the relaxivity enhancement of GdIII chelates anchored on mesoporous silica nanoparticles Chemistry-A Eur. J. 16 10727 10734 10727–34 10.1002/chem.201000499
- LinW-ILinC-YLinY-SWuS-HHuangY-RHungYChangCMouC-Y 2013 High payload Gd (iii) encapsulated in hollow silica nanospheres for high resolution magnetic resonance imaging J. Mater. Chem. B 1 639 645 639–45 10.1039/c2tb00283c
- LiuJBuWZhangSChenFXingHPanLZhouLPengWShiJ 2012 Controlled synthesis of uniform and monodisperse upconversion core/mesoporous silica shell nanocomposites for bimodal imaging Chemistry-A Eur. J. 18 2335 2341 2335–41 10.1002/chem.201102599
- Guillet-NicolasRBridotJ LSeoYFortinM AKleitzF 2011 Enhanced relaxometric properties of MRI ‘Positive’ contrast agents confined in three-dimensional cubic mesoporous silica nanoparticles Adv. Funct. Mater. 21 4653 4662 4653–62 10.1002/adfm.201101766
- KimTMominEChoiJYuanKZaidiHKimJParkMLeeNMcMahonM TQuinones-HinojosaA 2011 Mesoporous silica-coated hollow manganese oxide nanoparticles as positive T 1 contrast agents for labeling and MRI tracking of adipose-derived mesenchymal stem cells J. Am. Chem. Soc. 133 2955 2961 2955–61 10.1021/ja1084095
- ChenYYinQJiXZhangSChenHZhengYSunYQuHWangZLiY 2012 Manganese oxide-based multifunctionalized mesoporous silica nanoparticles for pH-responsive MRI, ultrasonography and circumvention of MDR in cancer cells Biomaterials 33 7126 7137 7126–37 10.1016/j.biomaterials.2012.06.059
- ChenP-JHuS-HHsiaoC-SChenY-YLiuD-MChenS-Y 2011 Multifunctional magnetically removable nanogated lids of Fe3O4–capped mesoporous silica nanoparticles for intracellular controlled release and MR imaging J. Mater. Chem. 21 2535 2543 2535–43 10.1039/c0jm02590a
- LeeJ ELeeNKimHKimJChoiS HKimJ HKimTSongI CParkS PMoonW K 2009 Uniform mesoporous dye-doped silica nanoparticles decorated with multiple magnetite nanocrystals for simultaneous enhanced magnetic resonance imaging, fluorescence imaging, and drug delivery J. Am. Chem. Soc. 132 552 557 552–7 10.1021/ja905793q
- ChenYChenHZengDTianYChenFFengJShiJ 2010 Core/shell structured hollow mesoporous nanocapsules: a potential platform for simultaneous cell imaging and anticancer drug delivery ACS Nano 4 6001 6013 6001–13 10.1021/nn1015117
- ThomasC RFerrisD PLeeJ-HChoiEChoM HKimE SStoddartJ FShinJ-SCheonJZinkJ I 2010 Noninvasive remote-controlled release of drug molecules in vitro using magnetic actuation of mechanized nanoparticles J. Am. Chem. Soc. 132 10623 10625 10623–5 10.1021/ja1022267
- BaezaAGuisasolaERuiz-HernaándezEVallet-RegiáM a 2012 Magnetically triggered multidrug release by hybrid mesoporous silica nanoparticles Chem. Mater. 24 517 524 517–24 10.1021/cm203000u
- BringasEKöysürenÖQuachD VMahmoudiMAznarERoehlingJ DMarcosM DMartínez-MáñezRStroeveP 2012 Triggered release in lipid bilayer-capped mesoporous silica nanoparticles containing SPION using an alternating magnetic field Chem. Commun. 48 5647 5649 5647–9 10.1039/c2cc31563g
- SchrandAHensS A CShenderovaO 2009 Nanodiamond particles: properties and perspectives for bioapplications Crit. Rev. Solid State Mater. Sci. 34 18 74 18–74 10.1080/10408430902831987
- CaoLWangXMezianiM JLuFWangHLuoP GLinYHarruffB AVecaMMurrayDXieS YSunY P 2007 Carbon dots for multiphoton bioimaging J. Am. Chem. Soc. 129 11318 11319 11318–19 10.1021/ja073527l
- YangKZhangSZhangGSunXLeeS TLiuZ 2010 Graphene in mice: ultrahigh in vivo tumor uptake and efficient photothermal therapy Nano Lett. 10 3318 3323 3318–23 10.1021/nl100996u
- KimJ WGalanzhaE IShashkovE VMoonH MZharovV P 2009 Golden carbon nanotubes as multimodal photoacoustic and photothermal high-contrast molecular agents Nature Nanotechnol. 4 688 694 688–94 10.1038/nnano.2009.231
- MrozPTegosG PGaliHWhartonTSarnaTHamblinM R 2007 Photodynamic therapy with fullerenes Photochem. Photobiol. Sci. 6 1139 1149 1139–49 10.1039/b711141j
- HuangHPierstorffEOsawaEHoD 2008 Protein-mediated assembly of nanodiamond hydrogels into a biocompatible and biofunctional multilayer nanofilm ACS Nano 2 203 212 203–12 10.1021/nn7000867
- LiuXTaoHYangKZhangSLeeS TLiuZ 2011 Optimization of surface chemistry on single-walled carbon nanotubes for in vivo photothermal ablation of tumors Biomaterials 32 144 151 144–51 10.1016/j.biomaterials.2010.08.096
- JiaNLianQShenHWangCLiXYangZ 2007 Intracellular delivery of quantum dots tagged antisense oligodeoxynucleotides by functionalized multiwalled carbon nanotubes Nano Lett. 7 2976 2980 2976–80 10.1021/nl071114c
- LamRChenMPierstorffEHuangHOsawaEHoD 2008 Nanodiamond-embedded microfilm devices for localized chemotherapeutic elution ACS Nano 2 2095 2102 2095–102 10.1021/nn800465x
- KimT WChungP WSlowingI ITsunodaMYeungE SLinV S 2008 Structurally ordered mesoporous carbon nanoparticles as transmembrane delivery vehicle in human cancer cells Nano Lett. 8 3724 3727 3724–27 10.1021/nl801976m
- BhirdeA APatelVGavardJZhangGSousaA AMA.LeapmanR DWeigertRGutkindJ SRuslingJ F 2009 Targeted killing of cancer cells in Vivo and in Vitro with EGF ACS Nano 3 307 316 307–16 10.1021/nn800551s
- KrotoH WHeathJ RO'BriemS CCurlR FSmalleyR E 1985 C-60: Buckminsterfullerene Nature 318 162 163 162–63 10.1038/318162a0
- IijimaS 1991 HELICAL Microtubules of graphitic carbon Nature 354 56 58 56–8 10.1038/354056a0
- BaughmanR HZakhidovA Ade HeerW A 2002 Carbon nanotubes—the route toward applications Science 297 787 792 787–92 10.1126/science.1060928
- GeimA KNovoselovK S 2007 the rise of graphene Nature Mater. 6 183 191 183–91 10.1038/nmat1849
- ZhangYNayakT RHongHCaiW 2012 Graphene: a versatile nanoplatform for biomedical applications Nanoscale 4 3833 3842 3833–42 10.1039/c2nr31040f
- YangKFengLShiXLiuZ 2013 Nano-graphene in biomedicine: theranostic applications Chem. Soc. Rev. 42 530 547 530–47 10.1039/c2cs35342c
- XuXRayRGuYPloehnH JGearheartLRakerKScrivensW A 2004 Electrophoretic analysis and purification of fluorescent single-walled carbon nanotube fragments J. Am. Chem. Soc. 126 12736 12737 12736–7 10.1021/ja040082h
- LiuHYeTMaoC 2007 Fluorescent carbon nanoparticles derived from candle soot Angew. Chem. Int. Edn Engl. 46 6473 6475 6473–5 10.1002/anie.200701271
- RayS CSahaAJanaN RSarkarR 2009 Fluorescent carbon nanoparticles: synthesis, characterization, and bioimaging application J. Phys. Chem. C 113 18546 18551 18546–51 10.1021/jp905912n
- KamN W SLiuZ ADaiH J 2006 Carbon nanotubes as intracellular transporters for proteins and DNA: an investigation of the uptake mechanism and pathway Angew. Chem. Int. Edn. Engl. 45 577 581 577–81 10.1002/anie.200503389
- KatzEWillnerI 2004 Biomolecule-functionalized carbon nanotubes: applications in nanobioelectronics ChemPhysChem 5 1084 1104 1084–104 10.1002/cphc.200400193
- FriebelMMeinkeM 2005 Determination of the complex refractive index of highly concentrated hemoglobin solutions using transmittance and reflectance measurements J. Biomed. opt. 10 064019 10.1117/1.2138027
- ShiDGuoYDongZLianJWangWLiuGWangLEwingR C 2007 Quantum-dot-activated luminescent carbon nanotubes via a nano scale surface functionalization for in vivo imaging Adv. Mater. 19 4033 4037 4033–7 10.1002/adma.200700035
- WelsherKLiuZDaraciangDDaiH 2008 Selective probing and imaging of cells with single walled carbon nanotubes as near-infrared fluorescent molecules Nano Lett. 8 586 590 586–90 10.1021/nl072949q
- de la ZerdaALiuZBodapatiSTeedRVaithilingamSKhuri-YakubB TChenXDaiHGambhirS S 2010 Ultrahigh sensitivity carbon nanotube agents for photoacoustic molecular imaging in living mice Nano Lett. 10 2168 2172 2168–72 10.1021/nl100890d
- GuoYet al 2008 In vivo imaging and drug storage by quantum-dot-conjugated carbon nanotubes Adv. Funct. Mater. 18 2489 2497 2489–97 10.1002/adfm.200800406
- HartmanK BLausSBolskarR DMuthupollaiRHelmLTothEMebrachA EWilsonL J 2008 Gadonanotubes as ultrasensitive pH-smart probes for magnetic resonance imaging Nano Lett. 8 415 419 415–19 10.1021/nl0720408
- HeisterENevesVTîlmaciuCLipertKBeltránV SColeyH MSilvaS R PMcFaddenJ 2009 Triple functionalisation of single-walled carbon nanotubes with doxorubicin, a monoclonal antibody, and a fluorescent marker for targeted cancer therapy Carbon 47 2152 2160 2152–60 10.1016/j.carbon.2009.03.057
- ChenJChenSZhaoXKuznetsovaL VWongS SOjimaL 2008 Functionalized single-walled carbon nanotubes as rationally designed vehicles for tumor-targeted drug delivery J. Am. Chem. Soc. 130 16778 16785 16778–85 10.1021/ja805570f
- DharSLiuZThomaleJDaiHLippardS J 2008 Targeted single-wall carbon nanotube-mediated Pt(IV) prodrug delivery using folate as a homing device J. Am. Chem. Soc. 130 11467 11476 11467–76 10.1021/ja803036e
- LiuZSunXRatchfordN NDaiH 2007 Supramolecular chemistry on water-soluble carbon nanotubes for drug loading and delivery ACS Nano 1 50 56 50–6 10.1021/nn700040t
- LiRWuRZhaoLWuMYangLZouH 2010 P-glycoprotein antibody functionalized carbon nanotube overcomes the multidrug resistance of human leukemia cells ACS Nano 4 1399 1408 1399–408 10.1021/nn9011225
- GhoshSDuttaSGnomesECarrollDJrR DOlsonJGutholdMGmeinerW H 2009 Increased heating efficiency and selective thermal ablation of malignant tissue with dna-encased multiwalled carbon nanotubes ACS Nano 3 2667 2673 2667–73 10.1021/nn900368b
- MoonH KLeeS HChoiH C 2009 In vivo near-infrared mediated tumor destruction by photothermal effect of carbon nanotubes ACS Nano 3 3707 3713 3707–13 10.1021/nn900904h
- ChakravartyPMarchesRZimmermanN SSwaffordA DBajajPMusselmanI HPantanoPDraperR KVitettaE S 2008 Thermal ablation of tumor cells with antibody-functionalized single-walled carbon nanotubes Proc. Natl Acad. Sci. USA 105 8697 8702 8697–702 10.1073/pnas.0803557105
- CarlsonL JKraussT D 2008 Photophysics of individual single-walled carbon nanotubes Acc. Chem. Res. 41 235 243 235–43 10.1021/ar700136v
- RobinsonJ TWelsherKTabakmanS MSherlockS PWangHLuongRDaiH 2010 High performance in vivo near-IR (> 1 mum) imaging and photothermal cancer therapy with carbon nanotubes Nano Research 3 779 793 779–93 10.1007/s12274-010-0045-1
- ZhouFXingDOuZWuBResascoD EChenW R 2009 Cancer photothermal therapy in the near-infrared region by using single-walled carbon nanotubes J. Biomed. Opt. 14 021009 10.1117/1.3078803
- KlingelerRHampelSBuchnerB 2008 Carbon nanotube based biomedical agents for heating, temperature sensoring and drug delivery Int. J. Hyperth. 24 496 505 496–505 10.1080/02656730802154786
- HatakeyamaHAkitaHKogureKOishiMNagasakiYKihiraYUenoMKobayashiHKikuchiHHarashimaH 2007 Development of a novel systemic gene delivery system for cancer therapy with a tumor-specific cleavable PEG-lipid Gene Ther. 14 68 77 68–77 10.1038/sj.gt.3302843
- MorilleMPassiraniCVonarbourgAClavreulABenoitJ P 2008 Progress in developing cationic vectors for non-viral systemic gene therapy against cancer Biomaterials 29 3477 3496 3477–96 10.1016/j.biomaterials.2008.04.036
- WaehlerRRussellS JCurielD T 2007 Engineering targeted viral vectors for gene therapy Nature Rev. Genetics 8 573 587 573–87 10.1038/nrg2141
- Ferrer-MirallesNVazquezEVillaverdeA 2008 Membrane-active peptides for non-viral gene therapy: making the safest easier Trends Biotechnol. 26 267 275 267–75 10.1016/j.tibtech.2008.02.003
- DassC RChoongP F 2006 Selective gene delivery for cancer therapy using cationic liposomes: in vivo proof of applicability J. Control. Release 113 155 163 155–63 10.1016/j.jconrel.2006.04.009
- HerreroM ATomaF MAl-jamalK TKosrarelosKBiancoARosT DBanoFCasalisLScolesGPratoM 2009 Synthesis and characterization of a carbon nanotube-dendron series for efficient siRNA delivery J. Am. Chem. Soc. 131 9843 9848 9843–48 10.1021/ja903316z
- YangXZhangXMaYHuangYWangYChenY 2009 Superparamagnetic graphene oxide–Fe3O4 nanoparticles hybrid for controlled targeted drug carriers J. Mater. Chem. 19 2710 10.1039/b821416f
- LuC HZhuC LLiJLiuJ JChenXYangH H 2010 Using graphene to protect DNA from cleavage during cellular delivery Chem. Commun. (Camb.) 46 3116 3118 3116–8 10.1039/b926893f
- MarkovicZ MHarhaji-TrajkovicL MTodorovic-MarkovicB MKepicD PArsikinK MJovanovicS PPantovicA CDramicaninM DTrajkovicV S 2011 In vitro comparison of the photothermal anticancer activity of graphene nanoparticles and carbon nanotubes Biomaterials 32 1121 1129 1121–9 10.1016/j.biomaterials.2010.10.030
- RobinsonJ TTabakmanS MLiangYWangHCasalongueH SVinhDDaiH 2011 Ultrasmall reduced graphene oxide with high near-infrared absorbance for photothermal therapy J. Am. Chem. Soc. 133 6825 6831 6825–31 10.1021/ja2010175
- YangXWangYHuangXMaYHuangYYangRDuanHChenY 2011 Multi-functionalized graphene oxide based anticancer drug-carrier with dual-targeting function and pH-sensitivity J. Mater. Chem. 21 3448 10.1039/c0jm02494e
- BottiniMBalasubramanianCDawsonM IBergamaschiABellucciSMustelinT 2006 Isolation and characterization of fluorescent nanoparticles from pristine and oxidized electric arc-produced single-walled carbon nanotubes J. Phys. Chem. B 110 831 836 831–6 10.1021/jp055503b
- FangYGuoSLiDZhuCRenWDongSWangE 2012 Easy synthesis and imaging applications of cross-linked green fluorescent hollow carbon nanoparticles ACS Nano 6 400 409 400–9 10.1021/nn2046373
- SunY Pet al 2006 Quantum-sized carbon dots for bright and colorful photoluminescence J. Am. Chem. Soc. 128 7756 7757 7756–7 10.1021/ja062677d
- HuangHPierstorffEOsawaEHoD 2007 Active nanodiamond hydrogels for chemotherapeutic delivery Nano Lett. 7 3305 3314 3305–14 10.1021/nl071521o
- ChenMPierstorffE DLamRLiS YHuangHOsawaEHoD 2009 Nanodiamond-mediated delivery of water-insoluble therapeutics ACS Nano 3 2016 2022 2016–22 10.1021/nn900480m
- ZhangX QChenMLamRXuXOsawaEHoD 2009 Polymer-functionalized nanodiamond platforms as vehicles for gene delivery ACS Nano 3 2609 2616 2609–16 10.1021/nn900865g
- ZhaoQ-LZhangZ-LHuangB-HPengJZhangMPangD-W 2008 Facile preparation of low cytotoxicity fluorescent carbon nanocrystals by electrooxidation of graphite Chem. Commun. (Camb.) 5116 5118 5116–8 10.1039/b812420e
- ZhangSHeQLiRWangQHuZLiuXChangX 2011 Study on the fluorescence carbon nanoparticles Mater. Lett. 65 2371 2373 2371–3 10.1016/j.matlet.2011.05.025
- LuWQinXLiuSChangGZhangYLuoYAsiriA MAl-YoubiA OSunX 2012 Economical, green synthesis of fluorescent carbon nanoparticles and their use as probes for sensitive and selective detection of mercury(II) ions Anal. Chem. 84 5351 5357 5351–7 10.1021/ac3007939
- MrozPPawlakASattiMLeeHWhartonTGaliHSarnaTHamblinM R 2007 Functionalized fullerenes mediate photodynamic killing of cancer cells: Type I versus Type II photochemical mechanism Free Rad. Biol. Med. 43 711 719 711–9 10.1016/j.freeradbiomed.2007.05.005
- ShanbhagP PJogS VChogaleM MGaikwadS S 2013 Theranostics for cancer therapy Curr. Drug. Deliv. 10 357 362 357–62 10.2174/1567201811310030013
- PovoskiS PHatzarasI SMojzisikC MMartinE WJr. 2011 Oncologic theranostics: recognition of this concept in antigen-directed cancer therapy for colorectal cancer with anti-TAG-72 monoclonal antibodies Expert Rev. Mol. Diagn. 11 667 670 667–70 10.1586/erm.11.54
- OmidiY 2011 Smart multifunctional theranostics: simultaneous diagnosis and therapy of cancer Bioimpacts 1 145 147 145–7
- ChenWXuNXuLWangLLiZMaWZhuYXuCKotovN A 2010 Multifunctional magnetoplasmonic nanoparticle assemblies for cancer therapy and diagnostics (theranostics) Macromol. Rapid Commun. 31 228 236 228–36
- BanghamA DStandishM MWatkinsJ C 1965 Diffusion of univalent ions across the lamellae of swollen phospholipids J. Mol. Biol. 13 238 252 238–52 10.1016/S0022-2836(65)80093-6
- TorchilinV 2009 Multifunctional and stimuli-sensitive pharmaceutical nanocarriers Eur. J. Pharm. Biopharm. 71 431 444 431–44 10.1016/j.ejpb.2008.09.026
- LangerRFolkmanJ 1976 Polymers for the sustained release of proteins and other macromolecules Nature 263 797 800 797–800 10.1038/263797a0
- HarriesMEllisPHarperP 2005 Nanoparticle albumin-bound paclitaxel for metastatic breast cancer J. Clin. Oncol. 23 7768 7771 7768–71 10.1200/JCO.2005.08.002
- KhoeeSRahmatolahzadehR 2012 Synthesis and characterization of pH-responsive and folated nanoparticles based on self-assembled brush-like PLGA/PEG/AEMA copolymer with targeted cancer therapy properties: a comprehensive kinetic study Eur. J. Med. Chem. 50 416 427 416–27 10.1016/j.ejmech.2012.02.027
- AsteteC ESabliovC M 2006 Synthesis and characterization of PLGA nanoparticles J. Biomater. Sci. Polym. Ed. 17 247 289 247–89 10.1163/156856206775997322
- KlibanovA LMaruyamaKTorchilinV PHuangL 1990 Amphipathic polyethyleneglycols effectively prolong the circulation time of liposomes FEBS Lett. 268 235 237 235–7 10.1016/0014-5793(90)81016-H
- PapahadjopoulosDet al 1991 Sterically stabilized liposomes: improvements in pharmacokinetics and antitumor therapeutic efficacy Proc. Natl Acad. Sci. USA 88 11460 11464 11460–4 10.1073/pnas.88.24.11460
- LiuZRobinsonJ TSunXDaiH 2008 PEGylated nanographene oxide for delivery of water-insoluble cancer drugs J. Am. Chem. Soc. 130 10876 10877 10876–77 10.1021/ja803688x
- BuningH 2013 Gene therapy enters the pharma market: the short story of a long journey EMBO Mol. Med. 5 1 3 1–3 10.1002/emmm.201202291
- WenYPanSLuoXZhangXZhangWFengM 2009 A biodegradable low molecular weight polyethylenimine derivative as low toxicity and efficient gene vector Bioconjug. Chem. 20 322 332 322–32 10.1021/bc800428y
- Cavazzana-CalvoMet al 2000 Gene therapy of human severe combined immunodeficiency (SCID)-X1 disease Science 288 669 672 669–72 10.1126/science.288.5466.669
- ThomasC EEhrhardtAKayM A 2003 Progress and problems with the use of viral vectors for gene therapy Nature Rev. Genetics 4 346 358 346–58 10.1038/nrg1066
- FarokhzadO CLangerR 2006 Nanomedicine: developing smarter therapeutic and diagnostic modalities Adv. Drug. Deliv. Rev. 58 1456 1459 1456–9 10.1016/j.addr.2006.09.011
- AndersonD GPengWAkincAHossainNKohnAPaderaRLangerRSawickiJ A 2004 A polymer library approach to suicide gene therapy for cancer Proc. Natl Acad. Sci. USA 101 16028 16033 16028–33 10.1073/pnas.0407218101
- Maurer-JonesM AHaynesC L 2012 Toward correlation in in vivo and in vitro nanotoxicology studies J. Law Med. Ethics 40 795 801 795–801
- Maurer-JonesM ABantzK CLoveS AMarquisB JHaynesC L 2009 Toxicity of therapeutic nanoparticles Nanomedicine 4 219 241 219–41 10.2217/17435889.4.2.219
- Slowing,IIWuC WVivero-EscotoJ LLinV S 2009 Mesoporous silica nanoparticles for reducing hemolytic activity towards mammalian red blood cells Small 5 57 62 57–62 10.1002/smll.200800926
- LinY SHaynesC L 2009 Synthesis and characterization of biocompatible and size-tunable multifunctional porous silica nanoparticles Chem. Mater. 21 3979 3986 3979–86 10.1021/cm901259n
- JiS RLiuCZhangBYangFXuJLongJJinCFuD LNiQ XYuX J 2010 Carbon nanotubes in cancer diagnosis and therapy Biochim. Biophys. Acta. 1806 29 35 29–35
- HanS-oMahatoR IKimS W 2001 Water-soluble lipopolymer for gene delivery Bioconjug. Chem. 12 337 345 337–45 10.1021/bc000120w
- De la ZerdaAet al 2008 Carbon nanotubes as photoacoustic molecular imaging agents in living mie Nature Nanotechnol. 3 557 562 557–62 10.1038/nnano.2008.231
- ZavaletaCZerdaA D LLiuSKerenSChengZSchipperMChenXDaiHGambhirS S 2008 Noninvasive raman spectroscopy in living mice for evaluation of tumor targeting with carbon nanotubes Nano Lett. 8 2800 2805 2800–05 10.1021/nl801362a
- ShiXWangS HShenMAntwerpM EChenXLiCPetersenE JHuangQWeberW JJrBakerJ RJr 2009 Multifunctional dendrimer-modified multiwalled carbon nanotubes synthesis, characterization and in vitro cancer cell targeting and imaging Biomacromolecules 10 1744 1750 1744–50 10.1021/bm9001624
- WuWLiRBianXZhuZDingDLiXJiaZJiangXHuY 2009 Covalently combining carbon nanotubes with anticancer agent preparation and antitumor activity ACS Nano 3 2740 2750 2740–50 10.1021/nn9005686
- ZhangXMengLLuQFeiZDysonP J 2009 Targeted delivery and controlled release of doxorubicin to cancer cells using modified single wall carbon nanotubes Biomaterials 30 6041 6047 6041–7 10.1016/j.biomaterials.2009.07.025
- LiuZFanA CRakhraKSherlockSGoodwinAChenXYangQFelsherD WDaiH 2009 Supramolecular stacking of doxorubicin on carbon nanotubes for in vivo cancer therapy Angew. Chem. Int. Edn. Engl. 48 7668 7672 7668–72 10.1002/anie.200902612
- FeazellR PRatchfordN NDaiHLippardS J 2007 Soluble single-walled carbon nanotubes as longboat delivery systems for platinum (IV) anticancer drug design J. Am. Chem. Soc. 129 8438 8439 8438–39 10.1021/ja073231f
- Ali-BoucettaHAl-JamalK TMcCarthyDPratoMBiancoAKostarelosK 2008 Multiwalled carbon nanotube-doxorubicin supramolecular complexes for cancer therapeutics Chem. Commun. (Camb.) 459 461 459–61 10.1039/b712350g
- KimJ WShashkovE VGalanzhaE IKotagiriNZharovV P 2007 Photothermal antimicrobial nanotherapy and nanodiagnostics with self-assembling carbon nanotube clusters Lasers Surg. Med. 39 622 634 622–34 10.1002/lsm.20534
- GannonC Jet al 2007 Carbon nanotube-enhanced thermal destruction of cancer cells in a noninvasive radiofrequency field Cancer 110 2654 2665 2654–65 10.1002/cncr.23155