Abstract
Several microbes have evolved clinically significant resistance against almost every available antibiotic. Yet the development of new classes of antibiotics has lagged far behind our growing need. Frequent and suboptimal use of antibiotics particularly in developing countries aggravated the problem by increasing the rate of resistance. Therefore, developing new and multidimensional strategies to combat microbial infections is warranted. These include i) modification of existing antibiotics, ii) searching new and novel antibiotics, iii) development and improvement of antibiotics carrier system to reduce amount and frequency of antibiotic doses, iv) development of targeted antibiotic delivery systems. Here, the authors discuss trends and development of nano-materials and alternative antimicrobials to solve the problem of antibiotic resistance.
1. Problem of resistance and new antimicrobials: a brief update
Recently published reports have raised concerns that antibacterial drug development is too slow to address the threat of rapidly increasing antibiotic resistance among important bacterial pathogens Citation[1,2]. Clinicians are facing shortage in the availability of alternative therapeutic options like analogs of already known compounds and new agents belonging to completely new classes of antibiotics. Ongoing research in this direction has been reviewed recently. In case of gram-negative bacterial pathogens, research is focused on old antibiotics (cephalosporins, carbapenems, β-lactamase inhibitors, monobactams, aminoglycosides, polymyxin analogs, and tetracycline) and completely new antibacterial classes (boron-containing antibacterial protein synthesis inhibitors, bis-indoles, outer membrane synthesis inhibitors, antibiotics targeting novel sites of the 50S ribosomal subunit and antimicrobial peptides) Citation[3]. Likewise for gram-positive bacterial pathogens, research is going on old antimicrobials [quinolone, β-lactam, oxazolidinone, diaminopyrimidine, macrolide, pleuromutilin, aminoglycoside, ansamycin, FabI inhibitor, glycopeptide, metallic ion, streptogramin, tetracycline, vancomycin, dalbavancin and hybrid (oxazolidinone/quinolone and rifamycin/fluoroquinolone)] and completely new antibacterial classes (WAP 8294A2, PZ-601, ME1036, NXL101, friulimicin B, oritavancin, telavancin, ceftobiprole medocaril, ceftaroline fosamil, tomopenem, hLF1-11, lactoferrin, telactoferrin-α) with new target or new mechanism of action. But it will take long time for these drugs to reach the market because they are in the early stages of development Citation[1,4,5]. Therefore, until these new/modified antimicrobials are available for clinicians, infection control policies and optimization in the use of already existing molecules remain the most effective approaches to reduce the spread of resistance and preserve the activity of antibiotics.
2. Nano-materials to combat antibiotic resistance
Although conventional antibiotics have several disadvantages like i) evolution of resistance with time, ii) low therapeutic index, iii) cytotoxicity and side effects, iv) non-specific mode of action and v) inconvenient route of administration, but these problems can be solved to some extent using different antimicrobial delivery systems involving nano-technology which refers to the design, production and application of nano-sized materials (1–100 nm). Their unique physical and chemical properties (small size, high surface-to-volume ratio and amenable for surface modification) may be exploited as vehicles to carry various therapeutic or diagnostic agents to surpass most physiological barriers and gain access to biologic molecules and intended targets. High surface-to-volume ratio allows nano-materials for increased potential to interact with pathogens and membranes. Thus, they can be potentially used for medical applications including targeted drug delivery, gene therapy and cell labeling Citation[6]. Different types of nano-materials and alternative antimicrobials (organic and inorganic nano-materials, liposomes, biomolecules like polysaccharides, lipids, proteins/peptides and virus capsids) with medical applications have been summarized elsewhere that can help to combat antibiotic resistance Citation[7-10]. Nano-materials tagged with antibiotics facilitate increased concentration of antibiotics at the site of infection and enhance the binding of antibiotics to bacteria. Likewise, combining nano-particles with antimicrobial peptides and essential oils generates genuine synergy against bacterial resistance Citation[11]. Here, we describe the use of different types of nano-materials on the basis of their physio-chemical properties to facilitate targeted antimicrobial delivery and to combat the problem of antibiotic resistance.
2.1 Chitosan
Chitosan is a natural polysaccharide biopolymer derived from chitin. Being polycationic in nature, it favors the interaction of negatively charged microbial cell walls and cytoplasmic membranes, resulting in decreased osmotic stability, membrane disruption and eventual leakage of intracellular elements Citation[12-14]. In addition, chitosan is able to enter the nuclei of bacteria and fungi and inhibit mRNA and protein synthesis by binding to microbial DNA Citation[13]. Nano-scaled chitosan that has a higher surface-to-volume ratio, resulting in higher surface charge density, leads to increased affinity to bacteria and fungi and greater antimicrobial activity. Findings of previously published reports have encouraged using this nano-material for antibiotic encapsulation and efficient delivery Citation[6,12-17].
2.2 Liposomes
Liposomes are spherical vesicles consisting of one or more phospholipid bilayers surrounding a water space. The diameter of the liposome varies from 0.02 to 10 µm. Vesicle formulations are usually based on natural and synthetic phospholipids and cholesterol. The structure may also possess lipoproteins Citation[18]. The physicochemical properties of liposomes can be modified by changing the types of lipids: the composition and proportions of lipids in the liposomal formulation; the size of the liposome; the charge of the liposomal surface: positive, negative, or neutral; pH sensitivity; temperature sensitivity; the fluidity of the liposomal membrane: rigid and fluid liposomes. Regarding the variety of liposomal formulations, the vesicles are universal carriers for both hydrophilic and hydrophobic compounds. The size of the liposomal vesicles significantly influences drug distribution. Large (> 1 µm) MLV (Multilamellar Vesicles) formulations are usually not used as antibiotic carriers, but SUVs (Small Unilamellar Vesicles) of ∼ 100 nm exhibited high efficacy in the eradication of bacterial pathogens Citation[19].
Encapsulation of the antibiotics in lipid vesicles is a good solution for designing the required pharmacokinetic and pharmacodynamic properties Citation[20-23]. This process may improve pharmacokinetics and biodistribution, decreased toxicity, enhanced activity against intracellular pathogens, target selectivity, enhanced activity of antibiotics against extracellular pathogens, in particular to overcome antibiotic resistance. More details about advantages and recent applications about liposome can be found elsewhere Citation[19]. Antibiotic delivery (β-lactams, ampicillin, cephalosporins, macrolides, aminoglycosides, and fluoroquinolones) to enhance microbial killing, facilitated by nano-sized vehicles/liposomes have been reported recently.
2.3 Inorganic nano-materials
Inorganic nano-materials are either in use for centuries or under development as antimicrobials. Noble metals (like silver, gold, platinum, and palladium), carbon-based materials (carbon nano-fibers and different kinds of carbon nano-tubes), semiconducting materials (CdSe, CdS, ZnS, TiO2, PbS, InP, Si/SiO2), magnetic materials (Fe3O4, Co, CoFe2O4, FePt, CoPt and their composites) and lanthanide materials (Gd2O3, Eu2O3) are some of the important inorganic nano-materials used as antimicrobials as well as antibiotic delivery system; and have been described previously Citation[7-9].
2.4 Silver
Silver has been used for centuries for the treatment of burns and wounds to prevent infection. The exact mechanism remains unknown, but it has been proposed that due to the smaller size of silver and silver ions (< 10 nm), they are able to penetrate bacterial cell walls and membranes via interaction with sulfur-containing proteins or thiol groups Citation[24]. Once inside the cell, Ag/Ag+ targets and damages bacterial DNA and respiratory enzymes, leading to loss of the cell's replicating abilities and ultimately cell death Citation[14]. The small size and large surface area of silver nano-particles (AgNPs) makes them effective against a variety of pathogens Citation[13,14,24-28]. AgNPs have also been found to augment the efficacy of other antibiotics, like the activity of penicillin G, amoxicillin, erythromycin, clindamycin and vancomycin increased against S. aureus and E. coli when mixed with AgNPs Citation[26]. Microbes are also less likely to develop resistance against silver and AgNPs, as their broad range of targets would require multiple and simultaneous compensatory mutations. As a result, silver can be used to arrest bacterial resistance to antibiotics and enhance their efficacy Citation[14].
2.5 Copper
Copper is utilized for its antifungal and antibacterial activity but the mechanism remains unknown. The use of CuO nano-particles (CuO-NPs) as a novel antimicrobial agent has recently been investigated. When compared to AgNPs, CuO-NPs were shown to be less effective against E. coli and methicillin-resistant S. aureus but more effective against B. subtilis, which may be due to copper's greater interaction with amine and carboxyl groups on the cell surface of this pathogen Citation[27,29]. Other metals (like zinc, titanium and magnesium) are also used and reported to be potential antimicrobials/carriers which have been described elsewhere Citation[6].
2.6 Carbon nano-tubes
Carbon nano-tubes (CNTs) are emerging as a new family of nano-vectors for the delivery of different types of therapeutic molecules given their capacity to interact with macromolecules such as proteins, antibiotics and oligosaccharides Citation[30-32]. Covalent modification by the organic functionalization of end groups and side walls of f-CNTs allows for a dramatic increase of the solubility of functionalized carbon nano-tubes in a range of solvents, including water Citation[33]. Water-soluble carbon nano-tubes interact with mammalian cells, leading to their cytoplasmic translocation Citation[32]. These properties led us to believe that f-CNTs may be an effective vehicle for oral administration of AmB Citation[30].
2.7 Biodegradable polymeric nano-particles
Biodegradable polymeric nano-particles came into picture for the first time in 1980s and have been extensively investigated for enhanced intracellular drug delivery Citation[34]. Structural stability in biologic fluids and under harsh conditions, precisely tunable properties (e.g., size, zeta-potentials, and drug release profiles) Citation[35] and facile and versatile surface functionalization for conjugating drugs and targeting ligands Citation[36] are various advantages of polymeric nano-particles. Majority of polymeric nano-particles prepared with linear polymers are either nano-capsules or solid nano-spheres Citation[37]. Other kind of polymeric nano-particles are amphiphilic block copolymers which are self-assembled micellar NPs with the drug encapsulating hydrophobic core and the hydrophilic corona shielding the core from opsonization and degradation Citation[38].
2.8 Dendrimers
Dendrimers are hyperbranched polymers with precise nano-architecture and low polydispersity, which are synthesized in a layer-by-layer fashion around a core unit, resulting in a high level control of size, branching points (drug conjugation capability), and surface functionality Citation[39]. The highly branched nature of dendrimers provides enormous surface area to size ratios that generate great reactivity to microorganisms in vivo Citation[35]. The highly dense surface of functional groups allows the synthesis of dendrimers with specific and high binding affinities to a wide variety of viral and bacterial receptors Citation[40]. Both hydrophobic and hydrophilic drugs can be loaded/conjugated/adsorbed inside empty internal cavities in the core and on the multivalent surfaces of dendrimers respectively Citation[41]. Polymeric nano-particles have been explored to deliver various antimicrobial agents and greatly enhanced therapeutic efficacy in treating many types of infectious diseases has been reported and described in .
Table 1. Nano-carriers for antimicrobial drug delivery.
2.9 Targeted drug-carrying phages
Targeted drug-carrying phages are a new class of nano-medicines that combines biologic and chemical components into a modular nano-metric drug delivery system (bio-conjugated delivery system). The core of the system is filamentous phage particles which are produced in the bacterial host Escherichia coli. Target specificity is provided by a targeting moiety, usually an antibody that is displayed on the tip of the phage particle. A large drug payload is chemically conjugated to the protein coat of the phage via a chemically or genetically engineered linker that provides for controlled release of the drug after the particle homed to the target cell () Citation[42]. Hepatitis B virus (HBV) vaccine is one of the best examples of bio-conjugated nano-particles delivery system. These are bionano-capsules (BNCs) consisting of hepatitis B virus (HBV) surface antigen (HBsAg) (approximately 50-nm hollow particles) displaying a human hepatocyte-recognizing molecule (pre-S1 peptide) Citation[43].
Figure 1. Schematic representation of targeted drug delivery by using filamentous phage to kill pathogenic bacteria colonized in host. Receptor and antibody specificity can be exploited for targeted delivery. Genetically engineered filamentous phage loaded with drug molecules inside its protein coat and displaying antibody on its tip can burst to release drug molecules when it binds with its complementary receptors. The released drug molecules attack pathogenic bacteria and kill it specifically. Here ‘A' stands for antibody; ‘B' for bacteria; ‘P' for protein coat; ‘D' for drug/antibiotic; ‘L' for chemical linker and ‘R' for receptor.
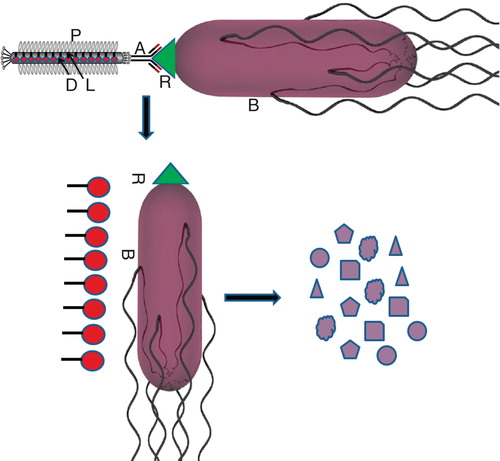
Despite its potential, there are various limitations of nano-carriers, which include size, charge, purity, solubility of contents, stability, antigenicity, and biocompatibility. Lower tolerance for variability in these parameters can lead to increased costs of synthesis and manufacture. The specificity of delivery of nano-carriers to target tissues can be passive or active. Passive targeting has been exemplified in recently published reports which described the use of enhanced permeability and retention (EPR) effect to deliver tumor-selective macromolecular drugs Citation[44]. Active targeting of nano-carriers is dependent on receptor-ligand interactions. Sometimes activation of nano-carrier drug delivery is dependent on cellular activity (pH change or oxidative burst).
3. Alternative strategies to cope up antibiotics resistance
New alternative strategies have been proposed recently to solve the problem of scarcity of novel antibiotics. One of the alternative approaches is to target functions essential for infection, such as virulence factors required to cause host damage and disease. This approach has several potential advantages including expanding the repertoire of bacterial targets, preserving the host endogenous microbiome, and exerting less selective pressure, which may result in decreased resistance. Recently published reviews have described this approach in detail Citation[45,46]. Another strategy is focused on natural antimicrobials, known as host defense peptides or antimicrobial peptides, which defend host organisms against microbes but most have modest direct antibiotic activity. Following this approach, enhanced variants have been developed using straightforward design and optimization strategies and are being tested clinically. Recently published review described advanced computer-assisted design strategies that address the difficult problem of relating primary sequence to peptide structure, and is delivering more potent, cost-effective, broad-spectrum peptides as potential next-generation antibiotics Citation[47,48].
4. Conclusion
This is high time to address the problem of antibiotic resistance and to work on alternative strategies to find out the solutions. Here, we suggested some of them very briefly. Nano-technology and nano-medicines are emerging fields that may be utilized for antibiotic delivery. It will not only increase therapeutic index but also reduce the problem of non-specific cytotoxicity as well as antibiotic resistance. It is essential to develop cost effective nano-materials and improve their capacity to carry antibiotics and deliver more efficiently. More focused studies are also required to develop targeted antibiotic delivery systems like bacteriophages. Other alternative strategies are also worthy to look for searching novel compound and antibiotics which can target virulence functioning of microbes. Antimicrobial peptides, lipopeptides and other natural compounds should also be screened and already existing compounds should be engineered to enhance their antimicrobial efficiency. Following these multiple approaches is a prerequisite to find the solution for antibiotic resistance.
Declaration of interest
A Sharma receives funding from JRF, UGC, Government of India. AK Johri and M Dua are supported by the Capacity Buildup Fund JNU. DKA receives funding from the UGC (Rajiv Gandhi Fellowship).
Bibliography
- Freire-Moran L, Aronsson B, Manz C, Critical shortage of new antibiotics in development against multidrug-resistant bacteria-Time to react is now. Drug Resist Updat 2011;14:118-24
- Bush K, Courvalin P, Dantas G, Tackling antibiotic resistance. Nat Rev Microbiol 2011;9:894-6
- Bassetti M, Ginocchio F, Mikulska M, Will new antimicrobials overcome resistance among Gram-negatives? Expert Rev Anti Infect Ther 2011;9:909-22
- Koomanachai P, Crandon JL, Nicolau DP. Newer developments in the treatment of Gram-positive infections. Expert Opin Pharmacother 2009;10:2829-43
- van Hal SJ, Paterson DL. New Gram-positive antibiotics: better than vancomycin? Curr Opin Infect Dis 2011;24:515-20
- Blecher K, Nasir A, Friedman A. The growing role of nanotechnology in combating infectious disease. Virulence 2011;2:395-401
- Veerapandian M, Yun K. Functionalization of biomolecules on nanoparticles: specialized for antibacterial applications. Appl Microbiol Biotechnol 2011;90:1655-67
- Faraji AH, Wipf P. Nanoparticles in cellular drug delivery. Bioorg Med Chem 2009;17:2950-62
- Gaucher G, Marchessault RH, Leroux JC. Polyester-based micelles and nanoparticles for the parenteral delivery of taxanes. J Control Release 2010;143:2-12
- Subbiah R, Veerapandian M, Yun KS. Nanoparticles: functionalization and multifunctional applications in biomedical sciences. Curr Med Chem 2010;17:4559-77
- Allahverdiyev AM, Kon KV, Abamor ES, Coping with antibiotic resistance: combining nanoparticles with antibiotics and other antimicrobial agents. Expert Rev Anti Infect Ther 2011;9:1035-52
- Banerjee M, Mallick S, Paul A, Heightened reactive oxygen species generation in the antimicrobial activity of a three component iodinated chitosan-silver nanoparticle composite. Langmuir 2010;26:5901-8
- Ma Y, Zhou T, Zhao C. Preparation of chitosan-nylon-6 blended membranes containing silver ions as antibacterial materials. Carbohydr Res 2008;343:230-7
- Sanpui P, Murugadoss A, Prasad PV, The antibacterial properties of a novel chitosan-Ag-nanoparticle composite. Int J Food Microbiol 2008;124:142-6
- Chadwick S, Kriegel C, Amiji M. Nanotechnology solutions for mucosal immunization. Adv Drug Deliv Rev 2010;62:394-407
- Friedman AJ, Han G, Navati MS, Sustained release nitric oxide releasing nanoparticles: characterization of a novel delivery platform based on nitrite containing hydrogel/glass composites. Nitric Oxide 2008;19:12-20
- Potara M, Jakab E, Damert A, Synergistic antibacterial activity of chitosan-silver nanocomposites on Staphylococcus aureus. Nanotechnology 2011;22:135101
- Ulrich AS. Biophysical aspects of using liposomes as delivery vehicles. Biosci Rep 2002;22:129-50
- Drulis-Kawa Z, Dorotkiewicz-Jach A. Liposomes as delivery systems for antibiotics. Int J Pharm 2010;387:187-98
- Allen TM. Liposomal drug formulations. Rationale for development and what we can expect for the future. Drugs 1998;56:747-56
- Bakker-Woudenberg IA, Lokerse AF, ten Kate MT, Liposomes with prolonged blood circulation and selective localization in Klebsiella pneumoniae-infected lung tissue. J Infect Dis 1993;168:164-71
- Bakker-Woudenberg IA, Storm G, Woodle MC. Liposomes in the treatment of infections. J Drug Target 1994;2:363-71
- Bakker-Woudenberg IA. Long-circulating sterically stabilized liposomes as carriers of agents for treatment of infection or for imaging infectious foci. Int J Antimicrob Agents 2002;19:299-311
- Pal S, Tak YK, Song JM. Does the antibacterial activity of silver nanoparticles depend on the shape of the nanoparticle? A study of the Gram-negative bacterium Escherichia coli. Appl Environ Microbiol 2007;73:1712-20
- Ghosh S, Kaushik R, Nagalakshmi K, Antimicrobial activity of highly stable silver nanoparticles embedded in agar-agar matrix as a thin film. Carbohydr Res 2010;345:2220-7
- Shahverdi AR, Fakhimi A, Shahverdi HR, Synthesis and effect of silver nanoparticles on the antibacterial activity of different antibiotics against Staphylococcus aureus and Escherichia coli. Nanomedicine 2007;3:168-71
- Ruparelia JP, Chatterjee AK, Duttagupta SP, Strain specificity in antimicrobial activity of silver and copper nanoparticles. Acta Biomater 2008;4:707-16
- Martinez-Gutierrez F, Olive PL, Banuelos A, Synthesis, characterization, and evaluation of antimicrobial and cytotoxic effect of silver and titanium nanoparticles. Nanomedicine 2010;6:681-8
- Ren G, Hu D, Cheng EW, Characterisation of copper oxide nanoparticles for antimicrobial applications. Int J Antimicrob Agents 2009;33:587-90
- Prajapati VK, Awasthi K, Yadav TP, An oral formulation of amphotericin B attached to functionalized carbon nanotubes is an effective treatment for experimental visceral leishmaniasis. J Infect Dis 2012;205:333-6
- Prato M, Kostarelos K, Bianco A. Functionalized carbon nanotubes in drug design and discovery. Acc Chem Res 2008;41:60-8
- Pantarotto D, Briand JP, Prato M, Translocation of bioactive peptides across cell membranes by carbon nanotubes. Chem Commun (Camb) 2004;1:16-17
- Georgakilas V, Kordatos K, Prato M, Organic functionalization of carbon nanotubes. J Am Chem Soc 2002;124:760-1
- Soppimath KS, Aminabhavi TM, Kulkarni AR, Biodegradable polymeric nanoparticles as drug delivery devices. J Control Release 2001;70:1-20
- Zhang L, Pornpattananangku D, Hu CM, Development of nanoparticles for antimicrobial drug delivery. Curr Med Chem 2010;17:585-94
- Santos-Magalhaes NS, Mosqueira VC. Nanotechnology applied to the treatment of malaria. Adv Drug Deliv Rev 2010;62:560-75
- Sosnik A, Carcaboso AM, Glisoni RJ, New old challenges in tuberculosis: potentially effective nanotechnologies in drug delivery. Adv Drug Deliv Rev 2010;62:547-59
- Croy SR, Kwon GS. Polymeric micelles for drug delivery. Curr Pharm Des 2006;12:4669-84
- Huh AJ, Kwon YJ. “Nanoantibiotics”: a new paradigm for treating infectious diseases using nanomaterials in the antibiotics resistant era. J Control Release 2011;156:128-45
- Gillies ER, Frechet JM. Dendrimers and dendritic polymers in drug delivery. Drug Discov Today 2005;10:35-43
- Cheng Y, Qu H, Ma M, Polyamidoamine (PAMAM) dendrimers as biocompatible carriers of quinolone antimicrobials: an in vitro study. Eur J Med Chem 2007;42:1032-8
- Vaks L, Benhar I. In vivo characteristics of targeted drug-carrying filamentous bacteriophage nanomedicines. J Nanobiotechnol 2011;9:58
- Jung J, Matsuzaki T, Tatematsu K, Bio-nanocapsule conjugated with liposomes for in vivo pinpoint delivery of various materials. J Control Release 2008;126:255-64
- Fang J, Nakamura H, Maeda H. The EPR effect: unique features of tumor blood vessels for drug delivery, factors involved, and limitations and augmentation of the effect. Adv Drug Deliv Rev 2011;63:136-51
- Silver LL. Challenges of antibacterial discovery. Clin Microbiol Rev 2011;24:71-109
- Clatworthy AE, Pierson E, Hung DT. Targeting virulence: a new paradigm for antimicrobial therapy. Nat Chem Biol 2007;3:541-8
- Arnusch CJ, Albada HB, van Vaardegem M, Trivalent ultrashort lipopeptides are potent pH dependent antifungal agents. J Med Chem 2012;55:1296-302
- Fjell CD, Hiss JA, Hancock REW, Designing antimicrobial peptides: form follows function. Nature Rev drug Disc 2012;11:37-51
- Manca ML, Manconi M, Valenti D, Liposomes coated with chitosan-xanthan gum (chitosomes) as potential carriers for pulmonary delivery of rifampicin. J Pharm Sci 2012;101:566-75
- Alexander BD, Winkler TP, Shi S, In vitro characterization of nebulizer delivery of liposomal amphotericin B aerosols. Pharm Dev Technol 2011;16:577-82
- Fielding RM, Lewis RO, Moon-McDermott L. Altered tissue distribution and elimination of amikacin encapsulated in unilamellar, low-clearance liposomes (MiKasome). Pharm Res 1998;15:1775-81
- Magallanes M, Dijkstra J, Fierer J. Liposome-incorporated ciprofloxacin in treatment of murine salmonellosis. Antimicrob Agents Chemother 1993;37:2293-7
- Omri A, Suntres ZE, Shek PN. Enhanced activity of liposomal polymyxin B against Pseudomonas aeruginosa in a rat model of lung infection. Biochem Pharmacol 2002;64:1407-13
- Onyeji CO, Nightingale CH, Marangos MN. Enhanced killing of methicillin-resistant Staphylococcus aureus in human macrophages by liposome-entrapped vancomycin and teicoplanin. Infection 1994;22:338-42
- Kim HJ, Jones MN. The delivery of benzyl penicillin to Staphylococcus aureus biofilms by use of liposomes. J Liposome Res 2004;14:123-39
- Schumacher I, Margalit R. Liposome-encapsulated ampicillin: physicochemical and antibacterial properties. J Pharm Sci 1997;86:635-41
- Gangadharam PR, Ashtekar DA, Ghori N, Chemotherapeutic potential of free and liposome encapsulated streptomycin against experimental Mycobacterium avium complex infections in beige mice. J Antimicrob Chemother 1991;28:425-35
- Cavalli R, Gasco MR, Chetoni P, Solid lipid nanoparticles (SLN) as ocular delivery system for tobramycin. Int J Pharm 2002;238:241-5
- Pandey R, Khuller GK. Solid lipid particle-based inhalable sustained drug delivery system against experimental tuberculosis. Tuberculosis (Edinb) 2005;85:227-34
- Sanna V, Gavini E, Cossu M, Solid lipid nanoparticles (SLN) as carriers for the topical delivery of econazole nitrate: in-vitro characterization, ex-vivo and in-vivo studies. J Pharm Pharmacol 2007;59:1057-64
- Jain D, Banerjee R. Comparison of ciprofloxacin hydrochloride-loaded protein, lipid, and chitosan nanoparticles for drug delivery. J Biomed Mater Res B Appl Biomater 2008;86:105-12
- Tsiolis P, Giamarellos-Bourboulis EJ, Mavrogenis AF, Experimental osteomyelitis caused by methicillin-resistant Staphylococcus aureus treated with a polylactide carrier releasing linezolid. Surg Infect (Larchmt) 2011;12:131-5
- Espuelas MS, Legrand P, Loiseau PM, In vitro antileishmanial activity of amphotericin B loaded in poly(epsilon-caprolactone) nanospheres. J Drug Target 2002;10:593-9
- Toti US, Guru BR, Hali M, Targeted delivery of antibiotics to intracellular chlamydial infections using PLGA nanoparticles. Biomaterials 2011;32:6606-13
- Ungaro F, d'Angelo I, Coletta C, Dry powders based on PLGA nanoparticles for pulmonary delivery of antibiotics: modulation of encapsulation efficiency, release rate and lung deposition pattern by hydrophilic polymers. J Control Release 2012;157:149-59
- Valizadeh H, Mohammadi G, Ehyaei R, Antibacterial activity of clarithromycin loaded PLGA nanoparticles. Pharmazie 2012;67:63-8
- Forestier F, Gerrier P, Chaumard C, Effect of nanoparticle-bound ampicillin on the survival of Listeria monocytogenes in mouse peritoneal macrophages. J Antimicrob Chemother 1992;30:173-9
- Turos E, Shim JY, Wang Y, Antibiotic-conjugated polyacrylate nanoparticles: new opportunities for development of anti-MRSA agents. Bioorg Med Chem Lett 2007;17:53-6
- Turos E, Reddy GS, Greenhalgh K, Penicillin-bound polyacrylate nanoparticles: restoring the activity of beta-lactam antibiotics against MRSA. Bioorg Med Chem Lett 2007;17:3468-72
- Abeylath SC, Turos E, Dickey S, Glyconanobiotics: Novel carbohydrated nanoparticle antibiotics for MRSA and Bacillus anthracis. Bioorg Med Chem 2008;16:2412-18
- Suri SS, Fenniri H, Singh B. Nanotechnology-based drug delivery systems. J Occup Med Toxicol 2007;2:16
- Ma M, Cheng Y, Xu Z, Evaluation of polyamidoamine (PAMAM) dendrimers as drug carriers of anti-bacterial drugs using sulfamethoxazole (SMZ) as a model drug. Eur J Med Chem 2007;42:93-8
- Mishra MK, Kotta K, Hali M, PAMAM dendrimer-azithromycin conjugate nanodevices for the treatment of Chlamydia trachomatis infections. Nanomedicine 2011;7:935-44
- Bhadra D, Bhadra S, Jain NK. Pegylated lysine based copolymeric dendritic micelles for solubilization and delivery of artemether. J Pharm Pharm Sci 2005;8:467-82