Abstract
We review current understanding of dynamic interactions between environmental variables and stream benthic communities within glacierized alpine catchments to provide a context for the central theme of habitat complexity within alpine streams. We present a conceptual model summarizing the important links between environmental variables, from large-scale (regional/catchment) to stream-reach/patch-scale processes, to illustrate this physical habitat complexity. Existing alpine stream classification and zonation criteria are examined, and the environmental characteristics representative of the different stream classes are identified. The theme of habitat complexity is developed to assess the applicability of traditional (principally temperature-based) alpine stream classifications. These traditional classifications do not take into account spatial and temporal variations in water source contributions to alpine streams unless associated temperature changes occur. However, different hydrological stores and pathways impart other physical and chemical influences upon stream benthic communities that are overlooked by traditional classifications. We propose a new classification system to better describe spatial and temporal variability in glacial, snowmelt, and groundwater inputs to alpine streams, based upon the mix of proportions of water contributed from each of these sources. Field data collected in the French Pyrénées are used to support this new alpine stream classification, which we propose as a tool for further research in alpine river catchments.
Introduction
Alpine river systems are fed by glacial icemelt, snowmelt, and groundwater (CitationMilner and Petts, 1994; CitationWard, 1994; CitationMalard et al., 1999; CitationWard et al., 1999; CitationFüreder et al., 2001). Although streams originating from these water sources share common features, such as steep gradients, high flow velocities, and high dissolved oxygen concentrations, each source produces a characteristic discharge regime and a distinctive suite of physical and chemical characteristics (CitationMilner and Petts, 1994; CitationWard, 1994; CitationTockner et al., 1997, Citation2002; CitationFriberg et al., 2001). Furthermore, the distribution of snow, ice, and groundwater springs varies spatially from stream-reach to catchment scale, resulting in stream segments with characteristics reflecting the different proportions of runoff sources (CitationBrittain and Milner, 2001). Physical variables (e.g., water temperature, channel stability, suspended sediment concentration) influence streams over time-scales ranging from diel to millennial (CitationSmith et al., 2001), adding further complexity to abiotic mechanisms controlling the ecological processes in alpine streams.
Many aspects of the hydrology and physicochemical features of glacier-fed streams are relatively well studied, especially in Europe (CitationMilner and Petts, 1994); in contrast, knowledge of the ecology of these systems has been relatively poor until recently (e.g., CitationBrittain and Milner, 2001; CitationLods-Crozet et al., 2001, CitationSnook and Milner, 2001; CitationZah et al., 2001). Recent work undertaken within a number of glaciated catchments in Europe as part of a coordinated project (Arctic and Alpine Stream Ecosystem Research [AASER]) has greatly enhanced understanding of alpine stream ecology (see CitationBrittain and Milner, 2001; CitationMilner et al., 2001). Importantly, this work illustrated that a range of spatiotemporal patterns in environmental conditions markedly affect the composition of benthic stream communities. Currently, the classification of alpine streams is predominantly based upon water source and temperature (e.g., CitationSteffan, 1971; CitationWard, 1994), although some studies refer to habitat conditions, including turbidity and flow regimes (CitationWard, 1994). However, the dynamic nature of water source contributions in alpine catchments results in a greater amount of stream physical habitat variation than these traditional classifications account for. A fuller understanding of the cascade of environmental processes involved in determining benthic community assemblages is required if these unique alpine ecosystems are to be utilized as indicators of climate change (e.g., CitationMcGregor et al., 1995).
This paper identifies the range of environmental variables that affect alpine stream communities. We then review recent advances in understanding the dynamic functioning of alpine water sources and the interrelationships between environmental variables and their roles in determining benthic communities in alpine streams. We examine the traditional classifications of alpine streams into “glacial,” “snowmelt,” and “groundwater” categories based upon water temperatures and critically evaluate the suitability of this approach. The paper's central theme of habitat complexity is developed with the major linkages between environmental variables and benthic communities highlighted through a conceptual model. We propose an alternative classification scheme with the aim of providing a basis for a more complete understanding of the dynamic nature of water store contributions, along with the physical and chemical properties they impart upon stream communities. Field data collected in the French Pyrénées are used to support this new classification scheme, which we put forward as a tool for further research in alpine river catchments.
Traditional Classification and Zonation Schemes for Alpine Streams
Since stream character in alpine catchments is strongly influenced by local water source and the origin of major tributaries (CitationFüreder, 1999), three general stream biotypes (kryal, rhithral, and krenal) have been designated based upon their water source which determines characteristic stream temperature ranges for these biotopes. These temperature ranges then play a major role in defining faunal distributions (e.g., CitationSteffan, 1971; CitationWard, 1994). Glacier-melt-dominated (kryal) streams occur at high altitude close to the glacier terminus and have the lowest water temperatures. Kryal streams are usually subdivided into an upper metakryal and lower hypokryal, reflecting contrasting faunal compositions: Diamesa dominated and predominantly Diamesinae/Orthocladiinae, respectively (CitationSteffan, 1971). Groundwater (krenal) streams are typified by relatively constant water temperature. Rhithral, or seasonal snowmelt-fed, streams are characterized by conditions intermediate between kryal and krenal (CitationWard, 1994).
In alpine regions, these zonations are generally applied to headwater streams that lie between the permanent snowline and the treeline (CitationBrittain and Milner, 2001). In undisturbed environments, the treeline, which forms the divide between subalpine/subarctic woodland and treeless alpine/tundra areas, approximates the 10°C July isotherm (CitationRemmert, 1980). Snowfields may be present year-round at higher altitudes. In some areas, glaciers (e.g., CitationBrittain et al., 2001; CitationRobinson et al., 2001) and glacier-melt-dominated streams (e.g., CitationBurgherr and Ward, 2001; CitationZah et al., 2001) actually extend through the treeline, and in such situations the stream classifications are usually still applied.
Alpine drainage basins containing water from multiple sources are complex systems. Water sources such as glaciers, snow, and groundwater act as hydrological stores within which flows are attenuated; sediment can be eroded, transported and deposited; energy exchanges can affect water temperature; and physicochemical reactions and the mixing of water from various sources can result in water quality variations (CitationSmith et al., 2001). Consequently, stream communities have to exist within, and respond to, a range of dynamic physical processes within alpine stream systems. These physical factors control both the structure and functioning of alpine stream communities. A summary of the environmental variables that contribute to the dynamic nature of alpine streams and their effect upon benthic stream communities is provided in .
KRYAL (GLACIAL STREAMS)
Glacial streams are characteristically very cold, with stream temperatures close to 0°C where meltwater emerges from the glacier snout (CitationMilner and Petts, 1994; CitationWard, 1994). Water temperature ranges are only a few degrees Celsius in kryal reaches due to their proximity to glaciers (reaching a maximum of 4°C: CitationFüreder, 1999) but typically show diel and seasonal fluctuations largely as a result of solar heating. The discharge from glaciers reaches a peak in summer, when energy input is at a maximum (CitationHannah et al., 1999). Diel flow variations of glacial rivers, with peak flow in late afternoon, are a characteristic feature during the ablation season, reflecting cycles of incoming solar radiation and glacier melt (CitationChurch, 1987; CitationRöthlisberger and Lang, 1987; CitationHannah et al., 2000a).
During summer melt periods, glacial rivers often carry high concentrations of fine sediment that can reach over 500 mg L−1 at peak flows (CitationGurnell, 1987). Proglacial river channels are usually highly unstable and characterized by frequent channel-shifting disturbances (CitationMilner and Petts, 1994). Over a range of time scales, interactions between glacier mass balance, sediment load, and riparian vegetation result in a wide variety of proglacial river channel forms (CitationHickin, 1993; CitationGurnell et al., 1999). Ca2+, HCO3 − and SO4 2− usually dominate bulk glacial outflow in varying proportions (CitationRaiswell, 1984; CitationTranter et al., 1993; CitationHodson et al., 2002) along with lesser quantities of Mg2+, Na+, and K+ (CitationFüreder et al., 2001). This solute dominance is due predominantly to subglacial drainage processes and bedrock type acting as key controls on weathering (CitationBrown et al., 1996)
RHITHRAL (SNOWMELT STREAMS)
In the Northern Hemisphere, snowmelt-dominated regimes generally show peak discharge in spring before maximum glacial runoff occurs, although at high altitudes, the peak may be delayed until as late as July (CitationMilner and Petts, 1994). A receding snowline and shrinking snowpacks through late spring and early summer reduce the influence of snowmelt on alpine stream hydrology (CitationSmith et al., 2001). Snowmelt streams typically exhibit the widest temperature range of all alpine stream types, with maximum temperatures reaching between 5 and 10°C (CitationWard, 1994). As snow cover and snowmelt generation can differ markedly over small spatial scales (CitationMarsh, 1999), the influence of meltwater on different stream sections will vary accordingly. Normally, snowmelt-dominated streams are very clear and transport little sediment. However, during high flows they may have elevated turbidity due to resuspension of fine sediments from the streambed (CitationMilner and Petts, 1994).
Peaks in stream solute load occur during snowmelt as a result of preferential elution of ions from snowpacks (e.g., CitationJohannessen and Henriksen, 1978; CitationTranter et al., 1987; CitationHelliwell et al., 1998). High concentrations of nitrogen (as nitrate-N) characterize spring snowmelt runoff (CitationRobinson et al., 2001; CitationHodson et al., 2002). The ionic composition of snowmelt also varies throughout the year, with winter samples being relatively dilute compared with samples collected in spring that may be enriched with Ca2+ and HCO3 − (CitationMalard et al., 1999).
KRENAL (GROUNDWATER STREAMS)
Water temperatures at the sources of high-altitude groundwater streams are related to annual mean air temperature and typically vary by only 1–2°C over an annual cycle (CitationWard, 1994; CitationFüreder, 1999). However, CitationFüreder et al. (2001) found that seasonal mean water temperature varied from <0.1–4.6°C in the groundwater spring-fed Königsbach stream, Austrian Central Alps. Increased discharge from groundwater springs is thought to result in lower daily temperature fluctuations downstream while also extending the distance of the groundwater's stabilizing influence.
In contrast to kryal and rhithral streams, spring-fed streams do not exhibit marked diel and seasonal flow fluctuations (CitationWard, 1994). However, in karstic limestone systems, spring discharge may respond quickly to extreme precipitation events due to the relatively short residence time of water in the aquifer (CitationFord, 1993). Some groundwater streams in the French Pyrénées flowed intermittently toward the end of the melt season (CitationSmith, 1999), following groundwater recharge by meltwaters. Thus, all alpine groundwater streams may not be characterized by predictable flow regimes.
Ionic enrichment and specific conductance of groundwater is often much greater than in either rhithral or kryal streams (e.g., CitationMilner and Petts, 1994; CitationWard, 1994; CitationWard et al., 1999), with relatively high concentrations of silica being characteristic of spring-fed streams (e.g., CitationMalard et al., 1999; CitationSmith, 1999; CitationWard et al., 1999). Annual variability in water chemistry of spring-fed streams is also much lower than in rhithral or krenal streams (CitationFüreder et al., 2001). Nevertheless, large differences may exist in the chemical composition of different groundwater streams both within (e.g., CitationMalard et al., 1999; CitationWard et al., 1999) and between catchments (e.g., CitationSabater and Roca, 1990) due to variations in rock-weathering reactions brought about by differences in geology and groundwater residence time.
The Applicability of Traditional Alpine Stream Zonation
Wide spatiotemporal variability in water store inputs contributes to the uniqueness of alpine stream systems (CitationSmith et al., 2001). To understand how this variability may affect the physical characteristics of streams and how, in turn, these characteristics interact to determine the structure of benthic communities, it is essential to consider the range and scales of links between the physical environment and stream ecosystems. A conceptual model summarizing these interactions is presented in . It is apparent that a range of environmental variables may dynamically shape the characteristics of alpine streams, interacting to produce a variety of habitats within which benthic communities exist.
Regional climatic influences play a major role in alpine stream dynamics by supplying and removing mass and energy at the glacier and snowpack surface, thereby determining accumulation and ablation rates of snow and ice (CitationHannah et al., 1999). This process drives variation in snow and ice meltwater inputs to glacial streams throughout the year. The timing and volume of bulk meltwater production (CitationHannah and Gurnell, 2001), along with inputs of groundwater from springs, seeps, and upwellings (CitationWard et al., 1999; CitationFüreder et al., 2001; CitationLafont and Malard, 2001; CitationMalard et al., 2001) result in wide spatiotemporal variation in the physicochemical properties of streams. The dynamic nature of inputs from all these sources may have a significant impact on benthic communities in alpine streams at the stream-reach scale (CitationSnook and Milner, 2001).
Water temperature has long been recognized as a major contributor to stream community structure and functioning (CitationHynes, 1970; CitationVannote and Sweeney, 1980; CitationVannote et al., 1980; CitationSweeney, 1984; CitationWard, 1985). In glacial streams, water temperature plays a primary role in structuring benthic communities (CitationMilner and Petts, 1994; CitationMilner et al., 2001). At the level of individual organisms, water temperature controls growth rates, the timing of life cycles, and rates of primary and secondary production (CitationHynes, 1970; CitationAllan, 1995). In alpine streams, water sources are the primary influence on water temperature, although climatic influences such as solar radiation input can also play an important role. Because stream water temperature may be influenced by factors other than the source, classifying streams on the basis of temperature alone is problematic. In addition, water sources impart a range of other physical and chemical properties that are discussed by current classification approaches (e.g., CitationWard, 1994) but rarely used in practice.
Even where water temperature is suitable, channel and bed stability may still inhibit colonization of certain taxa (CitationMilner and Petts, 1994). For this reason, stability must be considered in conjunction with water temperature when evaluating community composition of glacial rivers (CitationMilner and Petts, 1994; CitationMilner et al., 2001). Channel stability is related to channel slope, substratum composition, and stream power (CitationResh et al., 1988). The increased sediment loads that accompany high flows, and that are characteristic of glacial streams (CitationGurnell, 1987, Citation1995), may directly affect stream communities through abrasion and reduced light penetration (CitationCastella et al., 2001; CitationMilner et al., 2001). Stream communities are also affected indirectly by the reworking of channel profiles (CitationStatzner et al., 1988). Riparian vegetation can also indirectly affect stream communities by restricting local morphological and sedimentological change (CitationGurnell et al., 1999).
Inputs of allochthonous organic matter to streams (delivered by aerial inputs, erosion, or exfiltrating groundwater: e.g., CitationZah et al., 2001) act as an important energy source for benthic macroinvertebrates (CitationCummins et al., 1973; CitationAnderson and Sedell, 1979; CitationBird and Kaushik, 1981), and can influence their distribution and abundance (CitationVannote et al., 1980; CitationWard, 1992; CitationAllan, 1995). Although inputs of vegetation to streams act primarily as a food source, they also contribute to the nature of the substratum (CitationMinshall, 1984). The substratum consists of both inorganic (microscopic silts to boulders) and organic materials (bryophytes and land-derived vegetation) and forms the stage upon which aquatic insects move, rest, find shelter, and seek food (CitationMinshall, 1984). In alpine streams, deposits of suspended sediment affect the nature of the substratum. The substratum is also a key control on channel and bed stability (CitationPfankuch, 1975). The ability of an insect to adhere, cling, burrow, or build cases is directly affected by the nature of the substratum (CitationMackay, 1977; CitationMinshall, 1984). The substratum may indirectly affect the composition of benthic communities through alteration of the direction, force, and turbulence of water flows (CitationNewbury, 1984). These flow characteristics influence the metabolism, feeding, and behavior of lotic organisms (CitationStatzner et al., 1988).
The availability of ions from the weathering of rocks and soils along with atmospheric inputs influences the water chemistry of streams, which, in turn, may play an important role in determining benthic community composition (CitationHynes, 1970; CitationSabater and Roca, 1990). In alpine catchments, little research has been undertaken to determine specifically the influence of water chemistry on stream communities (CitationKownacka and Kownacki, 1972; CitationSabater and Roca, 1990; CitationRobinson et al., 2001). Water analyses have confirmed that some groundwater streams have distinctive chemical characteristics that vary spatially and temporally (CitationSabater and Roca, 1990; CitationRobinson et al., 2001). Such chemical variability is likely to result in habitat differences and thus to have a strong effect on benthic community composition (CitationSabater and Roca, 1990; CitationRobinson et al., 2001).
Given the occurrence of high spatiotemporal variability in physical habitat in alpine streams, the traditional classification system using water temperature alone to indicate water source is misleading. For example, a stream fed only by glacial meltwater is classified as kryal in its uppermost reaches (CitationWard, 1994). As water flows downstream, increases in temperature occur as a result of advective heat transfer and solar heating (CitationFüreder, 1999), leading to the classification of the stream as rhithral, even though it may have no true snowmelt influence. As such, the current classification system of kryal, rhithral, and krenal may be misleading due to the conflation of water source and water temperature. highlights the problem of using stream temperature to classify stream reaches. These data were collected on a glacial stream in the French Pyrénées over the 1997 melt season (see CitationSmith et al., 2001, for methodology details). Water temperature was consistently below 4°C (kryal) at the beginning of the melt season, when snowmelt was the major contributor to stream flow. As the melt season progressed, the temperature range increased due to a greater receipt of solar radiation input, so that use of the traditional classification method would result in a rhithral classification despite the fact that snowmelt contribution was decreasing and glacial icemelt increasing (CitationSmith, 1999).
The term “kryal” was used in a study of streams fed by a permanent snowfield but no glacier (CitationElgmork and Saether, 1970). In this study, five stream reaches were investigated in the Colorado Rocky Mountains between a snowfield and the first of a series of lakes. All five study sites had midsummer temperatures of approximately 1°C, and as such were deemed to be an example of kryal streams (CitationWard, 1994). In this situation, streams fed completely by snowmelt should be placed into a modified rhithral category, which would correctly reflect the origin and physical characteristics of these meltwaters.
A further example of the way in which traditional alpine stream categories are problematic is that outlets from cirque lakes have been classified as rhithral (e.g., CitationElgmork and Saether, 1970). Lakes may significantly alter the nature of headwaters by increasing channel stability downstream, modifying chemical characteristics, or increasing water temperature (CitationMilner and Petts, 1994) to such an extent that the streams draining them are markedly different from those supplying the lake. Even though the water temperature of snowmelt streams may be similar to those of lake outlets, other physical characteristics may be markedly different enough to require a separate classification.
The use of the term “rhithral” has also been questioned with reference to its application to precipitation as well as snowmelt-fed streams (e.g., CitationIllies, 1961; CitationIllies and Botosaneanu, 1963; CitationWard, 1994). More recently, the terms “ombral” and “chial” have been proposed to describe rainfall- and snowmelt-fed streams, respectively (CitationSchütz, 1999; CitationLencioni, 2000). However, even this division is problematic because streams are rarely influenced by a single water source. Snowmelt may flow overland into glacial- or groundwater-fed streams; precipitation may also fall directly (typically <1% contribution) or flow overland or through hillslopes into streams. In these situations, the stream would still be classified by its temperature. In a similar manner, spring-fed tributaries may markedly increase the temperature of kryal streams below confluences (e.g., CitationWard, 1994). Therefore, a stream reach downstream of a groundwater input to a glacial stream could be classified as rhithral if thermal conditions were substantially altered, regardless of the absence of meltwater from snowpacks.
In streams close to glacial margins, the subdivision of the kryal into an upper metakryal and lower hypokryal is also problematic. Snowpacks cover the ablation zone of glaciers, melting upglacier over the summer (CitationHannah et al., 2000a), and their meltwaters may flow into the proglacial zone, resulting in a mixed water source. However, as temperature is the principal physical variable used as a classification criterion, little account is taken of the influence of the snowmelt contributions.
The quantity and quality of water source inputs to alpine streams may also vary over a number of temporal scales from diel to intraannual (). This variety can result in changes in stream classification over an annual cycle. For example, springtime flows may be predominantly snowmelt driven. In summer, glacier melt often dominates, while toward the end of the melt season and through winter, groundwater inputs may be the principal water source for streams (CitationMalard et al., 1999; CitationSmith et al., 2001). Similarly, changes in water routing can occur diurnally. Groundwater sources may dominate stream channels at low flows through the late evening and night, when glacial icemelt or snowmelt is minimal. As solar radiation increases toward an afternoon peak, the proportion of snow- and icemelt increases, and streams shift to be either snowmelt or icemelt dominated. The current system does not take these temporal shifts into account unless they are accompanied by marked changes in water temperature.
An Alternative Approach to Alpine Stream Classification
Given the level of inconsistency in classifying alpine streams using traditional approaches, we suggest that future studies should concentrate on determining the actual relative contributions of different water sources rather than assigning streams to broad categories based upon their principal water source and associated temperature regime. Hence, we propose nine new categories for classification based upon the relative proportions of glacial meltwater (kryal), snowmelt (nival), and groundwater (krenal) present (). A greater number of categories, giving qualitative explanations of the stream type as determined by source waters alone, may improve comparisons of stream reaches between studies and allow changes in source waters within alpine catchments to be tracked over temporal scales ranging from diel to seasonal. A simple triangular diagram () to illustrate the proportion of source waters to a stream reach allows ease of classification.
The classification principally aims to categorize streams based on their relative proportions of source waters. Variations in environmental characteristics can be predicted within the nine categories, providing better resolution (). Characteristic temperature ranges for each category are not included due to the complicating influences of climate and distance from the source. The important role of water temperature in alpine streams is acknowledged, but its use as a primary classification tool is severely limited, particularly when water temperature is equated with water sources. However, to aid comparison between studies, water temperature ranges should be stated alongside the new classification categories to facilitate proper interpretation of habitat characteristics within a stream reach and so enable comparison between studies.
The new classification system accounts only for stream conditions between precipitation events, which is a limitation. However, given that precipitation events often provide volumes of water that exceed those from other sources (CitationMatthaei et al., 1997), their contribution should be evident from the examination of stream hydrographs (CitationHannah et al., 1999, Citation2000b). Nevertheless, since precipitation events are usually episodic, they are likely to result in relatively short-term deviations from the conditions described by a classification. However, it is important to acknowledge these short-term deviations because peak flows caused by precipitation events are likely to have an effect on stream communities (e.g., CitationMatthaei et al., 1997).
A Test Application of the Alternative Alpine Stream Classification
The new classification has been tested using data collected from a small alpine glacierized catchment (Taillon-Gabiétous) within the Cirque de Gavarnie region, French Pyrénées. The catchment contains two cirque glaciers (Taillon and Gabiétous), large valley snowpacks, and a karst groundwater system that contributes a number of springs to the lower catchment. The maximum altitude is 3144 m, comprising a catchment area of 6.4 km2 at the farthest downstream point sampled. Permanent snow and ice cover within the catchment is 5.4% (CitationSmith et al., 2001).
Temporal changes in the percentage of source water contributions to stream flow for the Taillon-Gabiétous catchment, 1997 ablation season (CitationSmith, 1999), are shown in . Close to the Taillon Glacier margin, the stream is predominantly classified as Nivo-kryal, reflecting the large input of snow and glacial meltwaters to the proglacial stream. Toward the end of the ablation season, the proportion of snowmelt input to the stream decreases to less than 20%, icemelt waters become dominant, and the classification becomes Kryo-nival. These subtle changes, which may be of importance to benthic communities, have not been documented previously since water temperature was always <2°C and the stream reach was classified simply as kryal throughout the melt season (CitationSnook and Milner, 2001). Other physical variables, notably diel discharge fluctuations and suspended sediment concentration also varied as water sources changed (CitationSmith et al., 2001). Classification approaches based principally upon temperature do not adequately describe this variability.
The classification may also be used to describe changes in streams with distance from glacier margins. shows proportions of source water contributions to the Taillon Glacier stream, averaged over the 1997 ablation season (for site descriptions, refer to CitationSnook and Milner, 2001). At the glacier margin (Site 1) and at Site 2, the stream is classified as Kryo-nival, highlighting the mix of waters from the snow-covered glacier. The classification changes to Nivo-kryal as distance from the glacier increases, reflecting the increased proportion of snowmelt input as the catchment area upstream of the stream reach increases and the percentage covered by glacier decreases. Between Sites 3 and 5 (Site 4 was located on a side tributary), approximately 1 km downstream of the Taillon Glacier, a number of groundwater springs enter the Taillon stream, thus accounting for the change in classification to Kreno-nival. These two examples illustrate the spatiotemporal variability in water source contributions that may be identified using the alternative classification. In comparison, traditional classification approaches would not identify this dynamism. Further evaluation is required to determine the effectiveness of this alternative classification system, in describing source water dynamics over longer time periods (interannually). Testing is also required to establish whether the approach adequately describes variations in source water inputs and the physicochemical characteristics of other alpine glacierized catchment streams.
Summary
Stream-reach characteristics in alpine catchments are strongly influenced by water source. Classifications based predominantly upon water temperature are problematic since the conflation of water source and water temperature is often inaccurate. Furthermore, alpine streams exhibit wide spatial and temporal variation in a range of physical and chemical characteristics, both within and between streams, which is largely unaccounted for by current classification approaches.
Even within the relatively small Taillon-Gabiétous catchment, temporal and spatial changes in water sources are evident throughout the melt season, and therefore, alternative stream classifications occur. In a much larger catchment (Val Roseg, Switzerland), groundwater contributions have been shown to vary from <10% to >70% over the course of an ablation season (CitationTockner et al., 2002), potentially leading to much greater variability in stream classification than is shown in this paper for the headwater Taillon-Gabiétous catchment.
Further examination of temporal changes in stream classification may yield important information on associated changes in stream physical and chemical characteristics, which, in turn, may be important determinants in the timing of life cycles of aquatic insects and benthic macroinvertebrate community structure. Water temperature and channel stability are recognized as fundamental stream characteristics influencing benthic communities of alpine streams at the reach scale (CitationMilner and Petts, 1994; CitationMilner et al., 2001). However, recent work has highlighted the roles of other environmental variables, such as hydrochemistry, suspended sediments, and particulate organic matter, in determining alpine stream community structure (e.g., CitationCastella et al., 2001; CitationLods-Crozet et al., 2001; CitationMilner et al., 2001). Although it may be argued that these variables are less important than water temperature and channel stability, further work is necessary to quantify their influence, especially at smaller spatial scales (i.e., patch) where their relative importance may be higher. This classification provides a framework for future research that should provide a more refined resolution for understanding dynamic changes in alpine stream physical characteristics and benthic communities.
FIGURE 1. A conceptual model of the environmental variables influencing benthic communities in alpine streams at different spatial scales
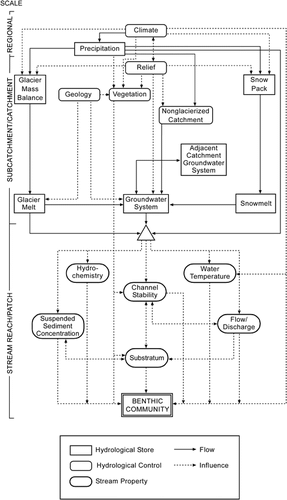
FIGURE 2. Time series of stream water temperature and discharge approximately 1 km downstream of the Taillon Glacier snout, French Pyrénées, for the 1996 ablation season
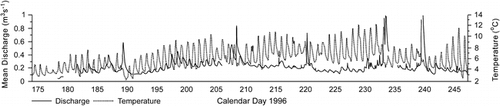
FIGURE 3. A conceptual model of spatial and temporal variations in daily bulk runoff hydrographs and source water contributions in alpine glacierized catchments over an annual cycle (modified from CitationSmith et al., 2001)
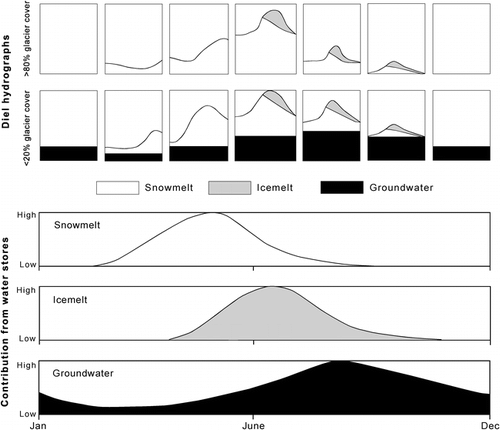
FIGURE 4. Classification of alpine streams based on percentage contribution of source water inputs (refer to for stream categories A–I)
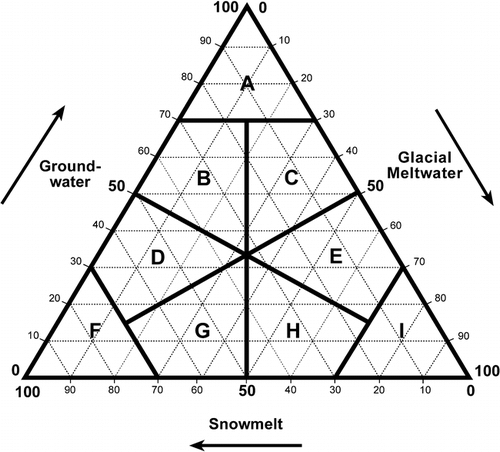
FIGURE 5. Temporal variations in source water contributions to stream flow at the Taillon Glacier snout (altitude 2500 m, catchment area 0.365 km 2 ) throughout the 1997 ablation season. Proportions of each source were estimated using End-Member Mixing Analysis (CitationSmith, 1999). Numbers refer to Julian Day, 1997
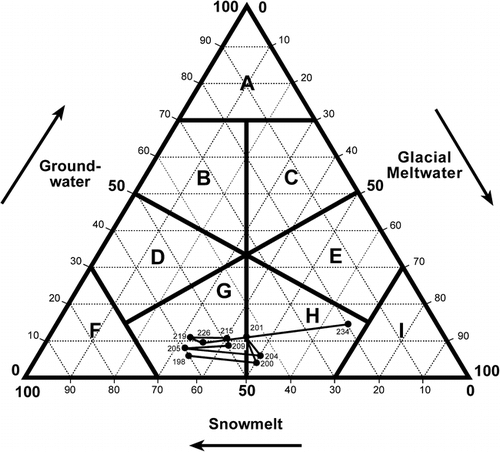
FIGURE 6. Longitudinal variations in source water contributions to stream flow downstream of the Taillon Glacier throughout the 1997 ablation season. Proportions of each source were estimated using End-Member Mixing Analysis and averaged over the ablation season (CitationSnook, 2000). Numbers refer to sampling locations (see text)
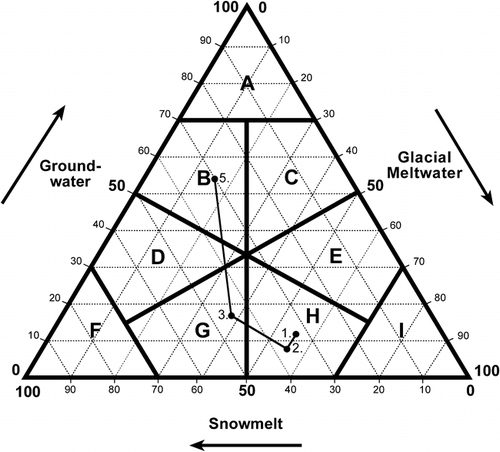
Table 1 Physical and chemical variables contributing to the dynamic nature of the alpine stream environments, with summaries of their influence on benthic communities (modified after CitationMilner and Petts, 1994)
Table 2 Summary of alternative alpine stream classifications based on proportions of water sources (refer to for water source classifications)
Table 3 Characteristic physical and chemical variables expected for alpine stream categories based on relative proportions of source water contributions
Acknowledgments
Lee Brown is funded by a Natural Environment Research Council (NERC) studentship (NER/S/A/2001/05984). We thank the Parc National des Pyrénées for kind permission to undertake fieldwork in the Taillon-Gabiétous catchment. Barnaby Smith provided the stream temperature data presented in . We thank Barnaby Smith and Debbie Snook for access to previously unpublished data from their Ph.D. theses. We are grateful to Andrew Boulton, John Brittain, Christopher Robinson, Urs Uehlinger, Mike Winterbourn, and two anonymous reviewers for comments that enhanced the paper.
References Cited
- Allan, D. J. 1995. Stream Ecology, Structure and Function of Running Waters. London, Chapman and Hall.
- Anderson, N. H. and J. R. Sedell . 1979. Detritus processing by macroinvertebrates in stream ecosystems. Annual Review of Entomology 24:351–377.
- Bird, G. A. and N. K. Kaushik . 1981. Coarse particulate organic matter in streams. In Lock, M. A., and Williams, D. D. (eds.), Perspectives in Running Water Ecology. New York: Plenum Press, 41–68.
- Brown, G. H. , M. Tranter , and M. J. Sharp . 1996. Subglacial chemical erosion-seasonal variations in solute provenance, Haut Glacier d'Arolla, Switzerland. Annals of Glaciology 22:25–31.
- Brittain, J. E. and A. M. Milner . 2001. Ecology of glacier-fed rivers: current status and concepts. Freshwater Biology 46:1571–1578.
- Brittain, J. E. , S. J. Saltveit , E. Castella , J. B. Bogen , T. E. Bonsnes , I. Blakar , T. Bremnes , I. Haug , and G. Velle . 2001. The macroinvertebrate communities of two contrasting Norwegian glacial rivers in relation to environmental variables. Freshwater Biology 46:1723–1736.
- Burgherr, P. and J. V. Ward . 2001. Longitudinal and seasonal distribution patterns of the benthic fauna of an alpine glacial stream (Val Roseg, Swiss Alps). Freshwater Biology 46:1705–1722.
- Castella, E. , H. Adalsteinsson , J. E. Brittain , G. M. Gíslason , A. Lechmann , V. Lencioni , B. Lods-Crozet , B. Maiolini , A. M. Milner , J. S. Ólafsson , S. J. Saltveit , and D. L. Snook . 2001. Macrobenthic invertebrate richness and composition along a latitudinal gradient of European glacier-fed streams. Freshwater Biology 46:1811–1832.
- Church, M. 1987. Floods in cold climates. In Baker, V. R., Kochel, R. C., and Patton, P. C. (eds.), Flood Geomorphology. New York: Wiley, 205–229.
- Cummins, K. W. , R. C. Peterson , F. O. Howard , J. C. Wuycheck , and V. I. Holt . 1973. The utilization of leaf litter by stream detritivores. Ecology 54:336–345.
- Elgmork, K. and O. A. Saether . 1970. Distribution of invertebrates in a high mountain brook in the Colorado Rocky Mountains. University of Colorado Studies, Series in Biology 31:1–55.
- Fenn, C. R. 1987. Electrical Conductivity. In Gurnell, A. M., and Clark, M. J. (eds.), Glacio-fluvial Sediment Transfer. Chichester, UK: Wiley, 377–414.
- Ford, D. C. 1993. Karst in cold environments. In French, H. M., and Slaymaker, O., (eds.), Canada's Cold Environments. Montreal: McGill Queen's University Press, 199–222.
- Friberg, N. , A. M. Milner , L. M. Svendsen , C. Lindegaard , and S. E. Larsen . 2001. Longitudinal distribution of macroinvertebrates in two glacier-fed New Zealand rivers. Freshwater Biology 46:1753–1764.
- Füreder, L. 1999. High alpine streams: cold habitats for insect larvae. In Margesin, R., and Schinner, F. (eds.), Cold Adapted Organisms: Ecophysiology, Enzymology and Molecular Biology. Berlin: Springer-Verlag, 181–196.
- Füreder, L. , C. Schütz , M. Wallinger , and R. Burger . 2001. Physico-chemistry and aquatic insects of a glacier-fed and a spring-fed alpine stream. Freshwater Biology 46:1673–1690.
- Gurnell, A. M. 1987. Suspended sediment. In Gurnell, A. M., and Clark, M. J. (eds.), Glacio-fluvial Sediment Transfer. Chichester UK, John Wiley, 305–354.
- Gurnell, A. M. 1995. Sediment yield from alpine glacier catchments. In Foster, I. D. L., Gurnell, A. M., and Webb, B. W. (eds.), Sediment and Water Quality in River Catchments. Chichester, UK: John Wiley, 407–435.
- Gurnell, A. M. , P. J. Edwards , G. E. Petts , and J. V. Ward . 1999. A conceptual model for alpine proglacial river channel evolution under changing climatic conditions. Catena 38:223–242.
- Hannah, D. M. , A. M. Gurnell , and G. R. McGregor . 1999. A methodology for investigation of the seasonal evolution in proglacial hydrograph form. Hydrological Processes 13:2603–2621.
- Hannah, D. M. , A. M. Gurnell , and G. R. McGregor . 2000a. Spatio-temporal variation in microclimate, the surface energy balance and ablation over a cirque glacier. International Journal of Climatology 20:733–758.
- Hannah, D. M. , B. P G. Smith , A. M. Gurnell , and G. R. McGregor . 2000b. An approach to hydrograph classification. Hydrological Processes 14:317–338.
- Hannah, D. M. and A. M. Gurnell . 2001. A conceptual linear reservoir runoff model to investigate melt season changes in cirque glacier hydrology. Journal of Hydrology 246:123–141.
- Helliwell, R. C. , C. Soulsby , R. C. Ferrier , A. Jenkins , and R. Harriman . 1998. Influence of snow on the hydrology and hydrochemistry of the Allt a'Mharcaidh, Cairngorm Mountains, Scotland. Science of the Total Environment 217:59–70.
- Hodson, A. , M. Tranter , A. M. Gurnell , M. Clark , and J. O. Hagen . 2002. The hydrochemistry of Bayelva, a high arctic proglacial stream in Svalbard. Journal of Hydrology 257:91–114.
- Hickin, E. J. 1993. Fluvial facies models: a review of Canadian research. Progress in Physical Geography 17:205–222.
- Hynes, H. B N. 1970. Ecology of Running Waters. Liverpool: Liverpool University Press.
- Illies, J. 1961. Versuch einer allgemeinen biozönotischen Gliederung der Flieβgewässer. Internationale Revue der Gesamten Hydrobiologie 46:205–213.
- Illies, J. and L. Botosaneanu . 1963. Problèmes et methods de la classification et de la zonation écologiques des eaux courantes, considerées surtout du point de vue faunistique. Internationale Vereinigung für Theoretische und Angewandte Limnologie Mitteilungen 12:1–57.
- Johannessen, M. and A. Henriksen . 1978. Chemistry of snow meltwater: changes in concentration during melting. Water Resources Research 14:615–619.
- Kownacka, M. and A. Kownacki . 1972. Vertical distribution of zoocenoses in the streams of the Tatra, Caucasus and Balkan Mts. Verhandlungen International Verein. Limnologie 18:742–750.
- Lafont, M. and F. Malard . 2001. Oligochaete communities in the hyporheic zone of a glacial river, the Roseg River, Switzerland. Hydrobiologia 463:75–81.
- Lencioni, V. 2000. Chironimid (Diptera: Chironimidae) assemblages in three alpine glacial systems. Ph.D. thesis. University of Innsbruck, Innsbruck, Austria.
- Lods-Crozet, B. , E. Castella , D. Cambin , C. Ilg , S. Knispel , and H. Mayor-Simeant . 2001. Macroinvertebrate community structure in relation to environmental variables in a Swiss glacial stream. Freshwater Biology 46:1641–1662.
- Mackay, R. J. 1977. Behaviour of Pycnopsyche (Trichoptera:Limnephilidae) on mineral substrates in laboratory streams. Ecology 58:191–195.
- Malard, F. , K. Tockner , and J. V. Ward . 1999. Shifting dominance of subcatchment water sources and flow paths in a glacial floodplain, Val Roseg, Switzerland. Arctic, Antarctic, and Alpine Research 31:135–150.
- Malard, F. , A. Mangin , U. Uehlinger , and J. V. Ward . 2001. Thermal heterogeneity in the hyporheic zone of a glacial floodplain. Canadian Journal of Fisheries and Aquatic Sciences 58:1319–1335.
- Marsh, P. 1999. Snowcover formation and melt: recent advances and future prospects. Hydrological Processes 13:2117–2134.
- Matthaei, C. D. , U. Uehlinger , and A. Frutiger . 1997. Response of invertebrates to natural versus experimental disturbance in a Swiss prealpine river. Freshwater Biology 37:61–77.
- McGregor, G. , G. E. Petts , A. M. Gurnell , and A. M. Milner . 1995. Sensitivity of alpine stream ecosystems to climate change and human impacts. Aquatic Conservation: Marine and Freshwater Ecosystems 5:233–247.
- Milner, A. M. and G. E. Petts . 1994. Glacial rivers: physical habitat and ecology. Freshwater Biology 32:295–307.
- Milner, A. M. , J. E. Brittain , E. Castella , and G. E. Petts . 2001. Trends of macroinvertebrate community structure in glacier-fed rivers in relation to environmental conditions: a synthesis. Freshwater Biology 46:1833–1848.
- Minshall, G. W. 1984. Aquatic insect-substratum relationships. In Resh, V. H., and Rosenberg, D. M. (eds.), The Ecology of Aquatic Insects. New York and London: Praeger Publishers, 358–400.
- Newbury, R. W. 1984. Hydrologic determinants of aquatic insect habitats. In Resh, V. H., and Rosenberg, D. M. (eds.), The Ecology of Aquatic Insects. New York and London: Praeger Publishers, 323–357.
- Pfankuch, D. J. 1975. Stream Reach Inventory and Channel Stability Evaluation. Missoula, MT: U.S. Department of Agriculture Forest Service, Region 1.
- Raiswell, R. 1984. Chemical models of solute acquisition in glacial meltwaters. Journal of Glaciology 30:49–57.
- Remmert, H. 1980. Arctic Animal Ecology. Berlin: Springer-Verlag.
- Resh, V. H. , A. V. Brown , A. P. Covich , M. E. Gurtz , H. W. Li , G. W. Minshall , S. R. Reice , A. L. Sheldon , J. B. Wallace , and R. C. Wissmar . 1988. The role of disturbance in stream ecology. Journal of the North American Benthological Society 7:433–455.
- Robinson, C. T. , U. Uehlinger , and M. Hieber . 2001. Spatio-temporal variation in macroinvertebrate assemblages of glacial streams in the Swiss Alps. Freshwater Biology 46:1663–1672.
- Röthlisberger, H. and H. Lang . 1987. Glacial Hydrology. In Gurnell, A. M., and Clark, M. J. (eds.), Glacio-fluvial Sediment Transfer. Chichester, UK: John Wiley, 207–284.
- Sabater, S. and J. R. Roca . 1990. Some factors affecting distribution of diatom assemblages in Pyrenean streams. Freshwater Biology 24:493–507.
- Saltveit, S. J. , I. Haug , and J. E. Brittain . 2001. Invertebrate drift in a glacial river and its non-glacial tributary. Freshwater Biology 46:1777–1790.
- Schütz, C. 1999. The benthic fauna of high alpine streams. Ph.D. thesis. University of Innsbruck, Innsbruck, Austria.
- Smith, B. P G. 1999. Water source and storage areas within a small alpine catchment in the French Pyrénées. Ph.D. thesis. University of Birmingham, Birmingham, UK.
- Smith, B. P G. , D. M. Hannah , A. M. Gurnell , and G. E. Petts . 2001. A hydrogeomorphological context for ecological research on alpine glacial rivers. Freshwater Biology 46:1579–1596.
- Snook, D. L. 2000. Macroinvertebrate communities in alpine glacier-fed streams: the Taillon catchment in the French Pyrénées. Ph.D. thesis,. University of Birmingham, Birmingham, UK.
- Snook, D. L. and A. M. Milner . 2001. The influence of glacial runoff on stream macroinvertebrate communities in the Taillon catchment, French Pyrénées. Freshwater Biology 46:1609–1624.
- Statzner, B. , J. A. Gore , and V. H. Resh . 1988. Hydraulic stream ecology: observed patterns and potential applications. Journal of the North American Benthological Society 7:307–360.
- Steffan, A. W. 1971. Chironomid (Diptera) biocoenoses in Scandinavian glacier brooks. The Canadian Entomologist 103:477–485.
- Sweeney, B. W. 1984. Factors influencing life-history patterns of aquatic insect. In Resh, V. H., and Rosenberg, D. M. (eds.), The Ecology of Aquatic Insects. New York and London: Praeger Publishers, 56–100.
- Tockner, K. , F. Malard , P. Burgherr , C. T. Robinson , U. Uehlinger , R. Zah , and J. V. Ward . 1997. Physico-chemical characterization of channel types in a glacial floodplain ecosystem (Val Roseg, Switzerland). Archiv für Hydrobiologie 140:433–463.
- Tockner, K. , F. Malard , U. Uehlinger , and J. V. Ward . 2002. Nutrients and organic matter in a glacial river-floodplain system (Val Roseg, Switzerland). Limnology and Oceanography 47:226–277.
- Tranter, M. , P. W. Abrahams , I. Blackwood , T. D. Davies , P. Brimblcombe , I. P. Thompson , and C. E. Vincent . 1987. Changes in streamwater chemistry during snowmelt. In Jones, H. G., and Orville-Thomas, W. J. (eds.), Seasonal Snowcovers, Physics, Chemistry, Hydrology. Dordrecht, Netherlands: D. Riedel Publishing Co., 575–597.
- Tranter, M. , G. H. Brown , R. Raiswell , M. J. Sharp , and A. M. Gurnell . 1993. A conceptual model of solute acquisition by alpine glacial meltwaters. Journal of Glaciology 39:573–581.
- Vannote, R. L. and B. W. Sweeney . 1980. Geographic analysis of thermal equilibria: a conceptual model for evaluating the effect of natural and modified thermal regimes on aquatic insect communities. The American Naturalist 115:667–695.
- Vannote, R. L. , G. W. Minshall , K. W. Cummins , J. R. Sedell , and C. E. Cushing . 1980. The river continuum concept. Canadian Journal of Fisheries and Aquatic Sciences 37:130–137.
- Ward, J. V. 1985. Thermal characteristics of running waters. Hydrobiologia 125:31–46.
- Ward, J. V. 1992. Aquatic Insect Ecology, Vol. 1: Biology and Habitat. New York, Wiley.
- Ward, J. V. 1994. Ecology of Alpine Streams. Freshwater Biology 32:277–294.
- Ward, J. V. , F. Malard , K. Tockner , and U. Uehlinger . 1999. Influence of groundwater on surface water conditions in a glacial floodplain of the Swiss Alps. Hydrological Processes 13:277–293.
- Zah, R. , P. Burgherr , S. M. Bernasconi , and U. Uehlinger . 2001. Stable isotope analysis of macroinvertebrates and their food sources in a glacier stream. Freshwater Biology 46:871–882.