Abstract
Senescence mainly manifests as a series of degenerative changes in the morphological structure and function of the body. Osteoporosis is a systemic bone metabolic disease characterized by destruction of bone microstructure, low bone mineral content, decreased bone strength, and increased brittleness and fracture susceptibility. Osteoblasts, osteoclasts and osteocytes are the main cellular components of bones. However, in the process of aging, due to various self or environmental factors, the body’s function and metabolism are disordered, and osteoporosis will appear in the bones. Here, we summarize the mechanism of aging, and focus on the impact of aging on bone remodeling homeostasis, including the mechanism of ion channels on bone remodeling. Finally, we summarized the current clinical medications, targets and defects for the treatment of osteoporosis.
Introduction
At present, human life expectancy is significantly longer than ever before, the process of aging is still not considered to be healthy; instead, the proportion of an individual’s healthy life expectancy within their total life expectancy remains low. The World Health Organization (WHO) defines healthy ageing as the process of maintaining the functional ability of the elderly to enable them to achieve wellbeing. The incidence of many age-related diseases, including cardiovascular, cerebrovascular, and neurodegenerative conditions, is increasing year by year, bringing about suffering and a great economic burden to many elderly people.Citation1 Moreover, aging has become a primary risk factor of certain chronic and devastating diseases. Therefore, in the pursuit of longevity, attention to aging has become an important part of people’s pursuit of healthy aging process.Citation2 In this review, we will first explain the mechanism of aging, and then focus on the effect of aging on the homeostasis of bone remodeling, and summarize the pathological mechanism of bone remodeling after aging including some recent evidence of ion channels in bone remodeling.
Mechanism of Aging
Aging is considered in the literature to be the main cause of many chronic diseases in the body, yet its mechanism is still unclear. The mechanism of cell senescence can inhibit the proliferation of damaged cells but, with the advancement of age, senescent cells accumulate in various tissues and organs and the substances they secrete may destroy the structure and function of tissues.Citation3 As Berdasco reported, aging is a complex process that leads to complex biological changes in the body and increases an organism’s susceptibility to disease and death.Citation4 Several hypotheses about the mechanism of aging exist at this time. Unraveling the mechanism of aging can explain many age-related diseases and provide theoretical basis for the research and development of anti-aging drugs. Cellular senescence is a basic activity of cells, along with proliferation, differentiation, and apoptosis. Cellular senescence is a sign of aging and the accumulation of senescence cells in senescence tissues is considered to be the driving factor of the senescence process.Citation5 Cell senescence has been shown to consist of three stages—namely, early, complete, and late.Citation6 Here, in the early stage, the response to stress is manifested by morphological and functional changes, while the second stage is the main senescence-associated secretory phenotype (SASP) response. The late stage involves interferon type I reactions and the upregulation of L1 (line-1) retrotransposable elements.Citation7 In general, the aging process encompasses genomic damage and telomere reduction epigenetic changes that alter the protein balance and trigger nutrient perception disorders, mitochondrial dysfunction, stem-cell pool collapse, intercellular communications disorders, and cellular senescence.Citation8
Free Radical Theory and Senescence
Harman, a British molecular biologist, first proposed the free radical theory of aging: he believed that “oxidative stress determines the life expectancy.” Aging is the result of the destruction of cells and tissues by oxygen free radicals in a process closely related to the aging process controlled by the environment, disease, and heredity factors. With aging, the metabolic mechanism maintaining the balance in vivo weakens and the concentration of free radicals increases, facilitating irreversible changes to the biofilm, amino acid chain, and DNA molecular structure and accelerating the aging process. Free radical inhibitors and antioxidants extend cell life. Some people believe that free radicals and their associated oxidants are only part of the stressor. The body can adjust the homeostasis range of stress defense and repair system through adaptive homeostasis and produce the most suitable complex structure for induction and specific requirements of enzyme protection.Citation9
Telomere and Telomerase Theory in Aging
Telomere is a complex of telomere DNA and binding proteins located at the end of chromosomes in eukaryotic cells. Telomerase can regulate the replication of telomeres under specific conditions and keep them short in chromosome replication. Maintaining the stability of the effect of telomerase on the regulation of telomeres is an important consideration in the process of aging. It has been shown that overexpression of telomerase can rejuvenate tissues and prolong life span.Citation10 Telomerase may be a target to treat some senile diseases and even delay senescence. However, DNA damage spreads the consequences of aging throughout the body, and it is the cause of age-related diseases. Some genetic syndromes that encompass defective DNA repair are associated with premature aging.Citation11
Autophagy and Senescence
Autophagy is one of the important regulatory mechanisms in the aging process that can regulate cell growth and realize the homeostasis of substances and energy in cells. Three types of autophagy exist: macrophage autophagy (MA), chaperon-mediated autophagy (CMA), and microautophagy, with MA being the main form among the three. It has been reported that aging leads to decreased autophagy levels. Autophagy can be either nonselective or cargo-specific, with mitochondria-specific autophagy known as mitochondrial autophagy. Increasing evidence supports the critical role of autophagy and mitochondrial autophagy in regulating baseline homeostasis and stress. The decrease in autophagy level leads to the aging of many organs such as bone, muscle and kidney.Citation12 Autophagy theory explains the phenomenon of slow metabolism and homeostasis destruction in the elderly.
Cell Senescence and Epigenetic Mechanisms of Senescence
Epigenetic factors, including histone modification, DNA methylation and microRNA (miRNA) expression, may play a key role in regulating gene expression changes and genomic instability during aging. Epigenetic modifications can regulate gene expression and participate in the occurrence and development of many diseases.Citation8 Moreover, particularly in the mammalian system, global and local changes in DNA methylation, heterochromatin site-specificity of loss and gain, and significant restructuring occur.Citation13 Prior research suggests that cytosine-methylation patterns of cytosine–guanine dinucleotide (located at the CpG site) in the mouse and human genomes change with age.Citation14 On this basis, an epigenetic clock reflecting the CpGs methylation level was establishedCitation15 and used to measure the age of cells in different tissue types; notably, research has suggested its accuracy was significantly higher than that of classical telomere length. This could become a new standard for judging aging.Citation16 DNA methylation plays an important role in maintaining DNA stability, cell proliferation, and senescence. Evidences show that most methylation changes are programmed and occur in subsets of tissue cells during normal aging.Citation17 It has been reported that age-related diseases are often accompanied by a drop in DNA methylation levels. Although DNA methylation clocks were initially considered molecular biomarkers of real age, DNA methylation is now being used as a marker of disease risk.Citation18
Aging Caused by Inflammation
The immune system can recognize and remove senescent cells, while the progenitor cells in the tissue can differentiate to form young cells to replace senescent cells, preventing the spread of harmful cells such as a decaying or potential tumor cell. With the aging of the body, however, cell senescence is accelerated and the immune system slows down the elimination of senescence cells, resulting in more senescence cells remaining in the body. Senescence secretion of proinflammatory cytokines and matrix metal albumin by senescent cells on peripherals is referred to as the SASP. SASP and nuclear factor kappa B overactivate immune system dysfunction and reduce autophagy function and, together with other factors, act on the body to cause inflammatory aging. This provides new ideas for the development of anti-aging drugs. Aging releases many inflammatory factors, such as interleukin-1 and tumor necrosis factors (). Reducing the inflammatory factors that the body releases as it ages may delay aging. There have been reported that the inhibition of nuclear factor kappa B by a drug or substrate led to a trend toward youth on the aging spectrum in aging mice.Citation19
Figure 1 Mechanism of aging.
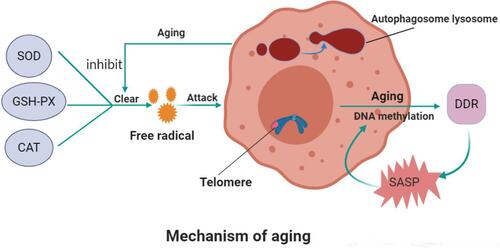
The Epidemiology of Bone Aging and Senile Osteoporosis
Human beings are gradually moving towards an aging society, which has become a consensus. For example, China is facing significant medical challenges brought on by the aging problem; by 2050, the country will have 400 million people older than 65 years, including 150 million over the age of 80 years.Citation20 The 2015 United Nations (UN) report on the ageing of the world’s population claimed that in the next 35 years the number of people aged 60 years or over will more than double to 2.1 billion.Citation21
Osteoporosis is a systemic metabolic bone disease defined by the World Health Organization (WHO). When bone mass is reduced and the microstructure of bone tissue is impaired, it is characterized by fragility and increased fracture susceptibility. With more and more of the population aging, osteoporosis has gradually become a global health problem for people older than 50 years of age. As highlighted earlier, the proportion of the elderly in China is increasing and osteoporosis has become a serious challenge faced by Chinese society.Citation22 The annual number and cost of osteoporosis-related fractures are expected to double by 2035 and increase to 5.99 million cases by 2050 at a cost of $25.43 billion per year.Citation23 At the level of clinical research and medical policy-making, assessing the vulnerability of older individuals contributes to the assessment, management, and decision-making of osteoporosis and osteoporotic fractures.Citation24 Osteoporosis caused by aging has become a social problem that cannot be ignored.
Marrow Stem Cell Depletion in Bone Aging Breaks the Homeostasis of Bone Remodeling
Aging leads to the decline of the function of every tissue in the body and lead to age-related bone dysfunction.Citation8 Bone aging is characterized by massive loss of bone mass and accumulation of bone marrow adipose tissue (BMAT), which is related to the exhaustion and abnormal differentiation of stem cells.Citation25 Mesenchymal stem cells (MSCs) are found widely in the bone marrow and spongy bone and play an important role in maintaining the dynamic balance of bone resorption and formation.Citation26 In older people, the ability of osteoblasts to differentiate and proliferate is reduced, while that of fat cells is increased. This results in accumulation of fat in the bone marrow cavity, which threatens the survival of osteoblasts.Citation27 Abnormal pedigree specification of bone stem cells contributes to decreased bone mass and increased bone marrow adipose tissue in the context of osteoporosis and bone aging. Some studies have suggested that peroxisome proliferator-activated receptor-γ coactivator 1-α (PGC-1α) may act as a key switch that determines the fate of cells. PGC-1α expression levels decrease with age in bone stem cells in both humans and mice; thus, PGC-1α can be a potentially important therapeutic target in the treatment of osteoporosis and bone aging.Citation28 In animal experiments, it was found that miR-188 expression in bone marrow–derived MSCs (BMSCs) significantly increased during the aging process, which resulted in increased conversion of BMSCs to adipocytes and reduced differentiation to osteoblasts, culminating in reduced fat accumulation; bone formation in bone marrow; and, eventually, age-related bone loss and osteoporosis. However, the injection of a miR-188–specific antagonist into the bone marrow cavity reduced the degree of fat accumulation and increased bone formation in the bone marrow of aged mice.Citation29 A peroxisome proliferation-activated receptor is the main regulatory factor of adipogenic differentiation and can inhibit the activity of osteoblasts by blocking the expression of core-binding factor 1, thus playing an important role in the adipogenic differentiation of BMSCs.Citation30 Aging increases the adipogenic differentiation of BMSCs, which may be related to the decrease of PGC-1α and the increase of miR-188. Inhibiting the adipogenic differentiation of BMSCs may be a new way to treat senile osteoporosis.
Telomere dysfunction caused by aging of mesenchymal stem cells may also be another important reason for the imbalance of bone remodeling. Through in vitro culturing of MSCs in a mouse model, Wang et al found that telomere dysfunction could induce senescence and apoptosis in MSCs by stimulating the P53/P21 pathway, downregulating the expression of osteoblastic transcription factor Runx2 in MSCs, and inhibiting the transformation and differentiation of MSCs into osteoblasts, resulting in a reduced bone mass.Citation31 Telomere dysfunction may be one of the causes of senile osteoporosis.
The Accumulation of Oxidative Stressors in Aging Accelerates the Imbalance of Bone Remodeling
A lot of evidence suggests that aging and the associated increase in reactive oxygen species (ROS) are the main culprits of osteoporosis.Citation32 The decrease in estrogen or androgen levels reduces the bone’s defenses against oxidative stress. The accumulation of ROS simultaneously produces and survives osteoclasts, osteoblasts and osteocytes.Citation33–Citation35 Aging can lead to the accumulation of oxidative stress products and the inhibition of bone formation, which may be related to the blocking of the Wnt signal and the transfer of β-catenin from T-cell–specific transcription factors to the transcription-factor binding site mediated by Forkhead box O.Citation32 The important role of advanced protein-oxidation products in many age-related diseases has been widely noted.Citation36 As mentioned above, aging is closely related to free radicals, which can also damage bone cells and reduce bone mass, leading to osteoporosis. Zeng et al found that advanced protein-oxidation products accelerated bone deterioration in elderly rats.Citation37
Decreased Estrogen Levels Caused by Aging Lead to Bone Loss
The ovarian function declines in postmenopausal women, and the decline in estrogen levels is an irreversible fact. Estrogen protects the remodeling homeostasis of adult bones by slowing down the speed of bone remodeling while maintaining the local balance between bone formation and resorption.Citation38,Citation39 Kyunghee’s study found that RANKL caused a decline in ovarian function. Decreased estrogen levels in vivo promote osteoclast differentiation by inducing the phosphorylation of ERK1 and ERK2.Citation40 Experiments have shown that estrogen decline is associated with an increased OPG/RANKL ratio. Estrogen inhibits osteoclasts through OPG/RANKL pathway in early menopause, thus reducing bone loss. Moreover, with greater estrogen doses, local vascular bone formation increases, suggesting that estrogen is related to angiogenic osteoporosis.Citation41 miRNA is a class of uncoded single-stranded RNA commonly found in eukaryotic organisms, with a length of about 21 to 25 nucleotides, which is used for regulatory purposes after radical transcription. The abnormal expression of miRNA is closely related to the occurrence of osteopenia. Zhang found that miR-221 can directly target and inhibit Runx-2–mediated osteoblast differentiation,Citation42 while Chen found that miR-125b inhibited the differentiation and proliferation of bone marrow MSCs by downregulating the osterix expression.Citation43 In addition, miR-145 can inhibit the expression of OPG, while estrogen can increase the expression of OPG by reducing the expression of miR-145.Citation44 Estrogen levels fall as women age, a risk factor for postmenopausal osteoporosis.
Immune System and Its Imbalance with Aging
The clearance of senescent cells is obviously a programmed process in which cellular immunity and humoral immunity participate together.Citation45 Immune senescence refers to a decline in the vitality and precision of the adaptive immune system in old age, mainly due to thymus degeneration and changes in T lymphocyte subsets.Citation46 Furthermore, senescent cells appear to escape immune suppression, such as paracrine suppression of CD8+ cells by natural killer factors and proinflammatory cytokines. RANKL is a member of the tumor necrosis factor superfamily that is secreted by T-cells and B-cells and which binds to the biological receptor RANK on the surface of osteoclasts, thus stimulating the differentiation of osteoclasts into mature multinucleated osteoclasts. B-cells are the main source of OPG in the bone microenvironment. Activated B-cells can also overexpress RANKL. Studies have found that the number of B-cells expressing RANKL in the bone marrow of ovoidless mice is significantly increased, while the loss of RANKL in B-cells can protect from bone loss after ovoidectomy.Citation47 With age, the immune system’s ability to recognize senescent cells decreases, leading to the differentiation and maturation of osteoclasts, leading to osteoporosis.
The decrease in CD4+ T and CD8+ T lymphocytes leads to a decrease in OPG production.Citation48–Citation50 Surface T cells also have anti-osteoclast properties. It shows that T cells play an important role in bone remodeling. In the aging immune system, the decreased diversity of T cells may lead to aging osteoporosis.Citation51
Autophagy and Senile Osteoporosis
Autophagy is a process in which the substrates in the cytoplasm are phagocytic by assembling the membrane structure derived from the cell’s own organelles, such as misfolded or senescent proteins, while damaged organelles are combined with lysosomes to degrade the ingestion phagocytes and recycle them.Citation52 Studies have shown that, as the human body ages, the autophagy level of bone cells gradually decreases, which increases the secretion of proinflammatory factors such as interleukin-1β (IL-1β), accelerates bone loss, and affects bone metabolism, leading to osteoporosis.Citation53 Almeida demonstrated that autophagy can prevent bone loss in bone cells and reduce the apoptosis of bone cells with aging.Citation54 A loss of autophagy may lead to increased dysfunction of bone cells.Citation55 In addition, autophagy induction can lead to the survival response of BMSCs against oxidative stress.Citation56 Studies have shown that BMSCs in osteoporosis patients present a senescent phenotype with significantly lower autophagy levels and significantly reduced osteogenic potential as compared with those in healthy subjects.Citation57 Autophagy plays a role in downregulating oxidative stress in osteoblasts and osteocytes. In a mouse model of osteoblast-specific autophagy, bone loss because of aging and estrogen deficiency worsened significantly.Citation58 Autophagy also regulates the homeostasis of the miRNA network, which can regulate bone formation through the Wnt signaling pathway and bone resorption through the RANKL/RANK pathway.Citation59 In the aging process, osteocyte autophagy is not correlated with osteocyte apoptosis, suggesting that osteocyte autophagy may be related to bone loss. Senescence can cause a decrease of autophagy in cells and the decrease of autophagy in osteoblasts and osteocytes related to senescence is considered to be another hotspot in the theory of osteoporosis. The decreased autophagy activity of bone cells may be one of the causes of bone loss in senile osteoporosis.Citation52
Vitamin D and Senile Osteoporosis
Studies have shown that 1,25-hydroxyvitamin D promotes osteogenic differentiation of human BMSCs. Senescence can downregulate the expression and activity of 1α-hydroxylase in human BMSCs. Parathyroid hormone can promote the activity of 1α-hydroxylase to repair bone defects, and can rejuvenate the osteogenesis of BMSCs in the elderly. Moreover, aging affects the skin’s ability to make vitamin D. Senescence is associated with decreased kidney function, reduced vitamin D receptors, a lack of intestinal responsiveness to active vitamin D, and limitations on calcium absorption, which affects the production of active vitamin D.Citation60
The Role of Ion Channels in Bone Remodeling
The transport of ions across cell membranes can participate in the basic life processes of cells through ion channels.Citation61–Citation65 The process of bone remodeling is finely regulated by various ion channels. For example, the mechanically controlled ion channel Piezo1, Piezo1 is expressed in osteoblasts and osteocytes and regulates mechanical load-dependent bone formation.Citation66–Citation68 Conditional knockout of Piezo1 in osteoblasts and osteocytes will result in a significant reduction in bone mass and damage to bone structure and bone strength.Citation69 In addition, abnormal subchondral osteoblastic metabolism changes the NaV1.8 for osteoarthritis.Citation70 Purinergic P2X receptors and their family proteins play a role in osteoclast bone resorption, bone pain and inflammation, and may be promising potential therapeutic targets for osteoporosis.Citation71
Five families of chloride ion channels exist: ligand-gated anion channels, CFTR, ClCs, bestrophins, and anoctamins.Citation72 When the CFTR chloride channel is missing, it can cause OPG to decrease, RANK to increase, and RANKL/OPG ratio to decrease, thereby enhancing bone resorption.Citation73 Chlorine channels may affect bone remodeling by controlling acid secretion. When the proton pump and chlorine channel are activated, the pH in the local absorption cavity of osteoclasts drops to about 4.5.Citation74 Other research also shows osteoclasts secrete hydrogen ions through proton pumps, while chlorine ions dissolve minerals in bone through chloride ion channels on the cell membrane near the bottom of calcified bone.Citation75 It is speculated that the opening of the chloride channel may be a key step of osteoclast acid secretion and bone resorption. In addition, the chlorine channel also has a regulatory effect on bone formation. On the one hand, the expression of the ClC-3 chloride channel in osteoblasts is conducive to the stimulation of PTH by osteoblasts, thus mediating the differentiation of osteoblasts.Citation76 The ClC-3 chloride channel regulates the bone formation function of osteoblasts by regulating the estrogen a receptor.Citation77,Citation78 The increased expression levels of ClC-3 chlorine channel proteins can upregulate the expression of the downstream Runx2 gene, thus promoting osteogenic differentiation.Citation79 The lack of ClC-7 function can lead to bone sclerosis ().Citation80,Citation81
Figure 2 Mechanism of SOP.
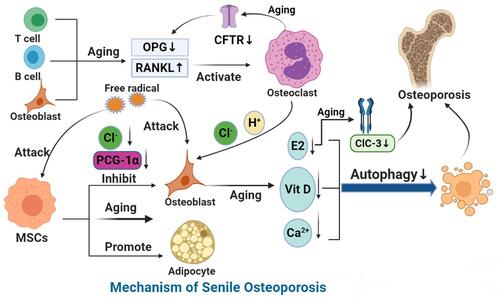
Treatment Strategies for Senile Osteoporosis
The drugs currently used to treat osteoporosis are mainly based on the principle of inhibiting bone resorption or promoting bone formation. Commonly used anti-osteoporotic drugs include bisphosphonates, parathyroid hormone, vitamin D, calcium and estrogen. Among them, calcium, vitamin D, simvastatin and parathyroid hormone mainly promote bone formation, and estrogen, bisphosphonates and calcitonin mainly function by inhibiting bone resorption. In ,Citation82–Citation89 we have summarized the current methods, shortcomings and mechanisms of clinical medications for the treatment of osteoporosis. Traditional drugs such as raloxifene and tamoxifen bind to estrogen α or β receptors in osteoblasts and osteoclasts, promoting osteoclast apoptosis and inhibiting bone resorption. Teriparatide as well as calcium tablet and vitamin D promote calcium and phosphorus metabolism and increase bone mineralization. Bisphosphonates and salmon calcificin inhibit osteoclast activity and formation to reduce bone resorption. In addition, some new drugs like simvastatin can improve the high metabolic state of bone, while monoclonal antibody drugs can affect osteoclast activity and have a positive effect on bone reconstruction. A good diet that includes calcium-rich foods can help to prevent osteoporosis. Aging and SASP play a mechanistic role in age-related and obesity-related fat deterioration, including inflammation and metabolic defects, which can be prevented or attenuated by exercise.Citation90 Mechanical signaling promotes bone and muscle anabolism through exercise, while limiting the formation and expansion of fat. By regulating the function of cells in bone tissue, machinery adjustment of bone and bone marrow fat can be achieved while preserving bias in selection of bone marrow mesenchymal stem cells lineages.Citation91 Calcium and vitamin D are critical in the treatment of osteoporosis in older adults. Increased antiresorption or anabolic therapy or combination therapy, at least in older adults with osteoporosis, may be effective in reducing the risk of vertebral fractures.Citation92 Aging is closely related to osteoporosis. The study of the pathogenesis of aging can provide theoretical basis for the treatment of senile osteoporosis.
Table 1 Common Clinical Medications and Limitations in the Treatment of Osteoporosis
Conclusion
To sum up, aging is a complex biological problem: the pathogenesis of aging is multicausative and comprehensive and complex aging is also a process in which various factors participate in and act together and various mechanisms interact with each other to jointly promote aging of the body. To explore the various mechanisms of aging in many aspects is helpful for us to fully understand the essence of aging and even delay the progress of aging. In the aging process, autophagy levels decrease and proinflammatory factors are produced, thus increasing the incidence of osteoporosis. Osteoporosis is a common metabolic disease, the incidence of which is increasing year by year in the population, seriously affecting the global economy and health. However, few studies on the relevant mechanism that connects aging and osteoporosis exist. It is believed that improving the level of autophagy, enhancing immune defense, limiting adipogenic differentiation, antioxidant-free radicals, slowing down the decline of estrogen, can delay the occurrence of senile osteoporosis. Therefore, we can enhance the immunity through daily exercise and limit the intake of fatty foods to strengthen bone. In addition, in terms of drug research, we can design new antioxidation or ion channel-based estrogen drugs to reduce bone loss.
Acknowledgments
The authors thank Dr Murad Alahdal for discussion and critical reading of the article.
Disclosure
The authors report no conflicts of interest in this work.
Additional information
Funding
References
- Kim HJ, Min JY, Min KB. Successful aging and mortality risk: the Korean longitudinal study of aging (2006–2014). J Am Med Dir Assoc. 2019;20:1013–1020. doi:10.1016/j.jamda.2018.12.010
- Aramillo Irizar P, Schäuble S, Esser D, et al. Transcriptomic alterations during ageing reflect the shift from cancer to degenerative diseases in the elderly. Nat Commun. 2018;9:327. doi:10.1038/s41467-017-02395-2
- Baker DJ, Wijshake T, Tchkonia T, et al. Clearance of p16Ink4a-positive senescent cells delays ageing-associated disorders. Nature. 2011;479:232–236. doi:10.1038/nature10600
- Berdasco M, Esteller M. Hot topics in epigenetic mechanisms of aging: 2011. Aging Cell. 2012;11:181–186. doi:10.1111/j.1474-9726.2012.00806.x
- Schmeer C, Kretz A, Wengerodt D, Stojiljkovic M, Witte OW. Dissecting aging and senescence-current concepts and open lessons. Cells. 2019;8:1446. doi:10.3390/cells8111446
- Herranz N, Gil J. Mechanisms and functions of cellular senescence. J Clin Invest. 2018;128:1238–1246. doi:10.1172/JCI95148
- De Cecco M, Ito T, Petrashen AP, et al. L1 drives IFN in senescent cells and promotes age-associated inflammation. Nature. 2019;566:73–78. doi:10.1038/s41586-018-0784-9
- López-Otín C, Blasco MA, Partridge L, Serrano M, Kroemer G. The hallmarks of aging. Cell. 2013;153:1194–1217. doi:10.1016/j.cell.2013.05.039
- Pomatto LCD, Davies KJA. Adaptive homeostasis and the free radical theory of ageing. Free Radic Biol Med. 2018;124:420–430. doi:10.1016/j.freeradbiomed.2018.06.016
- Jaskelioff M, Muller FL, Paik JH, et al. Telomerase reactivation reverses tissue degeneration in aged telomerase-deficient mice. Nature. 2011;469:102–106. doi:10.1038/nature09603
- de Boer J, Andressoo JO, de Wit J, et al. Premature aging in mice deficient in DNA repair and transcription. Science. 2002;296:1276–1279. doi:10.1126/science.1070174
- Shirakabe A, Ikeda Y, Sciarretta S, Zablocki DK, Sadoshima J. Aging and autophagy in the heart. Circ Res. 2016;118:1563–1576. doi:10.1161/CIRCRESAHA.116.307474
- Sen P, Shah PP, Nativio R, Berger SL. Epigenetic mechanisms of longevity and aging. Cell. 2016;166:822–839. doi:10.1016/j.cell.2016.07.050
- Horvath S, Zhang Y, Langfelder P, et al. Aging effects on DNA methylation modules in human brain and blood tissue. Genome Biol. 2012;13:R97. doi:10.1186/gb-2012-13-10-r97
- Horvath S. DNA methylation age of human tissues and cell types. Genome Biol. 2013;14:R115. doi:10.1186/gb-2013-14-10-r115
- Horvath S, Raj K. DNA methylation-based biomarkers and the epigenetic clock theory of ageing. Nat Rev Genet. 2018;19:371–384. doi:10.1038/s41576-018-0004-3
- Klutstein M, Nejman D, Greenfield R, Cedar H. DNA methylation in cancer and aging. Cancer Res. 2016;76:3446–3450. doi:10.1158/0008-5472.CAN-15-3278
- Field AE, Robertson NA, Wang T, Havas A, Ideker T, Adams PD. DNA methylation clocks in aging: categories, causes, and consequences. Mol Cell. 2018;71:882–895. doi:10.1016/j.molcel.2018.08.008
- Franceschi C, Campisi J. Chronic inflammation (inflammaging) and its potential contribution to age-associated diseases. J Gerontol a Biol Sci Med Sci. 2014;69(Suppl 1):S4–S9. doi:10.1093/gerona/glu057
- Fang EF, Scheibye-Knudsen M, Jahn HJ, et al. A research agenda for aging in China in the 21st century. Ageing Res Rev. 2015;24:197–205. doi:10.1016/j.arr.2015.08.003
- Wyss-Coray T. Ageing, neurodegeneration and brain rejuvenation. Nature. 2016;539:180–186. doi:10.1038/nature20411
- Lin X, Xiong D, Peng YQ, et al. Epidemiology and management of osteoporosis in the People’s Republic of China: current perspectives. Clin Interv Aging. 2015;10:1017–1033. doi:10.2147/CIA.S54613
- Si L, Winzenberg TM, Jiang Q, Chen M, Palmer AJ. Projection of osteoporosis-related fractures and costs in China: 2010–2050. Osteoporos Int. 2015;26:1929–1937. doi:10.1007/s00198-015-3093-2
- Li G, Thabane L, Papaioannou A, Ioannidis G, Levine MA, Adachi JD. An overview of osteoporosis and frailty in the elderly. BMC Musculoskelet Disord. 2017;18:46. doi:10.1186/s12891-017-1403-x
- Farr JN, Khosla S. Cellular senescence in bone. Bone. 2019;121:121–133. doi:10.1016/j.bone.2019.01.015
- Idris AI, Sophocleous A, Landao-Bassonga E, et al. Cannabinoid receptor type 1 protects against age-related osteoporosis by regulating osteoblast and adipocyte differentiation in marrow stromal cells. Cell Metab. 2009;10:139–147. doi:10.1016/j.cmet.2009.07.006
- Mirsaidi A, Genelin K, Vetsch JR, et al. Therapeutic potential of adipose-derived stromal cells in age-related osteoporosis. Biomaterials. 2014;35:7326–7335. doi:10.1016/j.biomaterials.2014.05.016
- Yu B, Huo L, Liu Y, et al. PGC-1α controls skeletal stem cell fate and bone-fat balance in osteoporosis and skeletal aging by inducing TAZ. Cell Stem Cell. 2018;23:615–623. doi:10.1016/j.stem.2018.09.001
- Li CJ, Cheng P, Liang MK, et al. MicroRNA-188 regulates age-related switch between osteoblast and adipocyte differentiation. J Clin Invest. 2015;125:1509–1522. doi:10.1172/JCI77716
- Xu C, Wang J, Zhu T, et al. Cross-talking between PPAR and WNT signaling and its regulation in mesenchymal stem cell differentiation. Curr Stem Cell Res Ther. 2016;11:247–254. doi:10.2174/1574888X10666150723145707
- Wang H, Chen Q, Lee SH, Choi Y, Johnson FB, Pignolo RJ. Impairment of osteoblast differentiation due to proliferation-independent telomere dysfunction in mouse models of accelerated aging. Aging Cell. 2012;11:704–713. doi:10.1111/j.1474-9726.2012.00838.x
- Manolagas SC. From estrogen-centric to aging and oxidative stress: a revised perspective of the pathogenesis of osteoporosis. Endocr Rev. 2010;31:266–300. doi:10.1210/er.2009-0024
- Liu Y, Wang C, Wang G, et al. Loureirin B suppresses RANKL-induced osteoclastogenesis and ovariectomized osteoporosis via attenuating NFATc1 and ROS activities. Theranostics. 2019;9:4648–4662. doi:10.7150/thno.35414
- Callaway DA, Jiang JX. Reactive oxygen species and oxidative stress in osteoclastogenesis, skeletal aging and bone diseases. J Bone Miner Metab. 2015;33:359–370. doi:10.1007/s00774-015-0656-4
- Rharass T, Lucas S. High glucose level impairs human mature bone marrow adipocyte function through increased ROS production. Front Endocrinol. 2019;10:607. doi:10.3389/fendo.2019.00607
- Zhang YB, Zhong ZM, Hou G, Jiang H, Chen JT. Involvement of oxidative stress in age-related bone loss. J Surg Res. 2011;169:e37–e42. doi:10.1016/j.jss.2011.02.033
- Zeng JH, Zhong ZM, Li XD, et al. Advanced oxidation protein products accelerate bone deterioration in aged rats. Exp Gerontol. 2014;50:64–71. doi:10.1016/j.exger.2013.11.014
- Khosla S, Monroe DG. Regulation of bone metabolism by sex steroids. Cold Spring Harb Perspect Med. 2018;8:a031211. doi:10.1101/cshperspect.a031211
- Compston JE. Sex steroids and bone. Physiol Rev. 2001;81:419–447. doi:10.1152/physrev.2001.81.1.419
- Lee K, Seo I, Choi MH, Jeong D. Roles of mitogen-activated protein kinases in osteoclast biology. Int J Mol Sci. 2018;19:3004. doi:10.3390/ijms19103004
- Zhang Y, Hua F, Ding K, Chen H, Xu C, Ding W. Angiogenesis changes in ovariectomized rats with osteoporosis treated with estrogen replacement therapy. Biomed Res Int. 2019;2019:1283717. doi:10.1155/2019/1283717
- Zhang Y, Gao Y, Cai L, et al. MicroRNA-221 is involved in the regulation of osteoporosis through regulates RUNX2 protein expression and osteoblast differentiation. Am J Transl Res. 2017;9:126–135.
- Chen S, Yang L, Jie Q, et al. MicroRNA-125b suppresses the proliferation and osteogenic differentiation of human bone marrow-derived mesenchymal stem cells. Mol Med Rep. 2014;9:1820–1826. doi:10.3892/mmr.2014.2024
- Jia J, Zhou H, Zeng X, Feng S. Estrogen stimulates osteoprotegerin expression via the suppression of miR-145 expression in MG-63 cells. Mol Med Rep. 2017;15:1539–1546. doi:10.3892/mmr.2017.6168
- Prata LG, Ovsyannikova IG, Tchkonia T, Kirkland JL. Senescent cell clearance by the immune system: emerging therapeutic opportunities. Semin Immunol. 2018;40:101275. doi:10.1016/j.smim.2019.04.003
- Salminen A, Kaarniranta K, Kauppinen A. Immunosenescence: the potential role of myeloid-derived suppressor cells (MDSC) in age-related immune deficiency. Cell Mol Life Sci. 2019;76:1901–1918. doi:10.1007/s00018-019-03048-x
- Onal M, Xiong J, Chen X, et al. Receptor activator of nuclear factor κB ligand (RANKL) protein expression by B lymphocytes contributes to ovariectomy-induced bone loss. J Biol Chem. 2012;287:29851–29860. doi:10.1074/jbc.M112.377945
- Choi Y, Woo KM, Ko SH, et al. Osteoclastogenesis is enhanced by activated B cells but suppressed by activated CD8(+) T cells. Eur J Immunol. 2001;31:2179–2188. doi:10.1002/1521-4141(200107)31:7<2179:AID-IMMU2179>3.0.CO;2-X
- Toraldo G, Roggia C, Qian WP, Pacifici R, Weitzmann MN. IL-7 induces bone loss in vivo by induction of receptor activator of nuclear factor kappa B ligand and tumor necrosis factor alpha from T cells. Proc Natl Acad Sci U S A. 2003;100:125–130. doi:10.1073/pnas.0136772100
- Srivastava RK, Dar HY, Mishra PK. Immunoporosis: immunology of osteoporosis-role of T cells. Front Immunol. 2018;9:657. doi:10.3389/fimmu.2018.00657
- Müller L, Di Benedetto S, Pawelec G. The immune system and its dysregulation with aging. Subcell Biochem. 2019;91:21–43.
- Chen K, Yang YH, Jiang SD, Jiang LS. Decreased activity of osteocyte autophagy with aging may contribute to the bone loss in senile population. Histochem Cell Biol. 2014;142:285–295. doi:10.1007/s00418-014-1194-1
- Pierrefite-Carle V, Santucci-Darmanin S, Breuil V, Camuzard O, Carle GF. Autophagy in bone: self-eating to stay in balance. Ageing Res Rev. 2015;24:206–217. doi:10.1016/j.arr.2015.08.004
- Almeida M, Han L, Martin-Millan M, et al. Skeletal involution by age-associated oxidative stress and its acceleration by loss of sex steroids. J Biol Chem. 2007;282:27285–27297. doi:10.1074/jbc.M702810200
- Abrigo J, Rivera JC, Aravena J, et al. High fat diet-induced skeletal muscle wasting is decreased by mesenchymal stem cells administration: implications on oxidative stress, ubiquitin proteasome pathway activation, and myonuclear apoptosis. Oxid Med Cell Longev. 2016;2016:9047821. doi:10.1155/2016/9047821
- Song C, Song C, Tong F. Autophagy induction is a survival response against oxidative stress in bone marrow-derived mesenchymal stromal cells. Cytotherapy. 2014;16:1361–1370. doi:10.1016/j.jcyt.2014.04.006
- Wan Y, Zhuo N, Li Y, Zhao W, Jiang D. Autophagy promotes osteogenic differentiation of human bone marrow mesenchymal stem cell derived from osteoporotic vertebrae. Biochem Biophys Res Commun. 2017;488:46–52. doi:10.1016/j.bbrc.2017.05.004
- Camuzard O, Santucci-Darmanin S, Breuil V, et al. Sex-specific autophagy modulation in osteoblastic lineage: a critical function to counteract bone loss in female. Oncotarget. 2016;7:66416–66428. doi:10.18632/oncotarget.12013
- Shen G, Ren H, Qiu T, et al. Implications of the interaction between miRNAs and autophagy in osteoporosis. Calcif Tissue Int. 2016;99:1–12. doi:10.1007/s00223-016-0122-x
- Gallagher JC. Vitamin D and aging. Endocrinol Metab Clin North Am. 2013;42:319–332. doi:10.1016/j.ecl.2013.02.004
- Sims NA, Ng KW. Implications of osteoblast-osteoclast interactions in the management of osteoporosis by antiresorptive agents denosumab and odanacatib. Curr Osteoporos Rep. 2014;12:98–106. doi:10.1007/s11914-014-0196-1
- Deng Z, Lin Z, Zhong Q, et al. Interleukin 1 beta-induced chloride currents are important in osteoarthritis onset: an in vitro study. Acta Biochim Biophys Sin. 2021;53:400–409. doi:10.1093/abbs/gmab010
- Liang Y, Duan L, Xiong J, et al. E2 regulates MMP-13 via targeting miR-140 in IL-1β-induced extracellular matrix degradation in human chondrocytes. Arthritis Res Ther. 2016;18:105. doi:10.1186/s13075-016-0997-y
- Zhang H, Deng Z, Yang L, et al. The AQP-3 water channel is a pivotal modulator of glycerol-induced chloride channel activation in nasopharyngeal carcinoma cells. Int J Biochem Cell Biol. 2016;72:89–99. doi:10.1016/j.biocel.2016.01.009
- Zhang N, Deng Z, Li W, et al. Expression of LRRC8A is elevated in the cytoplasm of osteosarcoma tissues: an immunohistochemical study with tissue microarrays. Exp Ther Med. 2021;21:71. doi:10.3892/etm.2020.9503
- Sun W, Chi S, Li Y, et al. The mechanosensitive Piezo1 channel is required for bone formation. Elife. 2019;8:e47454. doi:10.7554/eLife.47454
- Li X, Han L, Nookaew I, et al. Stimulation of Piezo1 by mechanical signals promotes bone anabolism. Elife. 2019;8:e49631. doi:10.7554/eLife.49631
- Hendrickx G, Fischer V, Liedert A, et al. Piezo1 inactivation in chondrocytes impairs trabecular bone formation. J Bone Miner Res. 2021;36:369–384. doi:10.1002/jbmr.4198
- Wang L, You X, Lotinun S, Zhang L, Wu N, Zou W. Mechanical sensing protein PIEZO1 regulates bone homeostasis via osteoblast-osteoclast crosstalk. Nat Commun. 2020;11:282. doi:10.1038/s41467-019-14146-6
- Zhu J, Zhen G, An S, et al. Aberrant subchondral osteoblastic metabolism modifies NaV1.8 for osteoarthritis. Elife. 2020;9:e57656. doi:10.7554/eLife.57656
- Jørgensen NR. Role of the purinergic P2X receptors in osteoclast pathophysiology. Curr Opin Pharmacol. 2019;47:97–101. doi:10.1016/j.coph.2019.02.013
- Duran C, Thompson CH, Xiao Q, Hartzell HC. Chloride channels: often enigmatic, rarely predictable. Annu Rev Physiol. 2010;72(1):95–121. doi:10.1146/annurev-physiol-021909-135811
- Stalvey MS, Clines KL, Havasi V, et al. Osteoblast CFTR inactivation reduces differentiation and osteoprotegerin expression in a mouse model of cystic fibrosis-related bone disease. PLoS One. 2013;8:e80098. doi:10.1371/journal.pone.0080098
- Du J, Wang Q, Hu F, et al. Effects of estradiol on voltage-gated potassium channels in mouse dorsal root ganglion neurons. J Membr Biol. 2014;247:541–548. doi:10.1007/s00232-014-9670-z
- Boyce BF, Li J, Xing L, Yao Z. Bone remodeling and the role of TRAF3 in osteoclastic bone resorption. Front Immunol. 2018;9:2263. doi:10.3389/fimmu.2018.02263
- Lu X, Ding Y, Niu Q, et al. ClC-3 chloride channel mediates the role of parathyroid hormone [1–34] on osteogenic differentiation of osteoblasts. PLoS One. 2017;12:e0176196. doi:10.1371/journal.pone.0176196
- Deng Z, Li W, Xu J, et al. ClC-3 chloride channels are involved in estradiol regulation of bone formation by MC3T3-E1 osteoblasts. J Cell Biochem. 2018;10:8366–8375.
- Deng Z, Peng S, Zheng Y, et al. Estradiol activates chloride channels via estrogen receptor-α in the cell membranes of osteoblasts. Am J Physiol Cell Physiol. 2017;313:C162–C172. doi:10.1152/ajpcell.00014.2017
- Wang H, Mao Y, Zhang B, et al. Chloride channel ClC-3 promotion of osteogenic differentiation through Runx2. J Cell Biochem. 2010;111:49–58. doi:10.1002/jcb.22658
- Tolar J, Teitelbaum SL, Orchard PJ. Osteopetrosis. N Engl J Med. 2004;351:2839–2849. doi:10.1056/NEJMra040952
- Jentsch TJ, Pusch M. CLC chloride channels and transporters: structure, function, physiology, and disease. Physiol Rev. 2018;98:1493–1590. doi:10.1152/physrev.00047.2017
- Lee J, Alqudaihi HM, Kang MS, et al. Effect of tamoxifen on the risk of osteoporosis and osteoporotic fracture in younger breast cancer survivors: a nationwide study. Front Oncol. 2020;10:366. doi:10.3389/fonc.2020.00366
- Olevsky OM, Martino S. Randomized clinical trials of raloxifene: reducing the risk of osteoporosis and breast cancer in postmenopausal women. Menopause. 2008;15(4 Suppl):790–796. doi:10.1097/gme.0b013e31817e6683
- Anagnostis P, Gkekas NK, Potoupnis M, Kenanidis E, Tsiridis E, Goulis DG. New therapeutic targets for osteoporosis. Maturitas. 2019;120:1–6. doi:10.1016/j.maturitas.2018.11.010
- Capozzi A, Scambia G, Lello S. Calcium, vitamin D, vitamin K2, and magnesium supplementation and skeletal health. Maturitas. 2020;140:55–63. doi:10.1016/j.maturitas.2020.05.020
- Zhou W, Liu Y, Guo X, Yang H, Xu Y, Geng D. Effects of zoledronic acid on bone mineral density around prostheses and bone metabolism markers after primary total hip arthroplasty in females with postmenopausal osteoporosis. Osteoporos Int. 2019;30:1581–1589. doi:10.1007/s00198-019-05005-7
- Chen C, Alqwbani M, Zhao J, Yang R, Wang S, Pan X. Effects of teriparatide versus salmon calcitonin therapy for the treatment of osteoporosis in Asia: a meta-analysis of randomized controlled trials. Endocr Metab Immune Disord Drug Targets. 2021;21:932–942. doi:10.2174/1871530320999200817114817
- Wu T, Sun J, Tan L, et al. Enhanced osteogenesis and therapy of osteoporosis using simvastatin loaded hybrid system. Bioact Mater. 2020;5:348–357. doi:10.1016/j.bioactmat.2020.03.004
- Deeks ED. Denosumab: a review in postmenopausal osteoporosis. Drugs Aging. 2018;35:163–173. doi:10.1007/s40266-018-0525-7
- Schafer MJ, Miller JD, LeBrasseur NK. Cellular senescence: implications for metabolic disease. Mol Cell Endocrinol. 2017;455:93–102. doi:10.1016/j.mce.2016.08.047
- Pagnotti GM, Styner M, Uzer G, et al. Combating osteoporosis and obesity with exercise: leveraging cell mechanosensitivity. Nat Rev Endocrinol. 2019;15:339–355. doi:10.1038/s41574-019-0170-1
- Vandenbroucke A, Luyten FP, Flamaing J, Gielen E. Pharmacological treatment of osteoporosis in the oldest old. Clin Interv Aging. 2017;12:1065–1077. doi:10.2147/CIA.S131023