Abstract
Burkitt’s lymphoma (BL) is an aggressive disorder associated with extremely high rates of cell proliferation tempered by high levels of apoptosis. Despite the high levels of cell death, the net effect is one of rapid tumor growth. The tumor arises within the germinal centers of secondary lymphoid tissues and is identifiable by translocation of the c-MYC gene into the immunoglobulin gene loci, resulting in deregulation of the proto-oncogene. Many of the major players involved in determining the development of BL have been characterized in human BL cell lines or in mouse models of MYC-driven lymphomagenesis. Both systems have been useful so far in characterizing the role of tumor suppressor genes (for example, p53), prosurvival signaling pathways, and members of the B-cell lymphoma-2 family of apoptosis regulators in determining the fate of c-MYC overexpressing B-cells, and ultimately in regulating lymphoma development. Signaling through phosphoinositide (PI)3-kinase stands out as being critical for BL cell survival. Recurrent mutations in ID3 or TCF3 (E2A) that promote signaling through PI3-kinase have recently been identified in human BL samples, and new therapeutic strategies based on coordinately targeting both the prosurvival factor, B-cell lymphoma-XL, and the PI3-kinase/AKT/mammalian target of rapamycin (mTOR) signaling pathway to synergistically induced BL apoptosis have been proposed. Now, engineering both constitutive c-MYC expression and PI3-kinase activity, specifically in murine B-cells undergoing the germinal center reaction, has revealed that there is synergistic cooperation between c-MYC and PI3-kinase during BL development. The resulting tumors phenocopy the human malignancy, and acquire tertiary mutations also present in human tumors. This model may, therefore, prove useful in further studies to identify functionally relevant mutational events necessary for BL pathogenesis. This review discusses these cooperating interactions, the possible influence of BL tumor-associated viruses, and highlights potential new opportunities for therapeutic intervention.
Introduction
Molecular pathology of Burkitt’s lymphoma
Burkitt’s lymphoma (BL) was originally identified by Dr Denis Burkitt in 1958 as an aggressive tumor of the jaw found in a cohort of children living in malarial areas of Africa (endemic BL).Citation1 Today, this lymphoma is subdivided into three different subclasses with some distinct pathological features evident in each subtype. The second subgroup, sporadic BL, accounts for a high proportion of western pediatric lymphoma cases. These commonly arise within the ileum, but progress to involve the bone marrow or blood where circulating lymphoma cells are evident. The third subgroup is associated with immunodeficiency, either as a consequence of human immunodeficiency virus (HIV) infection, or as a result of immunosuppressive therapy following organ transplantation. BL accounts for approximately 30% of those lymphomas related to HIV infection. Concurrent infection with the γ-herpesvirus, Epstein–Barr virus (EBV) is found in the majority of endemic BL cases, but EBV-positive lymphoma cells are also detected, to a variable degree, in sporadic (approximately 15%) and immunodeficiency-associated cases (between 40% and 50%) depending upon the demographic.Citation2 The common features of all subtypes are that they arise from differentiating B-cells within germinal center (GC) reactions and carry somatic hyper-mutations of the B-cell receptor (BCR) immunoglobulin (Ig) heavy chain variable region, indicative of this site of origin. Histologically, the lymphomas have a characteristic “starry sky” appearance and are comprised of densely packed, CD10/CD20/IgM-positive cells with a highly proliferative Ki-67-positive fraction of greater than 95%. Unlike follicular lymphoma, BLs are typically negative for the antiapoptotic protein, B-cell lymphoma (BCL)-2, again indicative of the cell of origin. All three subtypes also have a common genetic abnormality that is critically required for tumorigenesis. Deregulation and overexpression of the proto-oncogene c-MYC in BL is a result of translocation of the c-MYC gene on chromosome 8Citation3,Citation4 to either the heavy, light, or kappa chain Ig loci (t[8;14], t[2;8], or t[8;22] translocation) with t(8;14) being the most frequently observed. How malaria and EBV infections contribute to BL pathogenesis is not entirely understood, but they presumably help to establish a cellular- or micro-environment conducive for c-MYC translocation and survival of the c-MYC overexpressing B-cell, eventually leading to lymphoma development. Their involvement in BL pathogenesis may well involve establishing a state of chronic B-cell proliferation and activation (as reviewed in the case of malaria by Moormann et al).Citation5 The extraordinary ability of EBV to drive B-cell proliferation (interestingly, also involving a similar increase in c-MYC expression) while simultaneously abrogating apoptosis has also been reviewed elsewhere.Citation6 This review will discuss both the effects of oncogenic c-MYC deregulation in BL cells, and the subsequent cooperating events required for BL tumorigenesis.
c-MYC
The proto-oncogene c-MYC is commonly deregulated in human cancers. It is a member of the helix–loop–helix leucine zipper family of nuclear transcription factors (TCFs), regulating the expression of a whole host of genes (and micro ribonucleic acids [micro RNAs])Citation7,Citation8 involved in signal transduction, cell cycle regulation, metabolism, apoptosis, cell adhesion, and protein biosynthesis. The carboxyl-terminus of the 430aa protein contains a helix–loop–helix deoxyribonucleic acid (DNA)-binding domain. The dimerization domain mediates heterodimerization with MYC associated factor X (MAX), and this complex formation is required for the conversion of MYC into an active polypeptide capable of binding enhancer box (E-box) target DNA sequences (5’-CACGTG-3’).Citation9,Citation10 MYC/MAX activity may itself be inhibited by the competitive binding of MAX by MAD1.Citation11
Regulation of c-MYC expression is complex, occurring both at the level of gene transcription from one of four potential transcriptional promoters, and also via posttranslational modifications affecting protein stability. Moreover, c-MYC promoters P1 and P2 lie in the noncoding exon 1 of the gene, upstream of the coding exons 2 and 3. The vast majority (80%–90%) of MYC in normal cells is transcribed from P2; however, a switch to P1 promoter usage is observed following gene translocation when transcription falls under the control of Ig enhancer elements.Citation12 The normal c-MYC allele is silent in BL.Citation13,Citation14 The c-MYC protein itself is normally degraded very rapidly, having a half-life of only 20–30 minutes. MYC is usually difficult to detect by Western blot analysis in normal cells, however, high protein expression levels are observed (and tolerated) in BL cell lines which necessitates mutational events and crosstalk with other signaling pathways (which will be discussed).
Functionally, in normal cells, c-MYC has an important role in increasing the mass of a cell prior to its division – a process required to maintain the size of the two resulting daughter cells. Hypofunctioning MYC in drosophila, results in a small-body phenotype (minutes) caused by decreased cell size (as opposed to fewer cell numbers).Citation15 This phenotype correlates with a reduction in the expression of ribosomal protein genes required for protein synthesis.Citation16 MYC expression also subsequently promotes the cell division through the upregulation of cyclin D, E2F, and the cyclin-dependent kinase (CDK)4,Citation17 while repressing genes involved in cell cycle arrest (p27, p15, p21, p57, growth arrest and DNA damage [GADD]45, GADD34, and GADD153).
Deregulation of MYC in BL is undoubtedly a potent promoter of lymphocyte proliferation; however, overexpression of this proto-oncogene also induces powerful antiproliferative, apoptotic stress responses (), which ultimately must be overcome for lymphomagenesis.
Figure 1 Pathways regulating proliferation, survival, and cell death in BL.
Notes: BL is associated with chromosomal translocations that deregulate expression of the proto-oncogene c-MYC. Specifically, c-MYC increases cell size and cell proliferation in part through transcriptional induction of numerous genes including cyclin D, cyclin-dependent kinases, and E2F, which release cells from the G1/S cell cycle checkpoint regulated by RB/e2F. The capacity to regulate a vast array of cellular genes and drive cell proliferation is tempered by the induction of oncogene-induced apoptosis and senescence mediated by the ARF/p53 tumor suppressor pathway and “intrinsic” apoptosis. Intrinsic, or mitochondrial, apoptosis is regulated by pro-apoptotic BH3-only members of the BCL-2 family (for example, BIM and PUMA). These proteins activate the mitochondrial membrane proteins BAX and BAK to induce the release of cytochrome c from mitochondria into the cytoplasm and activation of the caspase cascade. At each point the effect of c-MYC can be regulated by cross-talk with other signaling pathways including the pro-proliferative, prosurvival PI3K/AKT pathway. The net outcome of cell cycle arrest and apoptosis versus proliferation and survival of B-cells carrying translocated c-MYC determines disease progression and lymphomagenesis.
Abbreviations: PI3K, phosphoinositide 3-kinase; CDK4, cyclin-dependent kinase 4; HDM2, human double minute 2 homolog; PUMA, p53-upregulated modulator of apoptosis; BL, Burkitt’s lymphoma; RB, retinoblastoma; BH3, Bcl-2 homology 3; BCL-2, B-cell lymphoma 2.
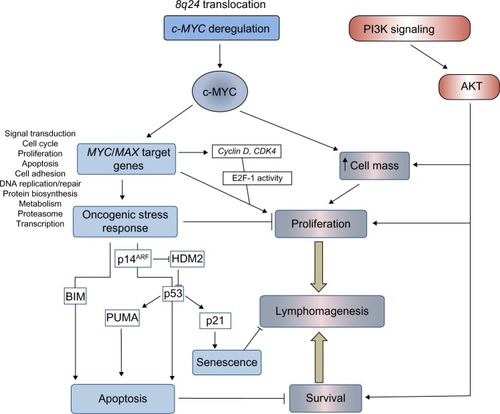
Functions of c-MYC in BL
The role of MYC in BL development, as well as the tumor suppressor pathways employed in an attempt to control lymphocyte proliferation, have been studied in depth using various experimental approaches. These include the analysis of both established BL cell lines and the engineered c-MYC-inducible cell line; P493-6, as well as the study of mouse models of MYC-driven lymphomas (Eμ-Myc and iMYCEμ).Citation18
It is clear that continuous MYC activity is required for BL lymphomagenesis. Pharmacological inhibition of MYC/MAX heterodimer formation and MYC function in BL cell lines (using 10058-F4; Sigma-Aldrich, St Louis, MO, USA)Citation19 has demonstrated conclusively that c-MYC is essential for BL cell proliferationCitation20,Citation21 and survival.Citation22 Using alternative approaches, conditional MYC expression systems have also been analyzed both in vitro and in vivo. Conditional activation of MYC in the P493-6 cell line, which contains an estrogen-regulatable estrogen receptor–c-MYC fusion protein, demonstrated that in BL cells (as in normal cells), c-MYC regulates cell growth through an increase in metabolism and protein synthesis. Following serum starvation, cell growth and division become uncoupled since the starved cells increase in size, but are unable to progress through the cell cycle. Proliferation itself is, therefore, dependent on serum factors.Citation23,Citation24 Conditional Tet-off MYC expression in murine lymphoid cells has also demonstrated that loss of transgenic MYC expression following treatment with doxycycline results in a G1 cell cycle arrest and apoptosis of the MYC-driven lymphoma cells.Citation25 MYC, however, does not appear to play a significant role in driving cells through stages of B-cell development since lymphomas in Eμ-MYC or MYC insertion iMYCEμ mice arise at the pre-B-cell or mature stages (a deviation from a faithful phenocopy of the GC-derived human disease). More mechanistic studies have examined the transcriptome associated with BL and MYC overexpression, either using the conditional systems mentioned above,Citation26–Citation28 or by genome-wide chromatin immunoprecipitation analysis of MYC DNA-binding sites in BL cell lines.Citation29,Citation30 What is evident from these studies is that MYC deregulation establishes a generalized increase in metabolic activity, gene transcription, and protein synthesis in the afflicted cell, rather than selective upregulation of certain cancer-causing genes (). Since many of the MYC target genes are TCFs themselves,Citation29 waves of time-dependent transcription are established through direct and indirect target gene regulation.Citation31 The critical question to ask in order for us to understand how lymphomas develop following c-MYC translocation is what cooperating genetic alterations and mutations enable a cell to tolerate such extensive transcriptional deregulation and oncogene-induced stress?
Cooperating mutations
Determining which cooperating mutations can promote the survival of MYC-driven lymphoma may help in the identification of more selective targeted therapies. Genomic analysis has revealed that BLs are genomically heterogeneous, with 70 genes identified as being recurrently mutated (and as many as 17 mutated per lymphoma).Citation32 Recent advances in our understanding of these mutations will be discussed in the following sections.
Mutations in MYC
Levels of c-MYC, even in proliferating normal cells, are usually relatively low. Since MYC has a short half-life,Citation33 any change in the transcriptional/translational output of the gene would therefore be “sensed” rapidly by the cell – a sensible precaution for such a potent metabolic and transcriptional activator. BL cells, which are dependent on continuous MYC expression for survival, select for cooperating events, which have the effect of increasing the availability of the transforming protein. These may involve crosstalk with activated signaling pathways (such as phosphoinositide 3-kinase [PI3K], discussed later) or mutations within MYC itself. The amino-terminus transactivation domain of c-MYC contains two conserved, functionally critical MYC family regions called box 1 and box 2.Citation34 Mutations within box 2 identified within the BL samples affect the transforming ability of MYC and its ability to promote apoptosis.Citation35 Box 1 contains phosphorylation sites involved in the proteolysis of c-MYC by the ubiquitin–proteasome pathway.Citation36 Residues within this region are mutated in approximately 20% of BLs, especially at the hotspot residues 39, 57, and 58. The threonine 58 (Thr58) to alanine mutation results in inefficient ubiquitination, a decrease in proteasomal degradation, and thus, an increase in protein stability ().Citation37–Citation39 Thr58 is a target of glycogen synthase kinase (GSK)3β phosphorylation, an event that is dependent on phosphorylation of Serine (Ser)62 and the proline residue at position 57. Therefore, any mutation of Proline (Pro)57 would also inhibit Thr58 modification.Citation40 The observation that the Thr58 mutation of c-MYC and the inactivating mutations of the p53 tumor suppressor pathway are mutually exclusive in BL, however, also implies that the mutation of MYC has a function in the avoidance of oncogene-induced apoptotic responses.
Figure 2 Cooperating mutational events in MYC-driven lymphomagenesis and novel therapeutic opportunities.
Notes: Continuous MYC expression is required for BL cell survival, as demonstrated by the apoptotic effect of the MYC inhibitor 10058-F4 on BL cell lines. (Inhibitors of various pathway components are indicated in red lettering throughout, and appropriate references citing potential therapeutic approaches are indicated.) As a defense against continuous, high-level MYC expression and lymphoma development, the induction of rate-limiting apoptosis is most efficient when both the p53 and the BIM-induced apoptosis pathways are activated simultaneously. BLs undergo mutational and epigenetic changes during tumorigenesis to inactivate either one of these tumor suppressor pathways. Mutational events include loss of the p53 activator p14ARF, mutation of p53, or an increase in expression of its upstream regulators MDM2 or MDM4, which target p53 for proteasomal degradation. The effect of MDM2 can be inhibited by nutlin in cells carrying wild type p53 resulting in p53 activation and apoptosis. Induction of the pro-apoptotic BH3-only protein BIM by MYC can be lost selectively in BL cells carrying mutant MYC protein. For example, MYC proteins mutated at Thr58 lose the ability to induce transcription of BIM. Transcription of BIM, or the p53 and TGF-β pro-apoptotic target gene PUMA, may also be suppressed through methylation of their promoters. BH3-mimetic compounds (ABT-737) are functionally similar to BIM and PUMA and have been shown to induce apoptosis of BL cell lines. Recent evidence has implicated signaling via PI3K/AKT/mTOR as being a critical cooperating oncogenic pathway in the development of BL. BL tumors display mutations in TCF3 (E2A), ID3, and the inhibitory phosphatase, PTEN, that function to increase “tonic” B-cell receptor signaling through PI3K. Tonic BCR signaling is used to describe low-level antigen-independent signaling by the B-cell receptor. TCF3 is a transcription factor normally regulated by ID3. Mutations in ID3 relieve repression of TCF3, resulting in TCF3-mediated expression of heavy and light immunoglobulin chains components of the BCR and increased BCR signaling. Cyclin D3 mutations are also recurrent, and BL cells are dependent on cyclin D/CDK6 activity for proliferation. The stability of MYC itself is regulated by proteolysis through the ubiquitin/proteasome pathway. Targeting MYC for degradation is dependent on GSK3β-mediated phosphorylation of Thr58. MYC may be stabilized in BL either through a Thr58–alanine mutational substitution, or through GSK3β inactivation by the cooperating PI3K pathway. Selectively blocking of PI3K, AKT, and/or mTOR signaling using various inhibitors (shown in red) correlates with induction of BL cell apoptosis. In addition, mTORC1/2 signaling through 4E-BP1 and eIF4E (not shown) is required for 5’ cap-dependent protein translation. Blocking 4EBP-1 activity using selective inhibitors (PP242 or BEZ235) decreases the expression of the prosurvival factor MCL-1 and MYC (short-lived proteins requiring continuous 5’ cap-dependent protein translation for maintenance of expression), or induces BMF as a cellular stress response.
Abbreviations: CDK6, cyclin-dependent kinase 6; TCF3, transcription factor 3; BCR, B-cell receptor; GSK3β, glycogen synthase kinase 3β; Thr58, threonine 58; PTEN, phosphatase and tensin homolog; MDM2, mouse double minute 2 homolog; MDM4, mouse double minute 4 homolog; PI3K, phosphoinositide 3-kinase; PUMA, p53-upregulated modulator of apoptosis; TGF, transforming growth factor; mTOR, mammalian target of rapamycin; MCL-1, induced myeloid leukemia cell differentiation protein; S6K, ribosomal protein S6 kinase; BL, Burkitt’s lymphoma; BH3, Bcl-2 homology 3.
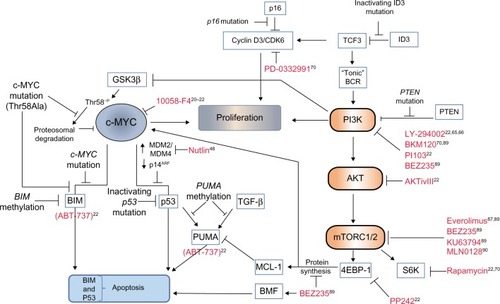
Avoiding oncogene-induced stress responses
Various mechanisms for avoiding such responses have been identified in BL and are represented schematically in . Analysis of the murine lymphoma model indicates that acute MYC expression while inducing cell proliferation also induces apoptosis, provided that two requirements are met – the first being the activation of the p53 tumor suppressor pathway.
To activate the p53 pathway, p53 is stabilized through MYC/Forkhead box protein (FOXO) transcription of the CDKN2A locus encoding p14ARF (named p19ARF in mice),Citation41 which inhibits degradation of p53 by mouse double minute (MDM)2 homolog. The resulting induction of apoptosisCitation42 and senescenceCitation43 in premalignant Eμ-Myc B-cells is rate limiting for lymphoma development. As might be predicted, p53 is frequently a target for mutation in BL.Citation44,Citation45 Elevated levels of MDM4,Citation46 MDM2 (called human double minute 2 homolog in humans) or loss of p14ARF expression have also been detected in BL cells carrying wild type p53,Citation47 which would also have the effect of inactivating p53 tumor suppressor function. The activity of p53 may be reactivated in cases of elevated MDM2/4 expression using inhibitors of MDM2 activity to induce apoptosis.Citation48
The second requirement for oncogene-induced apoptosis is the induction of BIM. BIM is a pro-apoptotic Bcl-2 homology 3 (BH3)-only member of the BCL-2 family, which acts via the mitochondrial membrane proteins Bcl-2 associated X protein (BAX) and Bcl-2 homologous antagonist/killer (BAK) to induce intrinsic mitochondrial apoptosis (). The loss of a single allele of BIM in Eμ-MYC mice accelerates the development of lymphomas.Citation49 This decision pointCitation50 where both p53 and the mitochondrial apoptotic machinery must be engaged for commitment to apoptosis is presumably a safeguard to ensure cell destruction only occurs when absolutely necessary. Lymphoma development applies a selective pressure such that at least one of these pathways must be inactivated. The evidence suggests that a loss of function of one of the arms of the stress response precludes any need for mutational inactivation of the other.Citation51
If, for example, BAX is experimentally inactivated (BAX activity being required for the BIM-induced apoptosis), the selection of p53 mutations in MYC-driven lymphoma is circumvented since the intrinsic pathway is now nonfunctional.Citation52 Similarly the MYC Thr58 mutation, as discussed above, is seen only in BL with an intact p53 pathway, and so it must provide a function that is not needed in the context of inactive p53. Analysis of lethally irradiated mice reconstituted with stem cells transfected with wild type or mutant MYC showed that this mutation, in fact, disables the ability of MYC to induce transcription of BIM, subsequently negating the intrinsic apoptosis arm of the oncogene-induced stress response. Consequently, only cells carrying wild type MYC developed inactivating p53 mutations. In biopsies of EBV-positive BLs, the BIM promoter may also be methylated at CpG dinucleotides, suggesting that epigenetic silencing of BIM could play an important role in tumorigenesis.Citation53,Citation54
Interestingly, p53-upregulated modulator of apoptosis (PUMA), a BH3 protein functionally akin to BIM, is also involved in the p53-induced apoptotic response.Citation55 While this protein is not mutated in BL, it may be subject to decreased expression through promoter methylation – even in tumors carrying mutant p53Citation56 – suggesting that there are p53-independent regulators of PUMA active in BL. We recently showed that PUMA is a downstream effector of a transforming growth factor (TGF)-β-induced apoptosis pathway active in both human BL cell lines and murine Eμ-MYC-derived lymphoma cells.Citation57 TGF-β also negatively regulates the BL survival factor, BCL-XL.Citation58 The selective pressure used to reduce PUMA expression in lymphomas, therefore, may arise from either the induction of p53, or through activation of the TGF-β tumor suppressor pathway ().
So far, the studies mentioned describe the impact of genetic changes directly affecting two rate-limiting tumor suppressor pathways acting through p53 and intrinsic apoptosis. There is, however, evidence to implicate mutational changes in other signaling pathways that indirectly impact on these tumor suppressor pathways. For instance, further mutations have been identified in BL samples which upregulate signaling through PI3K. This signaling pathway has a critical role in cooperating with MYC during lymphomagenesis and promoting BL survival. The involvement of PI3K signaling in BL pathogenesis and opportunities for therapeutic intervention will be discussed.
The role of PI3K: MYC is not the only one “AKT”ing up to cause BL
BL development has only recently, for the first time, been modeled faithfully in mice by Sander et al,Citation59 who investigated the effects of coordinately targeting both c-MYC and constitutively active PI3K. The idea that PI3K activity may be required for survival of BL cells harboring high levels of c-MYC expression was certainly not new, given that there have been numerous previous studies linking c-MYC-mediated transformations with the requirement for PI3K activity.Citation60–Citation64 The dependence of human BL cells on PI3K activity for proliferation and survival was also demonstrated by Curnock and KnoxCitation65 over a decade ago using PI3K inhibitors, and by others since.Citation22,Citation66 However, the new mouse model advances our understanding in that MYC and PI3K activity were targeted specifically to cells undergoing the GC reaction. Thus, the model more accurately recapitulates oncogenic processes occurring at the site of origin of BL, and these data unequivocally link PI3K signaling with MYC as cooperating factors in the development of BL. The lymphomas that formed in this model were a phenocopy of human tumors in terms of histology, cell surface marker expression, and ongoing somatic hypermutations of Ig-variable regions (indicative of GC B-cells). Importantly, the tumors exhibited gene and protein expression profiles similar to human BL, which distinguished them from those seen in diffuse large B-cell lymphoma (DLBCL). The tumors also developed tertiary stabilizing mutations in cyclin D3, an important regulator of GC B-cell proliferation,Citation67,Citation68 and frequently detected in BL samples.Citation69 In addition to this advance, however, there have been exciting new discoveries as to how PI3K might be activated in BL, and what downstream effectors could be targeted effectively in new therapeutic strategies.
Significantly, Schmitz et alCitation70 have identified recurrent mutations within approximately 70% of BL that increase signaling through PI3K. RNA sequencing on sporadic BL and BL cell lines revealed monoallelic mutations within TCF3 (also called E2A), biallelic mutations within its highly expressed negative regulator Inhibitor of DNA binding-3 (ID3), as well as infrequent (7%) mutations of the inhibitory phosphatase, PTEN (see ). In normal B-cells, E2A is involved in antigen-dependent B-cell responses and is expressed within the dark zone of GCs.Citation71 It is itself a target for translocation events in B-cell acute lymphoblastic leukemia. The mutant transcription factor 3 (TCF3) proteins identified in BLs escape from the inhibitory effects of the ID proteins to upregulate expression of the heavy and light Ig chains of the BCR. The authors suggest a dependency of BL cells on “tonic” BCR signaling for survival of the tumor cells. Such so-called tonic BCR signaling is a state of low-level BCR activation required for the survival of resting mature B cells in vivo. It involves constitutive signaling via Syk, but does not require binding of the BCR to its cognate antigen (antigen-independent signaling). Like those seen in the Sander et alCitation59 mouse model, mutations in BL samples were also identified within the TCF3 target gene cyclin D3. Furthermore, using the CDK4/6 inhibitor PD 0332991 the study demonstrated that the BL cells were dependent on cyclin D3/CDK6 activity (regardless of the mutational status of the cyclin D3 gene), and that the cyclin D3 mutations augmented cell cycle progression. Similar recurrent ID3 mutations were independently identified in BL (but notably not in diffuse large BCL also carrying MYC translocations);Citation32,Citation72 however, no global gene expression differences between cells carrying wild type or mutant ID3 were evident in this study.Citation72
Interestingly, Love et al,Citation32 in addition to identifying ID3 mutations in BL, also identified recurrent mutations in PI3KR1. The class 1 PI3Ks are comprised of a heterodimer made up of a catalytic subunit (p110α, p110β, or p110δ) and one of three regulatory subunits (p85α, p55α, and p50α, which are all derived from the same gene, PI3KR1). It is note-worthy that the p55α regulatory subunit is induced following infection of primary B-cells with the BL-associated virus EBV, and is required for the survival and proliferation of lymphoblastoid cell lines.Citation73 However, the functional significance of the mutations in PI3KR1, or the differential induction of regulatory subunits by EBV, have not been investigated in the context of lymphoma and warrant further study.
As in other cancer types,Citation74 signaling through PI3K may also be involved in stabilizing MYC through regulation of GSK3β activity.Citation75 The MYC p-Thr58 modification, mediated by GSK3β and required for MYC degradation, can be blocked via a PI3K-dependent inhibitory phosphorylation of GSK3β on Ser9. Potentially, constitutive PI3K activation in BL carrying wild type MYC would help promote its stability and may contribute to its tumorigenic effects. In addition, PI3K/AKT activation in the context of MYC-induced transformation interferes with the oncogene-induced apoptotic response, again through an inhibitory modification, but this time, by phosphorylation of FOXO TCFs downstream of MYC. Although described in an artificial in vitro MycER system, inhibition of FOXO activity by AKT is required for MYC-induced transformation and proliferation,Citation64 and it has been reported that PI3K/AKT and MYC together cooperatively repress PUMA and GADD45a expression by blocking their FOXO-dependent transcription (independently of PUMA induction by p53).Citation76 If inhibition of FOXO-mediated transcription by PI3K/AKT signaling also occurs in MYC-driven lymphomas, it could potentially contribute to some of the cooperation seen between MYC and PI3K. Indeed, using a dominant negative FOXO protein to inhibit FOXO transcription accelerates lymphomagenesis in Eμ-MYC mice by attenuating MYC-induced apoptosis.Citation41 In this case, the authors attribute the effect to a loss of transcription of the p53 regulator p19ARF, which correlated with reduced p53 and PUMA induction. In either event, posttranslational modifications of AKT substrates involved in oncogene-induced apoptosis are likely, at least in part, to account for the cooperative effects of MYC and AKT seen during lymphomagenesis. The alternative potential prosurvival mechanisms of AKT, which could be involved in subverting MYC-induced apoptosis include phosphorylation of HDM2, which increases its ligase activity and results in enhanced degradation of p53,Citation77 blocking caspase activity,Citation78 and increasing expression of prosurvival BCL-2 family members, such as induced myeloid leukemia cell differentiation protein MCL-1 (discussed in the following section).
The role of the AKT substrate mTOR and therapeutic opportunities
In a recent in vitro mechanistic study using selective pharmacological inhibitors to treat BL cell lines, we analyzed which effectors downstream of PI3K are critical for BL survival.Citation22 In agreement with previous work,Citation66 apoptosis of BL cell lines is induced by the PI3K inhibitor (LY-294002) and also by the dual PI3K/mTOR inhibitor PI103. The AKT inhibitor (AKTi-VIII) also induces BL apoptosis as a single agent, confirming that PI3K signaling via AKT is important for BL survival. Data on Eμ-MYC mice treated with the allosteric AKT inhibitor MK-2206 have now also demonstrated that AKT activity is required for lymphoma progression in vivo.Citation79 However, further analysis using inhibitors of the downstream effectors of AKT activity have identified functions of mTOR in both BL survival and chemoresistance.
It should be noted that mTOR exists as two complexes: mTORC2 (acting upstream of AKT), and the AKT substrate mTORC1, which regulates the AKT effectors p70 S6-kinase/S6 ribosomal protein, and 4E-BP1/eIF4E. Using PP242, an active site adenosine triphosphate-competitive inhibitor of both mTORC1 and mTORC2, as well as low doses of rapamycin (an inhibitor of mTORC1), we were able to distinguish between the rapamycin-sensitive and rapamycin-insensitive effectsCitation80–Citation83 of mTORC1 signaling in order to dissect the critical pathway components. PP242 inhibited phosphorylation of both S6 ribosomal protein and 4E-BP1, whereas rapamycin only inhibited phosphorylation of the S6 ribosomal protein. These studies revealed that the proapoptotic effects of PI3K/AKT/mTOR inhibitors correlated with an inhibition of 4E-BP1/eIF4E function, suggesting that inhibition of cap-dependent protein translation mediates the apoptotic effect in BL cells. (It is worth noting here that higher doses of rapamycin can inhibit both S6K and 4E-BP1 and, therefore, do not always discriminate between different mTOR functions in every study reported.) It is possible that activation of the S6-kinase by mTOR could additionally have a positive effect on MYC-mediated transcription through the phosphorylation and degradation of the MYC inhibitor, MAD1,Citation84 but in terms of 4E-BP1 function, it is interesting to note that the activity of eIF4E has been previously reported to rescue cells from MYC-induced apoptosis.Citation85 We found that inhibition of the PI3K/AKT/mTOR signaling pathway in BL cells decreased the expression of both c-MYC and MCL-1 (a prosurvival member of the BCL-2 family). Both proteins, which are short-lived, require continual protein synthesis for the maintenance of expression levels such that the inhibition of cap-dependent translation would rapidly affect their level of expression. In support of these findings, enhanced mTORC1 activity in mice (in the Eμ-MYC/TSC2+/− model) accelerates MYC-driven oncogenesis through lower levels of apoptosis and increased mTORC1-dependent MCL-1 expression.Citation86 In addition, targeting mTORC1 with Everolimus (RAD001; Novartis AG, Basel, Switzerland), an inhibitor that is now being investigated in clinical trials, both protected Eμ-MYC mice from lymphoma development and induced regression of preformed lymphoma.Citation87 Further evidence to support the conclusion that 4E-BP1 activity is important for lymphoma survival has subsequently been reported using the pan PI3K/mTOR inhibitor BEZ235 in the Eμ-MYC murine model. In this case, apoptosis was reported to be dependent on upregulation of the BH3-only protein Bcl-2 modifying factor (BMF), which is induced in response to inhibition of 4E-BP1/eIF4E mediated 5’ cap-dependent protein translation.Citation88,Citation89 Furthermore Eμ-MYC lymphomas have been shown to have elevated levels of 4E-BP1 phosphorylation on mTOR-specific sites (Thr37/46) and the mTOR active site inhibitor MLN0128 was shown to induce apoptosis in the BL Raji cell line.Citation90
A promising outcome of this research then, in terms of identifying potential strategies for the treatment of BL, is that mTOR activity is an attractive target for therapeutic intervention. An important finding was that the combination of PI3K, AKT, or mTOR inhibitors act synergistically to induce apoptosis in combination with the BH3-mimetic compound ABT-737.Citation22 ABT-737 acts like BIM or PUMA to inhibit BCL-XL function and induce intrinsic mitochondrial apoptosis (). PI3K inhibition rendered previously resistant BL cells sensitive to the effects of ABT-737, indicating that PI3K can induce chemoresistance to agents that target one of the critical oncogene-induced apoptosis pathways. These data also imply that MCL-1 inhibitors could be effective in combination with BH3 mimetics or activators of wild type p53 as a therapeutic strategy in BL. Such combination therapies may offer significant advantages over current treatment regimens by reducing drug-associated toxicity.
The impact of Epstein–Barr virus on c-MYC and PI3K
EBV persistently infects greater than 90% of the population and remains, in most cases, benign for life. In some individuals, however, EBV infection is clearly associated with the development of BL. It is not entirely clear how EBV infection may contribute to disease pathogenesis but, presumably, EBV infection creates the right environment for cells to undergo, and perhaps more significantly to survive, the process of MYC gene translocation. How EBV may complement the activation of MYC to promote pathogenesis has been reviewed comprehensively elsewhere.Citation91 However, in light of new studies, which have now established that the PI3K/AKT/mTOR pathway is a critical partner in MYC-driven lymphomagenesis, it is worth revisiting some aspects of EBV biology that may impact on these pathways. It is perhaps no coincidence that infection with this tumor-associated virus shows remarkable mimicry of many of the effects of the genomic mutational events recently described in BL.
Following infection, an initial phase of B-cell activation and proliferation is driven by viral genes and orchestrated by the viral TCF Epstein–Barr nuclear antigen (EBNA)-2.92 Significantly, one of the main cellular genes targeted by EBNA-2 for this purpose is c-MYC.Citation93,Citation94 In addition, EBNA-2 and two EBV-encoded membrane proteins activate the PI3K pathway. EBNA-2 appears to induce phospho-AKT via induction of the microRNA, miR-21 (probably through the posttranscriptional processing rather than by the induction of the primary transcript).Citation95 Meanwhile, latent membrane protein (LMP)-2A, a mimic of functional BCRs, is expressed by EBV potentially to inhibit negative selection. LMP-2A constitutively activates PI3K,Citation96,Citation97 thus providing the cell with the “tonic” BCR-like survival signals that are evident following TCF3 and ID3 mutation in BL. Interestingly, LMP-2A is often detected in tumor biopsies of EBV-related malignancies, although LMP-2A is down-regulated during later stages of viral latency and can, therefore, play no further role in promoting cell survival. It seems likely then, that LMP-2A may have a potential role in augmenting the survival of cellsCitation98 carrying tumorigenic genetic abnormalities within the GC, possibly by PI3K/AKT-mediated upregulation of BCL-XL.Citation96 A second EBV encoded membrane protein, LMP-1, functionally mimics activated CD40,Citation99 requires no ligand, and is capable of transforming rodent fibroblasts.Citation100 LMP-1 signals via a number of intracellular pathways such as nuclear factor-kappa B,Citation101 mitogen-activated protein kinase,Citation102 Janus kinase,Citation103 but also, through the binding of its cytoplasmic tail to the p85 regulatory subunit, which signals via PI3K to AKT.Citation104,Citation105 Thus, through the expression of EBNA-2, LMP-1, and LMP-2A, EBV provides similar survival and transforming signals that have been shown to cooperatively induce malignant transformations in BL.
There are also numerous viral products that can block the ability of infected cells to mount an effective intrinsic apoptotic response to oncogene-induced stress (reviewed in Spender and Inman).Citation6 EBV blocks this response either by directly interfering with BAX/BAK function (mediated by the viral genes LMP-1, BHRF-1, and BALF-1), inducing expression of other prosurvival BCL-2 family members such as MCL-1Citation106 and BFL-1 (induced by LMP-1),Citation107 or by inhibiting the function or expression of the BH3-only proteins PUMA (BHRF-1, miR-BART5) and BIM (EBNAs-3A and 3C).Citation108,Citation109 Inhibiting the function of BIM and PUMA or inducing MCL-1 are, as discussed previously, also the consequence of promoter methylation, MYC mutation or increased mTORC activity, all described in BL.
In light of the evidence that EBV infection appears to recapitulate the events required for BL pathogenesis (ie, MYC induction, activation of PI3K, and inactivation of the intrinsic apoptosis pathway), one might expect that p53 oncogene-induced stress response would also be targeted. Indeed, two potent viral transcriptional repressors (EBNA-3A and 3C) are implicated in the repression of p14ARF,Citation110 which is required for p53 induction. There are also data reporting that EBV infection deubiquitinates and stabilizes the p53-negative regulator MDM2 (a function of EBNA-3C),Citation111 or mediates p53 degradation independently of MDM2 (BZLF-1),Citation112 thus reducing p53 expression. The formation of EBNA-leader protein (LP)/MDM2/p53 complexes has also been proposed to selectively block p53-mediated transcription (of p21) and provide an explanation as to how rapidly proliferating infected cells tolerate high levels of wild type p53 without succumbing to p53-induced cell cycle arrest.Citation113
One deviation from the hypothesis that EBV infection exactly mimics the changes seen in BL applies to the expression of cyclins. Mutation and expression of cyclin D3 is associated with BL pathogenesis; however, cyclin D3 expression is rarely seen in EBV-infected cells with an activated phenotype.Citation114 The viral TCF EBNA-2 does, however, indirectly induce cyclin D2.Citation94 Cyclin D2 can compensate for loss of cyclin D3, at least in some circumstances,Citation115 so perhaps cyclin D2 upregulation in the context of EBV infection has similar net pro-proliferative effects as cyclin D3 activity within BL.
Taken together, the data suggest that EBV infection may functionally mimic the BL cellular environment, perhaps enabling the genomic aberrations associated with BL to establish themselves during the tumorigenic process. The latent viral gene expression program that persists within BL is very restricted, involving only EBNA-1, which is required for viral genome maintenance, and two small RNA species called Epstein-Barr virus encoded small RNAs (EBER)1 and EBER2, whose potential targets are unknown. Both EBNA-1 and the EBERs, however, also appear to have a role in preventing BL cell apoptosis,Citation116 which could help promote lymphomagenesis. It would also be relevant to study the significance of infection with different EBV subtypes and their association with BL. EBV type 1 (also called type A) differs from type 2 (or type B) predominantly through sequence variation of the EBNA-2 gene. While the type A virus is much more efficient than the type B virus at driving proliferation of EBV-infected lymphoblastoid cell lines in vitroCitation117 (potentially via better induction of LMP-1 expression),Citation118 in some areas of endemic BL, infection with the type 2 strain predominates.Citation119 Differential regulation of certain cell genes does occur in response to type 1 and type 2 EBNA-2 expression,Citation118 but it is presently unclear whether these genes may significantly affect BL pathogenesis. The new models of BL pathogenesis targeting the cell of origin may help to decipher the cooperating roles of mutated and viral genes in BL development – in particular, which EBV genes are actually required, and when they are required, to contribute to BL development. Such studies will also provide excellent model systems in which to test new therapeutic strategies.
Acknowledgments
Research performed in our laboratory described in this review was funded by an Association for International Cancer Research Fellowship and by Cancer Research UK.
Disclosure
The authors report no conflicts of interest in this work.
References
- BurkittDA sarcoma involving the jaws in African childrenBr J Surg19584619721822313628987
- CrawfordDHBiology and disease associations of Epstein-Barr virusPhilos Trans R Soc Lond B Biol Sci2001356140846147311313005
- Dalla-FaveraRBregniMEriksonJPattersonDGalloRCCroceCMHuman c-myc onc gene is located on the region of chromosome 8 that is translocated in Burkitt lymphoma cellsProc Natl Acad Sci U S A19827924782478276961453
- TaubRKirschIMortonCTranslocation of the c-myc gene into the immunoglobulin heavy chain locus in human Burkitt lymphoma and murine plasmacytoma cellsProc Natl Acad Sci U S A19827924783778416818551
- MoormannAMSniderCJChelimoKThe company malaria keeps: how co-infection with Epstein-Barr virus leads to endemic Burkitt lymphomaCurr Opin Infect Dis2011245435441
- SpenderLCInmanGJInhibition of germinal centre apoptotic programmes by epstein-barr virusAdv Hematol2011201182952522110506
- ChangTCYuDLeeYSWidespread microRNA repression by Myc contributes to tumorigenesisNat Genet2008401435018066065
- O’DonnellKAWentzelEAZellerKIDangCVMendellJTc-Myc-regulated microRNAs modulate E2F1 expressionNature2005435704383984315944709
- BlackwellTKKretznerLBlackwoodEMEisenmanRNWeintraubHSequence-specific DNA binding by the c-Myc proteinScience1990250498411491151
- BlackwellTKHuangJMaABinding of myc proteins to canonical and noncanonical DNA sequencesMol Cell Biol1993139521652248395000
- LüscherBMAD1 and its life as a MYC antagonist: an updateEur J Cell Biol2012916–750651421917351
- TaubRMouldingCBatteyJActivation and somatic mutation of the translocated c-myc gene in burkitt lymphoma cellsCell19843623393486319017
- ar-RushdiANishikuraKEriksonJWattRRoveraGCroceCMDifferential expression of the translocated and the untranslocated c-myc oncogene in Burkitt lymphomaScience198322246223903936414084
- HaydayACGilliesSDSaitoHActivation of a translocated human c-myc gene by an enhancer in the immunoglobulin heavy-chain locusNature198430759493343406420706
- JohnstonLAProberDAEdgarBAEisenmanRNGallantPDrosophila myc regulates cellular growth during developmentCell199998677979010499795
- GrewalSSLiLOrianAEisenmanRNEdgarBAMyc-dependent regulation of ribosomal RNA synthesis during Drosophila developmentNat Cell Biol20057329530215723055
- BouchardCStellarPEilersMControl of cell proliferation by MycTrends Cell Biol1998852022069695840
- ParkSSKimJSTessarolloLInsertion of c-Myc into Igh induces B-cell and plasma-cell neoplasms in miceCancer Res20056541306131515735016
- YinXGiapCLazoJSProchownikEVLow molecular weight inhibitors of Myc-Max interaction and functionOncogene200322406151615913679853
- Gomez-CuretIPerkinsRSBennettRFeidlerKLDunnSPKruegerLJc-Myc inhibition negatively impacts lymphoma growthJ Pediatr Surg2006411207211 discussion 207–21116410134
- SampsonVBRongNHHanJMicroRNA let-7a down-regulates MYC and reverts MYC-induced growth in Burkitt lymphoma cellsCancer Res200767209762977017942906
- SpenderLCInmanGJPhosphoinositide 3-kinase/AKT/mTORC1/2 signaling determines sensitivity of Burkitt’s lymphoma cells to BH3 mimeticsMol Cancer Res201210334735922241218
- SchuhmacherMStaegeMSPajicAControl of cell growth by c-Myc in the absence of cell divisionCurr Biol19999211255125810556095
- SchlosserIHölzelMHoffmannRDissection of transcriptional programmes in response to serum and c-Myc in a human B-cell lineOncogene200524352052415516975
- MarinkovicDMarinkovicTMahrBHessJWirthTReversible lymphomagenesis in conditionally c-MYC expressing miceInt J Cancer2004110333634215095297
- SchuhmacherMKohlhuberFHölzelMThe transcriptional program of a human B cell line in response to MycNucleic Acids Res200129239740611139609
- JiHWuGZhanXCell-type independent MYC target genes reveal a primordial signature involved in biomass accumulationPLoS One2011610e2605722039435
- LinCYLovénJRahlPBTranscriptional amplification in tumor cells with elevated c-MycCell20121511566723021215
- LiZVan CalcarSQuCCaveneeWKZhangMQRenBA global transcriptional regulatory role for c-Myc in Burkitt’s lymphoma cellsProc Natl Acad Sci U S A2003100148164816912808131
- SeitzVButzhammerPHirschBDeep sequencing of MYC DNA-binding sites in Burkitt lymphomaPLoS One2011611e26837
- FanJZellerKChenYCTime-dependent c-Myc transactomes mapped by Array-based nuclear run-on reveal transcriptional modules in human B cellsPLoS One201053e969120300622
- LoveCSunZJimaDThe genetic landscape of mutations in Burkitt lymphomaNat Genet201244121321132523143597
- HannSREisenmanRNProteins encoded by the human c-myc oncogene: differential expression in neoplastic cellsMol Cell Biol1984411248624976513926
- SakamuroDPrendergastGCNew Myc-interacting proteins: a second Myc network emergesOncogene199918192942295410378691
- KuttlerFAméPClarkHc-myc box II mutations in Burkitt’s lymphoma-derived alleles reduce cell-transformation activity and lower response to broad apoptotic stimuliOncogene200120426084609411593416
- SearsRNuckollsFHauraETayaYTamaiKNevinsJRMultiple Ras-dependent phosphorylation pathways regulate Myc protein stabilityGenes Dev200014192501251411018017
- SalghettiSEKimSYTanseyWPDestruction of Myc by ubiquitin-mediated proteolysis: cancer-associated and transforming mutations stabilize MycEMBO J19991837177269927431
- GregoryMAHannSRc-Myc proteolysis by the ubiquitin-proteasome pathway: stabilization of c-Myc in Burkitt’s lymphoma cellsMol Cell Biol20002072423243510713166
- BahramFvon der LehrNCetinkayaCLarssonLGc-Myc hot spot mutations in lymphomas result in inefficient ubiquitination and decreased proteasome-mediated turnoverBlood20009562104211010706881
- HoangATLutterbachBLewisBCA link between increased transforming activity of lymphoma-derived MYC mutant alleles, their defective regulation by p107, and altered phosphorylation of the c-Myc transactivation domainMol Cell Biol1995158403140427623799
- BouchardCLeeSPaulus-HockVLoddenkemperCEilersMSchmittCAFoxO transcription factors suppress Myc-driven lymphomagenesis via direct activation of ArfGenes Dev200721212775278717974917
- EischenCMWeberJDRousselMFSherrCJClevelandJLDisruption of the ARF-Mdm2-p53 tumor suppressor pathway in Myc-induced lymphomagenesisGenes Dev199913202658266910541552
- PostSMQuintás-CardamaATerzianTSmithCEischenCMLozanoGp53-dependent senescence delays Emu-myc-induced B-cell lymphomagenesisOncogene20102991260126919935700
- FarrellPJAllanGJShanahanFVousdenKHCrookTp53 is frequently mutated in Burkitt’s lymphoma cell linesEMBO J19911010287928871915267
- VousdenKHCrookTFarrellPJBiological activities of p53 mutants in Burkitt’s lymphoma cellsJ Gen Virol199374Pt 58038108492087
- LeventakiVRodicVTrippSRTP53 pathway analysis in paediatric Burkitt lymphoma reveals increased MDM4 expression as the only TP53 pathway abnormality detected in a subset of casesBr J Haematol2012158676377122845047
- LindströmMSKlangbyUWimanKGp14ARF homozygous deletion or MDM2 overexpression in Burkitt lymphoma lines carrying wild type p53Oncogene200120172171217711360201
- PujalsARenoufBRobertAChelouahSHollvilleEWielsJTreatment with a BH3 mimetic overcomes the resistance of latency III EBV (+) cells to p53-mediated apoptosisCell Death Dis20112e18421796156
- EgleAHarrisAWBouilletPCorySBim is a suppressor of Myc-induced mouse B cell leukemiaProc Natl Acad Sci U S A2004101166164616915079075
- DangCVO’donnellKAJuopperiTThe great MYC escape in tumorigenesisCancer Cell20058317717816169462
- HemannMTBricATeruya-FeldsteinJEvasion of the p53 tumour surveillance network by tumour-derived MYC mutantsNature2005436705280781116094360
- EischenCMRousselMFKorsmeyerSJClevelandJLBax loss impairs Myc-induced apoptosis and circumvents the selection of p53 mutations during Myc-mediated lymphomagenesisMol Cell Biol200121227653766211604501
- PaschosKSmithPAndertonEMiddeldorpJMWhiteREAlldayMJEpstein-barr virus latency in B cells leads to epigenetic repression and CpG methylation of the tumour suppressor gene BimPLoS Pathog200956e100049219557159
- Richter-LarreaJARoblesEFFresquetVReversion of epigenetically mediated BIM silencing overcomes chemoresistance in Burkitt lymphomaBlood2010116142531254220570860
- ErlacherMLabiVManzlCPuma cooperates with Bim, the rate-limiting BH3-only protein in cell death during lymphocyte development, in apoptosis inductionJ Exp Med2006203132939295117178918
- GarrisonSPJeffersJRYangCSelection against PUMA gene expression in Myc-driven B-cell lymphomagenesisMol Cell Biol200828175391540218573879
- SpenderLCCarterMJO’BrienDITransforming growth factor-β directly induces p53-up-regulated modulator of apoptosis (PUMA) during the rapid induction of apoptosis in myc-driven B-cell lymphomasJ Biol Chem201328875198520923243310
- SpenderLCO’BrienDISimpsonDTGF-beta induces apoptosis in human B cells by transcriptional regulation of BIK and BCL-XLCell Death Differ200916459360219136942
- SanderSCaladoDPSrinivasanLSynergy between PI3K signaling and MYC in Burkitt lymphomagenesisCancer Cell201222216717922897848
- RohnJLHueberAOMcCarthyNJThe opposing roles of the Akt and c-Myc signalling pathways in survival from CD95-mediated apoptosisOncogene19981722281128189879987
- ZhaoJJGjoerupOVSubramanianRRHuman mammary epithelial cell transformation through the activation of phosphatidylinositol 3-kinaseCancer Cell20033548349512781366
- KumarAMarquésMCarreraACPhosphoinositide 3-kinase activation in late G1 is required for c-Myc stabilization and S phase entryMol Cell Biol200626239116912517015466
- HanSSYunHSonDJNF-kappaB/STAT3/PI3K signaling crosstalk in iMyc E mu B lymphomaMol Cancer201099720433747
- BouchardCMarquardtJBrásAMedemaRHEilersMMyc-induced proliferation and transformation require Akt-mediated phosphorylation of FoxO proteinsEMBO J200423142830284015241468
- CurnockAPKnoxKALY294002-mediated inhibition of phosphatidylinositol 3-kinase activity triggers growth inhibition and apoptosis in CD40-triggered Ramos-Burkitt lymphoma B cellsCell Immunol1998187277879732695
- BrennanPMehlAMJonesMRoweMPhosphatidylinositol 3-kinase is essential for the proliferation of lymphoblastoid cellsOncogene20022181263127111850846
- PeledJUYuJJVenkateshJRequirement for cyclin D3 in germinal center formation and functionCell Res2010206631646
- CatoMHChintalapatiSKYauIWOmoriSARickertRCCyclin D3 is selectively required for proliferative expansion of germinal center B cellsMol Cell Biol201131112713720956554
- MøllerMBNielsenOPedersenNTCyclin D3 expression in non-Hodgkin lymphoma. Correlation with other cell cycle regulators and clinical featuresAm J Clin Pathol2001115340441211242797
- SchmitzRYoungRMCeribelliMBurkitt lymphoma pathogenesis and therapeutic targets from structural and functional genomicsNature2012490741811612022885699
- GoldfarbANFloresJPLewandowskaKInvolvement of the E2A basic helix-loop-helix protein in immunoglobulin heavy chain class switchingMol Immunol19963311–129479568960119
- RichterJSchlesnerMHoffmannSICGC MMML-Seq ProjectRecurrent mutation of the ID3 gene in Burkitt lymphoma identified by integrated genome, exome and transcriptome sequencingNat Genet201244121316132023143595
- SpenderLCLucchesiWBodelonGCell target genes of Epstein-Barr virus transcription factor EBNA-2: induction of the p55alpha regulatory subunit of PI3-kinase and its role in survival of EREB2.5 cellsJ Gen Virol200687Pt 102859286716963743
- SchildCWirthMReichertMSchmidRMSaurDSchneiderGPI3K signaling maintains c-myc expression to regulate transcription of E2F1 in pancreatic cancer cellsMol Carcinog200948121149115819603422
- van WeerenPCde BruynKMde Vries-SmitsAMvan LintJBurgeringBMEssential role for protein kinase B (PKB) in insulin-induced glycogen synthase kinase 3 inactivation. Characterization of dominant-negative mutant of PKBJ Biol Chem19982732113150131569582355
- AmenteSZhangJLavaderaMLLaniaLAvvedimentoEVMajelloBMyc and PI3K/AKT signaling cooperatively repress FOXO3a-dependent PUMA and GADD45a gene expressionNucleic Acids Res201139229498950721835778
- AshcroftMLudwigRLWoodsDBPhosphorylation of HDM2 by AktOncogene200221131955196211960368
- CardoneMHRoyNStennickeHRRegulation of cell death protease caspase-9 by phosphorylationScience19982825392131813219812896
- DevlinJRHannanKMNgPYAKT signalling is required for ribosomal RNA synthesis and progression of Eμ-Myc B-cell lymphoma in vivoFEBS J Epub1192013
- HsiehACCostaMZolloOGenetic dissection of the oncogenic mTOR pathway reveals druggable addiction to translational control via 4EBP-eIF4ECancer Cell201017324926120227039
- DowlingRJTopisirovicIAlainTmTORC1-mediated cell proliferation, but not cell growth, controlled by the 4E-BPsScience201032859821172117620508131
- FeldmanMEApselBUotilaAActive-site inhibitors of mTOR target rapamycin-resistant outputs of mTORC1 and mTORC2PLoS Biol200972e3819209957
- ThoreenCCSabatiniDMRapamycin inhibits mTORC1, but not completelyAutophagy20095572572619395872
- ZhuJBlenisJYuanJActivation of PI3K/Akt and MAPK pathways regulates Myc-mediated transcription by phosphorylating and promoting the degradation of Mad1Proc Natl Acad Sci U S A2008105186584658918451027
- LiSTakasuTPerlmanDMTranslation factor eIF4E rescues cells from Myc-dependent apoptosis by inhibiting cytochrome c releaseJ Biol Chem200327853015302212441348
- MillsJRHippoYRobertFmTORC1 promotes survival through translational control of Mcl-1Proc Natl Acad Sci U S A200810531108531085818664580
- WallMPoortingaGStanleyKLThe mTORC1 inhibitor everolimus prevents and treats Eμ-Myc lymphoma by restoring oncogene-induced senescenceCancer Discov201331829523242809
- GrespiFSoratroiCKrumschnabelGBH3-only protein Bmf mediates apoptosis upon inhibition of CAP-dependent protein synthesisCell Death Differ201017111672168320706276
- ShorttJMartinBPNewboldACombined inhibition of PI3K-related DNA damage response kinases and mTORC1 induces apoptosis in MYC-driven B-cell lymphomasBlood2013121152964297423403624
- PourdehnadMTruittMLSiddiqiINDuckerGSShokatKMRuggeroDMyc and mTOR converge on a common node in protein synthesis control that confers synthetic lethality in Myc-driven cancersProc Natl Acad Sci U S A201311029119881199323803853
- AlldayMJHow does Epstein-Barr virus (EBV) complement the activation of Myc in the pathogenesis of Burkitt’s lymphoma?Semin Cancer Biol200919636637619635566
- SinclairAJPalmeroIPetersGFarrellPJEBNA-2 and EBNA-LP cooperate to cause G0 to G1 transition during immortalization of resting human B lymphocytes by Epstein-Barr virusEMBO J19941314332133288045261
- KaiserCLauxGEickDJochnerNBornkammGWKempkesBThe proto-oncogene c-myc is a direct target gene of Epstein-Barr virus nuclear antigen 2J Virol19997354481448410196351
- SpenderLCCornishGHRowlandBKempkesBFarrellPJDirect and indirect regulation of cytokine and cell cycle proteins by EBNA-2 during Epstein-Barr virus infectionJ Virol20017583537354611264343
- RosatoPAnastasiadouEGargNDifferential regulation of miR-21 and miR-146a by Epstein-Barr virus-encoded EBNA2Leukemia201226112343235222614176
- PortisTLongneckerREpstein-Barr virus (EBV) LMP2A mediates B-lymphocyte survival through constitutive activation of the Ras/PI3K/Akt pathwayOncogene200423538619862815361852
- MancaoCHammerschmidtWEpstein-Barr virus latent membrane protein 2A is a B-cell receptor mimic and essential for B-cell survivalBlood2007110103715372117682125
- Swanson-MungersonMACaldwellRGBultemaRLongneckerREpstein-Barr virus LMP2A alters in vivo and in vitro models of B-cell anergy, but not deletion, in response to autoantigenJ Virol200579127355736215919890
- GiresOZimber-StroblUGonnellaRLatent membrane protein 1 of Epstein-Barr virus mimics a constitutively active receptor moleculeEMBO J1997162061316140
- WangDLiebowitzDKieffEAn EBV membrane protein expressed in immortalized lymphocytes transforms established rodent cellsCell1985433 Pt 28318403000618
- IzumiKMKieffEDThe Epstein-Barr virus oncogene product latent membrane protein 1 engages the tumor necrosis factor receptor-associated death domain protein to mediate B lymphocyte growth transformation and activate NF-kappaBProc Natl Acad Sci U S A1997942312592125979356494
- RobertsMLCooperNRActivation of a ras-MAPK-dependent pathway by Epstein-Barr virus latent membrane protein 1 is essential for cellular transformationVirology1998240193999448693
- EliopoulosAGYoungLSActivation of the cJun N-terminal kinase (JNK) pathway by the Epstein-Barr virus-encoded latent membrane protein 1 (LMP1)Oncogene19981613173117429582021
- DawsonCWTramountanisGEliopoulosAGYoungLSEpstein-Barr virus latent membrane protein 1 (LMP1) activates the phosphatidylinositol 3-kinase/Akt pathway to promote cell survival and induce actin filament remodelingJ Biol Chem200327863694370412446712
- MainouBAEverlyDNJrRaab-TraubNEpstein-Barr virus latent membrane protein 1 CTAR1 mediates rodent and human fibroblast transformation through activation of PI3KOncogene200524466917692416007144
- WangSRoweMLundgrenEExpression of the Epstein Barr virus transforming protein LMP1 causes a rapid and transient stimulation of the Bcl-2 homologue Mcl-1 levels in B-cell linesCancer Res19965620461046138840972
- PegmanPMSmithSMD’SouzaBNEpstein-Barr virus nuclear antigen 2 trans-activates the cellular antiapoptotic bfl-1 gene by a CBF1/RBPJ kappa-dependent pathwayJ Virol200680168133814416873269
- ClybouwCMcHichiBMouhamadSEBV infection of human B lymphocytes leads to down-regulation of Bim expression: relationship to resistance to apoptosisJ Immunol200517552968297316116183
- AndertonEYeeJSmithPCrookTWhiteREAlldayMJTwo Epstein-Barr virus (EBV) oncoproteins cooperate to repress expression of the proapoptotic tumour-suppressor Bim: clues to the pathogenesis of Burkitt’s lymphomaOncogene200827442143317653091
- MaruoSZhaoBJohannsenEKieffEZouJTakadaKEpstein-Barr virus nuclear antigens 3C and 3A maintain lymphoblastoid cell growth by repressing p16INK4A and p14ARF expressionProc Natl Acad Sci U S A201110851919192421245331
- SahaAMurakamiMKumarPBajajBSimsKRobertsonESEpstein-Barr virus nuclear antigen 3C augments Mdm2-mediated p53 ubiquitination and degradation by deubiquitinating Mdm2J Virol20098394652466919244339
- SatoYShirataNKudohAExpression of Epstein-Barr virus BZLF1 immediate-early protein induces p53 degradation independent of MDM2, leading to repression of p53-mediated transcriptionVirology2009388120421119375142
- KashubaEYurchenkoMYenamandraSPEpstein-Barr virus-encoded EBNA-5 forms trimolecular protein complexes with MDM2 and p53 and inhibits the transactivating function of p53Int J Cancer2011128481782520473904
- PokrovskajaKEhlin-HenrikssonBBartkovaJPhenotype-related differences in the expression of D-type cyclins in human B cell-derived linesCell Growth Differ1996712172317328959341
- MatarazaJMTumangJRGuminaMRGurdakSMRothsteinTLChilesTCDisruption of cyclin D3 blocks proliferation of normal B-1a cells, but loss of cyclin D3 is compensated by cyclin D2 in cyclin D3-deficient miceJ Immunol2006177278779516818732
- BradyGMacarthurGJFarrellPJEpstein-Barr virus and Burkitt lymphomaPostgrad Med J20088499337237718716017
- RickinsonABYoungLSRoweMInfluence of the Epstein-Barr virus nuclear antigen EBNA2 on the growth phenotype of virus-transformed B cellsJ Virol1987615131013173033261
- LucchesiWBradyGDittrich-BreiholzOKrachtMRussRFarrellPJDifferential gene regulation by Epstein-Barr virus type 1 and type 2 EBNA2J Virol200882157456746618480445
- AitkenCSenguptaSKAedesCMossDJSculleyTBHeterogeneity within the Epstein-Barr virus nuclear antigen 2 gene in different strains of Epstein-Barr virusJ Gen Virol199475Pt 1951008113744