Abstract
Bronchopulmonary dysplasia (BPD) and chronic obstructive pulmonary disease (COPD) are chronic lung diseases of human infants and adults, respectively, that are characterized by alveolar simplification. One-third of the infants with severe BPD develop pulmonary hypertension (PH). More importantly, PH increases morbidity and mortality in BPD patients. Additionally, COPD is a common respiratory morbidity in former BPD patients. The lack of an appropriate small animal model wherein echocardiography (Echo) can demonstrate PH is one of the major barriers to understand the molecular mechanisms of the disease and, thereby, develop rational therapies to prevent and/or treat PH in BPD patients. Thus, the goal of this study was to establish a model of experimental BPD and PH and investigate the feasibility of Echo to diagnose PH in neonatal mice. Since hyperoxia-induced oxidative stress and inflammation contributes to the development of BPD with PH, we tested the hypothesis that exposure of newborn C57BL/6J mice to 70% O2 (hyperoxia) for 14 days leads to lung oxidative stress, inflammation, alveolar and pulmonary vascular simplification, pulmonary vascular remodeling, and Echo evidence of PH. Hyperoxia exposure caused lung oxidative stress and inflammation as evident by increased malondialdehyde adducts and inducible nitric oxide synthase, respectively. Additionally, hyperoxia exposure caused growth restriction, alveolar and pulmonary vascular simplification, and pulmonary vascular remodeling. At 14 days of age, Echo of these mice demonstrated that hyperoxia exposure decreased pulmonary acceleration time (PAT) and PAT/ejection time ratio and increased right ventricular free wall thickness, which are indicators of significant PH. Thus, we have demonstrated the feasibility of Echo to phenotype PH in neonatal mice with experimental BPD with PH, which can aid in discovery of therapies to prevent and/or treat BPD with PH and its sequelae such as COPD in humans.
Introduction
Bronchopulmonary dysplasia (BPD) is a chronic lung disease of infancy that results from interrupted lung alveolar and vascular growth.Citation1,Citation2 The pathogenesis and pathophysiology of BPD is identical to chronic obstructive pulmonary disease (COPD), which is a chronic lung disease of human adults.Citation3 Alveolar simplification is a unique histological pattern of BPD that is characterized by larger but fewer alveoli with decreased septation.Citation2 Despite major advances in the respiratory care of premature infants, BPD remains among the most prevalent condition in these patients.Citation4,Citation5 Infants with BPD are more likely to have long-term pulmonary and neurodevelopmental morbidities.Citation4,Citation6 Importantly, COPD is a common long-term respiratory morbidity of former BPD patients.Citation7–Citation10
Evidence implicates hyperoxia-induced generation of reactive oxygen species (ROS) and lung inflammation as the major contributors to the development of BPD and its sequelae.Citation11,Citation12 Supplemental oxygen (O2) is commonly administered as a life-saving measure in patients with impaired lung function. Although it relieves the immediate life-threatening consequences transiently, O2 may also exacerbate lung injury.Citation13 Excessive O2 exposure leads to increased ROS production, and the generated ROS react with nearby molecules (eg, protein, lipids, DNA, and RNA) and modify their structure and function, resulting in chronic pulmonary diseases such as BPD and COPD.Citation14–Citation18
Pulmonary hypertension (PH) is a severe form of pulmonary vascular disease that affects 25%–43% of infants with moderate-to-severe BPD.Citation19,Citation20 The pathogenesis of PH in BPD is complex and may result from interactions between antenatal risk factors such as pregnancy-induced hypertension, intrauterine growth restriction and infection, and postnatal risk factors such as oxidative stress, inflammation, infection, and mechanical ventilation in a preterm infant with underlying genetic susceptibility.Citation21 Importantly, PH increases short-and long-term morbidity and mortality, including COPD, in BPD patients.Citation21 Hence, there is an urgent need to improve therapies for BPD patients with PH.
Small animal models such as genetically modified mice offer a unique opportunity to understand the molecular mechanisms that contribute to the development of BPD and PH and, thereby, discover novel therapies. Although right heart catheterization is the gold standard to diagnose PH in adult mice,Citation22,Citation23 it is a terminal procedure and precludes long-term follow-up in these animals. Moreover, animal size is a major limitation to perform heart catheterization in newborn mice. Echocardiography (Echo) is a noninvasive technique that can reliably diagnose PH and can circumvent the catheterization-associated problems in mice. However, lack of Echo studies to diagnose PH in neonatal mice has precluded the development of reliable neonatal mouse models of PH-associated disorders such as BPD, which is pivotal to understand the pathogenesis and improving therapies for PH in human infants with BPD and preventing long-term respiratory morbidities such as COPD. Thus, the goal of this study was to provide a platform to discover early biomarkers and interventions to prevent BPD with PH and COPD in humans by investigating the feasibility of Echo to diagnose PH in newborn mice with experimental BPD and PH. Since hyperoxia-induced oxidative stress and inflammation contributes to the development of BPD with PH in human infants, we tested the hypothesis that exposure of newborn C57BL/6J mice to 70% O2 (hyperoxia) for 14 days leads to lung oxidative stress, inflammation, alveolar and pulmonary vascular simplification, pulmonary vascular remodeling, and Echo evidence of PH.
Materials and methods
Animals
This study was approved and conducted in strict accordance with the federal guidelines for the humane care and use of laboratory animals by the Institutional Animal Care and Use Committee of Baylor College of Medicine (Protocol number: AN-5631). The C57BL/6J wild-type mice were obtained from the Jackson Laboratory (Bar Harbor, ME, USA). Timed-pregnant mice raised in our animal facility were used for the experiments.
Exposure
Within 24 hours of birth, male and female pups from multiple litters were pooled before being randomly and equally redistributed to the dams, following which they were exposed to either 21% O2 (air, n=21) or 70% O2 (hyperoxia, n=21) for 14 days. During each experiment, four dams with 4–8 pups/dam were equally allocated to air and hyperoxic conditions. The dams were rotated between air- and hyperoxia-exposed litters every 48 hours to prevent oxygen toxicity in the dams and to eliminate maternal effects between the groups. Oxygen exposures were conducted in Plexiglas chambers, and the animals were monitored as described previously.Citation24
Tissue preparation for lung morphometry
After completion of experiments, a subset of pups were euthanized, their lungs were inflated and fixed via the trachea with 10% formalin at 25 cm H2O pressure for at least 10 minutes, and sections of the paraffin-embedded lungs were obtained for the analysis of lung morphometry as described previously.Citation24
Lung morphometry
As per American Thoracic Society/European Respiratory Society task force guidelines,Citation25 a systematic, uniform, random sampling principle was used to evaluate the lung sections for morphometry. Alveolar development on selected mice (n=9/group) was evaluated by radial alveolar counts (RAC) and mean linear intercepts (MLI). The observers performing the measurements were masked to the slide identity. RAC was determined as described by Cooney and Thurlbeck.Citation26 RAC measurements were made by dropping a perpendicular line from the center of a respiratory bronchiole to the edge of the septum or pleura and counting the number of alveoli traversed by this line. MLIs were assessed as described previously.Citation27 Briefly, grids of horizontal and vertical lines were superimposed on an image and the number of times the lines intersected with the tissue was counted. The total length of the grid lines was then divided by the number of intersections to provide the mean linear intercept in micrometer. Photographs from at least ten random nonoverlapping lung fields (10× magnification) were taken from each animal for RAC and MLI measurements.
Analyses of pulmonary vascularization
Pulmonary vessel density was determined based on immunohistochemical staining for von Willebrand factor (vWF), which is an endothelial specific marker. At least ten counts from ten random nonoverlapping fields (20× magnification) were performed for each animal (n=9/group).
Analyses of pulmonary vascular remodeling
Pulmonary vascular remodeling, which reflects significant PH, was determined by the medial thickness index (n=9/group) of resistance pulmonary arteries (20–150 µm external diameter) calculated using the equation: Medial thickness index = [(areaext − areaint)/areaext] ×100, where areaext and areaint are the areas within the external and internal boundaries of the α-smooth muscle actin (α-SMA) layer, respectively.Citation28 Additionally, α-SMA, which is a marker of vascular smooth muscle cells, was quantified by immunoblotting lung proteins using anti-α-SMA (Sigma-Aldrich Co., St Louis, MO, USA; A5228, dilution 1:1,000) and anti-β-actin (Santa Cruz Biotechnologies, Santa Cruz, CA, USA; sc-47778, dilution 1:2,000) antibodies.
Analysis of lung oxidative stress and inflammation
Malondialdehyde (MDA) is a stable end product of lipid peroxidation and is a generally accepted marker of oxidative stress.Citation29 Likewise, inducible nitric oxide synthase (iNOS) is a well-known marker of lung inflammation.Citation30 Hence, we performed immunoblotting on lung proteins using anti-MDA (Cell Biolabs, Inc., San Diego, CA, USA; STA-331, dilution 1:1,000), anti-iNOS (Santa Cruz Biotechnologies; sc-7271, dilution 1:1,000), and anti-β-actin (Santa Cruz Biotechnologies; sc-47778, dilution 1:2,000) antibodies. The primary antibodies were detected by incubation with the appropriate horseradish peroxidase-conjugated secondary antibodies. The immunoreactive bands were detected by chemiluminescence methods, and the band density was analyzed by Image J software (National Institutes of Health, Bethesda, MD, USA).
Echocardiography
PH was assessed by performing functional Echo in mice as described previously.Citation31,Citation32 Briefly, the mice (n=11/group) were anesthetized by using inhaled isoflurane via facemask and subjected to transthoracic two-dimensional, M-mode, and pulsed-wave Doppler (PWD) Echo using the VisualSonics Vevo 2100 machine (VisualSonics Inc., Toronto, ON, Canada) and a 40 MHz linear transducer. Right ventricular free wall (RVFW) thickness was measured during end diastole in the right parasternal long-axis view by two-dimensional and M-mode Echo. PWD recording of the pulmonary blood flow was obtained at the level of the aortic valve in the parasternal short axis view to measure pulmonary acceleration time (PAT, defined as the time from the onset of flow to peak velocity), and RV ejection time (ET, the time from the onset to the termination of pulmonary flow).
Statistical analyses
The results were analyzed by GraphPad Prism 5 software (La Jolla, CA, USA). At least three separate experiments were performed for each measurement (n= total animals from the three experiments), and the data are expressed as mean ± SD. The effects of exposure for the outcome variables were assessed using Student’s t-test. A P-value of <0.05 was considered significant.
Results and discussion
The hallmarks of a murine hyperoxia model that mimics BPD with PH in human preterm infants include growth restriction, lung oxidative stress and inflammation, interruption in alveolar and pulmonary vascular development (alveolar and pulmonary vascular simplification), pulmonary vascular remodeling, and decreased PAT/ET with increased RVFW thickness (PH). In this study, we investigated the effects of 70% O2 (hyperoxia) on these hallmarks and demonstrated that exposure of newborn wild-type mice to hyperoxia for 14 days increases lung oxidative stress and inflammation and causes alveolar and pulmonary vascular simplification, pulmonary vascular remodeling, and PH. Additionally, our study demonstrates the feasibility of Echo to elucidate PH in these mice at 14 days postnatal age. Our studies are important to identify novel interventions to prevent and/or treat BPD and PH and, thereby, prevent COPD in former human preterm infants with BPD and PH.
The concentration of oxygen used in this study was comparable to those used in previous studies.Citation33–Citation35 We chose 70% oxygen for our experiments because it was the lowest oxygen concentration that caused significant alveolar and pulmonary vascular simplification and PH in our mouse model. The survival rate of pups exposed to air and hyperoxia was identical. Hyperoxia exposure is known to restrict growth by various mechanisms in neonatal mouse models of experimental BPD.Citation36–Citation40 We observed a similar finding. Although the body weights were comparable at birth, 70% O2 (hyperoxia) exposure for 14 days decreased the body weight by 21% when compared to corresponding air-breathing animals ().
Figure 1 Hyperoxia exposure decreases body weight in neonatal mice.
Abbreviation: SD, standard deviation.
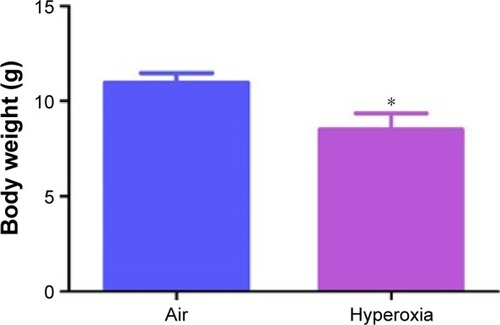
Oxidative stress contributes to the development of BPD with PH. It is difficult to measure and characterize hyperoxia-induced ROS in real time as they are very unstable. Hence, we determined the expression of MDA-protein adducts, which are stable aldehyde end products of ROS-mediated lipid peroxidation.Citation41 Our finding of hyperoxia induced MDA adducts in mice () are consistent with previous studiesCitation42,Citation43 and suggests that hyperoxia exposure causes oxidative stress in the lungs. Interestingly, hyperoxia induced MDA adducts in two specific regions between 40 and 80 kDa. This finding suggests that hyperoxia causes oxidative modifications of specific lung proteins in our model. The other possibility is that these proteins are abundant in the mouse lungs and are susceptible to hyperoxia-induced oxidative modification. Further studies are needed to identity these specific proteins because they could serve as potential biomarkers for oxidative stress in human infants with BPD. In addition to oxidative stress, inflammation plays a key role in the pathogenesis of BPD.Citation44 NO is used as a marker of respiratory tract inflammation in patients with asthma.Citation45 Three isoforms of NOS, neuronal NOS (nNOS, NOS-1), inducible NOS (iNOS, NOS-2), and endothelial NOS (eNOS, NOS-3), generate NO from the amino acid L-arginine. Although NO is critical for the homeostasis of lungs, excessive NO production increases nitrative stress and exerts proinflammatory effects. Inflammatory stimuli such as cytokines, chemokines, bacterial toxins, viral infections, allergens, hypoxia, and hyperoxia augment lung iNOS expression,Citation30,Citation46 which suggests that increased iNOS expression is biomarker of ongoing inflammation. Consistent with other murine hyperoxia models,Citation46,Citation47 hyperoxia exposure increased lung iNOS protein expression (), which indicates the presence of underlying lung inflammation.
Figure 2 Hyperoxia exposure increases lung MDA protein levels.
Notes: Lung protein obtained from neonatal mice exposed to air or hyperoxia for 14 days was subjected to immunoblotting using anti-MDA or -β-actin antibodies. Representative immunoblot showing differential MDA protein adduct expression in the region between 40 and 80 kDa (A). Densitometric analysis wherein the aforementioned MDA protein adduct band intensities were quantified and normalized to β-actin (B). Values are mean ± SD from four individual animals in each group from one experiment. Significant differences between air and hyperoxia groups are indicated by *P<0.05.
Abbreviations: MDA, malondialdehyde; SD, standard deviation.
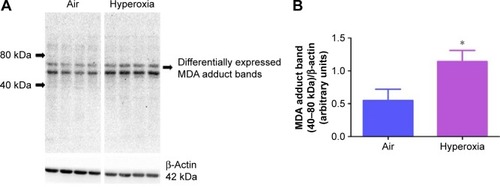
Figure 3 Hyperoxia exposure increases lung iNOS protein levels.
Abbreviations: iNOS, inducible nitric oxide synthase; SD, standard deviation.
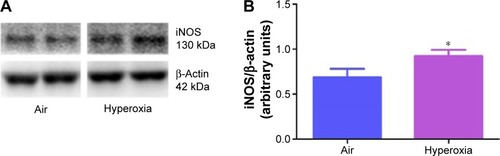
Hyperoxia is known to interrupt alveolar development by mechanisms entailing cell proliferation, cell death, and disruption of lung developmental signaling pathways,Citation48 which collectively results in alveolar simplification in preterm infantsCitation1,Citation2 and newborn mice.Citation49 In line with these studies, exposure to hyperoxia for 14 days decreased RAC by 35% () and increased MLI by 50% (), indicating that their alveoli were fewer in number and larger in diameter (alveolar simplification), respectively, when compared to corresponding air-breathing animals ().
Figure 4 Hyperoxia exposure induces alveolar simplification.
Abbreviations: WT, wild type; RAC, radial alveolar count; MLI, mean linear intercept; SD, standard deviation.
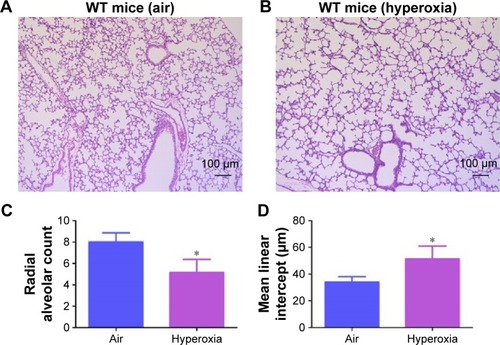
Pulmonary vascular and alveolar development are highly orchestrated interdependent processes and studies clearly support this concept by demonstrating that an interruption of distal lung angiogenesis secondary to decreased expression of vascular endothelial growth factor (VEGF) and/or its signaling receptor, vascular endothelial growth factor receptor2, leads to alveolar simplification.Citation50,Citation51 Additionally, pulmonary vascular simplification decreases pulmonary vascular surface area leading to high pulmonary vascular resistance and PH.Citation52 Our findings of hyperoxia-induced decrease in vWF-stained lung blood vessels () suggest that hyperoxia causes pulmonary vascular simplification, which is consistent with studies in human preterm infantsCitation1,Citation2 and newborn mice.Citation53
Figure 5 Hyperoxia exposure decreases pulmonary vascular density.
Abbreviations: WT, wild type; vWF, von Willebrand factor; SD, standard deviation.
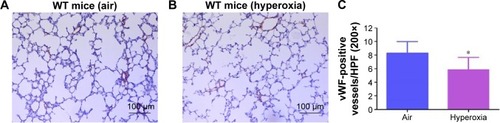
Pulmonary vascular remodeling secondary to increased smooth muscle cell proliferation of resistance pulmonary arteries is an additional risk factor that increases pulmonary vascular resistance and contributes to PH in BPD patients.Citation21 Consistent with several rodent models of experimental BPD with PH,Citation28,Citation35,Citation54 hyperoxia increased pulmonary vascular remodeling (), which indicates the presence of significant PH in our experimental animals. Two-dimensional and Doppler Echo have been used in several murine models to effectively assess heart function, chamber dimensions and thickness, and vascular properties.Citation55 It is a commonly used imaging modality in small animals because it is noninvasive, inexpensive, versatile, and is ideal for serial studies. PH is characterized by increased pulmonary artery (PA) and right ventricular systolic pressure, which is usually estimated indirectly from the tricuspid regurgitation (TR) peak flow velocity using Echo in human patients.Citation56 However, because of technical limitations preventing proper flow alignment, the measurement of TR by Doppler is inaccurate in mice. Moreover, TR is rare in rodents and occurs only with severe PH.Citation57 Right ventricular systolic time intervals such as PAT (the time from the onset of pulmonary flow to peak velocity) and ET (the time from onset to end of systolic flow) can be accurately obtained by high-resolution PWD Echo in rodents and are used as alternative indices of PA pressure in these animals.Citation31,Citation32 Although Echo has been the standard to diagnose PH in older murine models, there is lack of data on its feasibility to demonstrate PH in neonatal mice. In neonatal mouse models of BPD with PH, Echo has been used to diagnose PH several weeks later in the postneonatal period.Citation34,Citation58 This comes with a limitation of missing important earlier time points where the interventions can be targeted to successfully prevent and/or treat BPD with PH. Hence, we conducted Echo studies in neonatal mice with experimental BPD with PH.
Figure 6 Hyperoxia exposure induces pulmonary vascular remodeling.
Abbreviations: WT, wild type; α-SMA, alpha smooth muscle actin; SD, standard deviation.
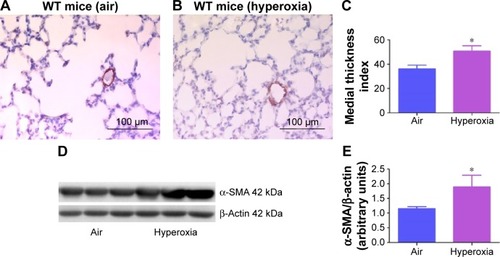
At a normal systolic pressure, the Doppler Echo pattern of pulmonary systolic flow is symmetric. With PH, the Doppler flow pattern becomes asymmetric, with the peak velocity occurring earlier because the PAT is decreased as the pulmonary valve closes prematurely due to high PA pressure. The reduced PAT also leads to a decrease in the ratio of PAT/ET. The normalization of PAT by ET offsets some of the confounders such as heart rateCitation59 and cardiac outputCitation60 that might independently affect the PAT. PAT and PAT/ET ratio estimated using high-frequency Echo have been shown to correlate with PA pressure measured by cardiac catheterization. In agreement with studies in older rodents, Doppler Echo of PA showed that exposure of neonatal mice to hyperoxia decreased PAT by 27% () and the ratio of PAT/ET by 28% (), resulting in an asymmetric flow pattern () when compared to air-breathing animals (). Additionally, right ventricular hypertrophy (RVH) reflects severe PH, and RVFW thickness measured in diastole is shown to strongly correlate with RVH.Citation32 Therefore, we determined RVFW thickness in diastole using M-mode Echo in our experimental animals. Consistent with its effects on other indices of PH, hyperoxia exposure increased RVFW by 70% () compared to air-breathing animals (). The Echo findings clearly demonstrate that our experimental animals have significant PH.
Figure 7 Hyperoxia exposure induces PH.
Notes: Representative PWD Echo recording of PA blood flow obtained at 14 days of age from neonatal mice exposed to air (A) or hyperoxia (B). PAT (C) and PAT/ET ratio (D) were estimated from the PWD Echo recordings of the PA blood flow. Values are mean ± SD from four individual animals in each group from one experiment. Significant differences between air and hyperoxia groups are indicated by *P<0.05.
Abbreviations: PWD, pulsed-wave Doppler; Echo, echocardiography; PA, pulmonary artery; PAT, pulmonary acceleration time; ET, ejection time; SD, standard deviation; PH, pulmonary hypertension.
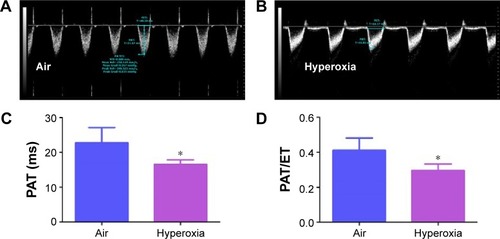
Figure 8 Hyperoxia exposure induces RVH.
Abbreviations: RVH, right ventricular hypertrophy; RVFW, right ventricular free wall; Echo, echocardiography; SD, standard deviation.

In summary, we have established a model of experimental BPD with PH and demonstrated that noninvasive assessment of PH is feasible in neonatal C57BL/6J mice using high-resolution Echo. To the best of our knowledge, this is the first study to demonstrate PH using high-resolution Echo in neonatal mice at 14 days postnatal age. This animal model may offer the unique opportunity to identify pathophysiological mechanisms that contribute to PH and to develop therapeutic strategies to prevent and/or treat BPD with PH in human preterm infants and thereby prevent adult-onset COPD in former BPD patients. Additionally, our findings have important implications for research in the prevention and treatment of other congenital disorders such as pulmonary hypoplasia, congenital diaphragmatic hernia, and congenital heart diseases that are associated with PH in human infants.
Acknowledgments
This work was supported by grants from the National Institutes of Health (NIH) HD-073323, American Heart Association BGIA-20190008, and American Lung Association RG-349917 to BS, and by the Mouse Phenotyping Core at Baylor College of Medicine with funding from the NIH (U54 HG006348). We thank Pamela Parsons for her timely processing of histopathology slides.
Disclosure
The authors report no conflicts of interest in this work.
References
- JobeAJThe new BPD: an arrest of lung developmentPediatr Res199946664164310590017
- HusainANSiddiquiNHStockerJTPathology of arrested acinar development in postsurfactant bronchopulmonary dysplasiaHum Pathol19982977107179670828
- BourbonJRBoucheratOBoczkowskiJCrestaniBDelacourtCBronchopulmonary dysplasia and emphysema: in search of common therapeutic targetsTrends Mol Med200915416917919303361
- FanaroffAAStollBJWrightLLTrends in neonatal morbidity and mortality for very low birthweight infantsAm J Obstet Gynecol20071962147e141e14817306659
- Van MarterLJEpidemiology of bronchopulmonary dysplasiaSemin Fetal Neonatal Med200914635836619783238
- ShortEJKleinNKLewisBACognitive and academic consequences of bronchopulmonary dysplasia and very low birth weight: 8-year-old outcomesPediatrics20031125e35914595077
- BhandariAMcGrath-MorrowSLong-term pulmonary outcomes of patients with bronchopulmonary dysplasiaSemin Perinatol201337213213723582968
- BoucheratOMorissetteMCProvencherSBonnetSMaltaisFBridging lung development with chronic obstructive pulmonary disease. Relevance of developmental pathways in chronic obstructive pulmonary disease pathogenesisAm J Respir Crit Care Med2016193436237526681127
- CarraroSFilipponeMDa DaltLBronchopulmonary dysplasia: the earliest and perhaps the longest lasting obstructive lung disease in humansEarly Hum Dev201389Suppl 3S3S523932350
- GibsonAMDoyleLWRespiratory outcomes for the tiniest or most immature infantsSemin Fetal Neonatal Med201419210511124239022
- BhandariVHyperoxia-derived lung damage in preterm infantsSemin Fetal Neonatal Med201015422322920430708
- WrightCJKirpalaniHTargeting inflammation to prevent bronchopulmonary dysplasia: can new insights be translated into therapies?Pediatrics2011128111112621646264
- ThielMChoukerAOhtaAOxygenation inhibits the physiological tissue-protecting mechanism and thereby exacerbates acute inflammatory lung injuryPLoS Biol200536e17415857155
- BhandariVChoo-WingRLeeCGHyperoxia causes angiopoietin 2-mediated acute lung injury and necrotic cell deathNat Med200612111286129317086189
- BagdonasERaudoniuteJBruzauskaiteIAldonyteRNovel aspects of pathogenesis and regeneration mechanisms in COPDInt J Chron Obstruct Pulmon Dis201510995101326082624
- BernardoIBozinovskiSVlahosRTargeting oxidant-dependent mechanisms for the treatment of COPD and its comorbiditiesPharmacol Ther2015155607926297673
- CarpagnanoGEKharitonovSAFoschino-BarbaroMPRestaOGramiccioniEBarnesPJSupplementary oxygen in healthy subjects and those with COPD increases oxidative stress and airway inflammationThorax200459121016101915563698
- RahmanIThe role of oxidative stress in the pathogenesis of COPD: implications for therapyTreat Respir Med20054317520015987234
- KhemaniEMcElhinneyDBRheinLPulmonary artery hypertension in formerly premature infants with bronchopulmonary dysplasia: clinical features and outcomes in the surfactant eraPediatrics200712061260126918055675
- MouraniPMIvyDDGaoDAbmanSHPulmonary vascular effects of inhaled nitric oxide and oxygen tension in bronchopulmonary dysplasiaAm J Respir Crit Care Med200417091006101315184202
- MouraniPMAbmanSHPulmonary hypertension and vascular abnormalities in bronchopulmonary dysplasiaClin Perinatol201542483985526593082
- SteinerMKSyrkinaOLKolliputiNMarkEJHalesCAWaxmanABInterleukin-6 overexpression induces pulmonary hypertensionCirc Res20091042236244228 following 24419074475
- MedoffBDOkamotoYLeytonPAdiponectin deficiency increases allergic airway inflammation and pulmonary vascular remodelingAm J Respir Cell Mol Biol200941439740619168697
- ShivannaBZhangWJiangWFunctional deficiency of aryl hydrocarbon receptor augments oxygen toxicity-induced alveolar simplification in newborn miceToxicol Appl Pharmacol2013267320921723337360
- HsiaCCHydeDMOchsMWeibelERAn official research policy statement of the American Thoracic Society/European Respiratory Society: standards for quantitative assessment of lung structureAm J Respir Crit Care Med2010181439441820130146
- CooneyTPThurlbeckWMThe radial alveolar count method of Emery and Mithal: a reappraisal 1 – postnatal lung growthThorax19823785725797179185
- van EijlSMortazEVersluisCNijkampFPFolkertsGBloksmaNA low vitamin A status increases the susceptibility to cigarette smoke-induced lung emphysema in C57BL/6J miceJ Physiol Pharmacol201162217518221673365
- AslamMBavejaRLiangODBone marrow stromal cells attenuate lung injury in a murine model of neonatal chronic lung diseaseAm J Respir Crit Care Med2009180111122113019713447
- Del RioDStewartAJPellegriniNA review of recent studies on malondialdehyde as toxic molecule and biological marker of oxidative stressNutr Metab Cardiovasc Dis200515431632816054557
- RicciardoloFLSterkPJGastonBFolkertsGNitric oxide in health and disease of the respiratory systemPhysiol Rev200484373176515269335
- ThibaultHBKurtzBRaherMJNoninvasive assessment of murine pulmonary arterial pressure: validation and application to models of pulmonary hypertensionCirc Cardiovasc Imaging20103215716320044514
- UrbonieneDHaberIFangYHThenappanTArcherSLValidation of high-resolution echocardiography and magnetic resonance imaging vs. high-fidelity catheterization in experimental pulmonary hypertensionAm J Physiol Lung Cell Mol Physiol20102993L401L41220581101
- WangHJafriAMartinRJSeverity of neonatal hyperoxia determines structural and functional changes in developing mouse airwayAm J Physiol Lung Cell Mol Physiol20143074L295L30124951774
- HansmannGFernandez-GonzalezAAslamMMesenchymal stem cell-mediated reversal of bronchopulmonary dysplasia and associated pulmonary hypertensionPulm Circ20122217018122837858
- HeilmanRPLagoskiMBLeeKJRight ventricular cyclic nucleotide signaling is decreased in hyperoxia-induced pulmonary hypertension in neonatal miceAm J Physiol Heart Circ Physiol201530812H1575H158225862831
- ChenHJChiangBLEffect of hyperoxia on retinoid metabolism and retinoid receptor expression in the lungs of newborn micePloS One20151010e014034326509921
- FritzellJAJrMaoQGundavarapuSFate and effects of adult bone marrow cells in lungs of normoxic and hyperoxic newborn miceAm J Respir Cell Mol Biol200940557558718988921
- GortnerLMonzDMildauCBronchopulmonary dysplasia in a double-hit mouse model induced by intrauterine hypoxia and postnatal hyperoxia: closer to clinical features?Ann Anat2013195435135823684450
- JonesCVAlikhanMAO’ReillyMThe effect of CSF-1 administration on lung maturation in a mouse model of neonatal hyperoxia exposureRespir Res20141511025192716
- RogersLKTippleTENelinLDWeltySEDifferential responses in the lungs of newborn mouse pups exposed to 85% or >95% oxygenPediatr Res2009651333818703992
- MooreKRobertsLJ2ndMeasurement of lipid peroxidationFree Radic Res19982866596719736317
- LiHDZhangZRZhangQXQinZCHeDMChenJSTreatment with exogenous hydrogen sulfide attenuates hyperoxia-induced acute lung injury in miceEur J Appl Physiol201311361555156323307012
- ShivannaBZhangSPatelAOmeprazole attenuates pulmonary aryl hydrocarbon receptor activation and potentiates hyperoxia-induced developmental lung injury in newborn miceToxicol Sci2015148127628726272953
- SaugstadODBronchopulmonary dysplasia-oxidative stress and antioxidantsSemin Neonatol200381394912667829
- KharitonovSAYatesDRobbinsRABarnesPJLogan-SinclairRShinebourneEAIncreased nitric oxide in exhaled air of asthmatic patientsLancet199434388901331357904001
- RadomskiASawickiGOlsonDMRadomskiMWThe role of nitric oxide and metalloproteinases in the pathogenesis of hyperoxia-induced lung injury in newborn ratsBr J Pharmacol19981257145514629884073
- DemchenkoITAtochinDNGutsaevaDRContributions of nitric oxide synthase isoforms to pulmonary oxygen toxicity, local vs mediated effectsAm J Physiol Lung Cell Mol Physiol20082945L984L99018326824
- SilvaDMNardielloCPozarskaAMortyRERecent advances in the mechanisms of lung alveolarization and the pathogenesis of bronchopulmonary dysplasiaAm J Physiol Lung Cell Mol Physiol201530911L1239L127226361876
- WarnerBBStuartLAPapesRAWispeJRFunctional and pathological effects of prolonged hyperoxia in neonatal miceAm J Physiol19982751 Pt 1L110L1179688942
- ThebaudBAbmanSHBronchopulmonary dysplasia: where have all the vessels gone? Roles of angiogenic growth factors in chronic lung diseaseAm J Respir Crit Care Med20071751097898517272782
- McGrath-MorrowSAChoCChoCZhenLHicklinDJTuderRMVascular endothelial growth factor receptor 2 blockade disrupts postnatal lung developmentAm J Respir Cell Mol Biol200532542042715722510
- NairJLakshminrusimhaSUpdate on PPHN: mechanisms and treatmentSemin Perinatol2014382789124580763
- ParkMSRieger-FackeldeyESchanbacherBLAltered expressions of fibroblast growth factor receptors and alveolarization in neonatal mice exposed to 85% oxygenPediatr Res200762665265717957151
- ChenSRongMPlatteauACTGF disrupts alveolarization and induces pulmonary hypertension in neonatal mice: implication in the pathogenesis of severe bronchopulmonary dysplasiaAm J Physiol Lung Cell Mol Physiol20113003L330L34021239535
- CollinsKAKorcarzCELangRMUse of echocardiography for the phenotypic assessment of genetically altered micePhysiol Genom2003133227239
- McLaughlinVVArcherSLBadeschDBACCF/AHA 2009 expert consensus document on pulmonary hypertension: a report of the American College of Cardiology Foundation Task Force on Expert Consensus Documents and the American Heart Association: developed in collaboration with the American College of Chest Physicians, American Thoracic Society, Inc., and the Pulmonary Hypertension AssociationCirculation2009119162250229419332472
- JonesJEMendesLRuddMARussoGLoscalzoJZhangYYSerial noninvasive assessment of progressive pulmonary hypertension in a rat modelAm J Physiol Heart Circ Physiol20022831H364H37112063310
- RamaniMBradleyWEDell’ItaliaLJAmbalavananNEarly exposure to hyperoxia or hypoxia adversely impacts cardiopulmonary developmentAm J Respir Cell Mol Biol201552559460225255042
- LeightonRFWeisslerAMWeinsteinPBWooleyCFRight and left ventricular systolic time intervals. Effects of heart rate, respiration and atrial pacingAm J Cardiol197127166725538714
- ChanKLCurriePJSewardJBHaglerDJMairDDTajikAJComparison of three Doppler ultrasound methods in the prediction of pulmonary artery pressureJ Am Coll Cardiol1987935495543546460