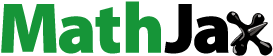
Abstract
Targeted drug delivery using polymeric nanostructures is an emerging cancer research area, engineered for safer, more efficient, and effective use of chemotherapeutic drugs. A pH-responsive, active targeting delivery system was designed using folic acid functionalized amphiphilic alternating copolymer poly(styrene-alt-maleic anhydride) (FA-DABA-SMA) via a biodegradable linker 2,4-diaminobutyric acid (DABA). The polymeric template is pH responsive, forming amphiphilic nanostructures at pH 7, allowing the encapsulation of hydrophobic drugs on its interior. Moreover, the structure is stable only at neutral pH and collapses in the acidic tumor microenvironment, releasing drugs on-site from its core. The delivery vehicle is investigated using human pancreatic PANC-1 cancer cells and RAW-Blue™ mouse macrophage reporter cell line, both of which have overly expression of folic acid receptors. To trace the cellular uptake by both cell lines, curcumin was selected as a dye and drug mimic owing to its fluorescence nature and hydrophobic properties. Fluorescent microscopy of FA-DABA-SMA loaded with curcumin revealed a significant internalization of the dye by human pancreatic PANC-1 cancer cells compared to those with unfunctionalized polymers (SMA). Moreover, the FA-DABA-SMA polymers exhibit rodlike association specific to the cells. Both empty SMA and FA-DABA-SMA show little toxicity to PANC-1 cells as characterized by WST-1 cell proliferation assay. These results clearly indicate that FA-DABA-SMA polymers show potential as an active tumor targeting drug delivery system with the ability to internalize hydrophobic chemotherapeutics after they specifically attach to cancer cells.
Introduction
Conventional chemotherapy is one of the most common treatments for cancer; however, current technologies suffer from limitations such as poor aqueous solubility, which causes elevated toxicity, lack of selectivity toward cancer cells, and multiple drug resistance against treatments.Citation1 The application of nanotechnology to deliver drugs to tumor cells is an attractive alternative. It allows drug to be delivered to cancer cells specifically with prolonged circulation time and with a controlled drug release.Citation2–Citation4 In addition, controlled drug delivery improves bioavailability by preventing premature degradation and enhancing uptake, as well as by maintaining drug concentration and release rate within the therapeutic window, while reducing side effects by targeting cancer cells.Citation5–Citation7 Commonly, there are three levels of targeting strategies using polymeric templates, including passive, active, and stimuli-responsive targeting. Passive targeting, also known as enhanced permeability and retention (EPR) effect, takes advantage of the leaky vascular solid tumor structures with poor drainage. This allows particles of a certain size to enter and accumulate in the tumor tissues.Citation7,Citation8 Once the drug is unloaded, the biocompatible polymers would clear through the excretory system. Despite the ability of the EPR effect to improve the accumulation of macromolecules, there are a few barriers to the EPR effect including high interstitial fluid pressure in tumor tissues,Citation7 layers of tissue penetration that is demanded for therapeutic macromolecules, and liver and spleen accumulation of these particles. As a result, additional targeting strategies are required to enhance the distribution of the therapeutic macromolecules. Active targeting mechanisms utilize tumor-specific receptor ligands to achieve a degree of specificity, and therefore it is used as a promising complementary strategy to EPR effect. Targeting molecules include carbohydrates, antibodies, and ligands where their receptors are overly expressed in tumor tissues but are limited in healthy cells. Active targeting would not only improve therapeutic efficiency but also enable reduction of the amount of drug that must be administered to achieve a therapeutic response, thus minimizing negative side effects.Citation2,Citation9–Citation11 Finally, stimuli-responsive delivery systems are designed in response to external stimuli (ie, pH, light, and magnetic field), so that the drug release is triggered only at the desired time and location. When exposed under external stimuli, the polymers will undergo physiochemical structural changes, and therefore lose the well-defined nanoarchitectures releasing drugs directly onto the tumor cells. Among the external stimuli, pH gradients have been widely used for controlled release, relying on the abnormally low pH of endosomes or tumor tissues compared with healthy tissues.Citation12–Citation15
The present study describes a strategy to develop a “smart” drug carrier with all three delivery strategies. In particular, alternating copolymer poly(styrene-alt-maleic anhydride) (SMA) was chosen as the polymeric template (). Amphiphilic alternating copolymers can be structurally very close to biological systems since the alternating pattern of hydrophilic and hydrophobic groups provides uniform interaction along the polymer chains within a nanoarch formed by self-assembly. Indeed, SMA can self-assemble into very ordered nanoarchitectures with cavities as small as 3 nm, resulting in increased volume-to-surface ratio that would further increase the delivering efficiency owing to the EPR effect (). The self-assembly process, only observed at neutral pH, is linked to the linearity of the polymer chain, reducing the entropic cost of chain interaction. The obtained nanostructure is either nanotubes or nanosheets depending on the molecular weight of the polymer, as described in the previous studies.Citation5,Citation6 The linearity observed only at neutral pH originates from a strong hydrogen bond formed within the hydrophilic groups of SMA when one acid group is hydrolyzed. The self-assembled structures characterized by transmission electron microscopy are shown as a function of molecular weight in . To implement the active delivery, folic acid, a commonly used targeting molecule was linked to SMA polymers on its hydrophilic exterior via a biological linker to actively bind to tumor sites. The biological linker was selected using a careful and complete characterization of the functionalized polymer using molecular modeling (such as density functional theory).Citation16 This study ensured that the functionalization of the polymer with the biological linker and folic acid would not affect the linearity of the polymer at pH 7 (). This property is crucial for an unaltered delicate self-assembly process, while preserving the pH sensitivity of the polymer.Citation16
Figure 1 (A) Chemical structure of SMA; (B) SMA self-assembly at pH 7 in aqueous solution.
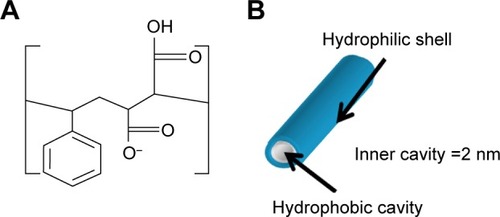
Figure 2 Molecular modeling and transmission electron microscopy characterization of the self-assembled structure of SMA at (A) low molecular weight and (B) high molecular weight. Adapted with permission of Taylor & Francis, from Molecular Simulation, Characterization of a novel self-association of an alternating copolymer into nanotubes in solution, Malardier-Jugroot C, van de Ven TGM, Whitehead MA, 31, 2–1, 2005; permission conveyed through Copyright Clearance Center, Inc.,Citation45 and adapted from Chemical Physics Letters, 636, McTaggart M, Malardier-Jugroot C, Jugroot M, Self-assembled biomimetic nanoreactors I: polymeric template, 206–220, Copyright (2015), with permission from Elsevier.Citation46
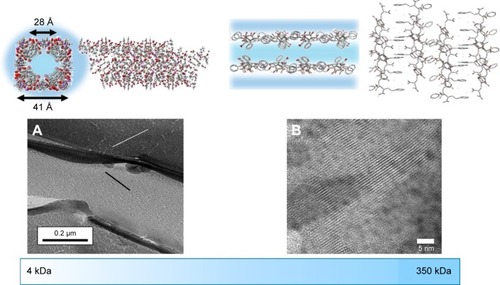
Figure 3 FA-DABA-PSMA oligomer at pH 7 at #1 carboxylic acid using ONIOM model: central trimer optimized to DFT, outer two to PM6. Adapted with permission from Biophy Chem, 214–215, Li X, McTaggart M, Malardier-Jugroot C, Synthesis and characterization of a pH responsive folic acid functionalized polymeric drug delivery system, 17–26. Copyright (2016), with permission from Elsevier.Citation16
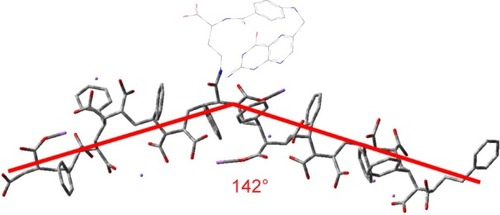
The active targeting using FA originates from folic acid receptors overly expressed in various cancer cells including malignancies of the pancreas, ovary, brain, kidney, breast, and lung.Citation15,Citation16 The binding efficiency between folate and its receptors is very high (KD ~10 m), and the folate conjugates will go through cellular uptake via receptor-mediated endocytosis.Citation15–Citation21 The linker was added to increase the flexibility between targeting agents and drug carrying unit so that the steric hindrance effect would be reduced and the reactivity of the folic acid-modified SMA drug carrier would be increased. Lastly, owing to the pH-responsive nature of SMA, drugs will be released once the carriers reach the tumor sites with lowered pH environment, causing the system to switch from the “off” state to the “on” state ().
Figure 4 Schematic representation of FA-DABA-SMA in its “off” state and “on” state.
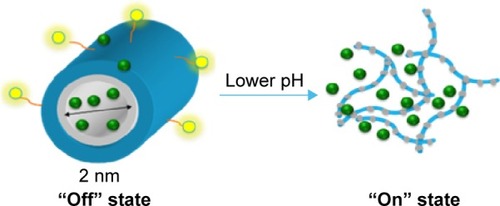
This study group has previously reported the synthesis and complete experimental and theoretical characterization of the above-mentioned delivery vehicle FA-DABA-SMA.Citation16 At neutral pH, the functionalized polymers form stable nanoparticles with a hydrodynamic radius of 144 nm and collapse at lower or higher pH, confirming FA-DABA-SMA as a good candidate for a pH-responsive targeted drug delivery vehicle (). The linker 2,4-diaminobutyric acid (DABA) was selected to improve the accessibility of the ligand. The biodegradable property of DABA and the fact that the linkage between folic acid and SMA through DABA conserved the pH responsiveness of FA-DABA-SMA confirmed its suitability as a linker. The results from cell studies are reported after incubating FA-DABA-SMA to two cell lines such as RAW-Blue macrophage cell lines and human pancreatic PANC-1 cancer cells. Curcumin was selected as a hydrophobic drug mimic and a fluorescent marker to be introduced to the hydrophobic core of FA-DABA-SMA. Curcumin is the extract of the natural ingredient of Curcuma longa (turmeric).Citation23 Traditionally, it has been used in the practice for the treatment of common cold, skin diseases, wound healing, and inflammation.Citation23,Citation24 Recent research has confirmed that curcumin possesses antioxidant, anti-inflammatory, antibacterial, and antiamyloid properties and suppresses proliferation of a wide variety of tumor cells such as pancreatic cancer, multiple myeloma, and colorectal cancer.Citation25–Citation29 In addition, curcumin could act as a fluorescent probe for tracing drug uptake in cell studies since the compound exhibits fluorescence maxima at around 540 nm after excitation at 420 nm.Citation25,Citation30–Citation33 However, its limited solubility and bioavailability prevent its usage. Presently, many research groups work to enhance the solubility and pharmacokinetic property of native curcumin by encapsulation through either a mechanical or a chemical process. The mechanical process utilizes the solution-enhanced dispersion technique by supercritical CO2 to produce curcumin nanoparticles.Citation34,Citation35 Although the method is able to manipulate the properties of the obtained nanoparticles by controlling the parameters of the synthesis process including the flow rate of curcumin solution, precipitation pressure, concentration of curcumin solution, and precipitation temperature, the experimental setups are complicated, and the products are not always in aqueous forms. Alternatively, chemical approaches employ polymeric nanocarrier systems such as liposomes, chitosan nanoparticles, micelles, and so on to solubilize curcumin in the core of the nanostructures.Citation26,Citation28,Citation36–Citation40 Although the encapsulation processes are usually simple, organic solvents are often used, which could introduce undesired toxicity. This study used FA-DABA-SMA as the synthesized drug carrier to deliver curcumin. The encapsulation process is chemical free as curcumin powders diffuse into the hydrophobic core over time. The delivery and release process will be noninvasive as the curcumin drug carrier will be circulated in the bloodstream, and the release is triggered by pH change in the microenvironment of the cancer cells.
Figure 5 Dynamic light scattering results of (A) pure 0.05 wt% SMA solution and (B) 1 wt% PSMA-DABA-FA with mean zeta potential of −39.89 mV.
Abbreviations: DABA, biodegradable linker 2,4-diaminobutyric acid; FA, folic acid; SMA, poly(styrene-alt-maleic anhydride).
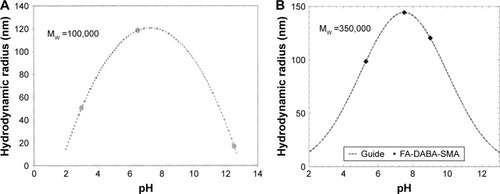
In general, drugs can be incorporated into the delivery vehicles through covalent and noncovalent interactions. In the former, anticancer drugs are linked to the polymer through covalent bonds forming “prodrug”, which will be converted into their active forms upon delivery. However, the process of getting the polymer–drug conjugation approval is slow, and many polymer–drug conjugates show unexpected release behavior and side effects in clinical testing.Citation8 Noncovalent interactions, on the other hand, utilize hydrogen bonding, π−π stacking, and ionic interactions to encapsulate drugs in the interior.Citation41,Citation42 In this study, the hydrophobic interior of SMA template cavity will encapsulate hydrophobic curcumin through diffusion and release the drug directly without prodrug conversion upon reaching the tumor site.
The uptake of curcumin in pancreatic PANC-1 cancer and RAW-Blue macrophage cell lines was studied using fluorescent microscopy. The cell viability using FA-DABA-SMA and curcumin-encapsulated FA-DABA-SMA was examined using the cell proliferation WST-1 assay.Citation44 The results from this study show the potential of using FA-DABA-SMA as a targeted hydrophobic drug delivery vehicle.
Materials and methods
Cell lines
The PANC-1 (American Type Culture Collection, Manassas, VA, USAATCC®; CRL-1469™) cells are a human pancreatic ductal epithelial carcinoma cell line. RAW-Blue™ (InvivoGen, San Diego, CA, USA) cells are derived from the murine RAW 264.7 macrophages. Both cell lines overly express folic acid receptors on the cell surfaces. Cells were grown in media containing Dulbecco’s Modified Eagle’s Medium (1×; Gibco, Rockville, MD, USA) supplemented in 10% fetal calf serum (HyClone Laboratories, Inc., Logan, UT, USA) and 5 μM/mL Plasmocin (InvivoGen) in a 5% CO2 incubator at 37°C.
When the cells reached 80% confluency, they were incubated with SMA or FA-DABA-SMA, each loaded with curcumin at four different concentrations of 0.3, 1, 3, and 10 μm. The cells were incubated for 12 hours before analysis by fluorescent microscopy.
The FA-DABA-SMA particles were synthesized following previous published protocols.Citation16
Curcumin encapsulation
Curcumin was loaded to SMA and FA-DABA-SMA polymers through physical entrapment. Briefly, excess curcumin was added to polymer stock solutions at 54 μm concentration. The solution was allowed to settle and was centrifuged at 4,000 rpm (2500× g) for 7 minutes to remove the remaining curcumin from the solution. Curcumin-loaded polymers were then diluted to four different concentrations of 0.3, 1, 3, and 10 μm. The curcumin-loaded SMA was characterized using Tecan M1000 Pro plate reader (Tecan Group Ltd, Männedorf, Switzerland) in 96-well microplates.
Fluorescent microscopy
The cellular uptake of curcumin was visualized using a fluorescent microscope. Briefly, 5×104 RAW-Blue or PANC-1 cells were seeded in 24-well plates on 12 mm circular glass cover slides in Dulbecco’s Modified Eagle’s Medium containing 10% fetal bovine serum at 37°C in 5% CO2 overnight to allow cells to attach to slides. Cells were treated with SMA, FA-DABA-SMA, or untreated as a control for overnight at 37°C. Subsequently, the cell culture media was removed, and the cells on circular glass slides were placed on 4 μL of fluorescence mounting medium (DAKO, Agilent Technologies, Santa Barbara, CA, USA). The fluorescent images were taken using a Zeiss M2 Imager epifluorescent microscopy (Carl Zeiss AG, Oberkochen, Germany) (40× objective, at 200× and 400× magnification).
WST-1 assay
The WST-1 assay was used as a measure of cell viability based on the reduction of a tetrazolium compound to a soluble derivative.Citation43,Citation44 The absorbance recorded at 420 nm is directly proportional to the number of living cells in culture. At 80%–90% confluence, cells were added to 96-well microplates at a density of 5,000 cells per well and incubated at 37°C overnight. They were then exposed to increasing concentrations of indicated polymer treatments or left untreated as controls for 24, 48, and 72 hours. About 100 μL of WST-1 reagent (Roche Diagnostics Division de Hoffman La Roche Limitée, Laval-des-Rapides, QC, Canada), diluted 1:10 in culture medium, was added to the wells for 2 hours prior to reading of absorbance at 420 nm at each time point. Cell viability was presented as a percentage of control using GraphPad Prism software (GraphPad Software, Inc., La Jolla, CA, USA). The following formula was used to determine cell viability as a percent of control for each time point and treatment:
Results and discussion
To confirm the increased solubility of encapsulated curcumin in SMA aqueous solution as compared with its solubility in water, fluorescent spectroscopy was performed on aqueous solutions of curcumin and SMA-curcumin. First, shows that both spectra exhibit emission maxima at around 540 nm with excitation wavelength at 420 nm, confirming the fluorescent properties of curcumin as a probe in the cells. Second, the emission spectrum of curcumin-loaded SMA shows a rapid increase in maxima intensity, indicating the enhanced solubility of curcumin in SMA and confirming that the polymers could act as delivery templates for curcumin.
Figure 6 Chemical structure of curcumin and fluorescence intensity of curcumin in water and when encapsulated in SMA polymers (PSMA) (1% wt to water) at excitation wavelength 420 nm.
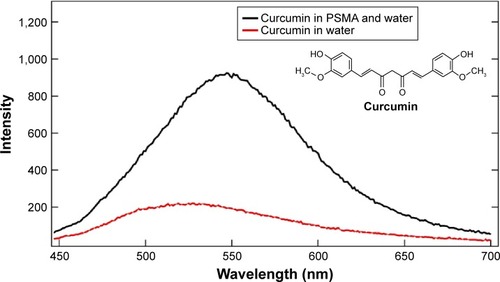
The cellular uptake of curcumin-loaded SMA and FA-DABA-SMA was visualized by the intrinsic fluorescence of curcumin using fluorescence microscopy. shows the fluorescent images of curcumim-loaded SMA in RAW-Blue macrophage cells () and PANC-1 pancreatic cancer cells (). It appears that curcumin is taken up by both cell lines displayed by fluorescence emitted from the accumulation of curcumin in the cytoplasm. In addition, the dark unstained nucleus shown in indicates that curcumin-loaded SMA did not interact with the nucleus.
Figure 7 Fluorescent images showing cellular uptake of curcumin-loaded SMA in (A) RAW-Blue and (B) PANC-1 cell lines.
Abbreviations: SMA, poly(styrene-alt-maleic anhydride); Cur, curcumin.
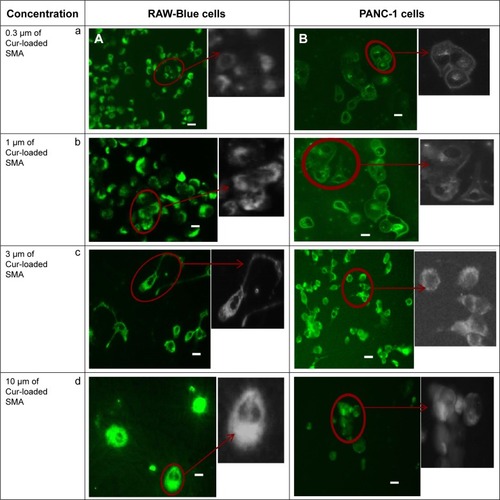
shows the fluorescent images of curcumin-loaded FA-DABA-SMA in RAW-Blue macrophage cells () and PANC1 pancreatic cancer cells (). It is observed for all four concentrations that the modified FA-DABA-SMA polymers are taken up by cells differently than pure SMA. At almost all concentrations, the polymer chain exhibits association around the cells into cylindrical filaments. This association is specific to cells as no association is observed when the polymer is not in contact with the cells. The enhanced fluorescent contrast at the filaments due to the curcumin inside the polymers confirms that the aggregation is formed by the FA-DABA-SMA polymers. Furthermore, the curcumin was taken up by both cell lines and was found to cross and agglomerate inside the nucleus and cytoplasm. Lastly, the morphological changes observed in the cells indicate that the cells are slowly losing their shapes, indicating the initiation of cell death. The exact mechanisms behind the polymer aggregation around cells are still under investigation; however, the enhanced fluorescent contrast in the filaments and the specificity toward cells exhibit great potential for enhanced delivery efficiency.
Figure 8 Fluorescent images showing cellular uptake of curcumin-loaded FA-DABA-SMA in (A) RAW-Blue and (B) PANC-1 cell lines.
Abbreviations: SMA, poly(styrene-alt-maleic anhydride); Cur, curcumin.
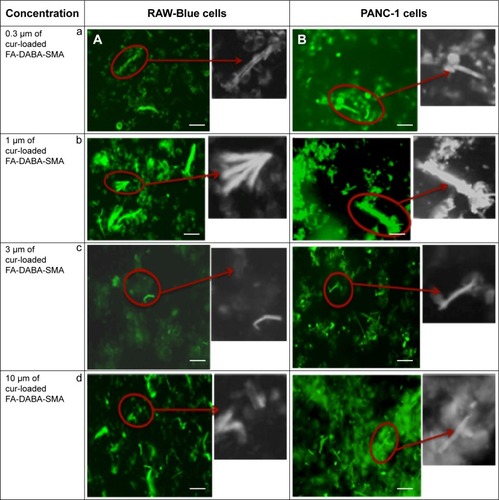
To exclude the possibility of toxicity from the polymeric template, we utilized empty nanoparticles (SMA and functionalized FA-DABA-SMA) treated PANC-1 cancer cell line using WST-1 reagents (). Four concentrations of SMA (0.3, 1, 3, and 10 μm) were tested, and the readings were taken at 24, 48, and 72 hours. The results shown in indicate that the SMA polymers do not exhibit strong toxicity on the cancer cells. However, the highest concentration of 10 μm results in cell death at 48 and 72 hours, indicating that the toxicity of SMA is dosage dependent. As a result, the 10 μm concentration of SMA was excluded from further testing. Empty FA-DABA-SMA was also tested against PANC-1 at 0.3, 1, and 3 μm concentrations. As shown in , the empty functionalized FA-DABA-SMA delivery vehicle did not interfere with cell growth, and is therefore deemed nontoxic to the cells.
Figure 9 Viability of PANC-1 cells treated with empty SMA at different doses using the WST-1 assay.
Abbreviations: SMA, poly(styrene-alt-maleic anhydride); SEM, standard error of mean.
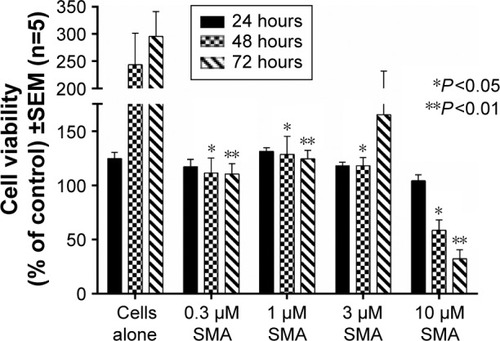
Figure 10 Viability of PANC-1 cells treated with empty FA-DABA-SMA at different doses using the WST-1 assay.
Abbreviations: SMA, poly(styrene-alt-maleic anhydride); SEM, standard error of mean.
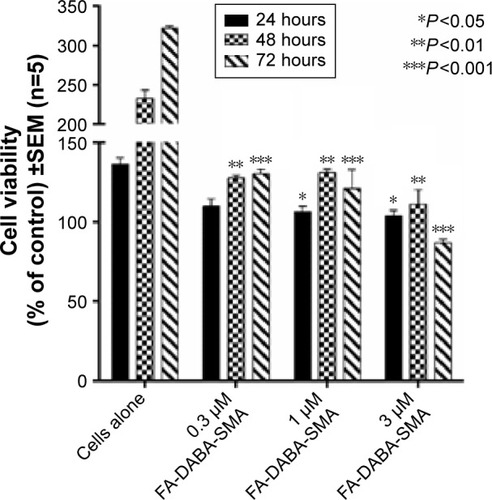
The effect of FA-DABA-SMA loaded with curcumin on PANC-1 cell viability was also investigated using the WST-1 assay at 0.3, 1, and 3 μm concentrations (). The results show that although the lower concentrations of curcumin (Cur)-loaded FA-DABA-SMA did not cause cell death, the 3 μm Cur/FA-DABA-SMA demonstrate significant toxicity and cell death. The toxicity at 3 μm after 72 hours of treatment, compared with empty SMA and FA-DABA-SMA, confirms the on-site release of curcumin by the FA-DABA-SMA delivery platform (). The folic acid functionalized SMA polymers present enhanced delivery effects on tumor cells, which are consistent with the fluorescent microscopy results shown in . Therefore, folic acid-conjugated amphiphilic alternating copolymer is a novel delivery platform for the delivery of hydrophobic chemotherapeutic agents against cancer cells.
Figure 11 Viability of PANC-1 cells treated with Cur-encapsulated FA-DABA-SMA at different doses using the WST-1 assay.
Abbreviations: SMA, poly(styrene-alt-maleic anhydride); Cur, curcumin; SEM, standard error of mean.
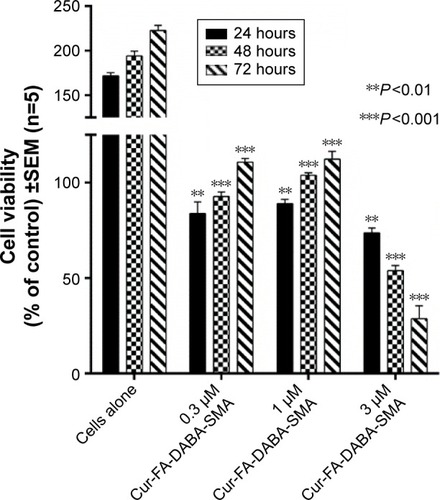
Conclusion
In conclusion, this paper presents a very unique interaction of cell lines containing overly expressed folic acid receptors in contact with a newly developed targeted drug delivery vehicle. Cell viability assay showed that empty SMA and FA-DABA-SMA nanoparticles did not cause cell death, confirming the biocompatible properties of the delivery vehicles. The enhanced fluorescence microscopy showed the cellular uptake of FA-DABA-SMA loaded with curcumin by both PANC-1 and RAW-Blue cell lines. The drug carrier FA-DABA-SMA is shown to release the drug efficiently inside the nucleus and cytoplasm, initiating cell death. This study revealed that the novel interactions between the modified FA-DABA-SMA polymers with the cells could lead to enhanced hydrophobic drug delivery efficiency and could possibly be developed as a probe for cancer therapeutics.
With three levels of control including EPR effect, folic acid active targeting, and pH responsiveness in the cancer cell microenvironment, FA-DABA-SMA polymers show promise as a new active tumor targeting drug delivery system.
More studies are being undertaken to investigate the encapsulation and release profiles of curcumin encapsulated in SMA and FA-DABA-SMA. In addition, clinical conventional approved anticancer drugs will be loaded and tested in these polymers to study the solubility, cellular uptake, and cytotoxicity.
Acknowledgments
This work was supported in part by grants to C Malardier-Jugroot and M R Szewczuk from the Natural Sciences and Engineering Research Council of Canada. Xia Li is the recipient of the Alexander Graham Bell Canada Graduate Scholarship from Natural Sciences and Engineering Research Council of Canada.
Disclosure
The authors report no conflicts of interest in this work.
References
- ChidambaramMManavalanRKathiresanKNanotherapeutics to overcome conventional cancer chemotherapy limitationsJ Pharm Pharm Sci2011141677721501554
- BertrandNWuJXuXKamalyNFarokhzadOCCancer nanotechnology: the impact of passive and active targeting in the era of modern cancer biologyAdv Drug Deliv Rev20146622524270007
- Brannon-PeppasLBlanchetteJONanoparticle and targeted systems for cancer therapyAdv Drug Deliv Rev201264206212
- BaeYHParkKTargeted drug delivery to tumors: myths, reality and possibilityJ Controlled Release20111533198205
- Malardier-JugrootCvan de VenTGCosgroveTRichardsonRMWhiteheadMANovel self-assembly of amphiphilic copolymers into nanotubes: characterization by small-angle neutron scatteringLangmuir20052122101791018716229543
- Malardier-JugrootCvan de VenTGWhiteheadMALinear conformation of poly(styrene-alt-maleic anhydride) capable of self-assembly: a result of chain stiffening by internal hydrogen bondsJ Phys Chem B2005109157022703216851798
- MaedaHNakamuraHFangJThe EPR effect for macromolecular drug delivery to solid tumors: improvement of tumor uptake, lowering of systemic toxicity, and distinct tumor imaging in vivoAdv Drug Deliv Rev2013651717923088862
- ZhangYChanHFLeongKWAdvanced materials and processing for drug delivery: the past and the futureAdv Drug Deliv Rev201365110412023088863
- AllenTMCullisPRDrug delivery systems: entering the mainstreamScience200430356651818182215031496
- MaedaHSMANCS and polymer-conjugated macromolecular drugs: advantages in cancer chemotherapyAdv Drug Deliv Rev199162181202
- BrownSDNativoPSmithJAGold nanoparticles for the improved anticancer drug delivery of the active component of oxaliplatinJ Am Chem Soc2010132134678468420225865
- RejinoldNSThomasRGMuthiahMRadio frequency triggered curcumin delivery from thermo and pH responsive nanoparticles containing gold nanoparticles and its in vivo localization studies in an orthotopic breast tumor modelRSC Adv201447439408
- GaoWChanJMFarokhzadOCpH-responsive nanoparticles for drug deliveryMol Pharm2010761913192020836539
- HenrySMEl-SayedMEPirieCMHoffmanASStaytonPSpH-responsive poly(styrene-alt-maleic anhydride) alkylamide copolymers for intracellular drug deliveryBiomacromolecules2006782407241416903689
- LaleSVAswathyRGAravindAKumarDSKoulVAS1411 aptamer and folic acid functionalized pH-responsive ATRP fabricated pPEGMA–PCL–pPEGMA polymeric nanoparticles for targeted drug delivery in cancer therapyBiomacromolecules20141551737175224689987
- LiXMcTaggartMMalardier-JugrootCSynthesis and characterization of a pH responsive folic acid functionalized polymeric drug delivery systemBiophy Chem2016214–2151726
- MatiniTFranciniNBattocchioASynthesis and characterization of variable conformation pH responsive block co-polymers for nucleic acid delivery and targeted cell entryPolym Chem2014516261636
- LeeRJLowPSDelivery of liposomes into cultured KB cells via folate receptor-mediated endocytosisJ Biol Chem19942695319832048106354
- KimSLJeongHJKimEMLeeCMKwonTHSohnMHFolate receptor targeted imaging using poly(ethylene glycol)-folate: in vitro and in vivo studiesJ Korean Med Sci200722340541117596645
- SudimackJLeeRJTargeted drug delivery via the folate receptorAdv Drug Deliv Rev200041214716210699311
- AntonyACThe biological chemistry of folate receptorsBlood19927911280728201586732
- BhattacharyaDDasMMishraDFolate receptor targeted, carboxymethyl chitosan functionalized iron oxide nanoparticles: a novel ultradispersed nanoconjugates for bimodal imagingNanoscale201134165321331392
- AggarwalBBSurhYJShishodiaSThe Molecular Targets and Therapeutic Uses of Curcumin in Health and DiseaseNew York, NYSpringer2007
- López-LázaroMAnticancer and carcinogenic properties of curcumin: considerations for its clinical development as a cancer chemopreventive and chemotherapeutic agentMol Nutr Food Res200852Suppl 1S103S12718496811
- KunwarABarikAMishraBRathinasamyKPandeyRPriyadarsiniKIQuantitative cellular uptake, localization and cytotoxicity of curcumin in normal and tumor cellsBiochim Biophys Acta BBA – Gen Subj200817804673679
- ChangPYPengSFLeeCYCurcumin-loaded nanoparticles induce apoptotic cell death through regulation of the function of MDR1 and reactive oxygen species in cisplatin-resistant CAR human oral cancer cellsInt J Oncol20134341141115023917396
- ShehzadALeeJHuhTLLeeYSCurcumin induces apoptosis in human colorectal carcinoma (HCT-15) cells by regulating expression of Prp4 and p53Mol Cells201335652653223686430
- DasMSahooSKFolate decorated dual drug loaded nanoparticle: role of curcumin in enhancing therapeutic potential of nutlin-3a by reversing multidrug resistancePLoS One201273e3292022470431
- BishtSFeldmannGSoniSPolymeric nanoparticle-encapsulated curcumin (“nanocurcumin”): a novel strategy for human cancer therapyJ Nanobiotechnology200751317439648
- AdhikaryRCarlsonPJKeeTWPetrichJWExcited-state intramolecular hydrogen atom transfer of curcumin in surfactant micellesJ Phys Chem B201011482997300420136104
- PuvvadaNRajputSKumarBNMandalMPathakAExploring the fluorescence switching phenomenon of curcumin encapsulated niosomes: in vitro real time monitoring of curcumin release to cancer cellsRSC Adv2013382553
- ChignellCFBilskjPReszkaKJMottenAGSikRHDahlTASpectral and photochemical properties of curcuminPhotochem Photobiol19945932953028016208
- PatraDBarakatCSynchronous fluorescence spectroscopic study of solvatochromic curcumin dyeSpectrochim Acta A Mol Biomol Spectrosc20117951034104121612978
- ZhaoZXieMLiYFormation of curcumin nanoparticles via solution-enhanced dispersion by supercritical CO2Int J Nanomedicine2015103171318125995627
- XieMFanDChenYAn implantable and controlled drug-release silk fibroin nanofibrous matrix to advance the treatment of solid tumour cancersBiomaterials2016103334327376557
- HuangQZhangLSunXZengKLiJLiuYNCoating of carboxymethyl dextran on liposomal curcumin to improve the anticancer activityRSC Adv201441035921159217
- TaurinSNehoffHDiongJLarsenLRosengrenRJGreishKCurcumin-derivative nanomicelles for the treatment of triple negative breast cancerJ Drug Target201321767568323679865
- Sanoj RejinoldNMuthunarayananMDivyaraniVVCurcumin-loaded biocompatible thermoresponsive polymeric nanoparticles for cancer drug deliveryJ Colloid Interface Sci20113601395121549390
- GouMMenKShiHCurcumin-loaded biodegradable polymeric micelles for colon cancer therapy in vitro and in vivoNanoscale201134155821283869
- KoleyPPramanikAMultilayer vesicles, tubes, various porous structures and organo gels through the solvent-assisted self-assembly of two modified tripeptides and their different applicationsSoft Matter20128195364
- DoaneTBurdaCNanoparticle mediated non-covalent drug deliveryAdv Drug Deliv Rev201365560762122664231
- YangCAttiaABTanJPThe role of non-covalent interactions in anticancer drug loading and kinetic stability of polymeric micellesBiomaterials201233102971297922244697
- NgamwongsatitPBanadaPPPanbangredWBhuniaAKWST-1-based cell cytotoxicity assay as a substitute for MTT-based assay for rapid detection of toxigenic Bacillus species using CHO cell lineJ Microbiol Methods200873321121518417231
- TanASBerridgeMVSuperoxide produced by activated neutrophils efficiently reduces the tetrazolium salt, WST-1 to produce a soluble formazan: a simple colorimetric assay for measuring respiratory burst activation and for screening anti-inflammatory agentsJ Immunol Methods20002381–2596810758236
- Malardier-JugrootCvan de VenTGMWhiteheadMACharacterization of a novel self-association of an alternating copolymer into nanotubes in solutionMol Simul2005312–1173178
- McTaggartMMalardier-JugrootCJugrootMSelf-assembled biomimetic nanoreactors I: polymeric templateChem Phys Lett2015636216220