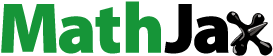
Abstract
Hyperpigmentation caused by melanin overproduction is a major skin disorder in humans. Inhibition of tyrosinase, a key regulator of melanin production, has been used as an effective strategy to treat hyperpigmentation. In this study, we investigated the use of solid lipid nanoparticles (SLNs) as a highly effective and nontoxic means to deliver a newly synthesized potent tyrosinase inhibitor, MHY498, and to target melanocytes through the skin. MHY498-loaded SLNs (MHY-SLNs) were prepared by an oil-in-water emulsion solvent-evaporation method, and their morphological and physicochemical properties were characterized. MHY-SLNs showed a prolonged drug-release profile and higher skin permeation than that of MHY solution. In an in vivo evaluation of antimelanogenic activity, MHY-SLNs showed a prominent inhibitory effect against ultraviolet B-induced melanogenesis, resulting in no change in the skin color of C57BL/6 mouse, compared with that observed in an MHY solution-treated group and an untreated control group. The antimelanogenic effect of MHY-SLNs was further confirmed through Fontana–Masson staining. Importantly, MHY-SLNs did not induce any toxic effects in the L929 cell line. Overall, these data indicate that MHY-SLNs show promise in the topical treatment of hyperpigmentation.
Introduction
Melanin is a determinant of skin color and produced by the melanogenesis pathwayCitation1 in melanocytes located in the basal layer of the epidermis. It plays an important role in protecting skin against ultraviolet (UV)-induced damage;Citation2,Citation3 however, melanin overproduction causes hyperpigmentation including freckles, melisma, and lentigines, which is the third most common dermatological disorder related to serious esthetic problems owing to its visible nature.Citation4–Citation6 Various causative factors, such as UV radiation, alpha-melanocyte-stimulating hormone (α-MSH), cyclic AMP-elevating agents (such as forskolin),Citation7,Citation8 and melanin-synthesizing enzymes (such as tyrosinase, tyrosinase-related protein 1, and dopachrome tautomerase),Citation9 have been studied to regulate melanin production and skin accumulation. Among them, inhibition of tyrosinase has emerged as an effective strategy to treat hyperpigmentation because tyrosinase controls the rate-limiting steps of melanogenesis where tyrosine is converted to dopaquinone, which subsequently produces eumelanin and pheomelanin.Citation10,Citation11
Conventional tyrosinase inhibitors such as kojic acid and hydroquinone have been used as skin-whitening agents in sunscreen and cosmetic formulations.Citation12 Their clinical uses, however, have been limited because of poor in vivo efficacy, poor skin permeation, and/or low formulation stability.Citation13,Citation14 This has led to the development of new tyrosinase inhibitors. Recently, a novel tyrosinase inhibitor – (Z)-5-(2,4-dihydroxy benzylidene)thiazolidine-2,4-dione (MHY498) – was synthesized based on the fact that compounds containing imidazole and 2,4-dihydroxy phenyl moiety inhibit tyrosinase activity more potently than other derivatives.Citation15,Citation16 MHY498 (mean IC50 [the half maximal inhibitory concentration]=3.55 µM) is 6.4-fold more potent than kojic acid (mean IC50=22.79 µM), a positive standard and the most intensively studied tyrosinase inhibitor.Citation15 Moreover, MHY498 was not cytotoxic to B16F10 melanoma cells at concentrations of up to 32 µM, while it inhibited tyrosinase in a dose-dependent manner. Kojic acid was cytotoxic at the same concentration.Citation15 Thus, MHY498 appears to be a promising tyrosinase inhibitor for treating hyperpigmentation.
Despite its potency, MHY498’s delivery through the skin is a major hurdle owing to the stratum corneum, which functions as a primary skin barrier.Citation17 The low delivery efficiency would necessitate frequent administration or the use of permeation enhancers, which compromise patients’ compliance or may cause skin toxicity. Moreover, direct contact of tyrosinase inhibitors with the stratum corneum may cause skin carcinogenesis.Citation18 Thus, a delivery system that ensures efficient penetration through the stratum corneum to melanocytes with safe MHY498 encapsulation (to avoid direct skin contact) is needed.
Solid lipid nanoparticles (SLNs) have long been used as a topical drug-delivery system because they eliminate the traditional disadvantages encountered during drug delivery through the skin.Citation19,Citation20 SLNs have unique features over other nanoparticle systems, which make SLNs a potential useful skin delivery system. Lipids used in SLNs are biocompatible and recognized as safe excipients that are devoid of acute and chronic dermal toxicity.Citation21,Citation22 Furthermore, lipids can have a beneficial interaction with the stratum corneum, which is composed of hexagonal corneocytes embedded in a lipid matrix of ceramide, free fatty acids, cholesterol, and triglycerides.Citation23 When SLNs are applied to the skin, they form an occlusive film that prevents transepidermal water loss and hydrates the stratum corneum.Citation20,Citation24 Thus, corneocyte packing of the stratum corneum becomes loose, which facilitates drug penetration.
To date, a few studies have been reported regarding the formulation of lipid nanoparticles containing tyrosinase inhibitors.Citation25–Citation29 However, most were confined to in vitro characterizations, and no extensive in vivo evaluation of hyperpigmentation treatment has been performed. Our main focus in this study was to deliver MHY498 into the basal layer of the epidermis using SLNs as carriers to effectively inhibit melanogenesis. We encapsulated MHY498 in lipid nanoparticles based on Compritol 888 ATO. Formulated SLNs were characterized using different parameters, and their in vivo melanogenesis inhibition efficacies were evaluated in a C57BL/6 mouse model. The toxicity profile of MHY498-loaded SLNs (MHY-SLNs) was evaluated in L929 skin fibroblast cells.
Materials and methods
Materials
(Z)-5-(2,4-dihydroxy benzylidene)thiazolidine-2,4-dione (MHY498) was synthesized as previously reported.Citation13 Glyceryl behenate (Compritol 888 ATO) was generously gifted by Gattefossé (Saint-Priest, France). Phospholipon 90 G (phosphatidylcholine 90%) was a gift from Lipoid GmbH (Ludwigshafen, Germany). Poloxamer 188 (Kolliphor® P 188), phosphotungstic acid, tetrazolium dye 3-(4,5-dimethylthiazol-2-yl)-2,5-diphenyltetrazolium bromide (MTT), and dimethyl sulfoxide (DMSO) were purchased from Sigma-Aldrich (St Louis, MO, USA). Roswell Park Memorial Institute (RPMI) 1640 medium, trypsin, fetal bovine serum, and penicillin–streptomycin were purchased from Hyclone (Logan, UT, USA). The Fontana–Masson Staining Kit was purchased from ScyTek (Logan, UT, USA). All other reagents and solvents were of the highest analytical grade commercially available.
Preparation of MHY-SLNs
MHY-SLNs were prepared by a modified oil-in-water emulsion solvent-evaporation method.Citation30 Briefly, 10 mg MHY498, 150-mg Compritol 888 ATO, and 100 mg Phospholipon 90 G were dissolved in 10 mL of anhydrous ethanol and heated at 80°C, which constituted the organic phase. The organic phase was slowly injected into 0.5% w/v cold poloxamer solution. The mixture was stirred at 1,000 rpm for 4 h in a fume hood, and the solvent was evaporated. The resultant SLNs were harvested by centrifugation at 15,000 rpm for 1 h. The obtained pellet was reconstituted in water and stored as a suspension. To evaluate crystallinity, MHY-SLNs suspension was freeze-dried at −50°C for 24 h.
Physicochemical characterization of MHY-SLNs by transmission electron microscopy (TEM)
MHY-SLNs morphology was investigated by TEM (H-7600, Hitachi, Japan), using a negative-staining method.Citation31 Briefly, 1 drop of diluted MHY-SLNs suspension was mounted on a 200-mesh formvar copper grid and dried at room temperature. Then, the sample was negatively stained with 1% (w/v) phosphotungstic acid and viewed by TEM.
Determination of particle size and analysis
Particle sizes and zeta potentials of MHY-SLNs were measured using a Malvern Zetasizer Nano ZS system (Malvern Instruments, Worcestershire, UK). Briefly, 5 µL of each SLN sample was diluted in 2 mL Milli-Q water, and particle sizes and zeta potentials were measured at 25°C.
Drug loading and encapsulation efficiency
The amount of MHY498 in SLNs was determined by UV spectrophotometry with some modifications. Briefly, 200 µL MHY-SLNs suspension was freeze-dried and dissolved in anhydrous ethanol. An ethanolic solution containing MHY498 was shaken overnight using a vortexer. Subsequently, the solution was centrifuged at 12,000 rpm for 20 min. The supernatant was collected and analyzed by UV spectrophotometry at 376 nm. All experiments were performed in triplicate. From these measurements, the total amount of MHY498 in SLNs and weight of SLNs were calculated. Drug loading and the encapsulation efficiency (%) were calculated using the following equations:
X-ray diffraction (XRD)
The physical status of MHY498 in SLNs was analyzed by XRD analysis using a Rigaku XRD (MiniFlex, Rigaku, Tokyo, Japan). The X-ray tube was run at 40 kV and 40 mA. Scattering angles were transformed into short spacings using Bragg’s equation (nλ=2dsinθ). All powdered samples were scanned in the 2θ range of 5°C–40°C, and the scanning rate was 2θ/min. Samples included MHY498 alone, bulk Compritol 888 ATO, and MHY-SLNs.
Differential scanning calorimetry (DSC)
DSC was performed using a DSC-8000 (Perkin-Elmer, Waltham, MA, USA). A heating rate of 10°C/min was applied in 20°C–220°C temperature range. Analysis was performed under a nitrogen purge of 20 mL/min. Approximately, 10 mg of sample was used for analysis. Samples included MHY498 alone, bulk Compritol 888 ATO, and MHY-SLNs. An empty aluminum sample pan was used as a reference.
In vitro release study
MHY498 in vitro release from SLNs was determined by the dialysis bag-diffusion technique.Citation32 Dialysis bags with a molecular weight cutoff of 12,000–14,000 (Visking® dialysis tubing, Servia, Greece) were used. Release medium contained 150 mL phosphate-buffered saline (PBS, pH 7.4) with 1% Tween 80. Dialysis membranes were soaked overnight and filled with 3 mL of MHY-SLNs suspension. Dialysis membranes were sealed at both ends and placed into the release medium. Experiments were performed at 37°C, with a stirring speed of 50 rpm. Samples were withdrawn at 0.5, 1, 2, 3, 4, 6, 8, 12, 24, and 48 h. At each sampling point, 1 mL of sample was withdrawn and replaced with fresh medium. The withdrawn sample was analyzed by UV spectrophotometry. The release from each formulation was measured in triplicate.
In vitro skin-permeation study
In vitro skin-permeation studies were performed using a 3-station Franz diffusion cell (PermeGear, Inc., Hellertown, PA, USA). Full-thickness dorsal skin from C57BL/6 mice (male, 7 weeks, 25 g) was used. Animal study protocols were approved by the Animal Care Committee of Pusan National University and were performed, following the guidelines for animal experiments issued by Pusan National University. Briefly, each mouse was euthanized using CO2 gas. Subsequently, hair from the dorsal portion was carefully removed using an electric trimmer. Application of Veet® Hair Removal Cream (Reckit Benckiser, Inc., Slough, UK) for 5 min facilitated complete hair removal. The skin was collected, and subcutaneous fatty tissues were removed using blunt tweezers with simultaneous washing with PBS. The Franz diffusion cell was connected with a circulatory water jacket to maintain the temperature at 37°C. The receptor compartment was filled with 7 mL PBS (pH 7.4) and 1 mL ethanol. Ethanol was used as a solubilizer to ensure a sufficient sink condition. Mouse skin was mounted on the cell with the stratum corneum side facing outside. The diffusion area of the skin was 0.95 cm2. The donor compartment was filled with a 300 µg equivalent of MHY-SLNs suspension and covered with paraffin to prevent evaporation. The receptor solution was stirred at constant rpm at predetermined time points (0.5, 1, 2, 3, 4, 6, 12, 24, and 48 h). Next, 2 mL of sample was withdrawn and replaced with the same volume of fresh solution. All samples were filtered through an aqueous 0.22 µm pore size cellulose membrane filter and analyzed by UV spectrophotometry. A similar procedure was performed for MHY solution containing 300 µg of MHY498 at a 2:1 ratio of ethanol:propylene glycol.
Skin-permeation parameters
The cumulative amount (QMHY) of MHY498 that permeated through mouse skin was plotted against time and determined using the following equation:
Here, Vr and Vd are the volume of the receptor and sample taken at each sampling time, respectively. Ct indicates the MHY498 concentration of the receptor compartment at each sampling time. Ci is the drug concentration of the ith sample. A is the effective skin surface area (0.95 cm2). MHY498 flux through the skin, J (µg/cm2⋅h), was calculated from the slope of a linear portion of a graph showing the cumulative amount of MHY498 permeating through mouse skin per unit surface area versus time.
In vivo evaluation of MHY-SLNs
To evaluate the depigmentation activity of MHY-SLNs, C57BL/6 mice were used as a model in animal experiments. Five-week-old male C57BL/6 mice were purchased from Samtako Bio (Osan, South Korea). Mice were housed under standard conditions with a 12 h/12 h light/dark cycle and fed standard food and drinking water. The animals were acclimatized for 7 days before initiating experiments. The dorsal side of mouse skin was used for the experiments. Mouse hair was removed using an electric trimmer and Veet Hair Removal Cream.
Mice were randomly divided into 4 groups of 6 animals per group: UV control, MHY solution, blank-SLNs, and MHY-SLNs. Each group was irradiated with UVB radiation at an intensity of 450 mJ/cm2 using a crosslinker (BEX-800, Ultra-Lum, Claremont, CA, USA) to induce melanogenesis in the mouse dorsal skin. Each group was exposed to UVB radiation for 2 weeks on alternate days. A 2×2 cm2 area was selected, and a 200 µg/mL equivalent of MHY-SLNs suspension or MHY solution alone was applied to the mouse skin for 14 days in the MHY-SLNs and MHY solution groups, respectively. The UV control group was untreated.
During treatment, skin brightness was measured using a CR-10 spectrophotometer (Konica Minolta Sensing, Sakai, Osaka, Japan), which describes colors using L*, a*, and b* values as described by the Commission Internationale de l’Eclairage color system.Citation33 Mice were sacrificed after 14 days, and designated skin portions were collected for histological evaluation.
Histological evaluation
Skin biopsy specimens were fixed in 10% formaldehyde solution for 24 h and embedded in a paraffin block according to standard procedures. Sections (5 µm thick) were fixed to glass slides and stained with Fontana–Masson stain to observe melanocytes and melanin. Slides were examined by light microscopy, and images were digitally captured without further processing.
In vitro cytotoxicity study using L929 skin cytotoxicity cells
The mouse fibroblast cell line L929 obtained from the Korean Cell Line Bank (KCLB, Seoul, South Korea) were cultured in RPMI 1640 medium supplemented with 10% (v/v) fetal bovine serum, 100 IU/mL penicillin G sodium, and 100 µg/mL streptomycin sulfate. The cells were grown at 37°C in an incubator supplied with 5% CO2 and a humidified air atmosphere. Cells were seeded in 96-well plates at 5×104 cells per well and incubated for 24 h. The media were then replaced with fresh media containing MHY-SLNs suspension and MHY solution containing different MHY498 concentrations (50, 100, 200, and 400 µg/mL) and incubated for 24 h. A standard MTT solution in sterile PBS was added to each well and incubated for 2 h. Media containing test substances and MTT solution were then removed, and 150 µL of DMSO was added to each well. The absorbance measured at 540 nm was proportional to the concentration of viable cells in each well. Untreated cells were used as a control. The data were expressed as the mean ± standard deviation (SD) of 4 replication (n=4). Cell viability was calculated using the following equation:
Statistical analysis
The results are expressed as the mean values (±SD) of 3–6 experiments. All in vivo data analysis was performed using an unpaired Student’s t-test in GraphPad Prism 5.0 (GraphPad Software, Inc., La Jolla, CA, USA). P-values of <0.05 were considered statistically significant.
Results and discussion
MHY-SLNs preparation and characterization
MHY-SLNs were prepared by a modified emulsion solvent-evaporation method.Citation28 Organic MHY498, Compritol 888 ATO, and Phospholipon 90 G were used as the organic phase, and poloxamer 188 was used as the aqueous phase. When hot organic phase was injected into hot poloxamer 188 solution, the yield of SLNs was very low (~25%). We found that hot poloxamer 188 solubilized MHY498, which might have caused expulsion of MHY498 from the SLN matrix. To avoid MHY498 expulsion, the hot organic phase was injected into a cold poloxamer 188 solution and stirred at 4°C. This modification resulted in a facile formulation of MHY-SLNs with a high yield (88.5%) and sufficient drug (3.4%) loading. The physicochemical characterization of the MHY-SLNs is shown in .
Table 1 Physicochemical characterization of MHY-SLNs
Size is an important parameter for the occlusive properties of SLNs. In general, SLNs particle size <400 nm possess occlusive properties that promote skin penetration.Citation24,Citation32 From a size-distribution histogram (), it can be seen that the MHY-SLNs were fabricated with a particle size of <400 nm. The TEM image also confirmed the particle sizes of MHY-SLNs are shown in . The smaller particle sizes observed by TEM (as compared to dynamic light scattering calorimetry [DLS] measurements) can be explained by the fact that DLS measures the hydrodynamic diameter, whereas TEM measures the particle cores only. Zeta potentials provide information regarding the stability of aqueous colloidal dispersions.Citation34 Particles with high zeta potential values (>|30| mV) have a reduced tendency to aggregate.Citation35 SLNs are negatively charged on the surface.Citation36 MHY-SLNs showed a zeta potential value of −29.8 mV, which confirmed that MHY498 loading into an SLNs matrix did not affect particle stability ().
Figure 1 In vitro characterization of MHY-SLNs.
Abbreviations: MHY-SLNs, MHY498-loaded solid lipid nanoparticles; endo, endothermic.
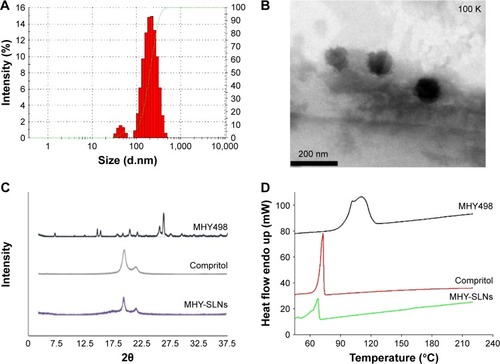
XRD and DSC are widely used techniques for determining the physical status of SLNs matrixes. XRD and DSC data help identify whether a drug is loaded into the particle matrix. XRD data for MHY498, bulk Compritol 888 ATO, and freeze-dried MHY-SLNs are represented in . Each sample has characteristic crystalline domains that can be identified through XRD by scanning over a wide range of incident angles. The XRD pattern of MHY498 showed several narrow peaks with a maximum peak intensity at 2θ=27.5°C, indicating its crystallinity. Compritol only showed 2 sharp peaks with a maximum at 2θ=21.18°C. In the MHY-SLNs diffractogram, 1 major peak was observed at 2θ=21.29°C, which appeared to overlap with the Compritol diffractogram. It was also noted that the MHY498-specific peaks disappeared in the diffractogram for MHY-SLNs, indicating an amorphous or molecularly dispersed state in the SLN matrix.Citation37 The degree of crystallinity is related to peak intensity. Sharp peaks indicate a highly crystallized particle state, whereas narrow peaks are indicators of low crystallinity.Citation38 In MHY-SLNs, the peak intensity was reduced when compared with Compritol, demonstrating a decreased crystallinity of MHY-SLNs. DSC measures the crystallinity of samples based on their corresponding melting enthalpies or melting points. Thermograms of MHY498, bulk Compritol 888 ATO, and freeze-dried MHY-SLNs are represented in . The thermogram of MHY-SLNs did not show a melting peak for crystalline MHY498 at 110°C, meaning that MHY498 adopted an amorphous state in the SLN matrix. In this study, MHY498 and Compritol were dissolved in anhydrous ethanol. Therefore, MHY498 was dispersed in the lipid homogenously. This result is consistent with that of a previous report showing podophyllotoxin in SLNs in an amorphous state.Citation39 The melting point of Compritol (79°C) was slightly reduced in MHY-SLNs (76°C), suggesting that the β form of SLNs was adopted.Citation40 This melting point depression might be due to the small particle size (nanometer range), high surface area, and presence of surfactant.Citation38
In vitro release study
Simulation-based in vitro release experiments were performed to determine drug-release behavior from the particle matrix. The release pattern observed following in vitro release helps in determining the dose and treatment period in real in vivo settings. As represented in , MHY-SLNs showed a biphasic release pattern. Nearly, 70% of MHY498 was released from SLNs matrix within first 6 h, which fits the Higuchi kinetic model (R2=0.996). This initial fast release of MHY498 might play a key role in enhancing skin penetration, as it increases the concentration gradient to provide higher drug diffusion through the skin.Citation20 The remainder of the MHY498 (>90%) was released over 48 h, confirming the prolonged-release characteristics of MHY-SLNs.Citation41 The amorphous form of the drug allows slow diffusion leading to prolonged release from SLN matrixes.Citation42 The XRD and DSC data of MHY-SLNs demonstrated the amorphous state of MHY498 in SLN matrixes (). This biphasic release pattern could be important in skin delivery. The initial fast release can facilitate MHY498 skin permeation by providing a sufficient concentration gradient, whereas the sustained release maintains the local concentration, leading to a prolonged antimelanogenesis effect of MHY498.
In vitro skin-permeation study
The skin permeability of MHY-SLNs was evaluated and compared with that of the MHY solution. MHY498 is a poor water-soluble drug. It is poorly soluble in common organic solvents, such as dichloromethane, chloroform, and acetone. Solubility of MHY498 is only prominent in low-molecular weight alcohol, such as methanol, ethanol, propylene glycol, and DMSO. Among them, ethanol and propylene glycol are widely used as emollients, solvents, and penetration enhancers in various topical dosage forms.Citation43 A 2:1 ratio of ethanol:propylene glycol was used as a solvent for MHY solution. DMSO was excluded from the study considering its smell that may hamper the aesthetic appeal of cosmetics or topical dosage form. demonstrates the skin-penetration pattern of MHY solution and MHY-SLNs. MHY-SLNs showed higher skin permeation than did the MHY solution. In the case of MHY solution, it took 4 h to initiate skin permeation. A lag time is a common phenomenon in skin-permeation studies. A similar lag time for ethanol and propylene glycol was previously reported.Citation44 This might be due to the fact that using propylene glycol can cause skin tortuosity by dehydrating the stratum corneum, resulting in a sufficiently increased diffusional length to enable passage through the skin.Citation45 However, MHY498 skin permeation from the SLN matrix began only after 30 min, which led to higher overall permeation than observed with the MHY solution after 48 h. Flux is another parameter used to evaluate skin permeability by measuring the amount of material crossing the skin per unit area per unit time. The flux at different time intervals (12, 24, and 48 h) was determined, and the results demonstrated that MHY-SLNs showed a 2-fold higher flux than did the MHY solution, in that 2 times more MHY498 from MHY-SLNs permeated through the skin than did MHY from MHY solution (). The high skin permeability of MHY-SLNs may result from the film-formation ability of SLNs, which is absent in solution formulations. Film formation causes skin hydration by preventing transepidermal water loss. The tightly arranged stratum corneum becomes swollen, corneocyte packing becomes loose, and MHY498 effectively permeates the skin through an intracellular route ().
Table 2 Flux determination of MHY-SLNs and MHY solution at different time intervals
In vivo evaluation of MHY-SLNs
The in vivo efficacy of MHY-SLNs was evaluated based on qualitative and quantitative brightness comparisons. C57BL/6 mouse dorsal skin was irradiated with UVB radiation. UVB radiation can penetrate the epidermal layer of the skin. UVB radiation (290–320 nm) increased MSH receptor activity on cutaneous melanocytes, which caused UVB-induced melanogenesis.Citation46,Citation47 Thus, melanocyte production increased and accumulated in the basal epidermal layer. Such melanocytes were responsible for skin darkening and pigmentation.Citation48,Citation49 represents the qualitative and quantitative skin-brightness comparisons, respectively, among the different groups tested. The L value is an indicator of skin brightness, where L values of 64–68 denote bright skin and L values of 40–45 denote dark skin. Before initiating UV radiation and treatment, the skin tissues were relatively bright with L values of 65±2. In the untreated group (UV control), UV radiation administered on alternate days over a 14-day period made the skin dark, and L value drops down to 40 (). In the MHY solution group, a gradual decrease of skin brightness was observed, but the skin remained brighter than the untreated group. In contrast, skin brightness was nearly unchanged after 14 days in the MHY-SLNs group. As mentioned above, MHY498 from an SLN matrix permeates the skin easily due to its occlusive property and enters into melanocytes in the epidermis. MHY498 prevents melanogenesis in melanocytes by inhibiting the rate-limiting enzyme tyrosinase. Therefore, melanin overproduction is controlled and helps maintain normal skin brightness. In blank-SLNs group, skin brightness dropped down to below 45 over 14 days of UVB radiation, supporting the fact that blank-SLNs do not have a role in preventing melanogenesis. Ethanol and propylene glycol also have the ability to penetrate the skin.Citation50 They can promote MHY498 permeation from MHY solution, but cannot prevent melanogenesis effectively. The above results were confirmed by histological evaluation. In response to UV radiation, the skin produces melanin from melanocytes to neutralize the effects of radiation. Fontana–Masson staining is a common histological technique used to stain melanin black and enable melanin detection in the epidermis.Citation51 As illustrated in , the melanin quantity was highest (indicated by arrow) in UV control group, providing evidence of UV radiation-induced melanogenesis. In the MHY solution group, significantly greater melanin production was observed, which was indicative of ineffective melanogenesis inhibition. However, in the MHY-SLNs group, almost no melanin was found in the epidermis, which demonstrated that MHY-SLNs inhibit UVB-induced melanogenesis in an effective manner.
Figure 5 In vivo evaluation of MHY-SLNs.
Abbreviations: MHY-SLNs, MHY498-loaded solid lipid nanoparticles; SD, standard deviation; UV control, ultraviolet control.
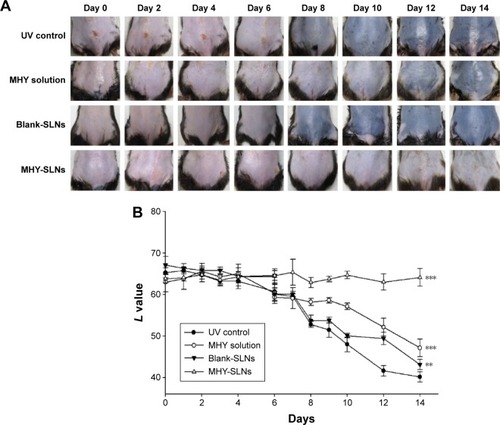
Figure 6 Fontana–Masson-stained sections of C57BL/6 skin from the UV control, MHY solution, and MHY-SLNs groups.
Abbreviations: MHY-SLNs, MHY498-loaded solid lipid nanoparticles; UV control, ultraviolet control.
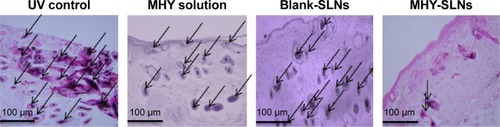
In vitro cytotoxicity study
The skin toxicity of topical medications is becoming a great concern.Citation52 The overall performance of any formulation is also related to its toxicity profile. Therefore, MHY-SLNs cytotoxicity was evaluated using the L929 mouse fibroblast cell line and compared with that of the MHY solution. The L929 cell line is commonly used in different experimental settings, such as when studying material biocompatibility and drug cytotoxicity.Citation53,Citation54 As shown in , MHY-SLNs showed no significant cytotoxicity (cell viability >90%) at concentrations of up to 400 µg MHY498/mL, whereas only 200 µg MHY498/mL was used for in vivo studies. Lipids used in SLN formulation are considered nontoxic and biocompatible. Potential toxicity concerns should be considered due to the presence of stabilizers (surfactants and cosurfactants).Citation55,Citation56 Poloxamer 188 and Phospholipon 90 G (used in the formulation) are nonionic compounds, which are considered the least problematic.Citation57 In the case of MHY solution, the cell viability was <80%, which indicated its more toxic nature. Comparing MHY solution with a blank solution indicated that toxicity might have been imparted from the solution used. It has been reported that ethanol and propylene glycol can change the lipid structures of the skin and damage the skin barrier, which might pose toxicity.Citation58 Moreover, the solvent concentration used was the minimum concentration required to dissolve MHY498.
Conclusion
MHY498 was successfully incorporated in SLNs with sufficient drug loading and encapsulation efficiency. MHY-SLNs were fabricated at the nanometer range and possessed occlusive properties that enhanced the skin permeation of MHY498. The results of in vitro release and in vitro skin-permeation studies revealed a prolonged release and skin permeation of MHY498 from SLN matrixes. In vivo evaluation in a C57BL/6 mouse model demonstrated that MHY-SLNs effectively prevented UVB-induced melanogenesis. MHY-SLNs were nontoxic at the minimum effective concentration. Thus, MHY-SLNs may be effective in treating hyperpigmentation.
Acknowledgments
This work was supported by a National Research Foundation of Korea (NRF) grant funded by the Korea government (MSIP; grant no 2009-0083538).
Disclosure
The authors report no conflicts of interest in this work.
References
- HearingVJMilestones in melanocytes/melanogenesisJ Invest Dermatol2011131E1E122094398
- BrennerMHearingVJThe protective role of melanin against UV damage in human skinPhotochem Photobiol200884353954918435612
- OlsonRLGaylorJEverettMASkin color, melanin, and erythemaArch Dermatol197310845415444745289
- HalderRMNoothetiPKEthnic skin disorders overviewJ Am Acad Dermatol200348Suppl 6S143S14812789168
- TimilshinaSBhuvanKKhanalMMarshaniBPaudyalBSkalko-BasnetNThe influence of ethnic origin on the skin photoageing: Nepalese studyInt J Cosmet Sci201133655355921635272
- ZoniosGBykowskiJKolliasNSkin melanin, hemoglobin, and light scattering properties can be quantitatively assessed in vivo using diffuse reflectance spectroscopyJ Invest Dermatol200111761452145711886508
- Roméro-GrailletCAberdamEBiagoliNMassabniWOrtonneJ-PBallottiRUltraviolet B radiation acts through the nitric oxide and cGMP signal transduction pathway to stimulate melanogenesis in human melanocytesJ Biol Chem19962714528052280568910416
- SchwahnDJXuWHerrinABBalesESMedranoEETyrosine levels regulate the melanogenic response to α-melanocyte-stimulating hormone in human melanocytes: implications for pigmentation and proliferationPigment Cell Res2001141323911277492
- KameyamaKTakemuraTHamadaYPigment production in murine melanoma cells is regulated by tyrosinase, tyrosinase-related protein 1 (TRP1), DOPAchrome tautomerase (TRP2), and a melanogenic inhibitorJ Invest Dermatol199310021261318429235
- SlominskiATobinDJShibaharaSWortsmanJMelanin pigmentation in mammalian skin and its hormonal regulationPhysiol Rev20048441155122815383650
- TiefKHahneMSchmidtABeermannFTyrosinase, the key enzyme in melanin synthesis, is expressed in murine brainEur J Biochem1996241112168898882
- ChangTSAn updated review of tyrosinase inhibitorsInt J Mol Sci20091062440247519582213
- ParvezSKangMChungHSSurvey and mechanism of skin depigmenting and lightening agentsPhytother Res2006201192193416841367
- HermannsJPierard-FranchimontCPierardGSkin colour assessment in safety testing of cosmetics. An overviewInt J Cosmet Sci2000221677218503462
- KimSHHaYMMoonKMAnti-melanogenic effect of (Z)-5-(2, 4-dihydroxybenzylidene)thiazolidine-2,4-dione, a novel tyrosinase inhibitorArch Pharm Res201336101189119723812774
- HaYMKimJAParkYJAnalogs of 5-(substituted benzylidene) hydantoin as inhibitors of tyrosinase and melanin formationBiochim Biophys Acta20111810661261921397665
- MarksRThe stratum corneum barrier: the final frontierJ Nutr200413482017S2021S
- MorpurgoGCatacuzzenoLPeruzziSBlasiPFiorettiBAre tyrosinase inhibitors in sunscreens and cosmetics enhancing UV carcinogenicity?Exp Dermatol201524754654725876676
- MuÈllerRHMaÈderKGohlaSSolid lipid nanoparticles (SLN) for controlled drug delivery – a review of the state of the artEur J Pharm Biopharm200050116117710840199
- MüllerRHRadtkeMWissingSASolid lipid nanoparticles (SLN) and nanostructured lipid carriers (NLC) in cosmetic and dermatological preparationsAdv Drug Deliv Rev200254Suppl 1S131S15512460720
- WissingSKayserOMüllerRSolid lipid nanoparticles for parenteral drug deliveryAdv Drug Deliv Rev20045691257127215109768
- XuWLeeMKDevelopment and evaluation of lipid nanoparticles for paclitaxel delivery: a comparison between solid lipid nanoparticles and nanostructured lipid carriersJ Pharm Investig2015457675680
- AblaMJSinghNDBangaAKRole of nanotechnology in skin delivery of drugsPercutaneous Penetration Enhancers Chemical Methods in Penetration EnhancementVerlagBerlinHeidelbergSpringer2016113
- WissingSLippacherAMüllerRInvestigations on the occlusive properties of solid lipid nanoparticles (SLN)J Cosmet Sci2000525313324
- AnsmannAKawaRNitscheMGondekHinventorsHenkel Kommanditgesellschaft Auf Aktien, assigneeContaining alkyl or alkenl oligoglycoside and fatty alcohol; cosmetics, drugsGoogle Patents US5795978 A1998818
- SoJWKimSParkJSPreparation and evaluation of solid lipid nanoparticles with JSH18 for skin-whitening efficacyPharma Dev Technol2010154415420
- FanHLiuGHuangYLiYXiaQDevelopment of a nanostructured lipid carrier formulation for increasing photo-stability and water solubility of phenylethyl resorcinolAppl Surf Sci2014288193200
- KiDHJungHCNohYWPreformulation and formulation of newly synthesized QNT3-18 for development of a skin whitening agentDrug Dev Ind Pharm201339452653322670797
- ToktonNPanichayupakaranantPDevelopment of ellagic acid rich pomegranate extract loaded nanostructured lipid carriers (NLC)Int J Pharm Pharm Sci20146259265
- ZhangHZhangF-MYanS-JPreparation, in vitro release, and pharmacokinetics in rabbits of lyophilized injection of sorafenib solid lipid nanoparticlesInt J Nanomedicine201272901291022787390
- SilvaAGonzález-MiraEGarcíaMPreparation, characterization and biocompatibility studies on risperidone-loaded solid lipid nanoparticles (SLN): high pressure homogenization versus ultrasoundColloids Surf B Biointerfaces201186115816521530187
- MüllerRDinglerAThe next generation after the liposomes: solid lipid nanoparticles (SLN, Lipopearls) as dermal carrier in cosmeticsEurocosmetics1998781926
- BrewerMSZhuLGBidnerBMeisingerDJMcKeithFKMeasuring pork color: effects of bloom time, muscle, pH and relationship to instrumental parametersMeat Sci200157216917622061360
- KomatsuHKitajimaAOkadaSPharmaceutical characterization of commercially available intravenous fat emulsions: estimation of average particle size, size distribution and surface potential using photon correlation spectroscopyChem Pharm Bull (Tokyo)1995438141214157553988
- LevyMYSchutzeWFuhrerCBenitaSCharacterization of diazepam submicron emulsion interface: role of oleic acidJ Microencapsul199411179928138877
- SchwarzCMehnertWSolid lipid nanoparticles (SLN) for controlled drug delivery II. Drug incorporation and physicochemical characterizationJ Microencapsul199916220521310080114
- YoushiaJKamelAOEl ShamyAMansourSDesign of cationic nanostructured heterolipid matrices for ocular delivery of methazolamideInt J Nanomedicine201272483249622679362
- VenkateswarluVManjunathKPreparation, characterization and in vitro release kinetics of clozapine solid lipid nanoparticlesJ Control Release200495362763815023472
- ChenHChangXDuDPodophyllotoxin-loaded solid lipid nanoparticles for epidermal targetingJ Control Release2006110229630616325954
- zur MühlenASchwarzCMehnertWSolid lipid nanoparticles (SLN) for controlled drug delivery – drug release and release mechanismEur J Pharm Biopharm19984521491559704911
- BaekJ-SChoC-WComparison of solid lipid nanoparticles for encapsulating paclitaxel or docetaxelJ Pharm Investi2015457625631
- BawaRAudetteGFRubinsteinIHandbook of Clinical Nanomedicine: Nanoparticles, Imaging, Therapy, and Clinical Applications1Boca Raton, FL, USACRC Press2016
- WilliamsACBarryBWPenetration enhancersAdv Drug Deliv Rev201264128137
- PanchagnulaRSalvePSThomasNSJainAKRamaraoPTransdermal delivery of naloxone: effect of water, propylene glycol, ethanol and their binary combinations on permeation through rat skinInt J Pharm20012191–29510511337170
- OstrengaJSteinmetzCPoulsenBYettSSignificance of vehicle composition II: prediction of optimal vehicle compositionJ Pharm Sci1971608118011835127091
- ChakrabortyAKFunasakaYSlominskiAUV light and MSH receptorsAnn N Y Acad Sci1999885110011610816644
- GilchrestBAParkHYEllerMSYaarMMechanisms of ultraviolet light-induced pigmentationPhotochem Photobiol19966311108577860
- LinJYFisherDEMelanocyte biology and skin pigmentationNature2007445713084385017314970
- HoglundEBalmPWinbergSSkin darkening, a potential social signal in subordinate arctic charr (Salvelinus alpinus): the regulatory role of brain monoamines and pro-opiomelanocortin-derived peptidesJ Exp Biol2000203111711172110804161
- LevangAKZhaoKSinghJEffect of ethanol/propylene glycol on the in vitro percutaneous absorption of aspirin, biophysical changes and macroscopic barrier properties of the skinInt J Pharm1999181225526310370221
- ProphetEBMillsBArringtonJBSobinLHLaboratory Methods in HistotechnologyWashington, DC, USAAmerican Registry of Pathology1992
- NohynekGJAntignacEReTToutainHSafety assessment of personal care products/cosmetics and their ingredientsToxicol Appl Pharmacol2010243223925920005888
- NurhasniHCaoJChoiMNitric oxide-releasing poly (lactic-co-glycolic acid)-polyethylenimine nanoparticles for prolonged nitric oxide release, antibacterial efficacy, and in vivo wound healing activityInt J Nanomedicine2015103065308025960648
- ZangeRKisselTComparative in vitro biocompatibility testing of polycyanoacrylates and poly (D, L-lactide-co-glycolide) using different mouse fibroblast (L929) biocompatibility test modelsEur J Pharm Biopharm1997442149157
- MüllerRMaabenSWeyhersHMehnertWPhagocytic uptake and cytotoxicity of solid lipid nanoparticles (SLN) sterically stabilized with poloxamine 908 and poloxamer 407J Drug Target1996431611708959488
- HeydenreichAVWestmeierRPedersenNPoulsenHSKristensenHGPreparation and purification of cationic solid lipid nanospheres – effects on particle size, physical stability and cell toxicityInt J Pharm20032541838712615415
- WeyenbergWFilevPVan den PlasDCytotoxicity of submicron emulsions and solid lipid nanoparticles for dermal applicationInt J Pharm20073371–229129817300887
- KwakSBriefELanglaisDKitsonNLafleurMThewaltJEthanol perturbs lipid organization in models of stratum corneum membranes: an investigation combining differential scanning calorimetry, infrared and 2H NMR spectroscopyBiochim Biophys Acta2012181851410141922366204