Abstract
Iron deficiency anemia is a frequent complication in clinical conditions such as chronic kidney disease, chronic heart failure, inflammatory bowel disease, cancer, and excessive blood loss. Given the ability of iron to catalyze redox reactions, iron therapy can be associated with oxidative stress. The lung is uniquely susceptible to oxidative stress, and little is known about the effects of intravenous iron treatment in this organ. This study characterized changes in markers of oxidative/nitrosative stress and inflammation in the lung of non-iron deficient, non-anemic rats treated with five weekly doses (40 mg iron per kg body weight) of low molecular weight iron dextran (LMWID), iron sucrose (IS), ferric carboxymaltose (FCM), ferumoxytol (FMX), iron isomaltoside 1000 (IIM), or saline (control). Rats treated with LMWID, FMX, or IIM showed significant changes in most measures of oxidative/nitrosative stress, inflammation, and iron deposition compared to the saline-treated controls, with greatest changes in the LMWID treatment group. Increases in products of lipid peroxidation (thiobarbituric acid reactive substances) and protein nitrosation (nitrotyrosine) were consistent with increases in the activity of antioxidant enzymes (catalase, Cu,Zn-SOD, GPx), decreases in antioxidative capacity (reduced:oxidized GSH ratio), increased levels of transcription factors involved in the inflammatory pathway (NF-κB, HIF-1α), inflammatory cytokines (TNF-α, IL-6), adhesion molecules (VCAM-1), markers of macrophage infiltration (ED-1), and iron deposition (Prussian blue, ferritin). Since changes in measured parameters in FCM- or IS-treated rats were generally modest, the results suggest that FCM and IS have a low propensity to induce lung inflammation. The relevance of these findings to clinical safety profiles of the tested intravenous iron products requires further investigation.
Introduction
Intravenous (iv) iron is utilized for the correction of iron deficiency (ID) and iron deficiency anemia (IDA). ID and IDA are common comorbidities of chronic conditions, including chronic kidney disease,Citation1 chronic heart failure,Citation2 inflammatory bowel disease,Citation3 and cancer,Citation4 but also associated with perinatal blood loss,Citation5 peri-surgical blood loss,Citation6 and abnormal uterine bleeding.Citation7 Direct uptake of iv iron by the reticuloendothelial system (RES) allows for rapid supply of iron to the bone marrow, even during treatment with erythropoiesis-stimulating agents.Citation4,Citation8,Citation9
Marketed iv iron products are formulations of low molecular weight iron dextran (LMWID), sodium ferric gluconate (FG), iron sucrose (IS), ferric carboxymaltose (FCM), and iron isomaltoside 1000 (IIM). One further product, ferumoxytol (FMX), was withdrawn from the European market upon request of the marketing authorization holder.Citation10 These products are colloidal solutions of complex polynuclear iron(III)-oxyhydroxide nanoparticles stabilized with a carbohydrate ligand, and differences in their composition and physicochemical properties may influence pharmacokinetics and side effect profiles.Citation11–Citation13 Iron carbohydrate complexes with lower stability, such as IS and FG, largely dissociate in the plasma. Consequently, a proportion of iron can be released into plasma before uptake by the RES.Citation14 This prematurely released “labile” iron can directly bind to transferrin and other proteins (referred to as non-transferrin bound iron, NTBI), leading to unspecific iron uptake by different tissues and organ toxicity.Citation15,Citation16
In a non-clinical model of iron-infused healthy, non-anemic rats, different iv iron compounds demonstrated significantly different effects in liver, heart, and kidney.Citation17–Citation19 Notably, even products that are marketed as generics of the reference product (IS) exerted different effects on oxidative stress and inflammation.Citation20 In a similar non-clinical model, a recent evaluation of selected iv iron compounds suggested that the induction of nitrosative stress markers is highest in the lung as compared to liver, heart, and kidney.Citation21
As the lung appears to be particularly susceptible to oxidative stress and injury, it is critical to accurately evaluate the potential of iv iron compounds for lung toxicity. In this study, by analyzing markers of oxidative/nitrosative stress and iron deposition, we compared the toxicological effects of currently available iv iron formulations in lung tissue of healthy rats.
Materials and methods
Animals and treatments
All animal experiments were approved by the Hospital Alemán Ethics Committee and the Teaching and Research Committee. Experiments were conducted in strict accordance with the Guide for the Care and Use of Laboratory Animals of the National Institutes of Health. Sprague-Dawley rats (n=8 per group; four male, four female) were fed, housed, and treated (five weekly iv injections of 40 mg iron/kg body weight or an equivalent volume of isotonic saline solution) as described previously.Citation22 As this study was designed to assess toxicological differences between various parenteral iron therapies, a high iron dose of 40 mg/kg was chosen to detect clear differences between iron compounds. The administered iv iron products comprised IS (Venofer®, American Regent, Shirley, NY, USA), FCM (Ferinject®, Vifor (International) Ltd., St Gallen, Switzerland), FMX (Feraheme®, AMAG Pharmaceuticals Inc., Lexington, MA, USA), LMWID (Infed®, Watson Pharma Inc., Morristown, NJ, USA), IIM (Monofer®, Pharmacosmos A/S, Holbæk, Denmark), or saline. Twenty-four hours after the final iv iron injection, rats were sacrificed and organs perfused with ice-cold saline before removal. Tissue samples for assessment of oxidative/nitrosative stress parameters were immersed in liquid nitrogen and stored at −80°C until analysis. Tissue samples for histological evaluation were submerged in fixation solution (phosphate-buffered 10% formaldehyde, pH 7.2), embedded in paraffin, and stored at room temperature.
Investigators were blinded to the treatments.
Oxidative stress evaluation
Portions of lung samples were homogenized in ice-cold 250 mM sucrose solution (1:3, w:v) for the determination of GSH, Cu,Zn-SOD, and GPx. For the determination of malondialdehyde (MDA), lung samples were homogenized in 50 mM sodium phosphate buffer (pH 7.4; 1:10, w:v). MDA was determined as thiobarbituric acid reactive species, and catalase as described previously.Citation17,Citation18
Nitrosative stress evaluation
Lung samples were homogenized in solubilization buffer (1:10 w/v), and clarified lysates were analyzed for nitrotyrosine via Western blotting with an anti-nitrotyrosine antibody (AB5411; EMD Millipore, Billerica, MA, USA) as previously described.Citation19,Citation23,Citation24 Immunoblots were developed by enhanced chemiluminescence (Amersham, Pittsburgh, PA, USA). Autoradiographs were scanned and band intensities quantified by digital densitometry using Gel-Pro Analyzer 4.0 software (Media Cybernetics, Rockville, MD, USA).
Protein loading was assessed by stripping the blot and re-probing with anti-β-actin (A2066; Sigma-Aldrich Co., St Louis, MO, USA).
Immunohistochemistry, cytochemistry, histomorphometry, and light microscopy
Preparation and processing of tissue samples for evaluation by light microscopy was performed as described previously.Citation17,Citation18 In brief, portions of lung were cut, fixed in phosphate-buffered 10% formaldehyde (pH 7.2), and embedded in paraffin. Then, 3 μm sections were prepared and processed. Ferritin was detected with goat anti-ferritin heavy chain (H) polyclonal antibody (sc-14416; Santa Cruz Biotechnology Inc., Dallas, TX, USA) and mouse anti-ferritin light chain (L) monoclonal antibody (sc-14420; Santa Cruz Biotechnology Inc.). Cytokines were detected with monoclonal antibodies against rat TNF-α (AF-510-NA; R&D Systems, Inc., Minneapolis, MN, USA) and IL-6 (sc-1265; Santa Cruz Biotechnology Inc.). VCAM-1, HIF-1α, and VEGF were detected with rabbit polyclonal anti-VCAM-1 (sc-8304; Santa Cruz Biotechnology Inc.), mouse monoclonal anti-HIF-1α (Novus Biologicals, Inc., Littleton, CO, USA), and mouse monoclonal anti-VEGF (sc-7269; Santa Cruz Biotechnology Inc.) antibodies, respectively. NF-κB65 was detected with mouse monoclonal anti-NF-κBp65 (sc-8008; Santa Cruz Biotechnology Inc.) antibody. Nitrotyrosine was detected with a rabbit polyclonal anti-nitrotyrosine antibody (AB5411; EMD Millipore). Macrophages were identified using a mouse anti-rat CD68 (ED1 clone) monoclonal antibody (MCA341; AbD Serotec, Raleigh, NC, USA).
Iron(III) deposits were detected with Prussian blue staining.
For histomorphometry, tissue sections were viewed by light microscopy and images were captured using a digital camera. Images were then processed by an image analyzer (Image-Pro Plus 4.5 for Windows; Media Cybernetics, Silver Spring, MD, USA). Morphological analyses were performed at a magnification of ×200 or ×400. Mean values of positive staining for iron(III), ferritin, TNF-α, IL-6, nitrotyrosine, VEGF, and VCAM-1 (expressed as the percentage of area with positive immunostaining/mm2) and nuclear HIF-1α, NF-κB65, and ED1 macrophages (expressed as number of cells with positive staining/mm2) were calculated for samples from each rat. All evaluations were performed by two independent observers. Both observers were blinded to sample identity.
Statistical analysis
Values are expressed as mean ± SD. Statistical analyses were performed with absolute values and processed using GraphPad Prism, version 5.01 for Windows (GraphPad Software, Inc., La Jolla, CA, USA). For parameters with Gaussian distribution, comparisons among groups were performed using ANOVA followed by Tukey’s post hoc test. For parameters with non-Gaussian distribution (ie, histology data) comparisons were performed by Kruskal–Wallis test (non-parametric ANOVA) and Dunn’s multiple comparison test. A value of P<0.05 was considered significant.
Results
Oxidative and nitrosative stress
Levels of MDA, a marker of lipid peroxidation, were significantly elevated in lung homogenates of iv iron-treated compared to saline-treated rats (P<0.05) (). Rats treated with LMWID or IIM showed the greatest increase in MDA levels. FCM-treated rats had MDA levels closest to those observed in the control group. Compared to the control group, activity levels of the antioxidant enzymes catalase, CuZn-SOD, and GPx were significantly increased in rats treated with LMWID, IIM, and FMX (P<0.05). The ratio of reduced to oxidized GSH (GSH:GSSG ratio), a measure for antioxidative capacity, was significantly decreased in lung samples from animals treated with LMWID, IIM, FMX, and IS compared to levels in controls (P<0.05).
Table 1 Oxidative and nitrosative stress parameters
Immunostaining of nitrotyrosine, a marker of nitrosative stress, was significantly increased in lungs of rats treated with LMWID, FMX, and IIM compared to controls (P<0.05). Moreover, nitrotyrosine formation was significantly greater in the LMWID and FMX groups than the FCM and IS groups (P<0.05). Nitrotyrosine formation was primarily localized to alveolar cells (). Western blot analysis of solubilized lung tissue () showed significantly elevated levels of nitrotyrosine in the LMWID, FMX, and IIM groups compared to the control group, with highest levels in the LMWID- and FMX-treated rats.
Figure 1 Nitrotyrosine immunohistochemistry.
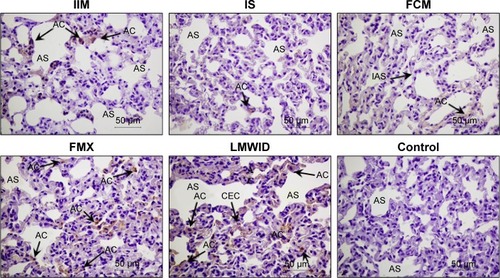
Figure 2 Nitrotyrosine Western blot analysis of lung homogenates.
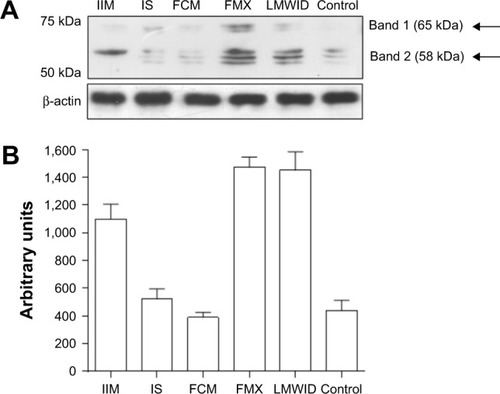
Inflammatory response
Immunohistochemistry of lung tissue sections indicated that levels of pro-inflammatory cytokines (TNF-α and IL-6) and relevant transcription factors (HIF-1α and NF-κB65) were significantly increased in animals treated with LMWID, FMX, and IIM compared to controls (P<0.05) (). Staining of all four inflammation markers was highest in the LMWID group. Light microscopy indicated that staining was primarily localized to alveolar cells ( and –). In agreement with the cytokine data, the number of cells with positive staining for the macrophage-specific CD68 (ED1) antigen was significantly increased in the LMWID, FMX, and IIM groups compared to controls (P<0.05). Light microscopy indicated that ED1-positive macrophages were localized in the lung interstitium ().
Table 2 Inflammation parameters
Figure 3 HIF-1α immunohistochemistry.
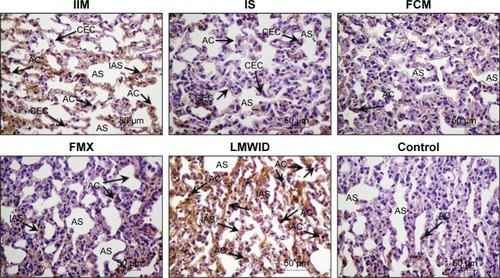
Figure 4 ED1 immunohistochemistry.
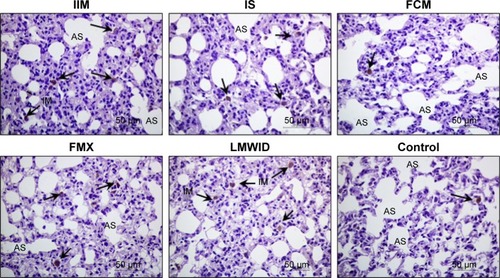
Compared to control animals, immunostaining for VCAM-1 and VEGF was significantly increased in animals treated with LMWID, FMX, and IIM (P<0.05) (). VEGF and VCAM-1 immunostaining was present in endothelial and alveolar cells. Marked VCAM-1 staining was, additionally, present in lung interstitium, particularly in the LMWID and FMX groups ( and ).
Tissue iron and ferritin
Prussian blue staining and ferritin L chain immunostaining indicated that, compared to saline-treated control, iv administration of LMWID, IIM, and FMX to rats significantly increased iron deposition (P<0.01) (, ). These parameters were not statistically different between FCM, IS, and control groups. Notably, Prussian blue staining was markedly elevated in LMWID-treated rats, whereas ferritin L chain levels were similarly elevated in LMWID-, FMX-, and IIM-treated rats. Ferritin H chain levels were only modestly elevated by LMWID and FMX treatment. Prussian blue staining was mainly localized in capillary endothelial cells, alveolar cells, interstitial macrophages (IMs), and interalveolar septum (). Ferritin L and H chain immunostaining was localized, predominantly, in alveolar cells ( and ). FCM and IS were not associated with significant iron deposition in the lung compared to control.
Table 3 Tissue iron and ferritin
Figure 5 Prussian blue staining.
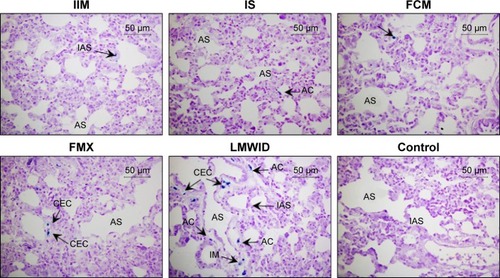
Discussion
Following administration of various iv iron products (LMWID, FMX, IIM, FCM, and IS) or saline to non-anemic healthy rats, we identified product-dependent toxicological effects such as oxidative/nitrosative stress, inflammation, and iron deposition in the lung of treated rats. Compared to saline control, administration of LMWID, FMX, and IIM was associated with statistically significant increases in virtually all measured parameters; LMWID treatment demonstrated the greatest effects on measured toxicological parameters. Increases in markers of lipid peroxidation (MDA) and protein nitration (nitrotyrosine) were consistent with increased activity of antioxidant enzymes (catalase, CuZn-SOD, GPx) and decreases in antioxidative capacity (GSH:GSSG ratio). In line with oxidative stress being a trigger of inflammation,Citation25–Citation28 levels of inflammatory pathway transcription factors (NF-κB, HIF-1α), inflammatory cytokines (TNF-α, IL-6), adhesion molecules (VCAM-1), and markers of macrophage infiltration (ED-1) were increased. Changes in measured parameters were generally modest in FCM- and IS-treated rats compared to animals receiving saline. This suggests that FCM and IS have a low propensity to induce lung inflammation. Notably, increases in oxidative stress markers aligned with increases in iron deposition.
While some studies report methods for direct measurement of reactive oxygen/nitrogen species (ROS/RNS), surrogate markers of oxidative/nitrosative stress are also widely established and have been measured in the present study. A typical surrogate marker of oxidative stress is MDA, a product formed when polyunsaturated lipids are degraded by ROS. A typical surrogate marker for nitrosative stress is nitrotyrosine formation, a consequence of the reaction between nitric oxide (NO) and a superoxide anion to form the highly reactive and damaging peroxynitrite (ONOO-) anion, which in turn reacts with tyrosine residues in proteins.
Using a similar model, a previous non-clinical study measured nitrotyrosine and dinitrophenyl in lung tissue after iv iron treatment.Citation21 Compared to our study, the iron dose was substantially lower (1 mg/kg, five times weekly), corresponding to the maximum single dose of FG rather than the maximum single dose of more stable iv iron compounds (20 mg/kg). Nonetheless, at this low dose, significant effects on nitrosative stress were detected in lung, liver, heart, and kidney.
Release of so-called “labile” iron from iv iron complexes and its unphysiological binding to transferrin and other proteins (referred to as NTBI) is associated with oxidative stress.Citation14 Labile iron release is highest in complexes of low or moderate stability (FG and IS) but considerably lower in stable complexes (LMWID, IIM, FMX, and FCM).Citation11,Citation13,Citation17 However, in the present study, LMWID, IIM, and FMX, but not FCM were associated with significant induction of oxidative/nitrosative stress in the lung. This is consistent with studies that evaluated these parameters in the liver, kidney, and heart.Citation17,Citation18 Therefore, in addition to labile iron release, other physicochemical properties must contribute to oxidative stress.
Differences in biodegradability of carbohydrate ligands may explain this observation.Citation14 While the carboxymaltose shell of FCM can be degraded by endogenous α-amylase, the depolymerization of dextran requires other mechanisms that depend on dextran structure or the production of O2 and NO radicals. Furthermore, dextran-based ligands can impart longer half-lives on iron complexes.Citation9,Citation17,Citation21 Together, these factors may result in iron accumulation in cells of the RES, increased generation of ROS/RNS, formation of hemosiderin, and retention of ferritin in macrophages.Citation14,Citation29
Increased Prussian blue staining in capillary endothelial cells, alveolar cells, IMs, and the interalveolar septum was particularly prominent in LMWID- and FMX-treated animals. Increased ferritin L and H chain levels were seen in alveolar cells of LMWID-, IIM-, and FMX-treated animals. This indicates product-dependent iron deposition in both the vascular system and lung tissue. Notably, accumulation of iron and inflammatory response was most evident in alveolar cells. Considering that nano-sized Fe2O3 particles increase microvascular permeability in lung epithelia, it is reasonable to suggest that iron from capillary endothelial cells may be rapidly accessed by alveolar epithelial cells.Citation30 As described previously, hepcidin does not appear to modify cellular iron metabolism in the lung. Greater supply of iron may, therefore, result in iron overload in alveolar cells.Citation31 Additionally, several in vitro studies indicate a positive correlation between iron concentration and chemokine release from alveolar epithelial cells. Collectively, this information suggests that alveolar cells may be more susceptible to iron uptake and subsequent inflammatory insult. Signs of thickening in the interalveolar septum may suggest formation of local edema or cellular infiltration.
Alveolar macrophages (AMs) defend against airway infection and airborne particles.Citation32 AMs are highly active in metal transport and can accumulate high concentrations of iron that overwhelm ferritin storage and lead to oxidative injury.Citation33 Due to low levels of ferritin H-subunits, AMs are themselves highly susceptible to oxidative/nitrosative stress.Citation34 Our findings show increased numbers of interstitial ED1 macrophages in animals treated with LMWID, IIM, and FMX. This may indicate an inflammatory response initiated by tissue iron deposition and ensuing ROS/RNS production.
In rats, AMs but not IMs are reported to be ED1-positive.Citation35 Without additional phenotyping, we cannot infer the origin of the interstitial ED1-positive macrophages detected in our study. AMs may have been derived from resident macrophages or from cells that migrated into the lung in response to iron-induced increases in VCAM-1 or increased capillary leakage mediated by VEGF. In inflammatory lung disorders like COPD, Prussian blue-positive AMs are detected in lung tissue, and AM iron levels correlate with disease severity.Citation36
In the lung, inflammation can exacerbate iron-induced oxidative/nitrosative stress by mechanisms that include increased iron uptake (eg, TNF-α induced upregulation of DMT-1 in bronchial epithelial cells) and increased iron retention (eg, IL-6 induced hepcidin production by airway epithelial cells and AMs).Citation37–Citation39 Therefore, lung iron deposition can trigger synergistic pathways that may perpetuate inflammation.
In terms of functional sequelae, it is possible that inflammation in the alveolar septum, as seen in our study, might impair normal bi-directional gas exchange between the alveolar space and capillary bed.
The primary objective of our study was to assess toxicological differences, that may relate to safety differences, between iv iron products. As such, distinctions between the non-clinical model used here and the clinical setting include the use of non-iron deficient, non-anemic animals and iron doses that exceed those used clinically. Despite these differences, findings from this study, and other rodent studies, demonstrate changes in oxidative stress biomarkers similar to those observed in the clinical setting.Citation14
In summary, repeat-dose administration of LMWID, FMX, and IIM to healthy rats was associated with significant increases in lung oxidative/nitrosative stress, inflammation, iron deposition, and numbers of interstitial ED1-positive macrophages compared to saline-treated controls. In contrast, FCM and IS administration was not associated with increases in most of the assessed parameters. The relevance of these findings to clinical safety requires further investigation.
Acknowledgments
The study was financially supported by Vifor (International) Ltd. Scientific writing support was provided by Lee Mizzen, Taija Koskenkorva-Frank (both Vifor Pharma) and Walter Fürst (SFL Regulatory Affairs & Scientific Communication, funded by Vifor Pharma). Support for graphics and illustrations were provided by Tracey Nichols (Tracey Nichols Graphic Design Ltd.).
Supplementary materials
Figure S1 NF-κB65 immunohistochemistry.
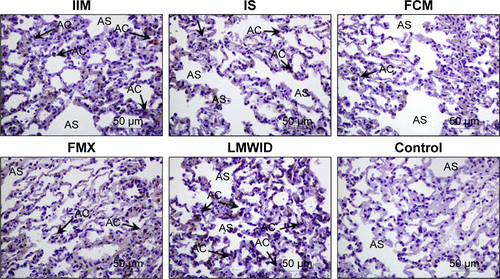
Figure S2 TNF-α immunohistochemistry.
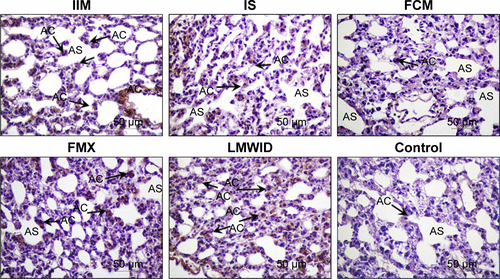
Figure S3 IL-6 immunohistochemistry.

Figure S4 VEGF immunohistochemistry.
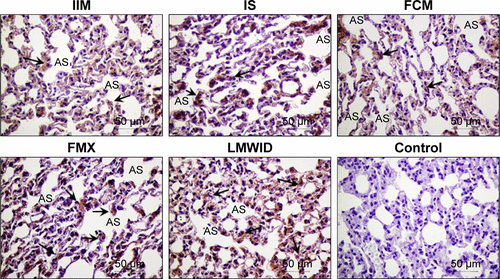
Figure S5 VCAM-1 immunohistochemistry.

Figure S6 Ferritin L chain immunohistochemistry.
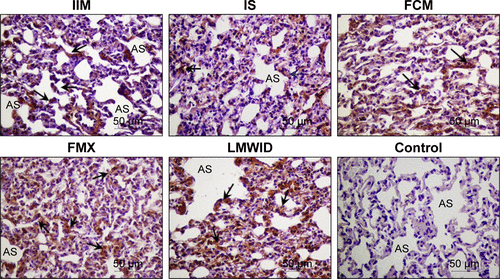
Figure S7 Ferritin H chain immunohistochemistry.
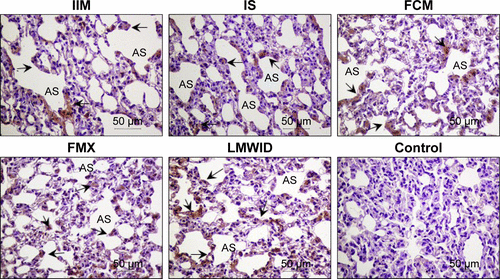
Disclosure
Jorge E Toblli has received research grants and consultancy fees from Vifor (International) Ltd., Switzerland. Gabriel Cao, Jorge F Giani, Fernando P Dominici, and Margarita Angerosa have no conflicts of interest to report.
References
- MacdougallICGeisserPUse of intravenous iron supplementation in chronic kidney disease: an updateIran J Kidney Dis201371 9 2223314137
- SilverbergDSWexlerDBlumMIainaAThe cardio renal anemia syndrome: correcting anemia in patients with resistant congestive heart failure can improve both cardiac and renal function and reduce hospitalizationsClin Nephrol200360Suppl 1 S93 S10212940539
- WilsonAReyesEOfmanJPrevalence and outcomes of anemia in inflammatory bowel disease: a systematic review of the literatureAm J Med2004116Suppl 7A 44S 49S15050885
- AaproMOsterborgAGasconPLudwigHBeguinYPrevalence and management of cancer-related anaemia, iron deficiency and the specific role of i.v. ironAnn Oncol2012238 1954 196222575608
- BodnarLMCogswellMEScanlonKSLow income postpartum women are at risk of iron deficiencyJ Nutr20021328 2298 230212163678
- BerisPMunozMGarcia-ErceJAThomasDManiatisAVan der LindenPPerioperative anaemia management: consensus statement on the role of intravenous ironBr J Anaesth20081005 599 60418372258
- MorrisonJPatelSTWatsonWZaidiQRMangioneAGossTFAssessment of the prevalence and impact of anemia on women hospitalized for gynecologic conditions associated with heavy uterine bleedingJ Reprod Med2008535 323 33018567277
- MacdougallICStrategies for iron supplementation: oral versus intravenousKidney Int Suppl199969 S61 S6610084288
- GeisserPBurckhardtSThe pharmacokinetics and pharmacodynamics of iron preparationsPharmaceutics201131 12 3324310424
- European Medicines AgencyRiensoWithdrawal of the Marketing Authorisation in the European UnionEMA2015 Available from: www.ema.europa.eu/docs/en_GB/document_library/Public_statement/2015/07/WC500189474.pdfAccessed July 13, 2017
- JahnMRAndreasenHBFuttererSA comparative study of the physicochemical properties of iron isomaltoside 1000 (Monofer), a new intravenous iron preparation and its clinical implicationsEur J Pharm Biopharm2011783 480 49121439379
- LocatelliFDel VecchioLNew erythropoiesis-stimulating agents and new iron formulationsContrib Nephrol2011171 255 26021625121
- NeiserSRentschDDipponUPhysico-chemical properties of the new generation IV iron preparations ferumoxytol, iron isomaltoside 1000 and ferric carboxymaltoseBiometals2015284 615 63525801756
- Koskenkorva-FrankTSWeissGKoppenolWHBurckhardtSThe complex interplay of iron metabolism, reactive oxygen species, and reactive nitrogen species: insights into the potential of various iron therapies to induce oxidative and nitrosative stressFree Radic Biol Med201365C 1174 1194
- AndrewsNCSchmidtPJIron homeostasisAnnu Rev Physiol200769 69 8517014365
- EvansRWRafiqueRZareaANature of non-transferrin-bound iron: studies on iron citrate complexes and thalassemic seraJ Biol Inorg Chem2008131 57 7417906879
- ToblliJECaoGOliveriLAngerosaMEvaluation of toxicity and oxidative stress induced by intravenous iron isomaltoside 1000 in a nonclinical modelArzneimittelforschung20116110 553 56522164963
- ToblliJECaoGOliveriLAngerosaMAssessment of the extent of oxidative stress induced by intravenous ferumoxytol, ferric car-boxymaltose, iron sucrose and iron dextran in a nonclinical modelArzneimittelforschung2011617 399 41021899208
- ToblliJECaoGGianiJFDominiciFPAngerosaMNitrosative stress and apoptosis by intravenous ferumoxytol, iron isomaltoside 1000, iron dextran, iron sucrose, and ferric carboxymaltose in a nonclinical modelDrug Res (Stuttg)2015657 354 36025050519
- ToblliJECaoGOliveriLAngerosaMComparison of oxidative stress and inflammation induced by different intravenous iron sucrose similar preparations in a rat modelInflamm Allergy Drug Targets2012111 66 7822309085
- BailieGRSchulerCLeggettREOxidative effect of several intravenous iron complexes in the ratBiometals2013263 473 47823681275
- ToblliJECaoGOliveriLAngerosaMDifferences between original intravenous iron sucrose and iron sucrose similar preparationsArzneimittelforschung2009594 176 19019517894
- GianiJFGironacciMMMunozMCPenaCTurynDDominiciFPAngiotensin-(1 7) stimulates the phosphorylation of JAK2, IRS-1 and Akt in rat heart in vivo: role of the AT1 and Mas receptorsAm J Physiol Heart Circ Physiol20072932 H1154 H116317496209
- MunozMCArgentinoDPDominiciFPTurynDToblliJEIrbesartan restores the in-vivo insulin signaling pathway leading to Akt activation in obese Zucker ratsJ Hypertens2006248 1607 161716877964
- ForsytheJAJiangBHIyerNVActivation of vascular endothelial growth factor gene transcription by hypoxia-inducible factor 1Mol Cell Biol1996169 4604 46138756616
- KiriakidisSAndreakosEMonacoCFoxwellBFeldmannMPaleologEVEGF expression in human macrophages is NF-kappaB-dependent: studies using adenoviruses expressing the endogenous NF-kappaB inhibitor IkappaBalpha and a kinase-defective form of the IkappaB kinase 2J Cell Sci2003116Pt 4 665 67412538767
- RahmanIMacNeeWOxidative stress and regulation of glutathione in lung inflammationEur Respir J2000163 534 55411028671
- SantusPCorsicoASolidoroPBraidoFDi MarcoFScichiloneNOxidative stress and respiratory system: pharmacological and clinical reappraisal of N-acetylcysteineCOPD2014116 705 71724787454
- KurzTTermanAGustafssonBBrunkUTLysosomes in iron metabolism, ageing and apoptosisHistochem Cell Biol20081294 389 40618259769
- ZhuMTFengWYWangBComparative study of pulmonary responses to nano- and submicron-sized ferric oxide in ratsToxicology20082472–3 102 11118394769
- GiorgiGD’AnnaMCRoqueMEIron homeostasis and its disruption in mouse lung in iron deficiency and overloadExp Physiol201510010 1199 121626047483
- AggarwalNRKingLSD’AlessioFRDiverse macrophage populations mediate acute lung inflammation and resolutionAm J Physiol Lung Cell Mol Physiol20143068 L709 L72524508730
- GhioAJDisruption of iron homeostasis and lung diseaseBiochim Biophys Acta200917907 731 73919100311
- MateosFBrockJHPerez-ArellanoJLIron metabolism in the lower respiratory tractThorax1998537 594 6009797761
- van der Brugge-GamelkoornGJDijkstraCDSminiaTCharacterization of pulmonary macrophages and bronchus-associated lymphoid tissue (BALT) macrophages in the rat. An enzyme-cytochemical and immunocytochemical studyImmunobiology19851695 553 5622995243
- PhilippotQDesleeGAdair-KirkTLIncreased iron sequestration in alveolar macrophages in chronic obstructive pulmonary diseasePLoS One201495 e9628524789352
- WangXGarrickMDYangFDaileyLAPiantadosiCAGhioAJTNF, IFN-gamma, and endotoxin increase expression of DMT1 in bronchial epithelial cellsAm J Physiol Lung Cell Mol Physiol20052891 L24 L3315749739
- FrazierMDMamoLBGhioAJTuriJLHepcidin expression in human airway epithelial cells is regulated by interferon-gammaRespir Res201112 10021810240
- NguyenNBCallaghanKDGhioAJHaileDJYangFHepcidin expression and iron transport in alveolar macrophagesAm J Physiol Lung Cell Mol Physiol20062913 L417 L42516648237