Abstract
The oral cavity is an excellent place for various microorganisms to grow. Spectrococcus mutans and Spectrococcus sanguinis are Gram-negative bacteria found in the oral cavity as pioneer biofilm formers on the tooth surface that cause caries. Caries treatment has been done with antibiotics and therapeutics, but the resistance level of S. mutans and S. sanguinis bacteria necessitates the exploration of new drug compounds. Black cumin (Nigella sativa Linn.) is known to contain secondary metabolites that have antioxidant, antibacterial, anti-biofilm, anti-inflammatory and antifungal activities. The purpose of this review article is to present data on the potential of Nigella sativa Linn seeds as anti-biofilm. This article will discuss biofilm-forming bacteria, the resistance mechanism of antibiotics, the bioactivity of N. sativa extracts and seed isolates together with the Structure Activity Relationship (SAR) review of N. sativa compound isolates. We collected data from reliable references that will illustrate the potential of N. sativa seeds as anti-biofilm drug.
Introduction
The oral cavity is an excellent place for various microorganisms to grow, due to conditions that are always moist by saliva, especially on the surface of the teeth and mucosa. It is estimated that there are 500–1000 species of bacteria and fungi growing in the oral cavity.Citation1,Citation2 Spectrococcus mutans and Spectrococcus sanguinis are known to infect the oral cavity by synthesizing biofilm layer on the tooth surface that forms caries. As a biofilm-forming pioneer, S. mutans signals to other bacteria to form more colonies and strengthen the biofilm. S. sanguinis as one of the bacteria receiving signals from S. mutans cooperates to create a stronger biofilm layer. With the help of the enzyme gluconastransferase, the biofilm layer formed becomes caries.Citation3,Citation4 Dental caries is considered a chronic disease that can affect all groups. In fact, the handling and treatment of caries is still very lacking, which causes this disease to continue.Citation5 In addition, the use of antibiotics as a cure for dental caries is resistant. Treatment is very urgent and new drug alternatives are needed. Nigella sativa Linn. seeds are known to contain bioactive compounds that have potential as an alternative treatment for dental caries.
N. sativa seed extract contains secondary metabolite compounds of terpenoids, alkaloids, flavonoids and phenolics.Citation6,Citation7 Several researchers have reported that the compounds contained in N. sativa seeds have antibacterial, anti-biofilm, anti-inflammatory and antifungal activities.Citation8–10 Bioactivity of N. sativa has potential as a natural caries drug.Citation11 Based on these data, this review will focus on the activity of compounds from N. sativa seeds against biofilm-forming bacteria. This review will also review the Structural Relationship of compounds from N. sativa seeds and the mechanism of inhibition of biofilm-causing bacteria.
Biofilm Forming Gram-Positive and Gram-Negative Bacteria
Gram-Positive Bacteria
Spectrococcus mutans
S. mutans is an anaerobic Gram-positive bacterium found in the oral cavity.Citation12 The condition of the oral cavity always changes in pH, temperature and pressure requiring S. mutans adaptation. This situation causes S. mutans to synthesize new metabolites that change its physiological properties. The resulting consequences such as increased biofilm homeostasis become dental caries.Citation13 S. mutans mediates gluconastransferase (Gtf), which forms extracellular polysaccharides (EPS) on the tooth surface. EPS is formed at the adhesion stage utilizing sucrose with the help of the enzyme gluconastransferase (Gtfs). This enzyme catalyzes the breakdown of sucrose into glucose and fructose. Through glycoside bonds extracellular glucans are formed (EPS). At this stage, the biofilm that forms is still small, followed by the proliferation stage, specific caries bacteria will survive on the biofilm so that caries forms.Citation14 The EPS formed becomes a food source and ensures the survival of biofilm-forming bacterial colonies.Citation14,Citation15 In addition, EPS protects colonies from host attacks and antibiotics. Microorganisms such as Candida albicans, Porphyromonas, Prevotella, Fusobacterium nucleatum and others are incorporated into colonies contained in biofilms.Citation16
Biofilms can be inhibited by stopping the synthesis of EPS catalyzed by Gtf enzymes (shown in ). This can be done using micro molecules, natural products, probiotics and prebiotics. The introduction of components into EPS results in changes in EPS regulation.Citation17 At this stage, new EPS components are formed that need to be prevented from being occupied by new bacterial colonies using antibiotics. EPS degradation and the presence of antibiotics reduce biofilms and dental caries.Citation18
Figure 1 The mechanism of biofilm inhibition is by inhibiting the gluconastransferase enzyme of S. mutans as a catalyst for EPS formation. Unavailable EPS causes bacteria not to colonize to form biofilms.Citation18
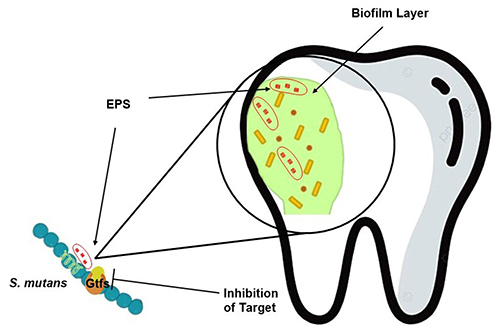
In the biofilm formed by S. mutans, amyloid fibers of different sizes were found. Amyloid fibers are fibers that can protect the biofilm layer from aggregation and environmental influences such as enzymes. S. mutans synthesizes amyloid with the help of the enzyme transpeptidase sortase which connects the substrate and cell wall peptidoglycan. The characterization results of amyloid fibers formed have two different types of sizes and add to the biofilm inhibition route. The presence of molecules that can lyse amyloid fibers can reduce the attachment strength of biofilms.Citation19,Citation20
Streptococcus sangunis
Streptococcus sanguinis (S. sanguinis) is a class of Gram-positive mythical Streptococcus found in toddlers, adolescents and adults.Citation21 Like its main pioneer (S. mutans), it is involved in forming biofilms that synergize with other bacterial species such as Corynebacterium durum.Citation22 Multiple biofilms were observed in OKF4/TERT-1 and hTERT TIGKs artificial cells containing S. sanguinis and C. durum as commensal bacteria and Porphyromonas gingivalis as pathobionts. S. sanguinis contributed almost 96.6% to the multiple biofilms formed by the three species, while C. durum and P. gingivalis only 1.6 and 1.8%, respectively. A very dominant contribution was shown by S. sanguinis in biofilm formation.Citation23 S. sanguinis also has the ability to ferment polysaccharides into acidic compounds. This causes demineralization of enamel in the teeth which initiates caries.Citation24
The close relationship between S. mutans and S. sanguinis affects the biofilm formed. Both support each other providing a source of attachment for other microorganisms.Citation25 However, research conducted by Cui et al membrane vesicles from S. mutans can inhibit biofilm formation from S. sanguinis. This unique property causes biofilm inhibition to be more diverse.Citation26
In addition to biofilm formation, S. sanguinis encodes pili IV in the metabolism that causes infective endocarditis (IE) disease. S. sanguinis can bind to the platelet protein matrix provided by S. mutans on biofilms.Citation27 This results in S. sanguinis being able to exit the oral cavity and be carried by the bloodstream to the heart. The accumulation of S. sanguinis in the heart initiates IE.Citation28
Gram-Negative Bacteria
Aggregatibacter actinomycetemcomitans
Aggregatibacter actinomycetemcomitans (A. actinomycetemcomitans) is a Gram-negative bacterium in the oral cavity.Citation29 A. Actinomycetemcomitans is incorporated into biofilm colonies that affect the rate of biofilm formation. Although not very significant, the gene expression of A. Actinomycetemcomitans in biofilm is most dominant compared to other Gram-negative bacteria (P. Gingivalis, V. dispar, A. oris, and S. anginosus).Citation30
A. Actinomycetemcomitans expresses a fimbriae protein and three non-fimbriae surface proteins (EmaA, Aae and ApiA). These proteins play a role in forming biofilms on the tooth surface. EmaA protein expression is known to be the most dominant in biofilm formation compared to other non-fimbriae proteins.Citation31 OmpA1 and OmpA2 are also expressed which ensures the survival of A. Actinomycetemcomitan. OmpA1 and OmpA2 can interact with C4 binding pockets that inhibit lectin activity. This binding ability is what makes A. Actinomycetemcomitan resistant.Citation32
Antibiotics
Antibiotics are compound species that can inhibit and/or kill a pathogenic microorganism. In the early era, the discovery of antibiotics gave many changes to the world of medicine.Citation33 There was an extension of human lifetime compared to before the discovery of antibiotics.Citation34 A very big discovery turned out to have a big negative impact. In addition to high resistance to antibiotics, massive use has an impact on environmental pollution. Exposure to waste such as in fresh water is difficult to degrade and threatens survival.Citation35
Common Antibiotics Used in Caries Treatment
Rifamycin
Rifamycin is an antibiotic that has an aromatic ring structure (shown in ). Excellent activity is shown by rifamycin against Gram-positive and Gram-negative bacteria. The mechanism of rifamycin inhibition is through inhibiting the enzyme RNA polymerase (RNAP) which causes RNA synthesis to be inhibited. The structure of rifamycin has hydroquinone which can be auto-oxidized by the enzyme Rox monooxygenase in bacterial cells. Biofilm forming bacteria such as Staphylococcus aureus have the ability to repair genetic information systems. This causes rifamycin to be unable to inhibit RNA synthesis from S. aureus, making it resistant.Citation36–38
Figure 2 Structure of Rifamycin.Citation37
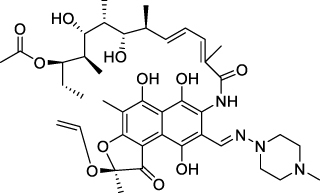
Amoxicillin
Amoxicillin has been used as an antibiotic since 1970 with a chemical structure having a β-lactam ring (shown in ). Treatment of dental caries infected by Gram-positive and Gram-negative bacteria often uses amoxicillin.Citation39 The inhibitory mechanism of amoxicillin by damaging the bacterial cell wall. Research results show that the use of amoxicillin increases the ability of bacteria to express β-lactamase enzymes that have structures similar to amoxicillin. Expression of β-lactamase enzyme makes bacteria recognize amoxicillin in the next treatment which causes resistance.Citation36 S. sanguinis and S. mitis are caries-causing bacteria that are resistant to amoxicillin.Citation40
Figure 3 β-lactam structure of amoxicillin.Citation41
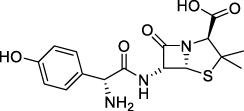
Chlorhexidine
Chlorhexidine is often used to treat dental caries to date. In the application of chlorhexidine, it is able to change the pH conditions in the oral cavity characterized by differences in the concentration of lactic acid, nitrate and nitrite.Citation42 The attachment of chlorhexidine to the bacterial cell wall is through charged interaction. Bacterial cell walls are negatively charged, while chlorhexidine ions are positively charged so that attachment occurs. Changes in pH in the oral environment cause an imbalance to occur, increasing the ability of microorganisms to express certain proteins. Like the main bacteria that cause dental caries, S. mutans is able to express the dlt operon which changes the surface structure of the cell wall to be very hydrophobic. As a result, the cell wall is positively charged and no ionic interaction with chlorhexidine occurs. The expression of the dlt operon from S. mutans causes it to be resistant to chlorhexidine (shown in ).Citation43
Figure 4 Bacterial member as a target for chlorhexidine attachment and bacterial member that is resistant to chlorhexidine due to the differential charge on the member surface by DltA.Citation43
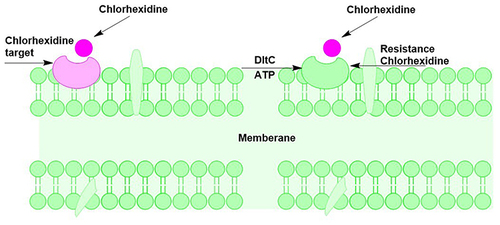
Florida Toothpaste
Florida is often used in toothpaste because of its excellent antibiotic activity. Florida can prevent tooth decay caused by pathogenic microorganisms. The tooth surface layer is re-mineralized by fluoride ions resulting in the erosion of dental calcium and phosphate to form the compound fluoride apatite [Ca5(PO4)3F]. Florida apatite is very useful for protecting teeth and preventing biofilm formation. In addition, the presence of chloride can suppress the amount of acid produced by bacteria so that teeth are protected from caries.Citation44,Citation45
The element fluoride has the highest electronegativity value among other chemical elements. Florida can attract electrons from bone and tooth building blocks such as calcium and cause damage to multicellular cell organelles. The use of fluoride in toothpaste in low concentrations is very beneficial, but the accumulation of fluoride due to continuous use raises concerns.Citation45 According to Tang et al, 2019 fluoride has a level of resistance to the cas3 gene that is expressed by the biofilm-forming bacteria Streptococcus mutans modifications to fluoride are needed to target cas3 so as to reduce resistance.Citation46
Antibiotics as MurA Enzyme Inhibitors
Various antibiotics target the destruction of the bacterial cell wall that holds the cell unit together. The main component of the bacterial cell wall is peptidoglycan, which is composed of a layer of disaccharides crosslinked with amino acids through peptide bonds.Citation47 Peptidoglycan is synthesized by a series of biological mechanisms in the cytoplasm, member and periplasm catalyzed by Mur (Muramidase) enzymes.Citation48 As well as caries-causing bacteria S. mutans synthesize peptidoglycan with the help of the enzyme Mur.Citation49 The enzymes MurA and MurB transfer PEP (phosphoenolvirupat) which converts UDP-GlcNac (uridine diphosphate-N-acetylglucosamine) into (uridine diphosphate-N-acetylmuramyl-pentapeptide) UDP-MurNAc.Citation50 UDP-MurNAc is then called the substrate to which the amino acids L-ala, D-Glu, L-Lys and D-Ala-D-Ala will be attached with the help of the enzymes MurC, MurD, MurE and MurF (shown in ). The amino acids on the substrate crosslink with the disaccharide to form a peptidoglycan. The enzyme MurA has been recognized as an important factor in peptidoglycan synthesis and is a target in antibiotic discovery.Citation51
Figure 5 Peptidoglycan formation mechanism. The MurA enzyme catalyzes the initial phase of peptidoglycan synthesis, making it a potential target for antibiotics to prevent bacterial cell wall formation.Citation47
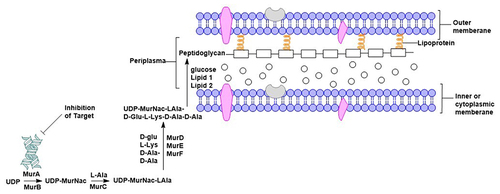
Secondary Metabolites as Caries Antibacterials
Flavonoids
Flavonoids are a class of secondary metabolites that have three distinctive rings. Flavonoid structures are classified into flavanone, flavanone, isoflavone, dihydroflavanol, flavonols, flavan-3,4-diol, flavan-3-ol and anthocyanins.Citation52 The fruitful structure makes flavonoids have antibacterial activity against Gram-positive and Gram-negative bacteria. The mechanism of flavonoid inhibition is through the interaction of hydroxy groups with bacterial proteins that are very important in metabolisms such as with topoisomerase, helicase, and DNA gyrase proteins.Citation53 This is based on the ability to transfer electrons and stabilize the core structure.Citation17,Citation52,Citation54–56
Terpenoids
Terpenoids have antibacterial activity against oral pathogens by damaging bacterial cell membranes.Citation56–59 Damaged cell membranes evidenced by protein release from oral bacterial cell membranes.Citation60
Alkaloids
Alkaloids inhibit the formation of peptidoglycan which causes damage to the bacterial cell wall. Alkaloids are known to have antibacterial activity against oral pathogens such as S. mutans and P. gingivalis.Citation59–63
Phenolic
Phenolic group compounds have antibacterial activity that causes dental caries.Citation64,Citation65 The antibacterial mechanism of the phenolic group by damaging the bacterial cell wall. The hydroxy group bound to the benzene ring allows the release of hydrogen atoms that will interact with the membrane wall and changes in membrane permeability occur.Citation64–67
Saponins
The saponin group has anti-inflammatory, anticancer and antibacterial therapeutic activities. As antibacterial saponins damage bacterial cells, causing cell death. Pathogenic oral bacteria such as P. gingivalis that cause caries and bad breath can be inhibited by this group.Citation67–71
Tannins
Tannins have diverse pharmacological activities due to their fertile structure. As oral antibacterial agents, tannins have the ability to inhibit quorum sensing and biofilm formation. Through the mechanism of inhibiting cell wall synthesis, member lysis and inhibiting the enzyme gluconastransferase, tannins can inhibit the formation of biofilms.Citation72–74
Black Cumin (Nigella sativa Linn.)
Black cumin (Nigella sativa Linn.) is a plant from the Ranunculaceae family that is widely cultivated in Asia, Europe, Africa and the Americas.Citation75 Native to the middle and far eastern regions, N. sativa seeds have abundant ethnopharmacology.Citation76,Citation77 Anti-inflammatory, antioxidant, cardiovascular, anticancer, antibacterial and antifungal are some of the known pharmacological activities of N. sativa.Citation75,Citation78 Black cumin seeds have been widely used traditionally to treat cancer, mental disorders and treat bacterial-induced diseases.Citation79 In various parts of the world, black cumin seeds have been consumed as a safe spice that has immune-boosting properties.Citation80 Ethnopharmacology is supported by secondary metabolite compounds contained in black cumin seeds. Alkaloids, polyphenols, phytosterols, terpenes, terpenoids and essential oils are secondary metabolites in N. sativa.Citation81
Secondary Metabolites Nigella sativa Linn
Volatile Compound
Samples of N. sativa seeds from Bangladesh and India were analyzed for volatile compound components using GC-MS. A total of 200 g of black cumin seeds were ground and dried at 40 ºC. The powder was analyzed using GC-MS A Hewlett-Packard (HP) 6890 Series II with FID detector and helium carrier gas. The analysis revealed volatile compounds octanoic acid, thymoquinone, thymol, p-cymene, maculosin, hygrine, ethyl ester and 2-monomyristin with main components thymoquinone, thymol and p-cymene are as shown in .Citation82 Volatile compounds from N. sativa seed extracts have oral antibacterial activity as on S. aureus and P. aeruginosa.Citation79,Citation83
Figure 6 Major volatile compounds of Nigella sativa Linn. oil, octanoic acid (1), thymoquinone (2), thymol (3), p-cymene (4), maculosin (5), hygrine (6), ethyl ester (7), 2-monomyristin (8).Citation6,Citation82,Citation84–88
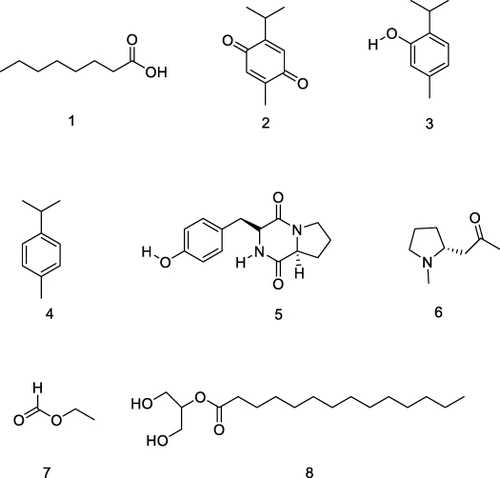
Polyphenols
Polyphenols were isolated from methanol and acetone extracts of N. sativa seeds. Separation using HPLC system variant pro-star model 230 with reversed-phase column. Eluents used were acetonitrile and glacial acetic acid with a 5% gradient. The methanol extract contained more polyphenol components than the acetone extract with concentrations of 13,714 and 0.5962 mg/g, respectively.Citation89 Polyphenolic compounds that were successfully isolated were reported by Enomoto et al was 2-(2-methoxy propyl)-5-methyl-1,4-benzenediol is shown in .Citation90
Figure 7 Structure polyphenols of 2-(2-methoxy propyl)-5-methyl-1,4-benzenediol.Citation90
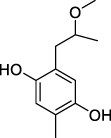
Terpenoids
Terpenoids are a class of nonpolar compounds obtained from N. sativa L. seed oil are shown in . Isolation of compounds can be done using preparative chromatography and open column chromatography. The oil from the refined seeds was extracted using the hydro-distillation method and columnized with the norm phase with the solvent n-hexanes-diethyl ether.Citation92
Figure 8 Terpenoid structures of N. sativa isolates cycloart-23-methyl-7,20,22-triene-3b, 30-diol (1) and cycloart-3-one-7,22-diene-24-ol (2).Citation96
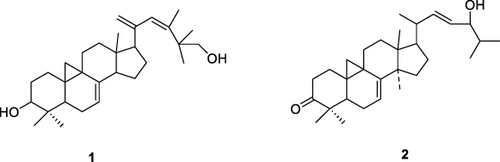
Alkaloids
Alkaloids were obtained from the polar fraction of N. sativa seed extracted using methanol-water 3:1. The dried polar fraction was analyzed using UPLC-C8-FT-MS/MS. The polar fraction was dissolved in 2000 μL of UPLC-grade MeOH/H2O (1:1, v/v). The column used was a C18 stationary phase, using a Waters Acquity UPLC system. Mass spectra were used to analyze the polar components through fragmentation patterns via ESI (-) and ESI (+) ionization methods.Citation94 Some of the alkaloid compounds successfully isolated from N. sativa seed are shown in .
Figure 9 Alkaloid structures of N. sativa isolates Derivate isoqunolin (1), 6.7-dimethoxy-1-methylisoquinoline (2), Nigellidine (3), Nigellicne (4), Nigellamines ((A1: (5a) R = Ph, X = CH), (A2: (5b) R = Ph, X = (N), (A3: (5c) R = n-C5H11, X = (N), (A4: (5d) R = n-C3H7, X = (N), (A5: (5e) R = PhCH2, X = (N)), Nigellamine C (6).Citation91,Citation92,Citation95,Citation96 Nigellicine (7),Citation93 Nigellidine (8),Citation97,Citation98 Strychnine (9),Citation99 17-O-(β-D-glucopyr-anosyl)-4-O-methylnigellidine (10), nigelanoid (11), 7-O-(β-D-Glucopyranosyl)-4-O-methylnigellidin (12), nigellidine (13), 8b4-O-methylnigellidine, 8bnigeglanine (14) dan 4-O-methylnigeglanin (15).Citation103
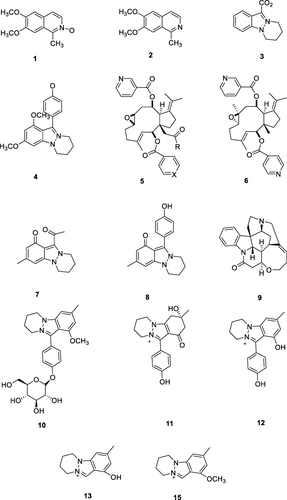
Saponins
Saponins can be extracted from N. sativa seeds using polar solvents such as methanol, butanol and ethanol. Saponin phytochemistry is very distinctive and can be analyzed quantitatively using TLC. The Rf (Retentions factor) value of saponins on the TLC plate is 0.8. TLC can be used in qualitative phytochemical analysis based on the Rf value.Citation97,Citation101,Citation102 Isolation of saponins from butanol extract can be done by open column chromatography. From column chromatography, twelve saponin isolates were obtained and are shown in .Citation100
Figure 10 Saponins structure of N. sativa are R1 = β-D-Xylp-(1-3)-α-L-Rhap-(l-2)- α-L-Arap-(b. R2 = α-L-Rhap-(1-4)- β-D-Glcp-(1-6)- β-D-Glcp-(1)(1),Citation91,Citation103 3-O-[β-D-xylopyranosyl-(1-3)-α-L-rhamnopyranosyl-(14)-β-D-glucopyranosyl]-11-methoxy-16-hydroxy-17-acetoxy (2),Citation104 a. R1= β-D-xylp-(1-2)- β-D-glcp-(1-b. R2= α-L-rhap-(1-4)-β-D-glcp-(1-6)-β-D-glcp-(1-flaaccidoside III (3), quercetin-3-gentiobioside (4), nigelflavonoside B (5), magnoflorine (6), nigelloside (7), quercetin sphorotrioside (8), kaempferol-3,7-diglucoside (9), kaempferol 3-O-rutinoside (10), rutin (11)Citation100 alpha-hederin (α-HN) (12).Citation109
![Figure 10 Saponins structure of N. sativa are R1 = β-D-Xylp-(1-3)-α-L-Rhap-(l-2)- α-L-Arap-(b. R2 = α-L-Rhap-(1-4)- β-D-Glcp-(1-6)- β-D-Glcp-(1)(1),Citation91,Citation103 3-O-[β-D-xylopyranosyl-(1-3)-α-L-rhamnopyranosyl-(14)-β-D-glucopyranosyl]-11-methoxy-16-hydroxy-17-acetoxy (2),Citation104 a. R1= β-D-xylp-(1-2)- β-D-glcp-(1-b. R2= α-L-rhap-(1-4)-β-D-glcp-(1-6)-β-D-glcp-(1-flaaccidoside III (3), quercetin-3-gentiobioside (4), nigelflavonoside B (5), magnoflorine (6), nigelloside (7), quercetin sphorotrioside (8), kaempferol-3,7-diglucoside (9), kaempferol 3-O-rutinoside (10), rutin (11)Citation100 alpha-hederin (α-HN) (12).Citation109](/cms/asset/44f7e999-2a3f-4086-9056-adc075f769ff/dddt_a_12303131_f0010_c.jpg)
Bioactivity of Extracts and Compounds of Nigella sativa Linn
Bioactivity of Nigella sativa Linn Extracts and Compounds
The content of compounds in extracts, oils and pure isolates from N. sativa seeds has been widely known and applied.Citation106 As in the Middle East, Europe and Asia, traditional and functional applications of N. sativa seeds are very promising drugs against various diseases.Citation107 Development of compounds in N. sativa seeds continues to be carried out to increase activity.Citation108 N. sativa bioactivities from different countries are presented in .
Table 1 Bioactivity of N. sativa from Different Countries
Antibacterial Activity of Oral Pathogens of Nigella sativa Linn
The antibacterial activity of N. sativa seeds against oral pathogenic bacteria is very good.Citation121 Especially the bacteria that cause caries extract and compound showed very positive activity.Citation105,Citation110,Citation111,Citation122 N. sativa extracts have a range of MIC and isolates have a range of MIC of 0.2–2048 mg/mL, while the isolate has a range of MIC at 8–16 mg/mL.Citation123 The excellent MIC ranges are at 1–8 mg/mL.Citation112 The data showed that isolates from N. sativa had better activity than extracts against bacteria that cause biofilms. This is a reference that N. sativa isolates have great potential as an alternative to natural anti-biofilms (presented in ).
Table 2 Anti-Biofilm of N. Sativa Seed
Conclusion
N. sativa seeds contain secondary metabolites such as essential oils, alkaloids, terpenoids, polyphenols and steroids. Extracts and isolates of N. sativa seeds from various countries have anticancer, antioxidant, antibacterial and anti-inflammatory activities that have been tested in vitro. On biofilm-causing bacteria, N. sativa has excellent activity. Through this article, it can provide information that N. sativa can be used as a potential drug candidate as an anti-biofilm and antibacterial oral pathogen that causes dental caries.
Author Contributions
All authors made a significant contribution to the work reported, whether that is in the conception, study design, execution, acquisition of data, analysis and interpretation, or in all these areas; took part in drafting, revising or critically reviewing the article; gave final approval of the version to be published; have agreed on the journal to which the article has been submitted; and agree to be accountable for all aspects of the work.
Disclosure
The authors declare no conflicts of interest.
Acknowledgments
The authors are grateful to Research Grant of Penelitian Fundamental Regular (PDPFR) – Kemenristek Dikti 2023, Academic Leadership Grant Universitas Padjadjaran 2024, and to Universitas Padjadjaran for all research facilities.
References
- Kay JG, Kramer JM, Visser MB. Danger signals in oral cavity-related diseases. J Leukoc Biol. 2019;106(1):193–200. doi:10.1002/JLB.4MIR1118-439R
- Voidarou C, Antoniadou M, Rozos G, et al. An in vitro study of different types of Greek honey as potential natural antimicrobials against dental caries and other oral pathogenic microorganisms. Case study simulation of oral cavity conditions. Appl Sci. 2021;11(14). doi:10.3390/app11146318
- Niu Y, Wang K, Zheng S, et al. Antibacterial effect of caffeic acid phenethyl ester on cariogenic bacteria and streptococcus mutans biofilms. Antimicrob Agents Chemother. 2020;64(9). doi:10.1128/AAC.00251-20
- Javed S, Zakirulla M, Baig RU, Asif SM, Meer AB. Development of artificial neural network model for prediction of post-streptococcus mutans in dental caries. Comput Methods Programs Biomed. 2020;186. doi:10.1016/j.cmpb.2019.105198
- Cheng L, Zhang L, Yue L, et al. Expert consensus on dental caries management. Int J Oral Sci. 2022;14(1). doi:10.1038/s41368-022-00167-3
- Pachaiappan R, Nagasathiya K, Singh PK, et al. Phytochemical profile of black cumin (Nigella sativa L.) seed oil: identification of bioactive anti-pathogenic compounds for traditional Siddha formulation. Biomass Convers Biorefin. 2022. doi:10.1007/s13399-022-02951-x
- Hannan MA, Rahman MA, Sohag AAM, et al. Black cumin (Nigella sativa l.): a comprehensive review on phytochemistry, health benefits, molecular pharmacology, and safety. Nutrients. 2021;13(6):1784. doi:10.3390/nu13061784
- Biswas A, Ahmed T, Rana MR, et al. Fabrication and Characterization of ZnO nanoparticles-based biocomposite films prepared using carboxymethyl cellulose, taro mucilage, and black cumin seed oil for evaluation of antioxidant and antimicrobial activities. Agronomy. 2023;13(1):147. doi:10.3390/agronomy13010147
- Adegbeye MJ, Elghandour MMMY, Faniyi TO, et al. Antimicrobial and antihelminthic impacts of black cumin, pawpaw and mustard seeds in livestock production and health. Agrofor Syst. 2020;94(4):1255–1268. doi:10.1007/s10457-018-0337-0
- Kolayli S, Kazaz G, Özkök A, et al. The phenolic composition, aroma compounds, physicochemical and antimicrobial properties of Nigella sativa L. (black cumin) honey. Eur Food Res Technol. 2023;249(3):653–664. doi:10.1007/s00217-022-04160-2
- Al-Khalifa KS, Alsheikh R, Al-Hariri MT, et al. Evaluation of the antimicrobial effect of thymoquinone against different dental pathogens: an in vitro study. Molecules. 2021;26(21):6451. doi:10.3390/molecules26216451
- Aqawi M, Sionov RV, Gallily R, Friedman M, Steinberg D. Anti-Bacterial Properties of Cannabigerol Toward Streptococcus mutans. Front Microbiol. 2021;12. doi:10.3389/fmicb.2021.656471
- Bedoya-Correa CM, Rincón Rodríguez RJ, Parada-Sanchez MT. Genomic and phenotypic diversity of Streptococcus mutans. J Oral Biosci. 2019;61(1):22–31. doi:10.1016/j.job.2018.11.001
- Zhang Q, Ma Q, Wang Y, Wu H, Zou J. Molecular mechanisms of inhibiting glucosyltransferases for biofilm formation in Streptococcus mutans. Int J Oral Sci. 2021;13(1). doi:10.1038/s41368-021-00137-1
- Lemos JA, Palmer SR, Zeng L, et al. The Biology of Streptococcus mutans. Microbiol Spectr. 2019;7(1). doi:10.1128/microbiolspec.gpp3-0051-2018
- Barma MD, Muthupandiyan I, Samuel SR, Amaechi BT. Inhibition of Streptococcus mutans, antioxidant property and cytotoxicity of novel nano-zinc oxide varnish. Arch Oral Biol. 2021;126. doi:10.1016/j.archoralbio.2021.105132
- Bin C, Al-Dhabi NA, Esmail GA, Arokiyaraj S, Arasu MV. Potential effect of Allium sativum bulb for the treatment of biofilm forming clinical pathogens recovered from periodontal and dental caries. Saudi J Biol Sci. 2020;27(6):1428–1434. doi:10.1016/j.sjbs.2020.03.025
- Lin Y, Chen J, Zhou X, Li Y. Inhibition of Streptococcus mutans biofilm formation by strategies targeting the metabolism of exopolysaccharides. Crit Rev Microbiol. 2021;47(5):667–677. doi:10.1080/1040841X.2021.1915959
- Barran-Berdon AL, Ocampo S, Haider M, et al. Enhanced purification coupled with biophysical analyses shows cross-β structure as a core building block for Streptococcus mutans functional amyloids. Sci Rep. 2020;10(1). doi:10.1038/s41598-020-62115-7
- Chen D, Cao Y, Yu L, et al. Characteristics and influencing factors of amyloid fibers in S. mutans biofilm. AMB Express. 2019;9(1). doi:10.1186/s13568-019-0753-1
- Iversen KH, Rasmussen LH, Al-Nakeeb K, et al. Similar genomic patterns of clinical infective endocarditis and oral isolates of Streptococcus sanguinis and Streptococcus gordonii. Sci Rep. 2020;10(1). doi:10.1038/s41598-020-59549-4
- Treerat P, Redanz U, Redanz S, Giacaman RA, Merritt J, Kreth J. Synergism between Corynebacterium and Streptococcus sanguinis reveals new interactions between oral commensals. Isme J. 2020;14(5):1154–1169. doi:10.1038/s41396-020-0598-2
- Redanz U, Redanz S, Treerat P, et al. Differential response of oral mucosal and gingival cells to Corynebacterium durum, Streptococcus sanguinis, and Porphyromonas gingivalis multispecies biofilms. Front Cell Infect Microbiol. 2021:11. doi:10.3389/fcimb.2021.686479
- Díaz-Garrido N, Lozano CP, Kreth J, Giacaman RA. Extended biofilm formation time by Streptococcus sanguinis modifies its non-cariogenic behavior, in vitro. Braz Oral Res. 2022;36:e107. doi:10.1590/1807-3107bor-2022.vol36.0107
- Lyu X, Wang L, Shui Y, et al. Ursolic acid inhibits multi-species biofilms developed by Streptococcus mutans, Streptococcus sanguinis, and Streptococcus gordonii. Arch Oral Biol. 2021:125. doi:10.1016/j.archoralbio.2021.105107
- Cui G, Li P, Wu R, Lin H. Streptococcus mutans membrane vesicles inhibit the biofilm formation of Streptococcus gordonii and Streptococcus sanguinis. AMB Express. 2022;12(1). doi:10.1186/s13568-022-01499-3
- Martini AM, Moricz BS, Ripperger AK, et al. Association of novel streptococcus sanguinis virulence factors with pathogenesis in a native valve infective endocarditis model. Front Microbiol. 2020:11. doi:10.3389/fmicb.2020.00010
- Martini AM, Moricz BS, Woods LJ, Jones BD. Type IV Pili of Streptococcus sanguinis Contribute to Pathogenesis in Experimental Infective Endocarditis. Microbiol Spectr. 2021;9(3). doi:10.1128/spectrum.01752-21
- Li X, Bosch-Tijhof CJ, Wei X, et al. Efficiency of chemical versus mechanical disruption methods of DNA extraction for the identification of oral Gram-positive and Gram-negative bacteria. J Int Med Res. 2020;48(5). doi:10.1177/0300060520925594
- Belibasakis GN, Maula T, Bao K, et al. Virulence and pathogenicity properties of Aggregatibacter actinomycetemcomitans. Pathogens. 2019;8(4). doi:10.3390/pathogens8040222
- Danforth DR, Melloni M, Tristano J, Mintz KP. Contribution of adhesion proteins to Aggregatibacter actinomycetemcomitans biofilm formation. Mol Oral Microbiol. 2021;36(4):243–253. doi:10.1111/omi.12346
- Lindholm M, Min Aung K, Nyunt Wai S, Oscarsson J. Role of OmpA1 and OmpA2 in Aggregatibacter actinomycetemcomitans and Aggregatibacter aphrophilus serum resistance. J Oral Microbiol. 2019;11(1). doi:10.1080/20002297.2018.1536192
- Hutchings M, Truman A, Wilkinson B. Antibiotics: past, present and future. Curr Opin Microbiol. 2019;51:72–80. doi:10.1016/j.mib.2019.10.008
- Butler MS, Paterson DL. Antibiotics in the clinical pipeline in October 2019. J Antibiotics. 2020;73(6):329–364. doi:10.1038/s41429-020-0291-8
- Danner MC, Robertson A, Behrends V, Reiss J. Antibiotic pollution in surface fresh waters: occurrence and effects. Science of the Total Environment. 2019;664:793–804. doi:10.1016/j.scitotenv.2019.01.406
- Ganapathy US, Dartois V, Dick T. Repositioning rifamycins for Mycobacterium abscessus lung disease. Expert Opin Drug Discov. 2019;14(9):867–878. doi:10.1080/17460441.2019.1629414
- Adams RA, Leon G, Miller NM, et al. Rifamycin antibiotics and the mechanisms of their failure. J Antibiotics. 2021;74(11):786–798. doi:10.1038/s41429-021-00462-x
- Sanchez CJ, Shiels SM, Tennent DJ, Hardy SK, Murray CK, Wenke JC. Rifamycin derivatives are effective against staphylococcal biofilms in vitro and elutable from PMMA. Clin Orthop Relat Res. 2015;473(9):2874–2884. doi:10.1007/s11999-015-4300-3
- Huttner A, Bielicki J, Clements MN, et al. Oral amoxicillin and amoxicillin–clavulanic acid: properties, indications and usage. Clin Microbiol Infect. 2020;26(7):871–879. doi:10.1016/j.cmi.2019.11.028
- Araújo Júnior AG, Costa ML, Silva FRP, et al. Amoxicillin-resistant streptococci carriage in the mouths of children: a systematic review and meta-analysis. Pathogens. 2022;11(10):1114. doi:10.3390/pathogens11101114
- Rebitski EP, Souza GP, Santana SAA, Pergher SBC, Alcântara ACS. Bionanocomposites based on cationic and anionic layered clays as controlled release devices of amoxicillin. Appl Clay Sci. 2019;173:35–45. doi:10.1016/j.clay.2019.02.024
- Brookes ZLS, Belfield LA, Ashworth A, et al. Effects of chlorhexidine mouthwash on the oral microbiome. J Dent. 2021:113. doi:10.1016/j.jdent.2021.103768
- Huang S, Wu M, Li Y, et al. The dlt operon contributes to the resistance to chlorhexidine in Streptococcus mutans. Int J Antimicrob Agents. 2022;59(3):106540. doi:10.1016/j.ijantimicag.2022.106540
- Taghdisi-Kashani A, Gholamshahi A, Fathizadeh H, et al. Antimicrobial activity of children’s toothpaste on the bacteria causing dental caries. Iranian J Med Microbiol. 2022;16(5):399–404. doi:10.30699/ijmm.16.5.399
- Hassan M, Shafique F, Bhutta H, et al. A comparative study to evaluate the effects of antibiotics, plant extracts and fluoride-based toothpaste on the oral pathogens isolated from patients with gum diseases in Pakistan. Braz J Biol. 2023:83. doi:10.1590/1519-6984.242703
- Tang B, Gong T, Zhou X, et al. Deletion of cas3 gene in Streptococcus mutans affects biofilm formation and increases fluoride sensitivity. Arch Oral Biol. 2019;99:190–197. doi:10.1016/j.archoralbio.2019.01.016
- Miyachiro MM, Granato D, Trindade DM, Ebel C, Paes Leme AF, Dessen A. Complex formation between mur enzymes from streptococcus pneumoniae. Biochemistry. 2019;58(30):3314–3324. doi:10.1021/acs.biochem.9b00277
- El Zoeiby A, Sanschagrin F, Levesque RC. Structure and function of the Mur enzymes: development of novel inhibitors. Mol Microbiol. 2003;47(1):1–12. doi:10.1046/j.1365-2958.2003.03289.x
- Li H, Zhou Y, Wang N, Xin Y, Tang L, Ma Y. Identification and Characterization of a MurA, UDP-N-acetylglucosamine enolpyruvyl transferase from cariogenic streptococcus mutans; 2012.
- Chabán MF, Hrast M, Frlan R, Graikioti DG, Athanassopoulos CM, Carpinella MC. Inhibition of mura enzyme from Escherichia coli and Staphylococcus aureus by diterpenes from lepechinia meyenii and their synthetic analogs. Antibiotics. 2021;10(12). doi:10.3390/antibiotics10121535
- Hrast M, Frlan R, Knez D, Zdovc I, Barreteau H, Gobec S. Mur ligases inhibitors with azastilbene scaffold: expanding the structure–activity relationship. Bioorg Med Chem Lett. 2021;40. doi:10.1016/j.bmcl.2021.127966
- Dias MC, Pinto DC, Silva AMS. Plant flavonoids: chemical characteristics and biological activity. Molecules. 2021;26(17). doi:10.3390/molecules26175377
- Donadio G, Mensitieri F, Santoro V, et al. Interactions with microbial proteins driving the antibacterial activity of flavonoids. Pharmaceutics. 2021;13(5):660. doi:10.3390/pharmaceutics13050660
- Gutiérrez-Venegas G, Gómez-Mora JA, Meraz-Rodríguez MA, Flores-Sánchez MA, Ortiz-Miranda LF. Effect of flavonoids on antimicrobial activity of microorganisms present in dental plaque. Heliyon. 2019;5(12):e03013. doi:10.1016/j.heliyon.2019.e03013
- Socorro Chagas MS D, Behrens MD, Moragas-Tellis CJ, Penedo GXM, Silva AR, Gonçalves-de-albuquerque CF. Flavonols and flavones as potential anti-inflammatory, antioxidant, and antibacterial compounds. Oxid Med Cell Longev. 2022;2022. doi:10.1155/2022/9966750
- Liang J, Huang X, Ma G. Antimicrobial activities and mechanisms of extract and components of herbs in East Asia. RSC Adv. 2022;12(45):29197–29213. doi:10.1039/d2ra02389j
- Machorowska-Pieniążek A, Morawiec T, Olek M, et al. Advantages of using toothpaste containing propolis and plant oils for gingivitis prevention and oral cavity hygiene in cleft lip/palate patients. Biomed Pharmacother. 2021:142. doi:10.1016/j.biopha.2021.111992
- Milutinovici RA, Chioran D, Buzatu R, et al. Vegetal compounds as sources of prophylactic and therapeutic agents in dentistry. Plants. 2021;10(10):2148. doi:10.3390/plants10102148
- Ito Y, Ito T, Yamashiro K, et al. Antimicrobial and antibiofilm effects of abietic acid on cariogenic Streptococcus mutans. Odontology. 2020;108(1):57–65. doi:10.1007/s10266-019-00456-0
- Moraes da TS, Leandro LF, Santiago MB, et al. Assessment of the antibacterial, antivirulence, and action mechanism of Copaifera pubiflora oleoresin and isolated compounds against oral bacteria. Biomed Pharmacother. 2020:129. doi:10.1016/j.biopha.2020.110467
- Bhagavathy S, Mahendiran C, Kanchana R. Identification of glucosyl transferase inhibitors from Psidium guajava against Streptococcus mutans in dental caries. J Tradit Complement Med. 2019;9(2):124–137. doi:10.1016/j.jtcme.2017.09.003
- Egra S, Kuspradini H, Kusuma IW, Batubara I, Yamauchi K, Mitsunaga T. Garcidepsidone B from Garcinia parvifolia: antimicrobial activities of the medicinal plants from East and North Kalimantan against dental caries and periodontal disease pathogen. Med Chem Res. 2023;32(8):1658–1665. doi:10.1007/s00044-023-03071-5
- Putri Adhiningtyas A, Khoswanto C, Luthfi M. Inhibitory potency of butterfly pea (Clitoria ternatea Linn.) extract against the growth of Streptococcus mutans. World J Advan Res Rev. 2023;17(1):150–156. doi:10.30574/wjarr.2023.17.1.1474
- Barrera C, Betoret N, Seguí L. Phenolic profile of cane sugar derivatives exhibiting antioxidant and antibacterial properties. Sugar Tech. 2020;22(5):798–811. doi:10.1007/s12355-020-00817-y
- Pavlović T, Dimkić I, Andrić S, et al. Linden tea from Serbia – an insight into the phenolic profile, radical scavenging and antimicrobial activities. Ind Crops Prod. 2020:154. doi:10.1016/j.indcrop.2020.112639
- Ochoa C, Solinski AE, Nowlan M, Dekarske MM, Wuest WM, Kozlowski MC. A bisphenolic honokiol analog outcompetes oral antimicrobial agent cetylpyridinium chloride via a membrane-associated mechanism. ACS Infect Dis. 2020;6(1):74–79. doi:10.1021/acsinfecdis.9b00190
- Domínguez-Avila JA, Villa-Rodriguez JA, Montiel-Herrera M, et al. Phenolic compounds promote diversity of gut microbiota and maintain colonic health. Dig Dis Sci. 2021;66(10):3270–3289. doi:10.1007/s10620-020-06676-7
- Efenberger-Szmechtyk M, Nowak A, Czyzowska A. Plant extracts rich in polyphenols: antibacterial agents and natural preservatives for meat and meat products. Crit Rev Food Sci Nutr. 2021;61(1):149–178. doi:10.1080/10408398.2020.1722060
- Jolly A, Kim H, Moon JY, Mohan A, Lee YC. Exploring the imminent trends of saponins in personal care product development: a review. Ind Crops Prod. 2023;205:117489. doi:10.1016/j.indcrop.2023.117489
- Ayyanaar S, Kesavan MP. One-pot biogenic synthesis of gold nanoparticles@saponins niosomes: sustainable nanomedicine for antibacterial, anti-inflammatory and anticancer therapeutics. Colloids Surf a Physicochem Eng Asp. 2023;676:132229. doi:10.1016/j.colsurfa.2023.132229
- Sun X, Yang X, Xue P, Zhang Z, Ren G. Improved antibacterial effects of alkali-transformed saponin from quinoa husks against halitosis-related bacteria. BMC Complement Altern Med. 2019;19(1). doi:10.1186/s12906-019-2455-2
- Schestakow A, Hannig M. Effects of experimental agents containing Tannic acid or Chitosan on the bacterial biofilm formation in situ. Biomolecules. 2020;10(9):1–17. doi:10.3390/biom10091315
- Farha AK, Yang QQ, Kim G, et al. Tannins as an alternative to antibiotics. Food Biosci. 2020:38. doi:10.1016/j.fbio.2020.100751
- Schestakow A, Guth MS, Eisenmenger TA, Hannig M. Evaluation of anti-biofilm activity of mouthrinses containing tannic acid or chitosan on dentin in situ. Molecules. 2021;26(5):1351. doi:10.3390/molecules26051351
- Yagoub SO. Chapter four - Black cumin: morphology, physiology, growth, and agricultural yield. In: Mariod AA editor. Biochemistry, Nutrition, and Therapeutics of Black Cumin Seed. Academic Press; 2023:19–25. doi:10.1016/B978-0-323-90788-0.00016-0
- Burdock GA. Assessment of black cumin (Nigella sativa L.) as a food ingredient and putative therapeutic agent. Regul Toxicol Pharmacol. 2022;128:105088. doi:10.1016/j.yrtph.2021.105088
- Islam MN, Hossain KS, Sarker PP, et al. Revisiting pharmacological potentials of Nigella sativa seed: a promising option for COVID-19 prevention and cure. Phytother Res. 2021;35(3):1329–1344. doi:10.1002/ptr.6895
- Nyemb JN, Shaheen H, Wasef L, Nyamota R, Segueni N, El-Saber Batiha G. Black cumin: a review of its pharmacological effects and its main active constituent. Pharmacogn Rev. 2022;16(32):107–125. doi:10.5530/phrev.2022.16.16
- Yimer EM, Tuem KB, Karim A, Ur-Rehman N, Anwar F, Nigella Sativa L. (Black Cumin): a promising natural remedy for wide range of illnesses. Evid Based Complement Alternat Med. 2019;2019:1–16. doi:10.1155/2019/1528635
- Padhye S, Banerjee S, Ahmad A, Mohammad R, Sarkar FH. From here to eternity-the secret of pharaohs: therapeutic potential of black cumin seeds and Beyond. Cancer Therapy. 2008;6(b):495.
- Yuan G, Guan Y, Yi H, Lai S, Sun Y, Cao S. Antibacterial activity and mechanism of plant flavonoids to gram-positive bacteria predicted from their lipophilicities. Sci Rep. 2021;11(1). doi:10.1038/s41598-021-90035-7
- Kabir Y, Akasaka-Hashimoto Y, Kubota K, Komai M. Volatile compounds of black cumin (Nigella sativa L.) seeds cultivated in Bangladesh and India. Heliyon. 2020;6(10):e05343. doi:10.1016/j.heliyon.2020.e05343
- Albakry Z, Karrar E, Mohamed Ahmed IA, et al. Nutritional composition and volatile compounds of black cumin (Nigella sativa L.) seed, fatty acid composition and tocopherols, polyphenols, and antioxidant activity of its essential oil. Horticulturae. 2022;8(7). doi:10.3390/horticulturae8070575
- Dey BK, Hossain MMM, Alam ME. Effect of black cumin seed oil on growth, innate immunity and resistance against Pseudomonas fluorescens infection in Nile tilapia Oreochromis niloticus. Aquacult Int. 2020;28(4):1485–1499. doi:10.1007/s10499-020-00539-8
- Habib N, Choudhry S. HPLC quantification of thymoquinone extracted from nigella sativa L. (Ranunculaceae) seeds and antibacterial activity of its extracts against bacillus species. Evid Based Complement Alternat Med. 2021;2021. doi:10.1155/2021/6645680
- Kiari FZ, Meddah B, Tir Touil Meddah A. In vitro study on the activity of essential oil and methanolic extract from Algerian Nigella sativa L. Seeds on the growth kinetics of micro-organisms isolated from the buccal cavities of periodontal patients. Saudi Dent J. 2018;30(4):312–323. doi:10.1016/j.sdentj.2018.05.011
- Kazemi M. Phytochemical Composition, Antioxidant, Anti-inflammatory and Antimicrobial Activity of Nigella sativa L. Essential Oil. J Essent Oil Bear Plants. 2014;17(5):1002–1011. doi:10.1080/0972060X.2014.914857
- Baaliouamer A, Benkaci-Ali F, Meklati BY, Chemat F. Chemical composition of seed essential oils from Algerian Nigella sativa extracted by microwave and hydrodistillation. J Basel. 2007;22:148–153. doi:10.1002/ffj
- Mechraoui O, Ladjel S, Said Nedjimi M, Lakhdar Belfar M, Moussaoui Y. Determination of polyphenols content, antioxidant and antibacterial activity of nigella sativa L. Seed PhenExtrac. 2018;19:1.
- Enomoto S, Asano R, Iwahori Y, et al. Hematological Studies on Black Cumin Oil from the Seeds of Nigella sativa L. Pharmac Soc Japan. 2001;24(3):307–310.
- Akram Khan M. Chemical composition and medicinal properties of nigella sativa linn; 1999.
- Bourgou S, Pichette A, Lavoie S, Marzouk B, Legault J. Terpenoids isolated from Tunisian Nigella sativa L. essential oil with antioxidant activity and the ability to inhibit nitric oxide production. Flavour Fragr J. 2012;27(1):69–74. doi:10.1002/ffj.2085
- Liu X, El-Aty AM A, Shim JH. Various extraction and analytical techniques for isolation and identification of secondary metabolites from nigella sativa seeds. Mini Reviews in Med Chem. 2011;11:947–955. doi:10.2174/138955711797068472
- Salem MA, El-Shiekh RA, Aborehab NM, et al. Metabolomics driven analysis of Nigella sativa seeds identifies the impact of roasting on the chemical composition and immunomodulatory activity. Food Chem. 2023;398:133906. doi:10.1016/j.foodchem.2022.133906
- Akram Khan M, Afzal M. Chemical composition of Nigella sativa Linn: part 2 Recent advances. Inflammopharmacology. 2016;24(2–3):67–79. doi:10.1007/s10787-016-0262-7
- Mehta BK, Sharma U, Agrawal S, Pandit V, Joshi N, Gupta M. Isolation and characterization of new compounds from seeds of Nigella sativa. Med Chem Res. 2008;17:462–473. doi:10.1007/s00044-007-9080-1
- Imran M, Khan SA. Nigella sativa L. and COVID-19: a Glance at The Anti-COVID-19 chemical constituents, clinical trials, inventions, and patent literature. Molecules. 2022;27(9):2750. doi:10.3390/molecules27092750
- Ali BH, Blunden G. Pharmacological and toxicological properties of Nigella sativa. Phytother Res. 2003;17(4):299–305. doi:10.1002/ptr.1309
- Veeramani S, Narayanan AP, Yuvaraj K, et al. Nigella sativa flavonoids surface coated gold NPs (Au-NPs) enhancing antioxidant and anti-diabetic activity. Process Biochem. 2022;114:193–202. doi:10.1016/j.procbio.2021.01.004
- Parveen A, Farooq MA, Kyunn WW. A new oleanane type saponin from the aerial parts of nigella sativa with anti-oxidant and anti-diabetic potential. Molecules. 2020;25(9):2171. doi:10.3390/molecules25092171
- Zafar I, Safder A, Imran Afridi H, et al. In silico and in vitro study of bioactive compounds of Nigella sativa for targeting neuropilins in breast cancer. Front Chem. 2023:11. doi:10.3389/fchem.2023.1273149
- Rathi B, Devanesan S, AlSalhi MS, Ranjith Singh AJ. In-vitro free radical scavenging effect and cytotoxic analysis of Black Cummins and Honey formulation. Saudi J Biol Sci. 2021;28(3):1576–1581. doi:10.1016/j.sjbs.2020.12.051
- Yuan T, Nahar P, Sharma M, et al. Indazole-type alkaloids from nigella sativa seeds exhibit antihyperglycemic effects via AMPK activation in vitro. J Nat Prod. 2014;77(10):2316–2320. doi:10.1021/np500398m
- Mehta BK, Mehta P, Gupta M. A new naturally acetylated triterpene saponin from Nigella sativa. Carbohydr Res. 2009;344(1):149–151. doi:10.1016/j.carres.2008.10.004
- Karaman K. Characterization of Saccharomyces cerevisiae based microcarriers for encapsulation of black cumin seed oil: stability of thymoquinone and bioactive properties. Food Chem. 2020;313. doi:10.1016/j.foodchem.2019.126129
- Almatroudi A, Khadri H, Azam M, et al. Antibacterial, antibiofilm and anticancer activity of biologically synthesized silver nanoparticles using seed extract of Nigella sativa. Processes. 2020;8(4):388. doi:10.3390/PR8040388
- Salehi B, Quispe C, Imran M, et al. Nigella plants – traditional uses, bioactive phytoconstituents, preclinical and clinical studies. Front Pharmacol. 2021:12. doi:10.3389/fphar.2021.625386
- Lin J, Gulbagca F, Aygun A, et al. Phyto-mediated synthesis of nanoparticles and their applications on hydrogen generation on NaBH4, biological activities and photodegradation on azo dyes: development of machine learning model. Food and Chemical Toxicology. 2022;163:112972. doi:10.1016/j.fct.2022.112972
- Adamska A, Stefanowicz-Hajduk J, Renata Ochocka J. Alpha-hederin, the active saponin of nigella sativa, as an anticancer agent inducing apoptosis in the SKOV-3 cell line. Molecules. 2019;24(16):2958. doi:10.3390/molecules24162958
- Bourgou S, Pichette A, Marzouk B, Legault J. Bioactivities of black cumin essential oil and its main terpenes from Tunisia. S Afr J Bot. 2010;76(2):210–216. doi:10.1016/j.sajb.2009.10.009
- Bourgou S, Rebey IB, Ben KS, et al. Green solvent to substitute hexane for bioactive lipids extraction from black cumin and basil seeds. Foods. 2021;10(7):1493. doi:10.3390/foods10071493
- Hameed S, Imran A, Nisa M, et al. Characterization of extracted phenolics from black cumin (Nigella sativa linn), coriander seed (Coriandrum sativum L.), and fenugreek seed (Trigonella foenum-graecum). Int J Food Prop. 2019;22(1):714–726. doi:10.1080/10942912.2019.1599390
- Soleimanifar M, Niazmand R, Jafari SM. Evaluation of oxidative stability, fatty acid profile, and antioxidant properties of black cumin seed oil and extract. J Food Meas Characteriz. 2019;13(1):383–389. doi:10.1007/s11694-018-9953-7
- Shaheed KA, Alsirraj MA, Allaith SA, et al. The biological activities of seeds extracts for fenugreek and black cumin and its inhibitory influences toward some pathogens; 2018. Available from: https://pubchem.ncbi.nlm.nih.gov/com-. Accessed May 06, 2024.
- Dalli M, Azizi SE, Kandsi F, Gseyra N. Evaluation of the in vitro antioxidant activity of different extracts of Nigella sativa L. seeds, and the quantification of their bioactive compounds. In: Materials Today: Proceedings; 2021:7259–7263. doi:10.1016/j.matpr.2020.12.743.
- Ramadan MF, Asker MMS, Tadros M. Antiradical and antimicrobial properties of cold-pressed black cumin and cumin oils. Eur Food Res Technol. 2012;234(5):833–844. doi:10.1007/s00217-012-1696-9
- Feng Y, Dunshea FR, Suleria HAR. LC-ESI-QTOF/MS characterization of bioactive compounds from black spices and their potential antioxidant activities. J Food Sci Technol. 2020;57(12):4671–4687. doi:10.1007/s13197-020-04504-4
- Albakry Z, Karrar E, Mohamed Ahmed IA, et al. A comparative study of black cumin seed (Nigella sativa L.) oils extracted with supercritical fluids and conventional extraction methods. J Food Meas Characteriz. 2023;17(3):2429–2441. doi:10.1007/s11694-022-01802-7
- Ahlina FN, Anggriani L, Salsabila IA, Jenie RI. Bioactivity of black cumin oil on the senescence of her-2-overexpressing breast cancer cells. Malays App Bio. 2022;51(1):91–98. doi:10.55230/MABJOURNAL.V51I1.2008
- Sutton KM, Greenshields AL, Hoskin DW. Thymoquinone, a bioactive component of black caraway seeds, causes G1 phase cell cycle arrest and apoptosis in triple-negative breast cancer cells with mutant p53. Nutr Cancer. 2014;66(3):408–418. doi:10.1080/01635581.2013.878739
- Besra M, Kumar V. In vitro investigation of antimicrobial activities of ethnomedicinal plants against dental caries pathogens. Biotech. 2018;8(5). doi:10.1007/s13205-018-1283-2
- Qureshi KA, Imtiaz M, Parvez A, et al. In vitro and in silico approaches for the evaluation of antimicrobial activity, time-kill kinetics, and anti-biofilm potential of thymoquinone (2-methyl-5-propan-2-ylcyclohexa-2, 5-diene-1,4-dione) against selected human pathogens. Antibiotics. 2022;11(1):79. doi:10.3390/antibiotics11010079
- Kokoska J, Vadlejch JLF, Vadlejch JLF, Vadlejch J. The growth-inhibitory effect of thymohydroquinone and thymoquinone on oral pathogenic bacteria in vitro. Planta Med. 2009;75(09):PJ116. doi:10.1055/s-0029-1234921
- Rusmarilin H, Lubis Z, Lubis LM, Barutu YAP. Potential of natural antioxidants of black cumin seed (Nigella sativa) and sesame seed (Sesamum indicum) extract by microencapsulation methods. In: IOP Conference Series: Earth and Environmental Science. Vol 260. Institute of Physics Publishing; 2019. doi:10.1088/1755-1315/260/1/012097.
- Oubannin S, Bijla L, Gagour J, et al. A comparative evaluation of proximate composition, elemental profiling and oil physicochemical properties of black cumin (Nigella sativa L.) seeds and argan (Argania spinosa L. Skeels) kernels. Chem Data Collect. 2022;41:100920. doi:10.1016/j.cdc.2022.100920
- Alamoudi RA, Alamoudi SA, Alamoudi RA. Biological potential of the main component, thymoquinone, of nigella sativa in pulp therapy—in vitro study. Life. 2022;12(9):1434. doi:10.3390/life12091434
- Shafodino FS, Lusilao JM, Mwapagha LM. Phytochemical characterization and antimicrobial activity of Nigella sativa seeds. PLoS One. 2022;17(8):e0272457. doi:10.1371/journal.pone.0272457
- Shabestari BN. The comparison of antimicrobial effect of nigella sativa nanoparticle and chlorhexidine emulsion on the most common dental cariogenicic bacteria; 2021.
- Tiwari AK, Jha S, Singh AK, et al. Innovative investigation of zinc oxide nanoparticles used in dentistry. Crystals. 2022;12(8). doi:10.3390/cryst12081063
- Rashid M, Ganaie MA, Khan S, et al. Comparative antibacterial study of black cumin oil of Saudi and Syrian origin seeds with the commercial product. J Rep Pharm Sci. 2021;10(1):148–152. doi:10.4103/jrptps.JRPTPS_118_20
- Othman L, Sleiman A, Abdel-Massih RM. Antimicrobial activity of polyphenols and alkaloids in Middle Eastern plants. Front Microbiol. 2019;10(MAY). doi:10.3389/fmicb.2019.00911
- Bourgou S, Pichette A, Marzouk B, Legault J. Antioxidant, anti-inflammatory, anticancer and antibacterial activities of extracts from nigella sativa (black cumin) plant parts. J Food Biochem. 2012;36(5):539–546. doi:10.1111/j.1745-4514.2011.00567.x
- Rostinawati T, Karipaya S, Iskandar Y. Antibacterial activity of ethanol extract of nigella Sativa L. seed against streptococcus mutans. In: IOP Conference Series: Earth and Environmental Science. Institute of Physics Publishing; 2019. doi:10.1088/1755-1315/334/1/012050.