Abstract
Purpose
Through network pharmacology combined with molecular docking and in vivo validation, the study examines the unexplored molecular mechanisms of Tongxieyaofang (TXYF) in the treatment of irritable bowel syndrome (IBS). In particular, the potential pharmacological mechanism of TXYF alleviating IBS by regulating CHRM3 and intestinal barrier has not been studied.
Patients and Methods
LC-MS technique and TCMSP database were used in combination to identify the potential effective components and target sites of TXYF. Potential targets for IBS were obtained from Genecards and OMIM databases. PPI and cytoHub analysis for targets. Molecular docking was used to validate the binding energy of effective components with related targets and for visualization. GO and KEGG analysis were employed to identify target functions and signaling pathways. In the in vivo validation, wrap restraint stress-induced IBS model was employed to verify the change for cytoHub genes and CHRM3 expression. Furthermore, inflammatory changes of colon were observed by HE staining. The changes of Ach were verified by ELISA. IHC and WB validated CHRM3 and GNAQ/PLC/MLCK channel variations. AB-PAS test and WB test confirmed the protection of TXYF on gut barrier. The NF-κB/MLCK pathway was also verified.
Results
In TXYF decoction, LC-MS identified 559 chemical components, with 23 remaining effective components after screening in TCMSP. KEGG analysis indicated that calcium plays a crucial role in TXYF treated for IBS. Molecular docking validated the binding capacity of the effective components Naringenin and Nobiletin with cytoHub-gene and CHRM3. In vivo validation demonstrated that TXYF inhibits the activation of Ach and CHRM3 in IBS, and inhibits for the GNAQ/PLC/MLCK axis. Additionally, TXYF downregulates TNF-α, MMP9, and NF-κB/MLCK, while modulating goblet cell secretion to protect gut barrier.
Conclusion
TXYF inhibits Ach and CHRM3 expression, regulating the relaxation of intestinal smooth muscle via GNAQ/PLC/MLCK. Additionally, TXYF inhibits NF-κB/MLCK activated and goblet cell secretion to protect gut barrier.
Introduction
Irritable bowel syndrome (IBS) is a complex and diverse chronic digestive system disorder characterized by abdominal pain and accompanying intestinal dysfunction. IBS affects 9% to 23% of the global population.Citation1 The latest Rome classification divides IBS into four subtypes: IBS with constipation (IBS-C), IBS with diarrhea (IBS-D), IBS mixed type with both constipation and diarrhea, and unclassified IBS.Citation2,Citation3 A global meta-analysis showed that the subtypes of IBS-D, IBS-C, IBS-M and IBS-U accounted for 23.4%, 22.0%, 24.0% and 22.2%, respectively, in patients with IBS. According to the Chinese research report, among IBS patients, IBS-D, IBS-C and IBS-M accounted for 74.1%, 15.1% and 10.8% respectively. Among these, IBS-D is the most clinically common sub-type and significantly impacts patients’ daily lives. The pathophysiology of IBS is not fully understood, and there is a lack of highly effective clinical treatments for IBS.Citation4 Therefore, there is an urgent need to research the molecular mechanisms of IBS to provide more evidence for clinical treatment.
Traditional Chinese Medicine (TCM) treatment of IBS has the characteristics of high safety and strong efficacy, and its therapeutic potential should not be overlooked. In terms of its mechanism, TCM exhibits the characteristic of addressing diseases at multiple levels and targets. However, this also makes the mechanism of action of the drugs more complex. The classic TCM formula Tongxieyaofang (TXYF) has been used for treating gastrointestinal diseases since ancient medical literature, and is now also used in the clinical treatment of IBS.Citation5 The TXYF formula contains four Chinese herbs: Salvia, Citrus peel, Atractylodes, and Paeonia lactiflora. These herbs contain many potential bioactive components that interact with multiple therapeutic targets. Clinical data suggests that TXYF can alleviate the clinical symptoms of IBS patients.Citation6 In animal experiments, researchers have found that TXYF can alleviate IBS-D symptoms in rats.Citation7 Furthermore, TXYF can alleviate IBS by regulating 5-hydroxytryptamine and substance P.Citation8 Additionally, TXYF can also alleviate IBS by regulating 5-HT levels through intervention in the intestinal flora.Citation9 In microbiota-gut-brain axis studies, TXYF has also been found to have a preventive effect on depressive behavior in mice.Citation10 Although the mechanism of TXYF in the treatment of IBS has been discussed and studied by scholars, there are still unclear aspects. In the pathogenesis of IBS, it is proposed that stimulation of cholinergic receptors (CHRM1 and CHRM3) by acetylcholine causes abnormal intestinal motility.Citation11 In addition, IBS is thought to be associated with low-grade inflammation, and inflammatory stimulation may lead to damage to the intestinal barrier.Citation12 These aspects have not been further studied in the treatment of IBS with TXYF. Therefore, further exploration of the treatment of IBS with TXYF decoction is necessary and can provide more reliable evidence for the clinical application of TXYF decoction.
Network pharmacology is an interdisciplinary approach that combines medical, biological and informatics aspects. It is convenient for comprehensively identifying drug targets and analyzing drug mechanisms of action. This is consistent with the emphasis on “compatibility” and “holistic concept” in traditional Chinese medicine. Network pharmacology can provide potential theoretical support for the clinical application of traditional Chinese medicine prescriptions by uncovering different drug targets and mechanisms of action between diseases and prescriptions.Citation13
CHRM3, TNF-α and MMP9 are core targets identified in the network pharmacology analysis, and they have captured our attention. CHRM3 is a member of the muscarinic acetylcholine receptor, belonging to the G protein-coupled receptor family.Citation14 Acetylcholine can stimulate CHRM3 to promote the coupling of Gq/G11 with CHRM3, activating PLC/MLCK to control smooth muscle contraction. Symptoms of diarrhea or constipation in patients with IBS are associated with abnormal bowel movements.Citation15 CHRM3 can stimulate macrophages to exert a pro-inflammatory effect.Citation16 Inflammatory macrophages can produce TNF-α and MMP9. TNF-α, as a systemic inflammatory cytokine, is believed to be associated with intestinal inflammation. Intestinal inflammation is thought to be related to damage to the gut barrier.Citation17 MMP9 plays an important role in the pathogenesis of intestinal inflammation and can increase intestinal epithelial permeability.Citation18
This study aims to evaluate the potential targets and pathways of TXYF in treating IBS using network pharmacology. Through the integration of resources in network pharmacology, the main effective components of TXYF decoction were selected, and molecular docking and in vivo validation were conducted for the core targets CHRM3, TNF-α, and MMP9. The impact of the Calcium signaling pathway on colonic smooth muscle in IBS, as indicated by the KEGG enrichment results, was also validated. Additionally, the study explores the influence of NF-κB/MLCK on the intestinal barrier. These findings aim to provide a more reliable basis for the clinical application of TXYF decoction in the treatment of IBS.
Materials and Methods
Network Pharmacology of TXYF and IBS
Active Ingredient and Target of TXYF
Search for the component drugs of TXYF (Chenpi, Fangfeng, Baizhu, Baishao) in TCMSP (http://lsp.nwu.edu.cn/tcmsp.php) using the criteria of OB (oral bioavailability) ≥ 30% and DL (drug-likeness) ≥ 0.18 to screen for effective components and their targets. UniProt was used for protein name conversion. The correlation between core effective components and targets was analyzed using Cytoscape software (version 3.7.2). LC-MS was used to conduct mass spectrometry analysis of the TXYF decoction and obtain the effective chemical components of the decoction.
Venn for IBS and TXYF
Enter “Intestinal stress syndrome” into public data websites such as OMIM, GeneCard and TTD, and download the disease targets provided by the websites. Take the intersection of the obtained targets with the targets of TXYF to obtained 90 targets (Group A). In addition, we took the intersection of disease targets obtained from different databases (OMIM and GeneCard) and then intersected them with the targets of TXYF to obtain more precise target objectives, resulting in a singular target, CHRM3.
Protein-Protein Interaction Networks (PPI)
“Group A” data and CHRM3 were separately input into the STRING website for protein-protein interaction (PPI) analysis, with the species set to human and a minimum required interaction score of 0.4. The data was then imported into Cytoscape software for cytoHub and MOCDE analysis.
Gene Ontology (GO) and Kyoto Encyclopedia of Genes and Genomes (KEGG)
GO and KEGG enrichment analysis was conducted to explore the potential signaling pathways of the interaction between TXYF and IBS. Metascape database (https://metascape.org) was used to separately process and conduct enrichment analysis of the common target genes in “Group A” and CHRM3, obtaining their BP (biological processes), CC (cellular components), MF (molecular functions), and KEGG results. With parameters set as P < 0.01, Min Overlap > 3, Min Enrichment > 15. All data was imported into the Hiplot website (https://hiplot.com.cn) for the visualization of GO and KEGG results.
Molecular Docking and Visualization
The most interactive effective components with the targets (top 5) and cytoHub targets or CHRM3 were selected for molecular docking. The receptor protein structure was obtained from the Protein Data Bank (PDB) (https://www1.rcsb.org/), and the 3D structures were optimized using PyMOL software (version 2.3.0). The 3D structure files of the effective components Naringenin, Nobiletin, Kaempferol, Beta-sitosterol, and Wogonin were downloaded from the PubChem database and molecular force field optimization was performed using Chem3D. AutoDock Tools software (version 1.5.6) was utilized for protein optimization. The grid set-up was designed for semi-flexible docking and Lamarckian genetic algorithm. AutoDock Vina (version 1.2.0) was executed for molecular docking to obtain the binding free energy. Naringenin and Nobiletin targets with binding energy scores less than −7.2 kcal·mol-1 were visualized using PyMOL software (version 2.3.0).
Frying and Identification of TXYF
TXYF Decoction
All herbs of TXYF (Saposhnikoviae Radix, Citri Reticulatae Pericarpium, Atractylodis Macrocephalae Rhizoma and Paeoniae Radix Alba) were purchased from the Outpatient Department of Zhejiang Chinese Medical University (Hangzhou, China). Place all the medicinal herbs in water. For each portion of the herbs (Atractylodis Macrocephalae Rhizoma 18g, Paeoniae Radix Alba 12g, Citri Reticulatae Pericarpium 9g and Saposhnikoviae Radix 6g), add 600mL of water. After boiling for 2h, the liquid was filtered. The extract is processed in a vacuum drying oven until no liquid is present. Finally, 15g of TXYF extract powder was obtained. According to the formula: . The results show that the extraction rate is about 33%. All plants name has been checked with http://www.theplantlist.org.
LC-MS
The analysis was conducted using the chromatograph: UltiMate 3000 RS and mass spectrometer: Q Exactive (Thermo Fisher Scientific Co., Ltd). A 200uL sample was added to 1mL of a mixed solution (methanol: water = 8:2) and vortexed. Then, 2–3 zirconium dioxide grinding beads were added and the mixture was vortexed for 3 minutes. The sample was subsequently centrifuged at 4°C (at 20000 × g) for 10 minutes, and the supernatant was filtered through a 0.22μm membrane filter for analysis. The data collected from high-resolution liquid chromatography was initially processed using CD2.1 (Thermo Fisher) and then subjected to database retrieval and comparison (mzCloud, mzVault, ChemSpider).
Mice Zoopery
Ethical Statement
The animal study was approved by the Ethics Committee for Animal Study in Animal Ethical and Welfare Committee of Zhejiang Chinese Medical University (approval number: No. ZSLL-2018–014). This study followed the 3R standard measures of animal welfare: Replacement, Reduction and Refinement. All the data comes from the public data platform Genecards, OMIM and TTD. The research on the public database has been exempted from ethical approval by the Ethics Committee of Zhejiang Hospital of Traditional Chinese Medicine (Acceptance number: 2024-YBK-002-01).
IBS Model
7-week-old male C57BL/6 mice were purchased from the Experimental Animal Center of Zhejiang Chinese Medical University. All mice were divided into 6 cages, with ad libitum access to food and water. The environmental conditions included a temperature of 22±1°C, humidity of 55±10%, low noise, and a 12-hour light-dark cycle. After 7 days of acclimatization, the mice were randomly divided into 3 groups (n = 8/group): control group, IBS group and IBS+TXYF group. IBS model was induced using wrap restraint stress (WRS) method. The IBS group received intragastric administration of PBS (10 mL/kg). The IBS+TXYF group received TXYF decoction. Reference to previous effective dose (11.2 g/kg).Citation19 Intragastric administration and body weight recordings were conducted once daily.
AWR Score Fecal Water Content
At the end of each experiment, each mouse was assessed for visceral sensitivity, according to the methodology outlined in the previous article.Citation20 Stimulation was applied in three different levels of distension (0.25/0.35/0.50 mL). Each distension was repeated three times. The average value of the abdominal withdrawal reflex (AWR) was calculated as the final score for each mouse. The AWR scores were quantified using the method described previously. The specific score is as follows: 0 = no behavioral response to distension; 1 = brief head movements followed by immobility; 2 = contraction of abdominal muscles without lifting of the abdomen; 3 = lifting of the abdomen; 4 = body arching and lifting of the pelvic structure. Fecal water content before and after treatment was measured by placing the feces in a drying oven for 10 hours and then assessing the changes in weight.
Western Blot (WB)
The method was consistent with the previous description. Protein extraction from the mouse colon segment was conducted for WB validation. Additionally, membrane re-probing technique was utilized, where stripping buffer was used to strip the previous antibody for subsequent incubation with a new antibody. The antibodies used were all diluted at a ratio of 1:1000. The antibodies included: anti-TNF-α (#11948), anti-PTGS2 (#4842), anti-CASP3 (#9662), anti-MMP9 (#3852), anti-ESR1 (PAB47907), anti-AKT (MAB51173), anti-BCL2 (#3498), anti-CHRM3 (PAB39793), anti-ZO-1 (PAB36669), anti-Occludin (#91131), anti-MUC2 (PAB39041), anti-GNAQ (WL05054), anti-GNA11 (WL00806), anti-MLCK (PAB35659) and anti-β-Actin (#4967). The antibodies are derived from: Cell Signaling Technologies, Danvers, MA, USA or Bioswamp Life Science Lab, Wuhan, China. Electro chemiluminescence detection reagents, RIPA Lysis Buffer, bicinchoninic acid (BCA) protein assay kit, Western Blot Fast Stripping Buffer, Anti-rabbit IgG and Anti-mouse IgG from Epizyme Biotech Company (Shanghai, China). Protease and Phosphatase Inhibitor Cocktail from Thermo Scientific, USA. Polyvinyl difluoride (PVDF) membranes pore size 0.2 µm from Thermo Scientific, USA. The images were captured with Bio-Rad gel imaging system and analyzed by Quantity One software. The Western blot test can only accommodate 15 samples, so only 4 mice were shown, and 8 mice were included in the statistics.
Enzyme-Linked Immunosorbent Assay (ELISA)
The colonic levels of MLCK (MU31148), PLC (MU307L5) and ACh (MU30372) were quantified using ELISA assay kits strictly following the instructions provided by the supplier Bioswamp Life Science Lab, Wuhan, China. The absorbance at a wavelength of 450nm was measured using Thermo Varioskan Flash (Thermo Scientific, USA). The sample concentrations of MLCK, PLC and ACh were calculated using the equation obtained from a four-parameter logistic curve fit based on the standard curve, into which the OD values were inputted.
Hematoxylin-Eosin (H&E) or AB-PAS Staining
The fixation, embedding, and sectioning of colon tissue were consistent with previous practices.Citation21 HE staining was performed using hematoxylin-eosin stain for 3–5 minutes, followed by differentiation and bluing. Subsequently, dehydration was carried out with 85% and 95% gradient alcohols for 5 minutes each, and then stained in eosin Y solution for 5 minutes. For AB-PAS staining, following the requirements of the reagent kit, staining was performed with solution C, followed by acidification with solution B and final light-protected staining with solution A. The sealing process was similar: the slices were successively placed in absolute ethanol I for 5 minutes, absolute ethanol II for 5 minutes, absolute ethanol III for 5 minutes, xylene I for 5 minutes, xylene II for 5 minutes for transparency, and then sealed with neutral gum.
Immunohistochemistry
The experimental procedure remains consistent with the previous method.Citation21 However, unlike the previous experiment, the antibody used this time is: anti-CHRM3 (1:200, BA1783-1, BOSTER, State of California, USA).
Immunofluorescence
The experimental procedure is consistent with the previous method.Citation21 However, unlike the previous experiment, the antibodies used this time are: anti-ZO-1 (1:50, PAB36669, Bioswamp, China) and anti-Occludin (1:200, GB111401, Servicebio, China). Slides were observed and scanned using a virtual slide microscope (Olympus, Japan, VS120-S6-W).
Biochemical Detection of Hepatorenal Toxicity
Set the corresponding parameters on the automatic biochemical instrument (version: Chemray 800, Rayto, Shenzhen, China), handle the serum sample according to the requirements, put the sample into the instrument for testing and derive the result. Detection target: ALT (aminotransferase), AST (aspartate transaminase) and Cre (creatinine).
Statistic Analysis
The experimental data were statistically analyzed using GraphPad Prism (version 8.0.2, La Jolla, CA, USA). All data were expressed as mean ±standard error of the mean (SEM). One-way analysis of variance (ANOVA) was used to compare the differences among groups, and P < 0.05 was considered statistical significance.
Results
Network Pharmacology Suggests That TXYF Regulates IBS Through Different Targets and Signaling Pathways
LC-MS technology was used to detect the chemical components of the TXYF decoction, revealing a total of 559 constituents (). Through screening in the TCMSP database, 23 effective constituents were identified, including 18 Saposhnikoviae Radix, 5 Citri Reticulatae Pericarpium, 7 Atractylodis Macrocephalae Rhizoma, and 13 Paeoniae Radix Alba. The corresponding targets for these effective constituents were obtained from TCMSP. The relationships between the herbs- component-targets were visualized using Cytoscape software (). The“irritable bowel syndrome (IBS)“ targets gathered from various disease databases were intersected with the TXYF targets to create a Venn diagram (). The intersecting targets were then used to construct a PPI network diagram through STRING (). Data was imported into Cytoscape for visualization of correlation levels. Further analysis using cytoHub and MODCE revealed CAPS3, MMP9, TNF, BCL2, IL6, PTGS2, ESR1, AKT, TP53 and TGFB1 as the core targets of this analysis ( and ). Subsequently, GO and KEGG analyses were conducted based on the intersecting targets for further exploration ( and ). To further identify the key targets regulated by TXYF in IBS, we intersected IBS targets obtained from different databases with TXYF targets. Consequently, we identified CHRM3 (). We conducted a PPI analysis using the single target “CHRM3” in the STRING database. Due to the single target, the website defaulted to a maximum number of interactors to show: no more than 10 interactors. We exported these results and input them into Cytoscape software for further cytoHub analysis (). We exported the GO and KEGG results obtained from the STRING analysis of CHRM3 and generated visual representations ( and ). One result that attracted our strong interest was the KEGG result showing enrichment of calcium signaling pathways and cholinergic synaptic targets. The size of the nodes represents the count results, indicating the degree of enrichment, while the darkness of the nodes indicates the relevance, representing statistical significance.
Figure 1 Identification of chemical components of TXYF decoction by LC-MS. (A) TXYF chemical composition identification total ion flow diagram. (B) Structural spectrogram information of Naringenin and Nobiletin compounds. (C) Main information on the top 20 compounds with TXYF-Area. (D) The results in Figure (C) are further analyzed using Cytoscape software (Versions 7.2) and arranged in Dgree circle sizes.
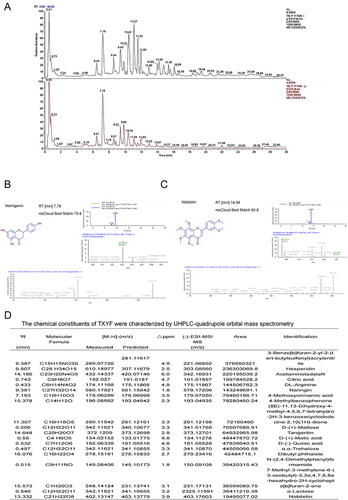
Figure 2 Network pharmacology analysis for TXYF. (A) herbal-ingredient-target relationship diagram. (B) Venn for TXYF targets and target of IBS from all databases. (C) Figure (E) Result Input STRING to draw the PPI network; (D) Figure (F) Results in Cytoscape analysis. (E) cytoHub and MODCE analysis. (F) KEGG analysis. (G) GO analysis. (H) Venn for IBS-target from different databases and TXYF targets. (I) PPI and cytoHub analysis for CHRM3. (J) CHRM3 for GO analysis. (K) KEGG analysis for CHRM3. Color depth is positively correlated with correlation, circle size is positively correlated with enrichment quantity.
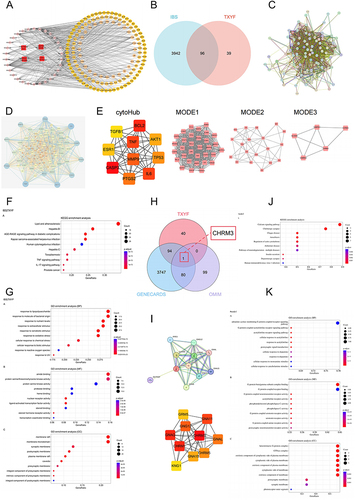
Select the top five effective components with the most interactions with targets from . Molecular docking them with cytoHub targets or CHRM3 ( and ). Consistently, a binding energy less than −7.2 is considered to indicate high binding affinity. Select Naringenin and Nobiletin, which exhibit the highest interactions. Visualize their molecular docking results with the specified targets (Naringenin vs CAPS3, MMP9, TNF, AKT, PTGS2, ESR1, BCL2, and CHRM3; Nobiletin vs TNF, PTGS2, AKT, CAPS3 and BCL2). The molecular docking score for these interactions should be less than −7.2 ().
Figure 3 Molecular docking verification. (A) The five active components of TXYF are molecularly docked with CHRM3. (B) Visualization of connection between Naringenin and CHRM3. (C) Five active components of TXYF are connected with cytoHub target molecules. (D and E) Naringenin and Nebiletin perform docking visualizations with targets scoring less than −7.2. The different types of interactions and binding modes generated by the docking were represented by dashed lines of various colors. Conventional hydrogen bonding was denoted by green dashed lines, π-σ interactions by blue-purple dashed lines, π-Alkyl interactions by light pink dashed lines, hydrophobic interactions by light green dashed lines, and π-sulfur interactions by yellow dashed lines.
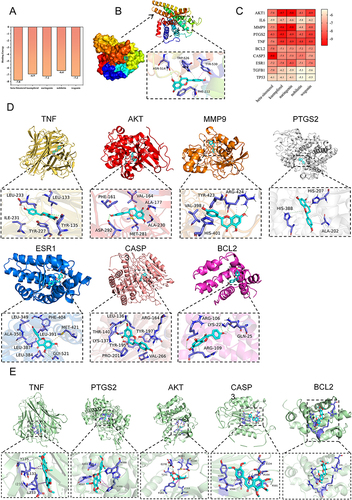
Therapeutic Effects of TXYF on IBS Mice (Body Weight, Fecal Water Content, AWR Score)
The IBS-D model was wrap-restraint stress (WRS) (). Daily weight changes were recorded. The weight of the IBS group mice was significantly lower than that of control group mice after WRS (P < 0.05). Following TXYF oral administration, mice showed a significant increase in weight compared to the IBS group (P < 0.05) (). The study results on fecal water content showed that the number of fecal pellets and water content in the IBS group were significantly higher than those in the control group, and TXYF treatment significantly reduced these parameters in the IBS model (). To assess the development of visceral hypersensitivity in the colon, we compared the AWR scores of the three groups under the pressure stimulation of 0.25, 0.35, or 0.5 mL. The AWR scores in the IBS group were significantly higher than those in the control group (P < 0.01 at 0.25 mL, P < 0.001 at 0.35 mL, and P < 0.001 at 0.5 mL). The AWR scores in the TXYF group were significantly lower than those in the IBS group under 0.25 mL, 0.35 mL, and 0.5 mL stimulation (P < 0.05, P < 0.01, and P < 0.001, respectively). Although the AWR scores in the TXYF group under 0.5 mL stimulation were higher than those in the control group (P < 0.01 for both comparisons), there was no statistically significant difference in AWR scores compared to the control group under 0.25 mL and 0.35 mL stimulations (P > 0.05) (). This evidence indicates that TXYF effectively alleviated IBS-induced diarrhea, weight loss, and visceral sensitivity. Serum AST, ALT and Cre tests proved that TXYF had no significant toxicity to liver and kidney (Figure S1).
Figure 4 Verify cytoHub targets in vivo. (A) Schematic illustrations of experimental protocols. (B) Changes of body weight of mice in each group (N = 8). (C) Fecal water content in each group (N = 8). (D) AWR scores at a pressure stimulation of 0.25 mL/0.35 mL/0.5 mL (N = 8). (E) Changes of protein expression of cytoHub target in colon tissue of mice in each group (N = 8). (F) Figure E was quantified in gray scale (N = 8). (G) Colon HE staining of mice in each group (N = 6). Values were means±SD. N.S. = P > 0.05 Control vs IBS/IBS+TXYF or IBS vs IBS+TXYF; *P < 0.05, **P < 0.01, ***P < 0.001 Control vs IBS/IBS+TXYF; #P < 0.05, ##P < 0.01, ###P < 0.001 IBS vs IBS+TXYF. N represents the number of mice selected, and one mouse represents one repeat.
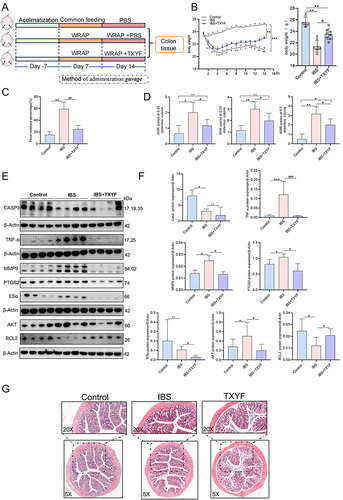
Validation of the Role of TXYF in vivo Intervention on the Core Target of cytoHub in the Colon
To verify the in vivo intervention effect of TXYF on the core targets of cytoHub, we collected colon tissues from IBS model mice. Colon tissues from 4 randomly selected mice in each group were ground and used for protein extraction experiments. The Western blot results showed a significant increase in TNF-α, MMP9, PTGS2, and AKT, and a decrease in CASP3 and BCL2 in the colon tissues of the IBS model (P < 0.05). Following TXYF treatment, TNF-α, MMP9, PTGS2, and AKT significantly decreased (P < 0.05), BCL2 was upregulated (P < 0.05), but there was no up-regulation of CASP3 after TXYF treatment (P > 0.05). Additionally, ESR1 did not show statistical significance for the model group due to large intra-group differences in the control group (P > 0.05), and TXYF was able to down-regulate the expression of ESR1 (P < 0.05) ( and ). TXYF treatment group did not show a down-regulation effect on CASP3 in the colon of IBS mice. However, the significant changes in MMP9 and TNF-α are closely associated with inflammation research. Therefore, HE staining was performed on the colon tissues. The results revealed no significant inflammatory infiltration in the colon of IBS (). In conclusion, the experimental results suggest the activation of inflammation-related factors in the water avoidance stress IBS model, and this activation can be inhibited by TXYF. However, there is no substantial inflammatory infiltration or organic damage.
TXYF Inhibits Up-Regulated CHRM3 and Ach in IBS
In vivo detection of CHRM3 expression in IBS model mice was conducted. The Western blot (WB) and immunohistochemistry (IHC) results indicated a significant increase in CHRM3 expression in the colon of the IBS model group compared to the control group (P < 0.05). Furthermore, the IHC results indicated that the increased CHRM3 expression primarily localized in the epithelial cells. After TXYF treatment, the expression of CHRM3 decreased compared to the IBS model group (P < 0.05) ( and ). CHRM3, as a muscarinic acetylcholine receptor, is regulated by acetylcholine (Ach). ELISA was utilized to detect the expression of Ach in the serum and colon. In both the serum and colon, Ach in the IBS group was significantly increased compared to the control group. Conversely, after TXYF treatment, Ach was significantly decreased compared to the IBS group (P < 0.05) ( and ). Based on these results, it is suggested that TXYF alleviates the diarrhea symptoms of IBS by reducing Ach and CHRM3 levels, inhibiting their binding, and thereby relieving colonic smooth muscle spasms.
Figure 5 The expression of CHRM3 and Gq/PLC/MLCK in colon of each group. (A) The IHC results of CHRM3 in the colon show that the arrow points to areas with high CHRM3 expression, and the dark brown stain shows positive CHRM3 expression (N = 6). (B) WB verified the expression of CHRM3 in colon tissue of each group of mice (N = 8). (C) The expression of Ach in colon tissue of each group by ELISA (N = 6). (D) The expression of Ach in serum tissues of each group by ELISA (N = 6). (E and F) The expressions of Gq, G11 and MLCK were verified by WB (N = 8). (G) The expression of MLCK in colon of each group was verified by ELISA (N = 6). (H) The expression of PLC in colon of each group was verified by ELISA (N = 6). Values were means±SD. N.S. = P > 0.05 Control vs IBS/IBS+TXYF or Control vs IBS/IBS+TXYF; *P < 0.05, **P < 0.01, ***P < 0.001 Control vs IBS/IBS+TXYF; #P < 0.05, ##P < 0.01, ###P < 0.001 IBS vs IBS+TXYF. N represents the number of mice selected, and one mouse represents one repeat.
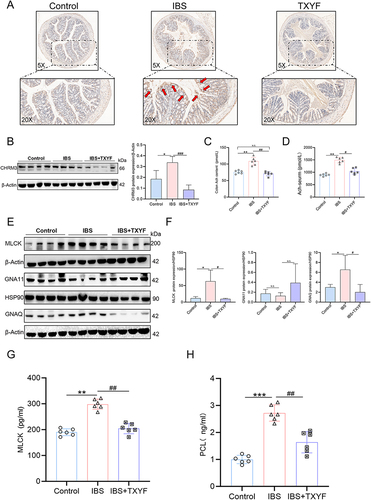
TXYF Alleviate IBS by Inhibiting GNAQ/PLC/MLCK Diastolic Smooth Muscle
In the KEGG enrichment analysis of CHRM3, the calcium signaling pathway shows the highest correlation. The activation of Ach and CHRM3 is thought to regulate smooth muscle. We can see that CHRM3 activation of Gq or G11 can affect the contractile function by affecting the expression of downstream MLCK (Figure S2). Therefore, we measured the expression of GNAQ (Gq), GNA11 (G11), PLC and MLCK. The activation of GNAQ and MLCK in the IBS model and their inhibition after TXYF treatment was confirmed in the WB experiment (P < 0.05). However, there was no statistical difference for GNA11 (P > 0.05) ( and ). ELISA results show that PLC and MLCK are activated in IBS (P < 0.05). TXYF can downregulate the expression of PLC and MLCK (P < 0.05) ( and ). Therefore, we believe that TXYF alleviates smooth muscle excessive contraction and spasms and relieves IBS diarrhea by inhibiting Ach and CHRM3, disrupting the coupling of CHRM3 with Gq, and down-regulating the expression of PLC/MLCK.
TXYF Enhances Intestinal Chemical Barrier by Regulating Goblet Cell Secretion
Through the counting of goblet cells, we observed a reduction in goblet cells in the IBS model compared to the control group. Following TXYF treatment, an increase in goblet cells was observed compared to the IBS model group. Statistical analysis of randomly selected slices from three groups did not show significant differences (P > 0.05) (). Further investigation into the secretion of goblet cells revealed that in the AB-PAS experiment, the secretion in the IBS group was relatively reduced compared to the control group, with a lighter blue color indicating an increase in acidic mucus produced by goblet cells. After TXYF treatment, the secretion of goblet cells still exhibited a light blue acidic mucus, but it was reduced compared to the IBS group, with an overall increase in mucus secretion compared to the IBS group (). Subsequently, we examined the intestinal-specific secretory protein MUC2 of goblet cells. The Western blot results indicated a significant decrease in MUC2 in the IBS group compared to the control, which increased after TXYF treatment ( and ). The experimental results suggest that TXYF can enhance intestinal chemical barrier by increasing goblet cell secretion, thereby alleviating IBS.
Figure 6 TXYF protects the gut barrier. (A) Goblet cell count (N = 6). (B) Typical representation of goblet cell secretion detected by AB-PAS staining (N = 6). Purple blue was neutral mucin and various glycoproteins, light blue was acidic mucin. (C and D) Changes in the expression of MUC2 protein associated with goblet cells and tight junction (TJ) related proteins ZO-1 and Occludin in each group (N = 8). (E and F) Changes of fluorescence expression of ZO-1 and Occludin in each group (N = 6). (G) Changes in expression of NF-κB signaling pathway related proteins in each group (N = 8). *P < 0.05, **P < 0.01, ***P < 0.001 Control vs IBS; #P < 0.05, ##P < 0.01, ###P < 0.001 IBS vs IBS+TXYF. N represents the number of mice selected, and one mouse represents one repeat.
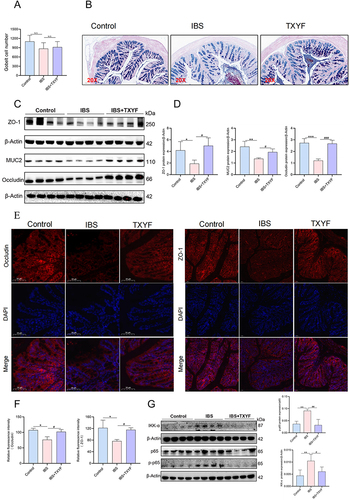
TXYF Reduces Physical Barrier Damage by Inhibiting NF-κB/MLCK
In this experiment, under the premise of TNF-α and MLCK activation in the IBS model, we examined the changes in the intestinal physical barrier components ZO-1 and Occludin. Both immunofluorescence and Western blot results demonstrated a decrease in the expression of ZO-1 and Occludin in the IBS model compared to the control group. Following TXYF treatment, the expression of ZO-1 and Occludin increased compared to the IBS group (). Subsequently, we analyzed the protein expression of Ikk-α, p65 and p-p65 in the NF-κB signaling pathway. Compared to the control group, the IBS model group showed increased protein expression of IKK-α and p-p65. In contrast, the TXYF treatment group exhibited decreased protein expression of IKK-α and p-p65 compared to the IBS model group (). Based on all the experimental results, we hypothesize that TXYF may reduce intestinal physical barrier damage through ZO-1 and Occludin, potentially achieved by inhibiting the activated NF-κB/MLCK signaling pathway.
Discussion
IBS, as one of the most common gastrointestinal functional disorders, significantly impacts patient quality of life. However, Clinical treatment strategies for IBS-D have shown unsatisfactory results.Citation22 TXYF has been widely and long-term employed in clinical practice for treating IBS. Despite considerable research by scholars, the mechanisms underlying its therapeutic effects remain not completely understood, especially concerning intestinal motility and mucosal barrier protection. In this study, we examined the molecular mechanisms of TXYF treatment in IBS-model mice. This included a network pharmacology analysis focusing on cytoHub-targets and CHRM3. We validated the tight binding of TXYF active components with the cytoHub-targets and CHRM3 from a virtual molecular docking perspective, with subsequent visualization. In vivo validation confirmed TXYF regulatory effects on cytoHub-related targets and CHRM3. The KEGG enrichment analysis of CHRM3 revealed the regulatory role of Gq/PLC/MLCK in IBS. Based on the premise that TNF-α activation of NF-κB/MLCK damages tight connections in the gut.Citation23 Finally, we established that TXYF can rescue the physical barrier damage in IBS-model colon by inhibiting the TNF-α/NF-κB/MLCK axis. Furthermore, TXYF can salvage the chemical barrier damage in IBS-model colon by regulating goblet cell secretion.
The LC-MS analysis detected 559 chemical components in the TXYF decoction. Subsequently, effective components of TXYF and their corresponding targets were screened in TCMSP. A network was constructed using Cytoscape to identify the top five effective components with the most interactions with targets. Naringenin and Nobiletin caught our attention. After obtaining the intersection of IBS targets from the disease database and effective component targets of TXYF, cytoHub analysis was conducted, resulting in the identification of core hub targets represented by CAPS3, MMP9 and TNF. Previous network pharmacology studies had briefly analyzed these targets. In this study, we performed molecular docking validation and in vivo verification of these targets. At the molecular docking level, Naringenin was found to tightly bind with CAPS3, MMP9, TNF, PTGS2, AKT1, BCL2, CASP3 and ESR1. Similarly, Nobiletin demonstrated tight binding with AKT1, PTGS2, TNF, CASP3 and BCL2. Consistent with the molecular docking results, in colon tissues of IBS group compared to the control group, increased expression of MMP9, TNF-α, PTGS2, AKT and IL6, along with decreased expression of CAPS3 and BCL2. But ESR1 did not exhibit any protein changes. Following TXYF treatment, compared to the IBS group, decreased expression of MMP9, TNF-α, PTGS2, AKT, IL6 and ESR1, along with increased expression of BCL2. But no changes were observed in CAPS3 protein expression. Overall, the data suggests that TNF-α form tight associations with the primary effective components of TXYF and are significantly regulated by TXYF in vivo. TNF-α has been found to increase in IBS and IBD indicating a close association with intestinal inflammation.Citation24,Citation25 Consequently, we conducted HE staining of colon tissues, but we did not observe significant inflammatory infiltration in the colon tissue. This suggests that even with the activation of relevant pro-inflammatory factors, IBS did not exhibit organic changes in the colon.
CHRM3 is a muscarinic acetylcholine receptor that is widely expressed in the intestines.Citation26 In this study, datasets from disease databases consistently revealed a close association between CHRM3 and IBS, and as CHRM3 acts as a target for the effective components of TXYF, it has piqued our interest. This study aimed to explore how TXYF alleviates IBS by modulating CHRM3 through molecular docking and in vivo validation. In the molecular docking analysis, the effective component of TXYF, Naringenin, tightly bound to CHRM3. Acetylcholine (Ach) is a signaling molecule in the central and peripheral nervous systems that can regulate intestinal smooth muscle by interacting with CHRM3 and coupling with Gq/G11 in the G protein.Citation14,Citation15 Previous research has demonstrated that increased Ach expression leads to increased intestinal motility and is mediated through CHRM3 regulation of chloride ion secretion.Citation27 In studies of constipation, a downregulation of CHRM3 expression was observed in constipation models, despite an upward trend in Ach expression, leading to abnormally decreased intestinal motility that results in constipation. Increasing CHRM3 expression can enhance intestinal motility and alleviate constipation.Citation28,Citation29 In IBS diarrhea, excessive contraction of intestinal smooth muscles leads to spasms. Normal levels of Ach and CHRM3 activity maintain normal intestinal contractions; however, excessive expression of Ach and CHRM3 results in frequent and intense contractions, causing abnormal intestinal motility and diarrhea.Citation11 In our results, IBS-model mice experienced a significant increase in Ach in the intestines and serum, accompanied by a significant increase in colon of CHRM3 protein expression. Following treatment with TXYF, Ach levels decreased, along with a reduction in CHRM3 protein expression. Therefore, we believe that the abnormal increase in Ach and CHRM3 is one of the causes of diarrhea in IBS, and that TXYF can alleviate diarrhea caused by abnormal intestinal motility by downregulating Ach and CHRM3.
In KEGG enrichment analysis of CHRM3, we found the strongest enrichment relevance in the Calcium signaling pathway. Within the Calcium signaling pathway, it is known that neurotransmitters regulate GPCR to activating G-protein, which in turn activates PLC/MLCK to participate in motility.Citation30 In other studies, it has been found that CHRM3 can increase the levels of Ca2+ by coupling with Gq/G11, thereby activating phospholipase C (PLC).Citation31 It is well established that after Ach activates CHRM3, CHRM3 coupling with Gq/G11 regulates intestinal smooth muscle contraction.Citation32 Both Ach as a neurotransmitter and CHRM3 as a member of GPCR have been confirmed to be activated in the IBS model in this experiment. Furthermore, following TXYF treatment, the expression of both Ach and CHRM3 was down-regulated. Therefore, we were curious about the downstream regulation of GNAQ/PLC/MLCK, speculating whether this is related to the diarrhea caused by abnormal intestinal motility in IBS. In the results, we observed that GNAQ/PLC/MLCK were activated in the IBS model group. In comparison to the IBS group, the expression of GNAQ/PLC/MLCK decreased after TXYF treatment. Therefore, we infer that after CHRM3 is activated by Ach and couples with GNAQ, it subsequently activates PLC/MLCK, leading to abnormal intestinal contractions that cause diarrhea in IBS. This effect is relieved after TXYF treatment.
Recent research indicates the presence of intestinal barrier damage in IBS.Citation33 In studies of the chemical barrier of the intestinal barrier, the latest findings suggest that when TNF-α is knocked out, secretory progenitor cells differentiate into goblet cells.Citation34 MMP9 has been found to be negatively correlated with the goblet cell-secreted protein MUC2, activation of MMP9 can inhibit the expression of the goblet cell-secreted protein MUC2.Citation35 Interestingly, in other studies, it has been shown that CHRM3 can stimulate goblet cell secretion in the intestinal crypts by activating Ca2+, which protects the intestine.Citation36 These are two contradicting results. To validate the changes in goblet cells in IBS, we conducted a count of goblet cells in colon sections. In the IBS model group with increased TNF-α, a decrease in goblet cells was observed, whereas in the group with reduced TNF-α expression after TXYF treatment, an increase in goblet cells was noted. However, after counting randomly selected slides, no statistically significant differences were found, implying that IBS did not have a significant impact on the number of goblet cells. We believe that this may be due to the incomplete elimination of TNF-α by TXYF inhibition. Some scholars have suggested that changes in goblet cell secretion in IBS can disrupt the intestinal chemical barrier.Citation33,Citation37 We verified and obtained results showing a decrease in MUC2 expression in goblet cell secretion in IBS model. In comparison to IBS group, following TXYF treatment, the expression of MUC2 in goblet cells increased. This contradicts the results of the activation of the CHRM3 Ca2+ pathway stimulating MUC2 secretion in the crypts of the small intestine. In other research, it has been suggested that this may be due to the disruption of intestinal flora in the restraint-stress model, leading to disruption caused by an imbalance of amino acids.Citation38 The CHRM3-regulated Ca2+ in the colon cannot inhibit the damage to the intestinal chemical barrier caused by the disruption of the intestinal flora in IBS. Further research is needed. However, the results of this study indicate that TXYF does not significantly affect the number of goblet cells, but it can increase goblet cell secretion, thereby inhibiting damage to the intestinal chemical barrier.
The intestinal barrier includes not only the chemical barrier but also the physical barrier and the immune barrier. Previous studies have indicated that MMP9 increases the permeability of intestinal epithelial TJ by activating the basal lateral membrane receptor.Citation39 At the same time, TNF-α can activate the NF-κB/MLCK signaling axis, contributing to the damage of the physical barrier in the intestine.Citation40 Furthermore, other studies on IBS have found a dysregulation of tight junction proteins, leading to damage of gut barrier.Citation41 Based on the activation of MMP9, TNF-α, and MLCK confirmed in this study in IBS, and their inhibition following TXYF treatment, we examined the expression of the physical barrier markers ZO-1 and Occludin. Our results show that in the colon of the IBS model mice, the expression of ZO-1 and Occludin is down-regulated. In comparison to the IBS group, the TXYF treatment group showed up-regulation of ZO-1 and Occludin expression. Additionally, we examined the NF-κB signaling pathway, the results of which also confirmed the activation of NF-κB in IBS. TXYF was found to inhibit the activation of NF-κB. Consequently, we conclude that TXYF rescues the damage to the physical barrier of the colon in IBS by inhibiting the up-regulation of ZO-1 and Occludin through the inhibition of NF-κB/MLCK.
This study has several limitations. While previous research has demonstrated that knocking out CHRM3 in colonic macrophages leads to an increase in TNF-α in the case of specific bacterial infections, the mutual relationship between TNF-α and CHRM3 in IBS has not been further investigated. Additionally, our study was only conducted in male mice and did not include control groups of different genders. Future research needs to address these limitations and clarify the broader implications of our findings. In conclusion, this study confirms the therapeutic effects of TXYF on restraint-stress-induced IBS mice through CHRM3/Gq/PLC/MLCK, as well as TXYF’s therapeutic effects on the intestinal barrier of restraint-stress-induced IBS mice through TNF-α/NF-κB/MLCK and regulation of goblet cell secretion. Overall, compared to the untreated group, the general condition, histopathology, and abnormal protein expression in the treated group of mice showed improvement. These findings underscore the potential of TXYF as a treatment for IBS and provide further evidence for the clinical application of TXYF in IBS treatment.
Conclusion
TXYF can reduce the expression of Ach and CHRM3, inhibit the Gq/PLC/MLCK signal axis, and relieve IBS diarrhea by dilating IBS colon. In addition, TXYF protects the intestinal barrier by regulating the TNF-α, NF-κB/MLCK axis, and goblet cell secretion.
Abbreviations
Ach, Acetylcholine; AB-PAS, Periodic Acid Schiff and Alcian Blue Stain; BCL2, B-cell Lymphoma-2; CASP3, Caspase-3; CHRM1, Cholinergic Receptor Muscarinic 1; CHRM3, Cholinergic Receptor Muscarinic 3; ESα, Estrogen Receptor; ELISA, Enzyme Linked Immunosorbent Assay; GO, Gene Ontology; GNAQ (Gq), G Protein Subunit Alpha Q; GNA11 (G11), G Protein Subunit Alpha 11; HE, Hematoxylin and Eosin Staining; IHC, Immunohistochemistry; KEGG, Kyoto Encyclopedia of Genes and Genomes; LC-MS, Liquid Chromatography-Tandem Mass Spectrometry; MLCK, Myosin Light-Chain Kinase; MMP9, Matrix Metalloproteinase-9; OMIM, Online Mendelian Inheritance in Man; PLC, Phospholipase C; PPI, Protein-Protein Interaction Networks; PTGS2, Prostaglandin-Endoperoxide Synthase 2; TNF-α, Tumor Necrosis Factor-α; TTD, Therapeutic Target Database; TCMSP, Traditional Chinese Medicine Systems Pharmacology Database and Analysis Platform.
Disclosure
The authors report no conflicts of interest in this work.
Data Sharing Statement
All the data involved in this study are available in this published article.
Additional information
Funding
References
- Ford AC, Lacy BE, Talley NJ. Irritable bowel syndrome. New Engl J Med. 2017;376(26):2566–2578. doi:10.1056/NEJMra1607547
- Fukudo S, Okumura T, Inamori M, et al. Evidence-based clinical practice guidelines for irritable bowel syndrome 2020. J Gastroenterol. 2021;56(3):193–217. doi:10.1007/s00535-020-01746-z
- Bonetto S, Fagoonee S, Battaglia E, Grassini M, Saracco GM, Pellicano R. Recent advances in the treatment of irritable bowel syndrome. Pol Arch Intern Med. 2021;131(7–8):709–715. doi:10.20452/pamw.16067
- Holtmann GJ, Ford AC, Talley NJ. Pathophysiology of irritable bowel syndrome. Lancet Gastroenterol Hepatol. 2016;1(2):133–146. doi:10.1016/S2468-1253(16)30023-1
- Chen M, Tang TC, Wang Y, et al. Randomised clinical trial: Tong-Xie-Yao-Fang granules versus placebo for patients with diarrhoea-predominant irritable bowel syndrome. Aliment Pharmacol Ther. 2018;48(2):160–168. doi:10.1111/apt.14817
- Liang S-B, Cao H-J, Kong L-Y, et al. Systematic review and meta-analysis of Chinese herbal formula tongxie yaofang for diarrhea-predominant irritable bowel syndrome: evidence for clinical practice and future trials. Front Pharmacol. 2022;13:904657.
- Zhang M, Zheng Y, Li X, et al. Tong-Xie-Yao-Fang alleviates diarrhea-predominant irritable bowel syndrome in rats via the GCN2/PERK-eIF2α-ATF4 signaling pathway. Phytomedicine. 2022;107:154350.
- Yin Y, Zhong L, Wang JW, Zhao XY, Zhao WJ, Kuang HX, Kuang H-X. Tong Xie Yao Fang relieves irritable bowel syndrome in rats via mechanisms involving regulation of 5-hydroxytryptamine and substance P. World J Gastroenterol. 2015;21(15):4536–4546. doi:10.3748/wjg.v21.i15.4536
- Li J, Cui H, Cai Y, et al. Tong-Xie-Yao-Fang regulates 5-ht level in diarrhea predominant irritable bowel syndrome through gut microbiota modulation. Front Pharmacol. 2018;9:1110. doi:10.3389/fphar.2018.01110
- Chen H, Kan Q, Zhao L, et al. Prophylactic effect of Tongxieyaofang polysaccharide on depressive behavior in adolescent male mice with chronic unpredictable stress through the microbiome-gut-brain axis. Biomed Pharmacother. 2023;161:114525.
- Gallegos-Perez J-L, Limon A, Reyes-Ruiz JM, Alshanqeeti AS, Aljohi MA, Miledi R. Analysis of free ACh and 5-HT in milk from four different species and their bioactivity on 5-HT3and nACh receptors. Food Funct. 2014;5(7):1489–1494. doi:10.1039/C3FO60458F
- Bennet SMP, Polster A, Törnblom H, et al. Global cytokine profiles and association with clinical characteristics in patients with irritable bowel syndrome. Am J Gastroenterol. 2016;111(8):1165–1176. doi:10.1038/ajg.2016.223
- Zhao L, Zhang H, Li N, et al. Network pharmacology, a promising approach to reveal the pharmacology mechanism of Chinese medicine formula. J Ethnopharmacol. 2023;309:116306. doi:10.1016/j.jep.2023.116306
- Qin K, Dong C, Wu G, Lambert NA. Inactive-state preassembly of G(q)-coupled receptors and G(q) heterotrimers. Nat Chem Biol. 2011;7(10):740–747. doi:10.1038/nchembio.642
- Wess J. Muscarinic acetylcholine receptor knockout mice: novel phenotypes and clinical implications. Annu Rev Pharmacol Toxicol. 2004;44(1):423–450. doi:10.1146/annurev.pharmtox.44.101802.121622
- McLean LP, Smith A, Cheung L, et al. Type 3 muscarinic receptors contribute to clearance of citrobacter rodentium. Inflamm Bowel Dis. 2015;21(8):1860–1871. doi:10.1097/MIB.0000000000000408
- Cheru L, Saylor CF, Lo J. Gastrointestinal barrier breakdown and adipose tissue inflammation. Curr Obes Rep. 2019;8(2):165–174. doi:10.1007/s13679-019-00332-6
- Al-Sadi R, Engers J, Haque M, King S, Al-Omari D, Ma TY. Matrix metalloproteinase-9 (MMP-9) induced disruption of intestinal epithelial tight junction barrier is mediated by NF-kappaB activation. PLoS One. 2021;16(4):e0249544. doi:10.1371/journal.pone.0249544
- Zhang H-Y, Zeng H-R, Wei H-Z, et al. Tongxie-Yaofang formula regulated macrophage polarization to ameliorate DSS-induced colitis via NF-κB/NLRP3 signaling pathway. Phytomedicine. 2022;107:154455.
- Zhou Y, Zhang F, Mao L, et al. Bifico relieves irritable bowel syndrome by regulating gut microbiota dysbiosis and inflammatory cytokines. Eur J Nutr. 2022;62(1):139–155. doi:10.1007/s00394-022-02958-0
- Guo L, Chen Q, Gao Y, et al. CDP-choline modulates cholinergic signaling and gut microbiota to alleviate DSS-induced inflammatory bowel disease. Biochem Pharmacol. 2023;217:115845. doi:10.1016/j.bcp.2023.115845
- Chey WD, Kurlander J, Eswaran S. Irritable bowel syndrome. JAMA. 2015;313(9):949–958—.
- Gong S, Zheng J, Zhang J, et al. Taxifolin ameliorates lipopolysaccharide-induced intestinal epithelial barrier dysfunction via attenuating NF-kappa B/MLCK pathway in a Caco-2 cell monolayer model. Food Res Int. 2022;158:111502.
- He WQ, Wang J, Sheng JY, Zha JM, Graham WV, Turner JR. Contributions of myosin light chain kinase to regulation of epithelial paracellular permeability and mucosal homeostasis. Int J Mol Sci. 2020;21(3):993. doi:10.3390/ijms21030993
- Brzozowski B, Mazur-Bialy A, Pajdo R, et al. Mechanisms by which stress affects the experimental and clinical inflammatory bowel disease (ibd): role of brain-gut axis. Curr Neuropharmacol. 2016;14(8):892–900. doi:10.2174/1570159X14666160404124127
- Alizadeh M, Schledwitz A, Cheng K, Raufman JP. Mechanistic clues provided by concurrent changes in the expression of genes encoding the m(1) muscarinic receptor, beta-catenin signaling Proteins, and downstream targets in adenocarcinomas of the colon. Front Physiol. 2022;13:857563. doi:10.3389/fphys.2022.857563
- Gros M, Gros B, Mesonero JE, Latorre E. Neurotransmitter dysfunction in irritable bowel syndrome: emerging approaches for management. J Clin Med. 2021;10(15):3429. doi:10.3390/jcm10153429
- Sun P, Zhou K, Wang S, et al. Involvement of MAPK/NF-kappaB signaling in the activation of the cholinergic anti-inflammatory pathway in experimental colitis by chronic vagus nerve stimulation. PLoS One. 2013;8(8):e69424. doi:10.1371/journal.pone.0069424
- Kim JE, Lee MR, Park JJ, et al. Quercetin promotes gastrointestinal motility and mucin secretion in loperamide-induced constipation of SD rats through regulation of the mAChRs downstream signal. Pharm Biol. 2018;56(1):309–317. doi:10.1080/13880209.2018.1474932
- Chen D, Xiong Y, Lin Y, et al. Capsaicin alleviates abnormal intestinal motility through regulation of enteric motor neurons and MLCK activity: relevance to intestinal motility disorders. Mol Nutr Food Res. 2015;59(8):1482–1490. doi:10.1002/mnfr.201500039
- Kan W, Adjobo-Hermans M, Burroughs M, et al. M3 muscarinic receptor interaction with phospholipase C beta3 determines its signaling efficiency. J Biol Chem. 2014;289(16):11206–11218. doi:10.1074/jbc.M113.538546
- Layunta E, Forcen R, Grasa L. TLR2 and TLR4 modulate mouse ileal motility by the interaction with muscarinic and nicotinic receptors. Cells. 2022;11(11):1791. doi:10.3390/cells11111791
- Chen Q, Zhang H, Sun CY, et al. Evaluation of two laboratory model methods for diarrheal irritable bowel syndrome. Mol Med. 2023;29(1):5. doi:10.1186/s10020-022-00599-x
- Reyes EA, Castillo-Azofeifa D, Rispal J, et al. Epithelial TNF controls cell differentiation and CFTR activity to maintain intestinal mucin homeostasis. J Clin Invest. 2023;133(20). doi:10.1172/JCI163591
- Garg P, Ravi A, Patel NR, et al. Matrix metalloproteinase-9 regulates MUC-2 expression through its effect on goblet cell differentiation. Gastroenterology. 2007;132(5):1877–1889. doi:10.1053/j.gastro.2007.02.048
- Dolan B, Ermund A, Martinez-Abad B, Johansson MEV, Hansson GC. Clearance of small intestinal crypts involves goblet cell mucus secretion by intracellular granule rupture and enterocyte ion transport. Sci Signaling. 2022;15(752):eabl5848. doi:10.1126/scisignal.abl5848
- Liu S, Huang Q, Huang Q, et al. The protective effects of electroacupuncture on intestinal barrier lesions in IBS and UC model. Sci Rep. 2023;13(1):7276. doi:10.1038/s41598-023-34182-z
- Yu J, Liu T, Guo Q, Wang Z, Chen Y, Dong Y. Disruption of the intestinal mucosal barrier induced by high fructose and restraint stress is regulated by the intestinal microbiota and microbiota metabolites. Microbiol Spectr. 2023;11(2):e0469822. doi:10.1128/spectrum.04698-22
- Augoff K, Hryniewicz-Jankowska A, Tabola R, Stach K. MMP9: a tough target for targeted therapy for cancer. Cancers. 2022;14(7):1847. doi:10.3390/cancers14071847
- He F, Peng J, Deng XL, et al. Mechanisms of tumor necrosis factor-alpha-induced leaks in intestine epithelial barrier. Cytokine. 2012;59(2):264–272. doi:10.1016/j.cyto.2012.04.008
- Xing Y, Xue S, Wu J, et al. Serum exosomes derived from irritable bowel syndrome patient increase cell permeability via regulating mir-148b-5p/rgs2 signaling in human colonic epithelium cells. Gastroenterol Res Pract. 2021;2021:6655900. doi:10.1155/2021/6655900