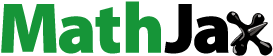
Abstract
Naturally occurring polymers, particularly of the polysaccharide type, have been used pharmaceutically for the delivery of a wide variety of therapeutic agents. Chitosan, the second abundant naturally occurring polysaccharide next to cellulose, is a biocompatible and biodegradable mucoadhesive polymer that has been extensively used in the preparation of micro-as well as nanoparticles. The prepared particles have been exploited as a potential carrier for different therapeutic agents such as peptides, proteins, vaccines, DNA, and drugs for parenteral and nonparenteral administration. Therapeutic agent-loaded chitosan micro- or nanoparticles were found to be more stable, permeable, and bioactive. In this review, we are highlighting the different methods of preparation and characterization of chitosan micro- and nanoparticles, while reviewing the pharmaceutical applications of these particles in drug delivery. Moreover, the roles of chitosan derivatives and chitosan metal nanoparticles in drug delivery have been illustrated.
Introduction
Drug delivery is a general term that refers to formulation and administration of a pharmacologically active compound for the purpose of providing an efficient drug plasma concentration, as well as bringing the drug to the specific site of action. Different strategies have been employed to overcome some drug stability issues in the gastrointestinal tract (GIT), control the drug release, enhance the transmucosal absorption, as well as get the drug into its site of action. Microfabrication is a technique that creates materials in the micrometer scale feature and has been reported to significantly improve diagnosis and biomedical applications. Modification of the material shape, surface characteristics, and release kinetics is a consequence of this micronization.Citation1 Nanotechnology has been utilized as one of these strategies in the development of novel drug delivery systems through entrapment of the drug in nanoparticulate systems.Citation2 In general, the potential applications of nanotechnology render this field an area of interest to many researchers and scientists. These applications have covered different scientific areas that extend from electronics to cosmetics.Citation3–Citation5
Polymers obtained from natural origins have been extensively employed not only in the food industry but also in pharmaceutical technology. Polysaccharide polymers have emerged as being one of these because they are less toxic, biocompatible, and biodegradable.Citation6,Citation7 Incorporation of the therapeutic agent into a polymeric matrix, particularly of a natural origin, might potentiate the protection of the biologically active compound from degradation, control drug release, improve absorption, enhance the therapeutic effect, and lead to the consequential decrease in the frequency of administration. Chitosan, alginate, and carrageenan are the most commonly used polysaccharide polymers in various pharmaceutical applications.Citation6–Citation12 Chitosan is a polymer of interest that has been widely used for delivery of different therapeutic agents, particularly those based on chitosan micro- and nanoparticles, owing to its unique properties. Preparation of mucoadhesive formulations, enhancing the dissolution rate especially for poorly water-soluble drugs, utilization in drug targeting, and improvement of protein absorption are common therapeutic applications of this naturally occurring polymer.Citation13
In this review, the importance of chitosan, as a naturally occurring polysaccharide polymer, and its derivatives in drug delivery are illustrated. The different methods of preparation and characterization of chitosan micro- and nanoparticles are addressed. The usefulness of these particles in parenteral and nonparenteral drug delivery is demonstrated. Finally, a very specific application of chitosan in the preparation of metal-based nanoparticles is clarified, establishing the advantages of chitosan metal nanoparticles over the metal nanoparticles.
Chitosan as a polymeric drug carrier
Chitosan is a molecule with a carbohydrate backbone structure similar to cellulose, which consists of two types of repeating units, N-acetyl-D-glucosamine and D-glucosamine, linked by (1-4)-β-glycosidic linkage.Citation14 It is a biopolyaminosaccharide cationic polymer that is obtained from chitin by alkaline deacetylation and characterized by the presence of a large numbers of amino groups on its chain (). Although chitosan is obtained from chitin, the applications of the latter compared to chitosan are limited because it is chemically inert. A common method for chitosan synthesis is the deacetylation of chitin, usually derived from the shells of shrimp and other sea crustaceans, using excess aqueous sodium hydroxide solution as a reagent. Chitosan is insoluble in water but soluble in dilute acidic solutions of acetic, citric, and tartaric but not phosphoric or sulfuric at pH less than 6.5.Citation15 In dilute aqueous acidic solution, the free amino groups of chitosan glucosamine units that have an apparent pKa of 6.5 undergo protonation and convert into the ionizable soluble R-NH3+ form.Citation16 Usually, dilute aqueous acetic acid solution in concentrations 1%–3% is used to make a soluble chitosan solution. Chitosan is available in low and high molecular weights, ranging between 3,800 and 20,000 Da, and with different grades of deacetylation degree. The molecular weight and degree of deacetylation strongly affect chitosan properties, particularly during the development of micro- and nanoparticles. Chitosan is precipitated with polyanions and in alkaline solutions. Although chitosan has revealed some therapeutic activity such as lowering of cholesterol,Citation17 wound healing,Citation18 antiulcer,Citation19 and antimicrobial effects,Citation20 it is widely used as a polymeric drug carrier owing to its biocompatibility, biodegradability, and nontoxic characters.
Chitosan is characterized by mucoadhesive properties owing to the electrostatic interaction between the positive charge on ionizable R-NH3+ group and the negative charge on the mucosal surfaces.Citation21 The interaction of the protonated amine groups with the cell membrane results in a reversible structural reorganization in the protein-associated tight junctions, which is followed by opening of these tight junctions. Sinha et alCitation22 showed that the molecular weight, strong electrostatic interaction, chitosan chain flexibility, possibility of hydrogen bond formation due to availability of bonding groups such as carboxylic and hydroxyl groups, and ease of spreading into the mucus owing to surface energy properties are factors that attribute to this character. Another advantage that makes chitosan superior to other polysaccharide polymers is the ease of chemical modifications in the structure, especially in the C-2 position, which provide derivatives with different characteristics, with potential use in different applications.Citation23 Pharmaceutically, chitosan-based polymeric drug carriers have been successfully utilized in the delivery of anticancer agents, proteins/peptides, growth factors, antibiotics, anti-inflammatory and other drugs, as well as a strategy in both vaccine delivery and gene therapy.Citation24,Citation25 In addition to the mucoadhesive nature and ease of chemical modification, chitosan possesses properties such as biocompatibility, low toxicity, and biodegradability that make this polymer a good candidate for pharmaceutical formulations through several routes of administration, as will be illustrated in the following sections.
In general, the mucoadhesive nature, which increases the time of attachment at the absorption site, the easy availability of free amino group for cross-linking, ease of fabrication of polymeric particles without using hazardous solvents, the cationic nature that permits ionic cross-linking with multivalent anions, and finally the ability to control the release of the administered drug makes chitosan the polymer of choice for developing the polymeric particle.
Chitosan derivatives
Different chitosan derivatives have been synthesized and studied for their potential applications. Modification does not result in change of the chitosan fundamental skeleton but brings derivatives characterized by new or improved properties. These derivatives have been reported to increase the permeation of drugs, especially hydrophilic macromolecules, achieve colon targeting, protect acid sensitive drugs, enhance drug release in basic environment, and overcome the limited solubility of chitosan in neutral pH.Citation26,Citation27 Various chemically modified chitosan derivatives have been produced through alteration in the chitosan amine or hydroxyl functional groups. Quaternized, thiolated, hydrophobic, and chemically grafted chitosan derivatives are types that have been reported to improve or impart new properties to chitosan.
Quaternary chitosan derivatives of the N-alkyl or quaternary ammonium types have been developed. These derivatives are characterized by their permanent cationic charge (resulting in increase of solubility of chitosan in water and keeping it soluble over a wide range of pH) and their enhanced mucoadhesive and loaded drug penetration properties. N-alkylated chitosan derivatives such as trimethyl, diethylmethyl, triethyl, and dimethyl ethyl chitosans are usually obtained by alkylation of the primary amine groups of chitosan with the suitable aldehyde in the presence of reducing agents. N-trimethyl chitosan (TMC) is synthesized by methylation of the chitosan amine groups with methyl iodide.Citation28 The water solubility of TMC is highly dependent on the degree of methylation.Citation29 An enhancement in the mucoadhesive and, hence, absorption even at neutral pH has been reported for soluble TMC.Citation30–Citation32 Quaternary ammonium chitosan derivatives such as N-(2-hydroxyl) propyl-3-trimethyl ammonium chitosan chloride (HTCC) has been produced by introducing a quaternary ammonium moiety such as glycidyl trimethyl ammonium to the chitosan amine groups, and that was used to formulate albumin-loaded chitosan derivative nanoparticles that showed a size range between 110 and 180 nm and more than 90% albumin encapsulation efficiency.Citation33
Thiolated chitosans are obtained by modification of the chitosan amine groups with cystein, 2-iminothiolane, thiobutylamidine, or thioglycolic acid. The obtained modified chitosan derivatives exhibited improved oral as well as nasal mucoadhesive drug delivery, drug permeation-enhancing effect, and showed in situ gelling behavior owing to inter-and intramolecular disulfide bonds formation when it came in contact with physiological fluids.Citation34–Citation36 Trimethyl chitosan–cysteine (TMC–Cy) is a modified chitosan conjugate that combines the mucoadhesive and permeation improvement effects of TMC and thiolated derivatives. TMC–Cy insulin-loaded nanoparticles have been developed and have demonstrated high insulin encapsulation efficiency and mucoadhesion compared to TMC–insulin nanoparticles.Citation37
Hydrophobic chitosans are chemically modified derivatives that have enhanced stability because of the decrease in the process of matrix hydration and, therefore, show high resistance to gastric enzymatic degradation. Chitosan chemically modified through introduction of carboxylic acid group renders chitosan poorly hydrophilic because of nonionization of the introduced carboxylic group in the acidic environment, while these derivatives are highly hydrophilic in the basic conditions. Chitosan succinate and chitosan phthalate are common examples that showed an insulin-loading capacity of approximately 60% and protected insulin from the gastric enzyme degradation.Citation38,Citation39 Lauryl succinyl chitosan possesses negatively charged mucoadhesive properties and was developed and utilized in the preparation of insulin-loaded micro- and nanoparticles. The prepared particles combined the hydrophilic and hydrophobic characters because of the presence of succinyl and lauryl moieties, respectively, and were found to augment chitosan mucoadhesiveness as well as insulin permeability and release when compared to chitosan particles.Citation40 Another way to introduce a hydrophobic group to chitosan was achieved by acylation using sodium salts of medium-chain fatty acids, fatty acid chlorides, and lactones for use as a polymeric matrix in drug delivery. Lauric, caprylic, and capric acids are examples of fatty acids used to improve the permeability of the incorporated compounds, especially those of hydrophilic compounds such as insulin.Citation41,Citation42 Oleoyl and octanoyl chitosan derivatives are examples of N-acylated chitosans produced with fatty acylchlorides, in which the former showed higher resistance to gastric enzymatic degradation, enhanced mucoadhesiveness, and permeability.Citation43
Synthesis of mono-N-carboxymethyl chitosan (MCC) is achieved by chemical modification of the chitosan amino groups with glyoxylic acid in presence of sodium borohydride as a reducing agent.Citation44 MCC is compatible with anionic agents and has been reported for its application in tissue culture as it decreases the transepithelial electrical resistance of Caco-2 cell monolayers.Citation44
Chemical grafting of chitosan is a process by which one or more species of blocks are connected as a side chain to the main chitosan chain, resulting in the formation of macromolecular copolymers with modified physical and chemical properties. The characteristics of the newly formed copolymer depend on length, number, and molecular structure of the side chain(s).Citation45 Chitosan might be grafted using radiation, free radical, or enzymatic and cationic copolymerization. Chitosan-poly(ethylene glycol) diacrylate and chitosan derivative with galactose groups have been produced using this technique by graft copolymerization of the macromonomer onto the chitosan backbone and chitosan amine group, respectively.Citation46,Citation47
Chitosan micro-/nanoparticles as drug delivery system
Among the novel drug delivery systems investigated, chitosan micro-/nanoparticles have offered great promise in oral, parenteral, topical, and nasal applications.Citation48–Citation50 In these systems, the drug is either confined and surrounded by a polymeric membrane or is uniformly dispersed in the polymer matrix. The size and surface characteristics of the prepared particles play an important role in their transport across the biological cell membranes. These particles could be used to deliver the pharmaceutically active agent in a controlled and, sometimes, site-specific manner. The mucoadhesive nature of chitosan renders the prepared particles the ability to improve both drug absorption and bioavailability because of extended drug contact with the mucosal layer and the high surface-to-volume ratio of nanoparticles that might also enhance this effect. Drug release at a specific site and for an extended period of time could also be achieved by mucoadhesion where chitosan adhere to specific mucosal surfaces in the body such as buccal, nasal, and vaginal cavities.Citation51–Citation53
Chitosan microparticles have shown varied applications in the delivery of a range of compounds owing to particle size reduction by micronization and their mucoadhesive properties. Dastan and TuranCitation54 developed chitosan–DNA microparticles and reported a sustained-release profile of DNA from the prepared microparticles with a potential transfer of the DNA into Human embryonic kidney, Swiss 3T3, and HeLa cell lines. Another research group prepared chitosan–DNA microparticles that have demonstrated suitable in vitro characteristics for mucosal vaccination in simulated intestinal fluid and simulated gastric fluid.Citation55 Their role in protein and peptide delivery has been illustrated by Chua et alCitation56 after developing luteinizing hormone-releasing hormone chitosan-based microparticles as a vaccine delivery vehicle. Successful delivery of hormones by these particles extends their application for induction of immunity against some tumor antigens and microorganisms such as bacteria and viruses.Citation56 Insulin delivery via the nasal route using chitosan microparticles was demonstrated by Varshosaz et alCitation57 who showed that the insulin-loaded microspheres exhibited a 67% lowering in the blood glucose level compared to insulin administered by the intravenous route (with absolute insulin bioavailability of 44%). Many research groups have thus described the applicability of chitosan microparticles in drug delivery. Encapsulation of diclofenac sodium,Citation58 5-flurouracil,Citation59 cisplatin,Citation60 felodipine,Citation61 and hydroquinoneCitation62 into these carriers has been reported, and the designed microparticles generally exhibited a controlled-release effect. Chitosan magnetic microparticles (CMM) are a special class of chitosan microparticles that have been developed and find wide applications in the delivery of anticancer drugs or radionuclide atoms to a targeted tissueCitation63 by binding the drug or the radioactive atom to a magnetic compound, which is then injected into the blood and stopped at the targeted tissue by an externally applied magnetic field.Citation64
One drawback of drug-loaded polymeric microspheres is the undesirable drug burst effect that is usually observed, which limits their application as a drug delivery system.Citation65–Citation67 Coating of chitosan microspheres by another polymer such as alginate has been reported to enhance the drug encapsulation efficiency, increase the stability, and reduce the burst release of the incorporated therapeutic agent. As the hydrophilic character and solubility of chitosan is increased in acidic medium, the effect that leads to limit the ability of chitosan to control the release and affect stability of loaded drugs, coating the surface with acid-resistant polymer such as sodium alginate might overcome these drawbacks. Alginate, an anionic polysaccharide polymer, electrostatically interacts with the cationic chitosan and produces a polyelectrolyte complex that enhances chitosan characters. This effect was reported by Li et alCitation68 for alginate-coated chitosan microspheres employed for vaccine delivery. Another research group illustrated the success of this approach in enhancing the encapsulation of lisinopril.Citation69 Alginate-coated chitosan microspheres have also been reported as good vectors for oral administration of protein and peptides, such as insulin with enhanced insulin stability, sustained-release effect, and ability to reduce the blood glucose level in diabetic rats for more than 60 hours following oral administration.Citation70 Another way to decrease the burst effect and enhance stability in pure chitosan microspheres was achieved by formation of polymer/layered silicate composites.Citation71 Attapulgite, a nanosized silicate clay naturally occurring polymer used in drug delivery,Citation72 has been introduced into cross-linked diclofenac sodium chitosan microspheres in which the prepared chitosan/attapulgite hybrid microspheres exhibited narrow size distribution and minimum drug release in the simulated gastric fluid.Citation73
Drugs of limited aqueous solubility and patients suffering from instability in the GIT show poor absorption, low bioavailability, and bad therapeutic responses.Citation74,Citation75 Polymeric nanoparticles have been reported to conquer these problems.Citation76,Citation77 Development of chitosan nanoparticles has maximized the benefits of chitosan as a polymeric drug carrier by enhancing drug aqueous solubility, systemic absorption, bioavailability, and stability. Curcumin is a common example of these drugs, and the preparation of curcumin-loaded chitosan nanoparticles has been reported to enhance the drug solubility and stability in the GIT.Citation78 Facilitation of the transmucosal delivery of two hydrophobic drugs, triclosan and furosemide, has been achieved by developing drug-loaded chitosan nanoparticles.Citation79 Low-molecular-weight heparin (LMWH) has been loaded into chitosan nanoparticles and showed improved oral absorption and relative bioavailability of 517% when compared to a solution of LMWH.Citation80 Recently, chitosan nanotherapeutics have received great attention in the field of oncology because of enhanced tumor targeting, ability to load different hydrophobic anticancer drugs, and the ability to control the anticancer drug-release rate.Citation81,Citation82 Chitosan-loaded paclitaxel nanoparticles illustrated excellent tumor-homing ability after systemic administration into SCC7 tumor-bearing mice.Citation83 Moreover, an augmented systemic absorption of some peptides has been illustrated after formulation of these peptides as chitosan nanocarriers.Citation84–Citation86 Protein/siRNA-loaded chitosan nanoparticles have been prepared and have showed 98% entrapment efficiency with adequate stability.Citation87 Bovine serum albumin has been encapsulated into nanoparticles of chitosan, and the prepared particles showed acceptable physicochemical properties as indicated by the size range (200–580 nm) and high positive zeta potential.Citation88 Genetic immunization using chitosan nanoparticles-loaded plasmid DNA was investigated, and results showed measurable and quantifiable levels of gene expression and considerable antigen titer.Citation89 Other workers investigated the potential of these nanoparticles as carriers for antigens by using recombinant hepatitis B surface antigen, in which the nanoparticles were stable, able to associate efficiently with the antigen (>60%), kept the antigenic epitope intact, released the antigen in a controlled-release manner, and illustrated induction of immunization through substantial anti-HBsAg IgG levels.Citation90 Chitosan nanoparticles for oral delivery of insulin have been successfully formulated, and these demonstrated improved interaction with the intestinal epithelium, enhanced in vitro as well as in vivo absorption, and acceptable insulin bioavailability.Citation86–Citation91 As previously illustrated during the discussion of chitosan microparticles, magnetic nanosized chitosan particles were also developed as a special type of chitosan nanoparticles and showed sustained drug release with minimal toxicity. This behavior was noticed after preparation of camptothecin magnetic chitosan nanoparticles in which polyethylene glycol was coupled onto the prepared magnetic nanoparticles to increase their biocompatibility.Citation92
As has been mentioned during the discussion of microparticles, the initial drug burst-release effect was still a problem with these systems. It has been reported that this weakness still presents some difficulties with the chitosan nanoparticulate system.Citation88 Coating of the nanoparticles can diminish this problem. Poly-lactic-co-glycolic acid was used as a polymeric coating material for dexamethasone chitosan nanoparticles in cultured cells, with a considerable modulation in drug release as well as controlled drug delivery.Citation93 Alginate has been also used as a coating material for chitosan nanoparticles. Alginate-coated chitosan nanoparticles loaded with low-molecular-weight heparin, enoxaprin, were synthesized and exhibited modified drug-release profile with overall significant increase in oral bioavailability when compared to drug solution and approximately a 60% decrease in thrombus formation in experimental rat venous thrombosis model.Citation94
Methods of preparation
Top–down and bottom–up are the two techniques used to develop micro- and nanoparticle drug carriers. In the latter, the particulate system is prepared from a state of molecular dispersion type and is allowed to associate with subsequent formation of solid particles. Bottom–up techniques, therefore, seek to arrange smaller components into assemblies of complex structure, While the former starts with large size materials and breaks these down into smaller particles. Conventional nanoparticle synthesis usually depends on bottom–up techniques.Citation95
Different methods have been utilized in the preparation of chitosan micro- and nanoparticles. The particle size, stability of the active constituent and the final product, residual toxicity present in the final product, and the kinetic of the drug-release profile are factors that should be considered during selection of the method.Citation13 During the preparation of chitosan particulate systems, the size of the prepared particles is greatly dependent on chitosan molecular weight, chitosan chemical structure, particularly the degree of deacetylation, and on the method of preparation. As a general rule, higher molecular weight chitosan produces larger-size particles.Citation96 Different methods are available to prepare chitosan micro-/nanoparticles in which the drug is mostly bound to chitosan by hydrogen bonding, electrostatic interaction, or hydrophobic linkage. Generally, loading the therapeutic agent into chitosan micro-/nanoparticles may be achieved either during the preparation process or after the particles have been formed. In the former, the therapeutic agent is incorporated and embedded in the chitosan matrix, whereas in the latter the therapeutic agent is adsorbed on the particle surface. Usually, the aim is to achieve high entrapment efficiency, which could be accomplished by incorporation into the matrix, but the therapeutic agent could be affected by the preparation method, additives, etc.Citation13 Generally, selection of the method is greatly dependent on the nature of therapeutic agent and the type of device utilized in the delivery.Citation13 A list of methods used in the preparation of these particles is given in . All these methods involve the bottom–up production process, in which assembly of the dissolved molecules is achieved to form a definite micro- or nanoparticulate structure.Citation97
Table 1 Methods of preparation of chitosan micro/nanoparticles loaded with different pharmacotherapeutic agents
The techniques used in the preparation of chitosan micro-/nanoparticles loaded with thermosensitive or less stable substances such as proteins, peptides, hormones, vaccines, plasmid DNA, and antigens may be broadly classified into cross-linking techniques and drying techniques. Cross-linking could be achieved chemically or physically. The stability of these thermosensitive or less stable substances are strongly affected by the organic solvent and the cross-linking agent used, with the consequence of denaturation or chemical modification.Citation98 So, physical cross-linking and drying techniques, such as spray drying, are preferred and widely used for these substances. Recently, reverse micellar method has been introduced. These aforementioned techniques – cross-linking, drying, and reverse micellar – in addition to sieving and solvent evaporation were used in the preparation of other drugs of different pharmacotherapeutic groups. An insight on these methods is described in the following sections.
Cross-linking techniques
Physical cross-linking
The ionic cross-linking method is the most common among physical cross-linking techniques since the preparation procedure is simple, does not involve use of organic solvent or high temperature, and no chemical interaction is involved.Citation33,Citation99 These advantages make this method efficient and safe for production of thermosensitive therapeutic agents such as proteins, peptides, hormones, and vaccines loaded into chitosan particulate systems. Assembly and formation of the particles is achieved by ionic cross-linking between chitosan or one of its derivatives, being cationic in nature, and either negatively charged macromolecules or anionic cross-linking agents. Acidic solution of chitosan is prepared, and the ionic cross-linker is added dropwise along with stirring and sonication. If cross-linking is achieved by anionic cross-linkers such as sodium sulfate or tripolyphosphate (TPP), the process is called ionic gelation, whereas negatively charged polyelectrolyte macromolecules, such as cyclodextrin derivatives, dextran sulfate, and poly-γ-glutamic acid produce electrostatic polyelectrolyte complexes (PEC) of ionic cross-linking type.
Formulation of felodipine-loaded chitosan microparticles has been achieved by ionic gelation. The chitosan molecular weight and concentration, concentration of the cross-linking agent (TPP), and TPP pH have been reported to play an important role in the drug-release pattern.Citation100 Slower felodipine release was obtained from TPP solution of low pH and higher TPP concentration, and higher chitosan molecular weight and concentration. Triclosan and furosemide, two hydrophobic drugs, were loaded into chitosan nanoparticles by ionic cross-linking of chitosan with TPP, and the release profile of both drugs from the prepared nanoparticles was characterized by fast initial release followed by a controlled-release stage.Citation79 TPP is widely used to prepare chitosan nanoparticles that have been successfully employed as a carrier for proteins and antigens such as insulin,Citation91 tetanus toxoid,Citation101 albumin,Citation102 and influenza subunit antigen.Citation103 Chitosan-loaded interleukin-2 (IL-2) microparticles were prepared using sodium sulfate as the anionic cross-linker.Citation104
Complex coacervation is a process of liquid–liquid phase separation that occurs when two solutions containing oppositely charged ions are mixed, resulting in the formation of an ionic complex.Citation105 Plasmid DNA was successfully loaded into chitosan nanoparticles of size range 450–820 nm by this method, and the encapsulation was more than 90% for chitosan of high degree of deacetylation and the release was extended for 24 hours.Citation106 As chitosan is a cationic polymer that is soluble in acidic solution, it is also possible to precipitate chitosan from its aqueous solution by addition of alkali, which is the theoretical principle of the precipitation/coacervation method. The technique involves preparation of chitosan aqueous solution that is introduced through a nozzle into aqueous or hydroalcoholic solution of sodium hydroxide or ethanediamine by blowing or dropping. The precipitated chitosan microparticles are separated by filtration/centrifugation and finally washed with hot and cold water. A cross-linking agent may be added to increase the hardness of the obtained particles, which can extend the drug release. Prednisolone-loaded chitosan microspheres were prepared by the precipitation method and were able to enhance the transport of prednisolone across the epithelial barrier.Citation107 Complex coacervation, as already described, has been employed for the preparation of ketorolac tromethamine chitosan microspheres that controlled the drug release, while potentially improving patient compliance due to the decrease in dosing frequency.Citation108
PEC chitosan nanoparticles produced through ionic interaction with the negatively charged dextran sulfate and loaded with insulinCitation109 or vascular endothelial growth factor (VEGF)Citation110 was reported. In another study, poly-γ-glutamic acid has been used in the preparation of PEC chitosan nanoparticles, which reduced the transepithelial electrical resistance of Caco-2 cell monolayers.Citation111 Recently, self-assembled, electrostatic PEC chitosan particles have been developed for delivery of some proteins such as insulin, heparin, hyaluronan, and VEGF.Citation109,Citation112–Citation116 Self-assembly is achieved through electrostatic interaction between the cationic amino group of chitosan and the chemically modified N-anionic chitosan amines such as N-sulfated chitosan. Schatz et alCitation116 reported formulation of self-assembled chitosan nanoparticles by electrostatic interaction between the protonated amino residues of chitosan, being cationic, and the sulfate functions, being anionic, and the nanoparticles were stabilized by an excess of surface sulfate groups.
Thermal cross-linking is another way of physical cross-linking in which citric acid, a commonly used cross-linking agent in this method, is added to an aqueous chitosan acidic solution in a constant molar ratio between citric acid and chitosan. The mixture is cooled to 0°C and added while stirring to an oily phase, such as corn oil or sesame oil, previously cooled to 0°C. The emulsion is thermally cross-linked at 120°C, and the obtained microspheres are filtered, washed, and finally dried. Indomethacin-loaded chitosan microspheres were prepared by this method.Citation117
Chemical cross-linking
In this method, chitosan micro-/nanoparticles are formed through a chemical interaction between a cross-linking agent and the primary amino groups of chitosan. Common cross-linkers are glutaraldehyde, p-phthaldehyde, ascorbyl palmitate, and dehydroascorbyl palmitate. Chitosan microparticles prepared using ascorbyl and dehydroascorbyl palmitate have lower toxicity and high insulin-loading efficiency, and release insulin in a controlled-release manner, for approximately 80 hours, when compared to that produced using di-aldehydes, glutaraldehyde, and p-phthaldehyde as chemical cross-linkers.Citation118 Chemical cross-linking may take place either through one or two steps. The procedure involves formation of a water/oil (w/o) emulsion in which chitosan and the therapeutic agent are in the aqueous phase that is emulsified into external immiscible solvent. The cross-linking agent is gradually added and, finally, the prepared particles are separated and washed with appropriate solvent to yield the desired particle.Citation119 Ascorbyl palmitate and dehydroascorbyl palmitate were used as a chemical cross-linking agent during the preparation of chitosan-loaded insulin microparticles in an external mineral oil phase.Citation118 Formation of these particles in the internal aqueous phase of w/o emulsion improves the entrapment of the therapeutic agent as the external oil phase prevents the escaping of the therapeutic agent.Citation120 An illustration of the steps involved in the production of chitosan particles by this technique is shown in .
Figure 2 Schematic representation of production of chitosan micro/nanoparticles by chemical cross-linking.
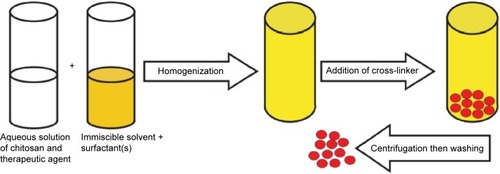
Some additives may be added to enhance stability and encapsulation efficiency of the therapeutic agent or decrease its leakage. Gelatin has been added to the aqueous phase to enhance insulin stability and encapsulation.Citation121 Preparation of chitosan microparticles containing bovine serum albumin (BSA) has been reported by this method.Citation122
Drugs of different pharmacotherapeutic groups such as nonsteroidal anti-inflammatory, antineoplastic, antifungal, antiseizure, opioid, methyl xanthenes, angiotensin-converting enzyme inhibitor, and bone-related drugs have been loaded into chitosan microparticles.Citation13 Glutraldehyde, glutraldehyde extracted in toluene, and ascorbyl palmitate are commonly used cross-linkers, while liquid paraffin or a mixture of mineral oil/petroleum ether is used as external oil phase. Common examples of drugs in each pharmacotherapeutic group are listed in . Recently, Ahmed and El-SayCitation120 have developed rabeprazole chitosan nanoparticles within a w/o nanoemulsion by emulsifying the aqueous phase into paraffin oil containing a surfactant mixture of spans and tweens. The optimized nanoparticles showed a nanosize range, 120±32 nm, and were spherical in shape as indicated by the scanning electron micrograph ().
Figure 3 Scanning electron micrograph of chitosan nanoparticles produced using nanoemulsion internal cross-linking technique.
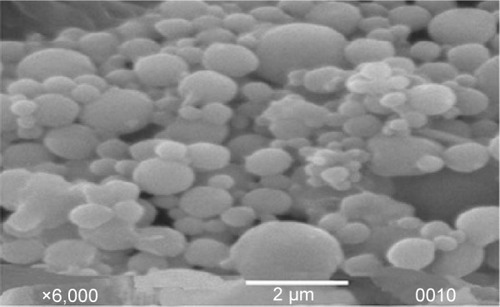
Recently, emulsion droplet coalescence technique that uses the principles of emulsion cross-linking and precipitation has been developed. Two separate emulsions are prepared using two different aqueous phases in paraffin oil. The aqueous phase of the first emulsion consists of chitosan and the studied anionic drug, while that of the second emulsion is composed of chitosan in sodium hydroxide solution. The two emulsions are mixed under high-speed stirring, which allows droplets of each emulsion to coalesce randomly, and the newly formed droplets are precipitated.Citation123 The advantage of this method over the emulsion cross-linking method lies in that it allows an electrostatic interaction between the free amino groups of chitosan and the used anionic drug that permits higher drug loading. This mechanism was not possible in the emulsion cross-linking technique owing to the blocking of the chitosan amino groups by the cross-linker. Gadopentetic acid, a bivalent anionic drug, was loaded into a chitosan nanoparticle by this method.Citation123
Drying techniques
Drying is the process of water/solvent removal from liquids, solids, or semisolids by evaporation, where the vapor that is produced is removed by vacuum. Hot air, microwave, spray drying, freeze drying, supercritical drying, and natural air drying represent the general methods of drying. Spray drying and supercritical drying are the most commonly used in the preparation of chitosan particulate systems as they are simple, adapt well with the incorporated drug, are easily scaled up, and have the ability to produce particles of different size and high stability.
Spray drying
Spray drying has been reported for manufacturing of granules and dry powders from drug-excipient mixtures either in a solution or suspension.Citation124 The expediency of this method extended to include preparation of microparticles from different polymeric materials loaded with protein,Citation125 vaccine antigens,Citation126 and drugs.Citation127 Spray drying offers an easy, efficient, one-step, and protein-friendly method for protein-loaded chitosan micro-/nanoparticles. The basic principle of spray drying relies on using a stream of hot air to dry atomized droplets. As illustrated in , the process involves preparation of aqueous chitosan–protein solution that is sprayed through a nozzle into a drying chamber to get the desired particles. BSA and salmon calcitonin are examples of proteins loaded into chitosan microparticles by this method.Citation128,Citation129 Drugs from different pharmacotherapeutic groups have been loaded into chitosan microparticles and studied for their effect as potential drug carrier. Chitosan has been used as a micro-/nanocarrier for corticosteroid, antimicrobial, proton pump inhibitor, NSAID, and antiemetic drugs that have been formulated by this method.Citation13 Examples of drugs loaded into chitosan particles by this method are listed in . The prepared particles were spherical, of smooth surface and narrow size distribution and showed good drug stability and high drug-entrapment efficiency. Other advantages of this method are the possibility of surface cross-linking and coating the spheres during the manufacturing steps, which could enhance stability and control drug release. He et alCitation130 reported on the preparation of nizatidine, cimetidine, and famotidine cross-linked and un-cross-linked chitosan microspheres using the spray drying technique, and ethyl cellulose-coated chitosan microspheres–loaded ergocalciferol were successfully produced by the same method.Citation131 The size of the prepared particles is influenced by spray flow rate, extent of cross-linking, and inlet air flow rate. Large particles were produced using high spray flow rate from large-sized nozzles and when there was a decrease in the extent of cross-linking, while smaller particle size was obtained at greater airflow rates and not by the inlet air temperature being between 140°C and 180°C. Most recently, vildagliptin nanospheres, of smooth surface, were prepared using the spray-drying technique. The prepared nanospheres were characterized by high drug content and nanospheres percentage yield of 76.2%±4.6% and 83%±2%, respectively.Citation132
Supercritical drying
This is a process of drying the liquid in a substance by converting it into a gas without crossing the phase boundary between the liquid and gas and without affecting the substance nanostructure network. A critical point, of specific temperature and pressure, is a characteristic feature of pure substance at which gaseous and liquid states of the substance has liquid-like viscosity and density, and so are difficult to differentiate. Substances possessing this property easily penetrate into substances like a gas and dissolve materials like a liquid.Citation133 Some fluids such as carbon dioxide, Freon, and nitrous oxide are suitable for drying by this process, and they can easily penetrate into the material like gas and dissolve the substance similarly as liquid.Citation133 Chitosan microparticles loaded with insulin have been successfully prepared using supercritical fluid drying method suitable for inhalation.Citation134 This method is fast, economical, and could produce much smaller particles suitable for delivery by the inhalational route.Citation135,Citation136
Reverse micellar method
Reverse micelles are water droplets in the nanometer size (1–10 nm) dispersed in organic solvent because of the effect of surfactants.Citation137 The aqueous core of these nanosized droplets can be used as a reactor to prepare nanoparticles. The preparation method includes preparation of aqueous drug–chitosan solution that is to be added under stirring to a mixture of organic solvent and surfactant molecules. A cross-linking agent is added, and the mixture is left on the stirrer overnight for complete cross-linking. The organic solvent is then evaporated to get a dried mass. To remove the surfactant, the obtained dried mass is dispersed in water and a suitable salt is added to precipitate the surfactant out, and finally the drug-loaded chitosan nanoparticles are recovered by centrifugation. represents the steps involved in this method. The reverse micellar method has been used to prepare BSA-loaded chitosan nanoparticles in a size range of 80–180 nm.Citation138 The doxorubicin dextran complex was loaded into chitosan nanoparticles using n-hexane as organic solvent, sodium bis(ethyl hexyl) sulfosuccinate as surfactant, and glutraldehyde as cross-linker. The prepared nanoparticles were of 100±10 nm size and enhanced the permeability and retention effect of doxorubicin, which was reflected in improving the drug therapeutic effect and reduction of the side effect in solid tumor.Citation139
Sieving method
Preparation of chitosan microparticles by this method involves formation of 4% chitosan hydrogel containing the drug, after which a cross-linking agent such as glutraldehyde is added to produce a cross-linked chitosan hydrogel that is passed through a sieve of definite size to get the drug-loaded microparticles. The excess glutraldehyde is removed by washing the obtained microparticles with 0.1 N sodium hydroxide, and then the particles are dried in an oven at 40°C.Citation140 Clozapine microparticles of the size range 543–698 μm showed an extended drug release up to 12 hours when prepared by this method.
Solvent evaporation
In this method, a polymeric drug solution in a volatile solvent such as acetone is prepared and emulsified into a nonaqueous phase such as liquid paraffin. The mixture is kept under stirring until complete evaporation of the solvent, and the formed microspheres are filtered, washed with suitable solvent such as petroleum ether, and finally dried. Drying is usually achieved by air or under vacuum. Metformin-loaded chitosan microspheres were successfully obtained using this procedure.Citation141
In general, the entrapment efficiency and particle size of the prepared particulate systems are affected by various processing and formulation parameters such as chitosan concentration, chitosan molecular weight, type of the chitosan derivative, nature of the drug, initial drug concentration used, drug–polymer ratio, nature of the cross-linking agent, type and concentration of the surfactant, and stirring speed. Optimizing these parameters using suitable optimization software is helpful in achieving the desired particles.
Characterization of chitosan micro-/nanoparticles
After preparation of the chitosan particulate system, parameters such as particle size analysis, zeta potential, morphological and surface characteristics, drug content, encapsulation and loading efficiency, specific surface properties, in vitro release, and characterization of the targeting action are analyzed.
Perhaps the most important physical property of particulate systems is particle size. Measurement of this property is critical to the success of technique employed during manufacturing. Malvern has developed instruments for all types of particle size analysis and characterization of particles ranging from the subnanometer to millimeter range. lists the technology and particle size instruments suitable for each measuring particle size. Information about the technology employed in each instrument could be found in the website for Malvern.Citation142 In addition to the technologies listed in , photon correlation spectroscopy is a technique widely used to characterize the nanoparticles’ particle size.
Table 2 The technology, instrument, and particle size range suitable for each particle size
Morphology, particle size distribution, and mean particle diameter characterization of the prepared microparticles could be carried out using optical microscope of suitable magnification power. A randomly chosen dried microparticles sample is examined under the eyepiece of the microscope that was calibrated so that the unit in the eyepiece micrometer is equal to a definite size in μm. The individual particle size is calculated, from which the size distribution and mean particle diameter are determined. For microparticles of size greater than 90 μm, the micromeritic properties are studied by determining the particle size distribution, geometric mean diameter, and geometric standard deviation.Citation143 The particle size distribution is determined using the sieve analysis. To do so, a definite weight of microparticles is placed on top of a standard set of sieves having a determined size range (eg, 710–90 μm) and is shaken for a specified time, usually 10 minutes, using an electric shaker. After the shaking period, the weight of the microparticles retained on each sieve is collected and weighed. The mean particle size is calculated using the following equation:
The geometric mean diameter and geometric standard deviation are estimated from the probability scale plot obtained by plotting the logarithm of the particle size against the cumulative percent frequency.
When microparticles are of very low range, 5–10 μm, sieving is not an accurate way to analyze particle size distribution. Techniques such as Feret, Martin, Krumbein, or Heywood diameter are very helpful in this case. Feret’s diameter, the most common among these, analyzes the particle size and size distribution through measuring the distance between two parallel tangential lines on the particle along a specified direction.Citation144
For nanoparticulate system, the morphological and surface characteristic of the prepared particles are usually evaluated using a scanning electron microscope (SEM), which provides image of the sample and information about the sample’s surface through scanning it with a focused beam of electrons that interact with the sample atoms and produce various signals that are detected using a highly sensitive detector. Unlike the optical microscope and telescope, the detector is not a camera and so has no diffraction limit for resolution. Samples of the dried particles are mounted in a metal stub and coated with a thin film of gold, or other conducing material, under vacuum, before the sample is observed by SEM, as observed in . SEM also provides information about the surface smoothness, roughness, or porous structure, all parameters that might be helpful on the interpretation of subsequent analyses, such as the dissolution behavior or in vivo responses.Citation145 SEM has been used to study the shape and occurrence of chitosan aggregatesCitation146 and also for studying alginate/chitosan nanoparticles.Citation147 Transmission electron microscope (TEM) was also used to examine the shape of doxorubicin-loaded chitosan nanoparticles in which a drop of the prepared nanoparticles was deposited onto a formvar-coated copper grid and was vacuum dried before TEM specification.Citation148
Zeta potential, which reflects the surface electric charges of the particles, are determined using zeta potential measurement, ZetaMeter (Staunton, VA, USA), which applies the principle of electrophoresis. An electric field is applied across the dispersed particles in which the particles will migrate to the oppositely charged electrode with a velocity that is proportional to the value of the zeta potential. The velocity of migration is measured using the technique of Laser Doppler Anemometer. The magnitude of zeta potential reflects the degree of electrostatic repulsion between charged particles. For dispersion system, high degree of zeta potential, negative or positive, indicates resistance of the particles to aggregation, and this denotes an apparently stable system. Rodrigues et alCitation149 reported the use of laser Doppler anemometry in the measurement of zeta potential for chitosan/carrageenan nanoparticles.
Drug content, encapsulation efficiency, loading efficiency, and micro-/nanoparticles yield are other characteristics that should be calculated for the prepared micro-/nanoparticles before therapeutic administration. First, the drug content has to be determined using a suitable drug analytical method such as spectrophotometry or high-performance liquid chromatography (HPLC). Then, drug encapsulation efficiency and loading efficiency are determined by the following equations:
Micro-/nanoparticles yield, however, is calculated from the following equation;
For microparticles, the drug content is determined after crushing of the microparticles and extracting the drug in suitable solvent or after complete drug release from the microparticles that was suspended in water or buffer and left shaking for 24 or 48 hours. For nanoparticles, usually, the obtained dispersion of the nanoparticulate system is centrifuged at high speed, up to 100,000 rpm according to the nanoparticle size, and the amount of the drug in the supernatant is analyzed, after which the drug content is determined indirectly.
The specific surface properties of the prepared particulate system could be examined using X-ray photoelectron spectroscopy (XPS) and time-of-flight secondary ion mass spectrometry (ToF-SIMS). Both provide information about the elements from which the particles originate, chemical bonding, and detailed molecular information with high sensitivity. The former identifies the size-dependent distributions and the chemical states of the elements, while the latter provides detailed surface and near-surface composition analysis, and so characterization and composition of the surface chemistry of the particles are illustrated. Casettari et alCitation150 studied the surface properties of PLGA/chitosan microparticles using XPS and ToF-SIMS to identify the presence of chitosan on the prepared particles surface. Another research group used XPS and ToF-SIMS to study the surface analysis and matrix chemical composition of chitosan/carrageenan nanoparticle.Citation149
Deformability of the prepared nanoparticles is measured by a simple filtration test method. A suspension of nanoparticles labeled with a fluorescent dye is prepared in phosphate buffer and passed through syringe filter membranes of decreasing pore sizes (0.8–0.2 μm). The filtrate is observed for the presence of the dye using near-infrared fluorescent (NIRF) images, and the stability of the particles is confirmed by measuring the change in the molecular weight of the sample after incubation in PBS with or without 10% weight of serum at 37°C for 6 hours.Citation151
In vitro release test is used to determine the drug release profile from the prepared chitosan micro-/nanoparticles. The mechanism of drug release from these particles is related to 1) drug present on the particle surface, 2) drug diffusion from chitosan matrix, and 3) chitosan degradation and erosion.Citation13 Generally, the drug release is diffusion controlled and follows the Higushi model, depending on the chitosan molecular weight, particle size and density, degree of cross-linking, excipients, polarity, pH, and the presence of enzymes in the dissolution media.Citation13,Citation19 The diffusion mechanism of drug release involves penetration of water into the polymeric particulate system, which results in swelling of the matrix, then formation of rubbery polymeric matrix, and, finally, diffusion of the drug from the produced swollen rubbery polymeric matrix.Citation13 Deviation of the drug release from diffusion to zero order could be achieved from large-sized particles, usually in the microsize range, prepared using higher chitosan concentration at specific pH medium. Indomethacin chitosan microspheres exhibited this behavior at pH 7.4.Citation117
Recently, characterization of the targeting action of drug-loaded chitosan nanoparticles particularly to the cancer cell has been reported. Kim et alCitation151 developed a paclitaxel-loaded chitosan nanoparticles containing Cy5.5, a near-infrared fluorescent (NIRF) dye, and evaluated their cytotoxicity on a culture of murine squamous carcinoma cells, as well as their targeting ability by performing in vivo and ex vivo NIRF imaging using a CCD camera image station in which the NIRF emission data of the tumor and major organs were recorded.
Potential applications of chitosan micro-/nanoparticles
Drugs of different classes such as anticancer, anti-inflammatory, cardiovascular, antibiotics, antihistaminic, antithrombic, steroids, antiosteoporotic, antidiabetics, CNS acting, opioid analgesics, corticosteroids, antihyperlipidemic, antiemetics, proton pump inhibitors, enzymes, toxoids, DNA, hormones, growth factors, proteins, and amino acids have been loaded or encapsulated into chitosan particulate systems.Citation22 Apart from the mentioned classes of drugs, chitosan/chitosan derivative nanoparticles have been used as a potential carrier for vaccines and as a nonviral vector for gene delivery. Both local and systemic immune responses have been produced following administration of vaccine-loaded chitosan nanoparticles. Immune response could be achieved by induction of antibodies;Citation152 production of inflammatory mediators such as cytokines, interleukins, colony-stimulating factors, and granulocyte-macrophage colony-stimulating factor;Citation153 enhancement of the capability of the antigen presentation to dendritic cells and stimulation of greater allogeneic T-cell proliferation;Citation154 and increasing the retention of the administered vaccine at the injection site, which results in the induction of transient cellular expansion in the draining lymph nodes.Citation155 Influenza vaccine, Cholera toxins, hepatitis B surface protein, Streptococcus equi bacterial extract containing antigen protein, and β-galactosidase are examples of vaccine antigens that have been loaded into these nanoparticles.Citation155–Citation159 The important feature in chitosan, particularly when formulated in particulate form and administered through parenteral or mucosal routes, is that it can be taken up and then processed by antigen-presenting cell, the effect that initiates an immune response against the loaded antigen.Citation160 The role of these particles in gene delivery has sparked the use of these as a nonviral gene delivery vector. Delivery of genes by viruses suffers from low transfection, low amount of the gene introduced into the mammalian cell, and cell toxicity.Citation161 These drawbacks could be overcome by delivering the gene in chitosan particles, which act as a nonviral carrier.Citation162,Citation163 Treatment of diseases, caused by a genetic defect, is the target for gene-loaded chitosan nanoparticles. Nanoparticles loaded with siRNA, especially when prepared using naturally occurring, positively charged chitosan of high molecular weight and degree of acetylation exhibited higher stability, easy adsorption onto the cell surface, and protection and maintenance of the activity of the loaded siRNA gene, resulting in enhanced effectiveness of gene silencing.Citation163 Nevertheless, the gene transfection efficacy remains low and needs further improvement.
Chitosan and its derivatives have been studied as an efficient nonviral vector involved in plasmid DNA delivery.Citation157–Citation164 The chemically modified chitosan derivatives have been reported to improve chitosan transfection efficiency without affecting its biodegradability and biocompatibility. Nanoparticles prepared with chitosan of a specific degree of deacetylation and ligand-mediated cell uptake chitosan nanoparticles have illustrated enhancement in cell internalization and transfection efficiency.Citation164–Citation167 Galactose, transferrin, folate, and mannose are common cell-specific ligands that promote cell uptake through receptor-mediated endocytosis. Transferrin receptor is a universal ligand as it is found on many mammalian cells.Citation165 Folate is overexpressed on macrophage surface as well as many human cancer cell surfaces,Citation166 whereas galactose ligand modification has been shown to target HepG2 cells through its interaction with asialoglycoprotein receptors.Citation164 Plasmid DNA–loaded chitosan nanoparticles were successfully formulated and showed transgene expression that was nearly equivalent to those of the two marketed products, Lipofectamine™ and FuGENE® 6.Citation167 Mansouri et alCitation168 tried to improve the rate of gene transfection by formulation of folic acid–chitosan–DNA nanoparticles. Incorporation of folic acid did not affect the properties of the particles and has promoted internalization of DNA into the cell through the membrane receptors both in vitro and in vivo. The incorporation of DNA into the nanoparticles permitted the preservation of the molecule, which was demonstrated to be intact within the carrier. Lower cytotoxicity against HEK 293 cell and good condensation of the loaded DNA were also observed, eliciting the potential of this system as a nonviral DNA vector.
The most important benefit of chitosan modification is the active targeting of the loaded therapeutic agent, which could be accomplished through chemical modification. Self-assembled chitosan–doxorubicin conjugate was prepared using succinic anhydride as a cross-linker, then the monoclonal antibody, trastuzumab, was conjugated to the chitosan–doxorubin conjugate nanoparticles that showed enhanced drug targeting by selective uptake of doxorubicin by Her2+ cancer cells when compared to either unmodified chitosan nanoparticles or free drug.Citation169 N-Caproyl chitosan nanoparticles, whose surface was chemically modified with glycyrrhizin by acylation of the chitosan amino group, were synthesized and effectively delivered in a targeted manner the chemotherapeutic agent, adriamycin, to the hepatocytes.Citation170 The hydrophobic moiety, cholanic acid, was used to modify the nanosized cisplatin-loaded glycol chitosan drug delivery system that released the drug in a controlled manner for 1 week, and the prepared system showed a tumor-targeting ability in tumor-bearing mice with higher antitumor effect and lower side effects when compared to free cisplatin.Citation171 The same finding was reported for the anticancer agent camptothecin, using the same system, glycol chitosan, in human breast cancer cells.Citation172 Avidin and biotin were used to form a complex with chitosan during the preparation of resveratrol chitosan nanoparticles to target the liver and hepatoma cells, the effect which results in enhancing the drug antioxidant and anticancer activity and minimizing the toxicity and side effects.Citation173
According to the route of administration, considerable researches have focused on the application of chitosan micro-/nanoparticles as a potential drug delivery system for parenteral and nonparenteral routes, such as oral, nasal, ocular, pulmonary, topical, and transdermal. The research findings of this review are illustrated in the following sections.
Parenteral route of administration
In practice, parenteral administration refers to introduction into the body of product(s) that are implanted, injected, or infused into blood vessels, tissue spaces, or body compartments. From the site of administration, the drug is then transported to the site of action. Since the administration procedure involves puncture or incision of the body, parenteral route is considered an invasive route of drug administration. Intradermal (ID), subcutaneous (SC), intramuscular (IM), intravenous (IV), and intra-arterial are the common routes of parenteral administration. shows these routes.
Figure 6 Structure of the skin and subcutaneous layers and common routes of parenteral drug administration.
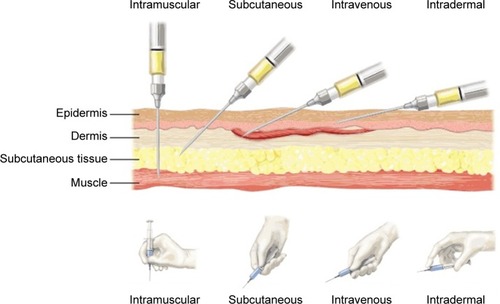
The size, surface charges, and hydrophilic/hydrophobic nature of the prepared particles control the IV parenteral administration and biodistribution.Citation174 Particles in the nanosize range are easily administered by IV route, because the smallest body microvessels are in the range 5–10 μm in diameter. Following IV administration of nanoparticles, the reticuloendothelial system (RES) in the spleen, lung, liver, and bone marrow entraps particles greater than 100 nm, whereas those of smaller size are characterized by prolonged circulation time.Citation175 Although many attempts have been made to illustrate the effect of nanoparticles’ surface charges on pharmacokinetics and tissue distribution, the controversy remains, and no clear interpretation has been reported. Hydrophobic particles easily penetrate into the tissues, which is not the case for hydrophilic ones. On the contrary, nanoparticles that are hydrophilic, even through coating with PEG or surfactants of nonionic type, and additionally, smaller than 100 nm, will bypass the phagocytosis performed by macrophages. Coating the prepared particles by PEG – PEGylation – has been reported to prolong the circulation time and avoid entrapment in the RES. Several attempts have been studied to use other polymers such as poloxamers, polyvinyl alcohol, and polysaccharides, yet PEGylation is still the effective and widely used technique.Citation176 There are two types of conformation for the process of PEG covering the nanoparticle surface – mushroom conformation at low PEGylation and brush conformation, which is formed when PEGylation is increased to a certain high level. The latter is the ideal mode to protect nanoparticles from serum absorption. PEGylation reduces opsonization, the process of binding of a specific serum protein to a substance that facilitates uptake of this serum protein substance–binding complex by RES, and so prevents recognition of the nanoparticles by the cells of the RES and allows nanoparticles to remain in the blood pool.Citation177 In addition to the IV route, but still in the parenteral route, nanoparticles are possibly administered by the subcutaneous and intramuscular routes.
Different drugs in the form of chitosan particles have been administered by the parenteral route and showed enhanced pharmacological effects and reduction in systemic toxicity. IV administration of doxorubicin chitosan nanoparticles illustrated marked regression in tumor growth and improved survival rate in experimental animal.Citation175 Patel et alCitation178 studied the pharmacokinetic and tissue distribution of doxorubicin chitosan nanoparticles after IV injection and reported enhanced drug distribution to the RES and lower drug systemic toxicity and cardiotoxicity. Paclitaxel was successfully loaded into modified chitosan carrier that has been administered by IV route, and this chitosan carrier system was reported to be less toxic and was characterized by increased tolerated dose when compared to commercial drug formulation.Citation179
Nonparenteral routes of administration
Nonparenteral routes of drug administration have tremendous advantages over injections. These routes represent alternatives for those drugs that cannot be delivered or for patients suffering problems associated with parenteral administration. Variation of serum drug concentration and high plasma concentration, problems often noticed with IM and IV administration, can be overcome by long-term transdermal delivery system. Bypassing the hepatic first-pass metabolism and prevention of the GIT degradation are advantages of the transdermal and transmucosal systems. Intranasal, ocular, buccal/sublingual, pulmonary, topical, and transdermal routes are the most promising, noninvasive systemic delivery options.
Oral route
Drugs administered orally must survive different pH and numerous GIT secretions such as potentially degrading enzymes. The process of oral drug absorption involves transport across the GIT membrane via passive diffusion, carrier-mediated transport, or pinocytosis. Carrier-mediated transport may be active transport or facilitated diffusion. In general, the process is affected by some GIT physiological factors, although physicochemical and formulation factors related to the administered drug and dosage account for some effects. Surface area of the absorption site, pH of the GIT fluids, blood perfusion, natural GIT secretions, and gastric emptying rate are the most important physiological factors. The oral mucosa is characterized by a thin epithelium and rich vascularity, which is a good place for buccal and sublingual administration. Although the stomach has a large epithelial surface, its thick mucous membrane, the effect of the gastric juice and food content on the administered drug, and gastric emptying rate may affect or even limit drug absorption. Perhaps the most important site for drug absorption in the GIT is the small intestine; because of its large surface area, high blood perfusion, its membrane being more permeable than that of the stomach, and wide pH range.Citation180
Drug delivery via the oral route is considered the easiest and the most convenient for patients. Therefore, great attention has been focused on this route for the enhancement of the oral bioavailability, particularly for those drugs presenting GIT problems. The mucoadhesive nature, protection of labile drugs from GIT enzymatic degradation, and the enhancement of absorption of the administered therapeutic agent without damaging the biological system, all result in prospective applications of the chitosan micro-/nanoparticles as oral delivery systems. Chitosan micro-/nanoparticles demonstrated benefits in the treatment of both local GIT and systemic diseases. Intestinal disinfection, suppression of Helicobacter pylori, and treatment of ulcerative colitis are examples of local GIT conditions following treatment with chitosan particles loaded with antibiotics. Amoxicillin and clarithromycin have been loaded into chitosan particles and showed local GIT effect for eradication of H. pylori.Citation181,Citation182 The mucoadhesive properties of chitosan have resulted in prolonged delivery of acyclovir, an antiviral agent, with considerable oral bioavailability improvement due to enhanced retention of the drug in the upper GIT following administration of acyclovir chitosan microspheres.Citation183 Protection against GIT degradation, enhancement of bioadhesion, and improvement of oral bioavailability of insulin have also been reported following encapsulation into chitosan microspheres.Citation184
Drug colon targeting is useful for treatment of some diseases such as irritable bowel syndrome, ulcerative colitis, and Crohn’s disease. Chitosan-based delivery systems have been reported to protect the loaded drugs, such as insulin, from degradation in the upper GIT and, therefore, release these drugs at the colon because of degradation of the chitosan glycosidic linkage by the specific microflora of the colon.Citation185 Chitosan microspheres coated with cellulose acetate butyrate and loaded with 5-aminosalicylic acid (5-ASA) have been developed for treatment of ulcerative colitis in which the bioadhesion character of the microspheres was significantly increased by increasing the molecular weight of chitosan and decreasing the coating content.Citation186 Furthermore, localization of 5-ASA in the colon and low drug systemic bioavailability were reported after oral administration of 5-ASA-loaded chitosan-Ca-alginate microparticles to Wistar male rats.Citation187 Although chitosan is highly soluble in the acidic medium, which results in drug burst in the stomach, coating of the prepared particles with pH-sensitive polymers such as Eudragit S-100 and L-100 was successfully used to overcome this problem, with drugs such as prednisolone,Citation188 satranidazole,Citation189 and metronidazole.Citation190
Nasal route
The mucosa of the nasal cavity is thin and well vascularized, and so the administered drug can be transferred into the systemic circulation quickly. Other advantages of drug delivery via this route are the avoidance of the hepatic first-pass metabolism and the possibility of systemic and local effect. Limitations for this route include the limited volume of the administered formulation, which restricts the application for potent drugs, and also it is not suitable for long-term or frequently administered drugs because of the harmful effect on the nasal epithelium.Citation191 Drugs of low and high molecular weight have been successfully delivered using this route, steroids, antiasthmatic, anesthetics, antihistaminic, antiemetic, sedatives, antimigraine, peptides, peptide analogs such as desmopressin, hormones, vaccines, and most recently drug delivery to the brain for primary meningoencephalitis.Citation192,Citation193
The process of drug delivery via the nasal route is challenging due to the short residence time of the drug, high nasal secretion rate, and low permeability of the nasal membrane. Drug physicochemical properties such as surface charges, hydrophilicity/hydrophobicity, partition coefficient, degree of ionization, and molecular size are very important factors that could affect the drug transport by this route.Citation194 Different nasal drug delivery systems have been studied and illustrated a potential application in this field. Among those, chitosan particles demonstrated some achievement in the nasal delivery of proteins, vaccines, and other therapeutic agents. The mucoadhesive properties accompanied with the small particle size facilitate both retention and permeation of the administered drug-loaded particles. Insulin-loaded chitosan micro- and nanoparticles showed a considerable decrease in blood glucose level after their nasal administration into rats and rabbits, respectively.Citation195,Citation196 Chitosan nanoparticles adsorbed with ovalbumin and cholera toxins induced systemic immune response in rats and were effective for targeting to nasal-associated lymphoid tissues in nasal vaccine delivery.Citation157 Induction of IgA antibodies at different mucosal sites in the body such as upper and lower respiratory tract, and small and large intestine as a result of the distribution of the intranasal administered antigen-loaded chitosan particles have been reported.Citation197–Citation199 Mice that were intranasally immunized with chitosan microspheres loaded with Bordetella bronchiseptica antigens showed significantly higher B. bronchiseptica–specific IgA antibody responses in saliva and serum, with high mice survival rate.Citation197,Citation198
Ocular route
Anatomically, the eye can be divided into two main parts – anterior and posterior. The former region comprises approximately one-third of the eye and consists of cornea, conjunctiva, aqueous humor, iris, ciliary body, and lens, while the latter includes the sclera, choroid, retinal pigment epithelium, neural retina, optic nerve, and vitreous humor. Glaucoma, cataract, allergic conjunctivitis, and anterior uveitis are common diseases affecting the anterior part, whereas diabetic retinopathy and age-related macular degeneration are prevalent diseases of the posterior part. Diseases affecting the anterior eye segment are usually treated using topical instillation of conventional ophthalmic dosage forms such as eye drops, but it is difficult to achieve effective drug concentration in the posterior part because of tear turnover, reflex blinking, nasolachrymal drainage, ocular static, and dynamic barriers that resist access/permeation of the administered drug to deeper segment, and, therefore, less than 5% of topically applied drug dose reaches the deeper ocular tissues.Citation200 Nanoparticle-based ophthalmic formulations are currently of high interest for both anterior and posterior-segment drug delivery because of low irritation, ocular biocompatibility, and convenient bioavailability.
The prolonged ocular residence time, due to mucoadhesiveness, and the absorption-promoting effect of the chitosan nanoparticles compared to the conventional ophthalmic preparations contribute to an improvement of drug ocular efficacy and bioavailability. β-blockers, antibiotics, anti-inflammatory, and antiallergic drugs are common categories of therapeutic agents loaded into chitosan nanoparticles. These nanoparticles improved the delivery of cyclosporin A, selected as a model drug for treatment of local eye disease, for the ocular mucosa in which the in vitro release data illustrated fast drug release in the first hour, followed by a gradual slow-release stage lasting for 24 hours.Citation201 Dorzolamide HCl and timolol maleate, drugs used for treatment of glaucoma, have been loaded into hyaluronic acid–modified chitosan nanoparticles, and the prepared nanoparticles potentially improved the mucoadhesiveness and significantly reduced the intraocular pressure compared to plain drug solution.Citation202
Recently, chitosan and its derivatives have been studied as a nonviral vector for retinal gene therapy. Ultrapure oligochitosans polyplexes have been prepared, characterized, and were reported to be efficient in protecting the incorporated plasmid against enzymatic digestion and transfection efficiency.Citation203 Chitosan–DNA nanoparticles based on ultrapure chitosan oligomers are another example that have been recently marketed and used as carriers for nonviral gene therapy. It has been mentioned that these newly developed nanoparticles, ultrapure chitosan–DNA nanoparticles, could be examined for the treatment of various ocular diseases outside the cornea and for different gene therapy applications.Citation204 In another study, a novel hyaluronan–chitosan nanocarrier-loaded DNA has been developed for topical ophthalmic gene therapy. The prepared nanoparticles were considered an efficient tool for delivery of the loaded plasmid DNA into the cells through reaching significant transfection levels and so was mentioned to be a new strategy toward gene therapy for various ocular diseases.Citation205
Pulmonary route
Owing to the large absorptive surface area (up to 70–140 m2), thin alveolar absorptive mucosal membrane (100–200 nm), and good blood supply due to the highly vascularized extensive capillary network, the pulmonary route can be used to administer drugs.Citation206 Low enzymatic activity and avoiding first-pass metabolism are other advantages of this route. So, growing attention has been given to the pulmonary route as a noninvasive pathway for both systemic and local drug delivery. These include topical treatment of lung infections, pulmonary hypertension, and asthma and systemic administration of hormones, peptides, and proteins such as insulin, oxytocin, and growth hormone.Citation207 The lung is composed of two regions namely conducting airways and the respiratory region. The airway is divided into nasal cavity and associated sinuses, pharynx, larynx, trachea, bronchi, and bronchioles, while the respiratory region consists of respiratory bronchioles, alveolar ducts, and alveolar sacs. Drug absorption from the upper airways is limited due to low blood supply, small surface area, the high filtering function of this part that can remove up to 90% of the administered drug, and the viscous mucus that coats the wall of the airway and expels foreign substance upward and out of the lung by the action of the ciliated cells present in this region.Citation208 Other obstacles that control the transport of drug from this route are the size of pores and tight junction depth of alveolar and endothelial cells and the pulmonary blood–gas barrier system.Citation209
Administration of drugs through this route could be achieved through intranasal or oral inhalation. The narrow lumen airway of the former limit the drug delivery compared to the latter, which allows administration of very small particles even in low concentration. The particle size, shape, and surface electrical charges of the administered drug particles influence drug diffusion through this route.Citation210 Small-sized particles diffuse rapidly based on the Brownian motion. Alveolar macrophages are predominantly responsible for nanoparticles clearance through phagocytosis of the administered particles. Macrophages that entrap the particles are then moved toward the mucociliary escalator to start the clearance process. Several studies reported the inefficiency of the alveolar macrophages to phagocytose ultrafine particles, and so these particles are not cleared in the alveolar region.Citation211 In this case, toxicity is a consequence of the long-term accumulation of these particles in the lung.
Nanoparticles of chitosan and its derivatives were studied for pulmonary administration of some drugs to treat different pulmonary diseasesCitation212 and infections such as tuberculosis.Citation213,Citation214 The biocompatibility of chitosan nanoparticles administered as respirable powder was evaluated by Grenha et alCitation215 using human respiratory epithelial Calu-3 and A549 cell lines, and the results demonstrated the mucoadhesive feature of the chitosan particles, no signs of overtoxicity, and no change in transepithelial electrical resistance or solute permeability. Both Calu-3 and A549 respiratory epithelial cell lines have been previously used to evaluate the in vitro efficacy and safety of particulate drug delivery systems. Calu-3 cell layers form tight junctions in vitro and, under suitable culture conditions, exhibit acceptable in vivo–like barrier properties.Citation215 Inhalation of chitosan microparticles has been reported to be of potential interest in pulmonary pharmacotherapy as indicated by the inflammation response produced in rat lungs, which was 1.8-fold greater in the group administered microparticles than the control group without chitosan microparticles. These effects were documented by an increase in bronchoalveolar lavage fluid protein and lactate dehydrogenase activity and also with an increase in lung tissue myeloperoxidase activity and leukocyte migration.Citation216 Intratracheal instillation of hydrophobically modified glycol chitosan nanoparticles to mice exhibited rapid uptake into systemic circulation and induced transient neutrophilic pulmonary inflammation in the tested animals from 6 hours to 3 days after instillation.Citation217 So, chitosan micro- and nanoparticles could be considered successful vehicles for pulmonary drug delivery. Recently, chitosan/carrageenan/tripolyphosphate nanoparticle-loaded BSA were prepared using spray drying technique to meet the required geometric diameter and mass density and aerodynamic character essential to pulmonary drug delivery and were evaluated for their capacity as protein carrier for nasal and pulmonary transmucosal delivery. A biocompatibility study was also conducted on Calu-3 and A549 cells, respiratory cell lines, in which no inflammatory response or change in the transepithelial electrical resistance of the cell lines were noticed.Citation218
Topical and transdermal routes
Physiologically, the skin is composed of four layers – stratum corneum, epidermis, dermis, and subcutaneous tissue. In addition to these layers, several pilosebaceous and sweat glands are dispersed throughout the skin. Although the skin has relatively a large surface area, it acts as a penetration barrier that represents a challenge for the delivery of any therapeutic agent, either into the skin strata regarding topical application or into the systemic circulation through transdermal application. This barrier function is mainly due to the stratum corneum, the outer most layer of the epidermis, which consists of aggregated keratin filaments covered with a cornified envelope surrounded by multiple lamellar lipid bilayer. This structure prevents both excessive water loss from the body and entry of topically applied drugs, which represents challenge for drugs applied for local or systemic effect. Drugs of low molecular weight and macromolecules have been formulated as topical and transdermal delivery systems, and many systems are undergoing clinical trials.Citation219 Skin permeation of the administered drug may be accomplished by intercellular or transcellular penetration of the stratum corneum layer, or across hair follicles, sebaceous glands, and sweat glands. Substances smaller than 500 kDa that exhibit sufficient oil solubility and high partition coefficient are absorbed easily through the skin. However, in contrast, larger drug molecules (molecular weight >500 kDa) cannot pass the skin cutaneous barrier.Citation220 Topical and transdermal routes offer many advantages compared to the other drug delivery routes. Transdermal is a noninvasive, inexpensive, and self-administered drug delivery system that provides extended drug-release effect without significant first-pass effect, whereas the topical route allows direct application of the drug to the site of action with minimal risk of systemic side effect.
Chitosan nanoparticles have been extensively used in topical as well as transdermal drug delivery. Retinol, a derivative of vitamin A, has been encapsulated in chitosan nanoparticles and was efficiently used in the treatment of acne and wrinkles.Citation221 Fabrication of acyclovir-loaded chitosan nanoparticles leads to a decrease in the photodegradation of the drug, thus contributing to increased drug stability and an improvement in drug penetration through porcine skin.Citation222 Delivery of macromolecules such as plasmid DNA and antisense oligonucleotides previously loaded into chitosan nanoparticles has been reported. Suppression of β-gal expression following administration of antisense oligonucleotides at 6 days posttransfection in rats has also been demonstrated.Citation223 Transfection of DNA in baby rat skin subsequent to topical application of DNA–chitosan nanoparticles was also described by the same authors.Citation224
Generally speaking, nonparenteral routes of therapeutic administration have gained intense interest, especially for the administration of macromolecules such as peptides, proteins, hormones, and antigens. Chitosan and its derivatives have been extensively used for mucosal as well as parenteral biotherapeutics delivery owing to the mucoadhesive properties and enhanced local and systemic therapeutic effect. The size of the prepared particles, which could be controlled through the processing and formulation parameters, is pivotal for the efficient delivery of the loaded substances.
Chitosan metal nanoparticles
Metal nanoparticles of copper (Cu), silver (Ag), and gold (Au) have demonstrated a wide spectrum of activity against gram-negative and gram-positive bacteria as well as fungi. However, there are great concerns about the human and environmental safety of these metal nanoparticles. In addition, the stability of these particles is also under discussion, especially regarding copper nanoparticles, which undergo rapid oxidation upon exposure to the air.Citation225 Synthesis of these metal nanoparticles in the presence of biocompatible polymers (such as PEG, polyvinyl pyrrolidone, and chitosan) and surfactants that are used as stabilizers could help overcome these limitations. The coating of the particles’ surface using polymeric materials, such as chitosan, has been reported to enhance the antimicrobial activity of these particles,Citation225 owing to chitosan antimicrobial activity.
Chitosan-coated silver nanoparticles were prepared by a chemical reduction method and have reported in applications as a biosensor and in cancer therapy. The prepared nanoparticles exhibited biodegradable character, good antimicrobial activity, and prolonged action of silver on the affected cells.Citation226 Honary et alCitation227 synthesized chitosan-coated silver nanoparticles by the same method and by utilizing chitosans of different molecular weight. The authors demonstrated that the nanoparticle characteristics were influenced by the molecular weight of chitosan, as well as by the process conditions, such as stirring speed and temperature. They also mentioned that higher antibacterial activity against Staphylococcus aureus was achieved with smaller particle size due to the increase in the particle surface area.
Gold nanoparticles have been reported to be useful in diagnosis and drug delivery. The incorporation of chitosan during the synthesis of these metal nanoparticles offers better penetration and uptake of therapeutic agents such as insulin, across the mucosal membrane, and chitosan itself acts as a reducing agent during gold nanoparticle synthesis.Citation228 The prepared insulin-loaded chitosan gold nanoparticles were stable, did not show any sign of aggregation for 6 months, and significantly lowered the blood glucose level in diabetic rats following oral and nasal administration.Citation228 Recently, Salehizadeh et alCitation229 mentioned the formation of Fe3O4–gold–chitosan nanostructure by the chemical coprecipitation method and reported the usefulness of the prepared nanoparticles in different biotechnological and biomedical applications.
Chitosan copper-loaded nanoparticles were prepared by ionic gelation between chitosan and TPP and showed a marked growth inhibition of a wide range of microorganisms, such as S. aureus, Salmonella typhimurium, Salmonella choleraesuis, and Escherichia coli, in which the minimum inhibitory concentration was less than 0.25 μg/mL.Citation230 Green synthesis is the technique widely used to prepare this type of chitosan metal nanoparticles, involving reduction of copper in an aqueous solution of chitosan and an organic acid, such as ascorbic acid, which prevents the formation of copper oxides.Citation231
More recently, chitosan cobalt oxide nanoparticles were developed, and their activity on human leukemic cells was investigated.Citation232 The authors demonstrated increase in the reactive oxygen species and caspase activation following exposure of the leukemic cells to these chitosan-coated metallic nanoparticles, effects that are known to lead to cancer cell death. Therefore, there is an indication of the potential of these nanoparticles for an application in cancer therapy.
Conclusion
This review shows that extensive research activities have been focused on the applications of chitosan-based micro- and nanoparticles. Successful loading and delivery of different molecules, including low-molecular-weight drugs and macromolecules, such as proteins, peptides, vaccines, hormones, and genes by these systems via different routes of administration, find potential therapeutic applications. The development of chitosan derivatives has extended these applications due to the enhancement of bioavailability accomplished by an increase in the stability, solubility, mucoadhesiveness, cellular permeability, absorption, biodistribution, and tissue targeting achieved when particulate carriers are based on these derivatives. This review has addressed the different techniques that could be utilized in the development of these particulate systems and methods of characterization of the obtained particles. An overview on the parenteral and nonparenteral applications of these chitosan and chitosan derivatives–based particulate system has been illustrated. Chitosan–metal nanoparticles are another type of particle that has demonstrated the ability to improve antimicrobial and pharmacodynamic activity when compared with metal nanoparticles devoid of chitosan coating.
Future challenges
Gene transfection efficiency for gene-loaded polymeric nanoparticles, such as chitosan, remains the most challenging in the development of most polymer-based nanoparticles for gene delivery. Although some researchers have made several attempts in this area, as illustrated in this review, the rate of transfection still remains low. Moreover, currently, several drugs of plant origin, especially those possessing antitumor activity, are being discovered and the process of loading these therapeutic agents into a suitable carrier and targeting to the active site of action needs improvement. Finally, although that chitosan particulate systems have been studied for more than 2 decades, always with good results and promise in delivery of different therapeutic agents, both in vitro and in vivo in experimental animals, no commercial formulations of these systems are available. This could be attributed to poor bioavailability, possible immunogenicity and long-term toxicity, low target specificity, limited stability, and lack of clinical trials in human, and these items need further investigation.
Acknowledgments
The authors gratefully acknowledge Dr Ana Grenha for her guidance. The authors also thank Dr Mohammed M Badran for his collaboration in the scanning electron microscope study.
Disclosure
The authors report no conflict of interest in this work.
References
- ChirraHDDesaiTAEmerging microtechnologies for the development of oral drug delivery devicesAdv Drug Deliv Rev201264141569157822981755
- Des RieuxAFievezVGarinotMSchneiderYJPreatVNanoparticles as potential oral delivery systems of proteins and vaccines: a mechanistic approachJ Control Release2006116112717050027
- SahooSKParveenSPandaJJThe present and future of nanotechnology in human health careNanomedicine200731203117379166
- ParkJHLeeSKimJHParkKKimKKwonICPolymeric nanomedicine for cancer therapyProg Polym Sci2008331113137
- SumerBGaoJTheranostic nanomedicine for cancerNanomedicine20083213714018373419
- DassCRChoongPThe use of chitosan formulations in cancer therapyJ Microencapsul200825427527918465306
- PrabaharanMReview paper: chitosan derivatives as promising materials for controlled drug deliveryJ Biomater Appl200823153618593819
- AugstADKongHJMooneyDJAlginate hydrogels as biomaterialsMacromol Biosci20066862363316881042
- GombotzWRWeeSFProtein release from alginate matricesAdv Drug Deliv Rev199831326728510837629
- PiyakulawatPPraphairaksitNChantarasiriNMuangsinNPreparation and evaluation of chitosan/carrageenan beads for controlled release of sodium diclofenacAAPS PharmSciTech200784E9718181557
- SantoVEFriasAMCaridaMCarrageenan-based hydrogels for the controlled delivery of PDGF-BB in bone tissue engineering applicationsBiomacromolecules20091061392140119385660
- GrenhaAGomesMERodriguesMDevelopment of new chitosan/carrageenan nanoparticles for drug delivery applicationsJ Biomed Mater Res A20109241265127219322874
- AgnihotriSAMallikarjunaNNAminabhaviTMRecent advances on chitosan-based micro- and nanoparticles in drug deliveryJ Control Release2004100152815491807
- RobertsGAFStructure of chitin and chitosanRobertsGAFChitin ChemistryHoundmills, BasingstokeMacmillan1992153
- LeHouxJGGrondinFSome effects of chitosan on liver function in the ratEndocrinology1993132107810847679967
- ChandyTSharmaCPChitosan – as a biomaterialBiomater Artif Cells Artif Organs19901811242185854
- SuganoMWatanabeSKishiAIzumeMOhtakaraAHypocholesterolemic action of chitosans with different viscosity in ratsLipids19882331871912836688
- AzadAKSermsinthamNChandrkrachangSStevensWFChitosan membrane as a wound-healing dressing: characterization and clinical applicationJ Biomed Mater Res B Appl Biomater200469221622215116411
- FeltOBuriPGurnyRChitosan: a unique polysaccharide for drug deliveryDrug Dev Ind Pharm199824119799939876553
- RabeaEIBadawyMESteurbautWFungicidal effect of chitosan derivatives containing an N-alkyl group on grey mould Botryti77s cinerea and rice leaf blast Pyricularia griseaCommun Agric Appl Biol Sci200570321922316637181
- KockischSReesGDYoungSATsibouklisJSmartJDPolymeric microspheres for drug delivery to the oral cavity: an in vitro evaluation of mucoadhesive potentialJ Pharm Sci2003921614162312884248
- SinhaVRSinglaAKWadhawanSChitosan microspheres as a potential carrier for drugsInt J Pharm20042741–213315072779
- HuoMZhangYZhouJSynthesis and characterization of low-toxic amphiphilic chitosan derivatives and their application as micelle carrier for antitumor drugInt J Pharm20103941–216217320457237
- DashaMChielliniaFOttenbritebRMChielliniEChitosan – a versatile semi-synthetic polymer in biomedical applicationsProg Polym Sci2011369811014
- CalderónLHarrisRCordoba-DiazMNano and microparticulate chitosan-based systems for antiviral topical deliveryEur J Pharm Sci2013481–221622223159663
- AiedehKTahaMOSynthesis of chitosan succinate and chitosan phthalate and their evaluation as suggested matrices in orallyadministered, colon-specific drug delivery systemsArch Pharm (Weinheim)1999332310310710228455
- FloreaBIThanouMGeldofMMeaneyCJungingerHEBorchardGModified chitosan oligosaccharides as transfection agents for gene therapy of cystic fibrosisProc Int Symp Control Release Bioact Mater200027846847
- SievalABThanouMKotzeAFVerhoefJEBrusseeJJungingerHEPreparation and NMR characterization of highly substituted N-trimethyl chitosanchlorideCarbohydr Polym199836157165
- VerheulRJAmidiMvan der WalSvan RietEJiskootWHenninkWESynthesis, characterization and in vitro biological properties of O-methyl free N,N,N-trimethylated chitosanBiomaterials2008293642364918556059
- HammanJHStanderMKotzeAFEffect of the degree of quaternisation of N-trimethyl chitosan chloride on absorption enhancement: in vivo evaluation in rat nasal epitheliaInt J Pharm200223223524211790507
- ThanouMVerhoefJCMarbachPJungingerHEIntestinal absorption of octreotide: N-trimethyl chitosan chloride (TMC) ameliorates the permeability and absorption properties of the somatostatin analogue in vitro and in vivoJ Pharm Sci20008995195710861597
- ThanouMNihotMTJansenMVerhoefJCJungingerHEMono-N-carboxymethyl chitosan (MCC), a polyampholytic chitosan derivative, enhances the intestinal absorption of low molecular weight heparin across intestinal epithelia in vitro and in vivoJ Pharm Sci200190384611064377
- XuYDuYHuangRGaoLPreparation and modification of N-(2-hydroxyl) propyl-3-trimethyl ammonium chitosan chloride nanoparticle as a protein carrierBiomaterials2003245015502214559015
- Bernkop-SchnurchAHornofMZoidlTThiolated polymers–thiomers: synthesis and in vitro evaluation of chitosan-2-iminothiolane conjugatesInt J Pharm200326022923712842342
- HornofMDKastCEBernkop-SchnurchAIn vitro evaluation of the viscoelastic properties of chitosan-thioglycolic acid conjugatesEur J Pharm Biopharm20035518519012637095
- RoldoMHornofMCalicetiPBernkop-SchnurchAMucoadhesive thiolated chitosans as platforms for oral controlled drug delivery: synthesis and in vitro evaluationEur J Pharm Biopharm20045711512114729087
- YinLDingJHeCCuiLTangCYinCDrug permeability and mucoadhesion properties of thiolated trimethyl chitosan nanoparticles in oral insulin deliveryBiomaterials200930295691570019615735
- UbaidullaUKharRKAhmadFJSultanaYPandaAKDevelopment and characterization of chitosan succinate microspheres for the improved oral bioavailability of insulinJ Pharm Sci200796113010302317588259
- UbaidullaUSultanaYAhmedFJKharRKPandaAKChitosan phthalate microspheres for oral delivery of insulin: preparation, characterization, and in vitro evaluationDrug Deliv2007141192317107927
- RekhaMRSharmaCPSynthesis and evaluation of lauryl succinyl chitosan particles towards oral insulin delivery and absorptionJ Control Release2009135214415119331862
- TomitaMHayashiMHorieTIshizawaTAwazuSEnhancement of colonic drug absorption by the transcellular permeation routePharm Res19885127867893247289
- Le TienCLacroixMIspas-SzaboPMateescuMAN-acylated chitosan: hydrophobic matrices for controlled drug releaseJ Control Release200393111314602417
- SoniaTASharmaCPChitosan and its derivatives for drug delivery perspectiveAdv Polym Sci20112432354
- ThanouMNihotMTJansenMVerhoefJCJungingerHEMono-N-carboxymethyl chitosan (MCC), a polyampholytic chitosan derivative, enhances the intestinal absorption of low molecular weight heparin across intestinal epithelia in vitro and in vivoJ Pharm Sci200190384611064377
- JayakumarRPrabaharanMReisRLManoJFGraft copolymerized chitosan-present status and applicationsCarbohydr Polym200562142158
- ShantaKLHardingDRKSynthesis and characterization of chemically modified chitosan microspheresCarbohydr Polym200248247253
- ZhangCPingQDingYChengYShenJSynthesis, characterization and microsphere formation of galactosylated chitosanJ Appl Polym Sci200491659665
- SezerADCevherETopical drug delivery using chitosan nano- and microparticlesExpert Opin Drug Deliv2012991129114622769587
- ChaudhuryADasSRecent advancement of chitosan-based nanoparticles for oral controlled delivery of insulin and other therapeutic agentsAAPS PharmSciTech2011121102021153572
- KumariAYadavSKYadavSCBiodegradable polymeric nanoparticles based drug delivery systemsColloids Surf B Biointerfaces20107511819782542
- RaoSBSharmaCPUse of chitosan as biomaterial: studies on its safety and hemostatic potentialJ Biomed Mater Res19973421288978649
- LehrCMBouwstraJASchachtEHJungingerHEIn vitro evaluation of mucoadhesive properties of chitosan and some other natural polymersInt J Pharm1992784348
- ChowdaryKPRaoYSDesign and in vitro and in vivo evaluation of mucoadhesive microcapsules of glipizide for oral controlled release: a technical noteAAPS PharmSciTech200343E3914621971
- DastanTTuranKIn vitro characterization and delivery of chitosan-DNA microparticles into mammalian cellsJ Pharm Pharm Sci20047220521415367377
- LiYHFanMWBianZChenZZhangQYangHRChitosan-DNA microparticles as mucosal delivery system: synthesis, characterization and release in vitroChin Med J (Engl)20051181193694115978195
- ChuaBYAl KobaisiMZengWMainwaringDJacksonDCChitosan microparticles and nanoparticles as biocompatible delivery vehicles for peptide and protein-based immunocontraceptive vaccinesMol Pharm201291819022149016
- VarshosazJSadraiHAlinagariRNasal delivery of insulin using chitosan microspheresJ Microencapsul200421776177415799226
- AcikgozMKasHSOrmanMHincalAAChitosan microspheres of diclofenac sodium: I. Application for factorial design and evaluation of release kineticsJ Microencapsul1996131411608999120
- AkbugaJBergisadiN5-fluorouracil-loaded chitosan microspheres: preparation and release characteristicsJ Microencapsul1996131611688999121
- WangYHSatoHAdachiIHorikoshiIOptimisation of the formulation design of chitosan microspheres containing cisplatinJ Pharm Sci19968512048923326
- KoJAParkHJHwangSJParkJBLeeJSPreparation and characterization of chitosan microparticles intended for controlled drug deliveryInt J Pharm20022491–216517412433445
- DiniEAlexandridouSKiparissidesCSynthesis and characterization of cross-linked chitosan microspheres for drug delivery applicationsJ Microencapsul200320337538512881117
- JansenJMaibachHIEncapsulation to deliver topical activesHandbook of Cosmetic Science and TechnologyNew York, NYMarcel Dekker2001
- KofujiKQianCJMurataYKawashimaSPreparation of chitosan microparticles by water-in-vegetable oil emulsion coalescence techniqueReact Funct Polym2005627783
- GiunchediPJulianoCGaviniECossuMSorrentiMFormulation and in vivo evaluation of chlorhexidine buccal tablets prepared using drug-loaded chitosan microspheresEur J Pharm Biopharm200253223323911880008
- CorriganDOHealyAMCorriganOIPreparation and release of salbutamol from chitosan and chitosan co-spray dried compacts and multiparticulatesEur J Pharm Biopharm200662329530516314079
- AlhalawehAAnderssonSVelagaSPPreparation of zolmitriptan-chitosan microparticles by spray drying for nasal deliveryEur J Pharm Sci200938320621419616094
- LiXKongXShiSPreparation of alginate coated chitosan microparticles for vaccine deliveryBMC Biotechnol200888919019229
- AbreuFOMSForteMMCKistTBLHonaiserLPEffect of the preparation method on the drug loading of alginate-chitosan microsphereseXPRESS Polym Lett201048456464
- ZhangYWeiWLvPWangLMaGPreparation and evaluation of alginate–chitosan microspheres for oral delivery of insulinEur J Pharm Biopharm2011771111920933083
- WangQXieXZhangXZhangJWangAPreparation and swelling properties of pH-sensitive composite hydrogel beads based on chitosan-g-poly(acrylic acid)/vermiculite and sodium alginate for diclofenac controlled releaseInt J Biol Macromol201046335636220096301
- KrekelerMPSGuggenheimSDefects in microstructure in palygorskite-sepiolite minerals: a transmission electron microscopy (TEM) studyAppl Clay Sci2008391–298105
- WangQWuJWangWWangAPreparation, characterization and drug-release behaviors of crosslinked chitosan/attapulgite hybrid microspheres by a facile spray-drying techniqueJ Biomater Nanobiotechnol20112250257
- ZhangFKohGYJeansonneDPA novel solubility enhanced curcumin formulation showing stability and maintenance of anticancer activityJ Pharm Sci201110072778278921312196
- YallapuMMGuptaBKJaggiMChauhanSCFabrication of curcumin encapsulated PLGA nanoparticles for improved therapeutic effects in metastatic cancer cellsJ Colloid Interface Sci20103511192920627257
- DasRKKasojuNBoraUEncapsulation of curcumin in alginate-chitosan-pluronic composite nanoparticles for delivery to cancer cellsNanomedicine20106115316019616123
- ThangapazhamRLPuriATeleSBlumenthalRMaheshwariKEvaluation of a nanotechnology-based carrier for delivery of curcumin in prostate cancer cellsInt J Oncol20083251119112318425340
- Guzman-VillanuevaDEl-SherbinyIMHerrera-RuizDSmythHDDesign and in vitro evaluation of a new nano-microparticulate system for enhanced aqueous-phase solubility of curcuminBiomed Res Int2013201372476323984402
- MaestrelliFGarcia-FuentesMMuraPAlonsoMJA new drug nanocarrier consisting of chitosan and hydoxypropylcyclodextrinEur J Pharm Biopharm2006632798616527473
- WangJTanHYuAPreparation of chitosan-based nanoparticles for delivery of low molecular weight heparinJ Biomed Nanotechnol20117569670322195488
- LeeSJParkKOhYKTumor specificity and therapeutic efficacy of photosensitizer-encapsulated glycol chitosan-based nanoparticles in tumor-bearing miceBiomaterials200930152929293919254811
- PrabaharanMChitosan-based nanoparticles for tumor-targeted drug deliveryInt J Biol Macromol2014721313132225450550
- KwonICChitosan-based nanoparticles for cancer therapy; tumor specificity and enhanced therapeutic efficacy in tumor-bearing miceJ Control Release20081323e69e70
- Garcia-FuentesMPregoCTorresDAlonsoMJA comparative study of the potential of solid triglyceride nanostructures coated with chitosan or poly(ethylene glycol) as carriers for oral calcitonin deliveryEur J Pharm Sci20052513314315854809
- VilaASanchezATobıoMCalvoPAlonsoMJDesign of biodedegradable particles for protein deliveryJ Control Release200278152411772445
- PanYLiYJZhaoHYBioadhesive polysaccharide in protein delivery system: chitosan nanoparticles improve the intestinal absorption of insulin in vivoInt J Pharm200224913914712433442
- KatasHRajaMALamKLDevelopment of chitosan nanoparticles as a stable drug delivery system for protein/siRNAInt J Biomater2013201314632024194759
- GanQWangTChitosan nanoparticle as protein delivery carrier – systematic examination of fabrication conditions for efficient loading and releaseColloids Surf B Biointerfaces2007591243417555948
- CuiZMumperRJChitosan-based nanoparticles for topical genetic immunizationJ Control Release200175340941911489327
- PregoCPaolicelliPDíazBChitosan-based nanoparticles for improving immunization against hepatitis B infectionVaccine201028142607261420096389
- MaZLimTMLimLYPharmacological activity of peroral chitosan–insulin nanoparticles in diabetic ratsInt J Pharm20052931–227128015778065
- QuJBShaoHHJingGLHuangFPEG-chitosan-coated iron oxide nanoparticles with high saturated magnetization as carriers of 10-hydroxycamptothecin: preparation, characterization and cytotoxicity studiesColloids Surf B Biointerfaces2013102374423000675
- ChronopoulouLMassimiMGiardiMFChitosan-coated PLGA nanoparticles: a sustained drug release strategy for cell culturesColloids Surf B Biointerfaces201310331031723261553
- BagreAPJainKJainNKAlginate coated chitosan core shell nanoparticles for oral delivery of enoxaparin: in vitro and in vivo assessmentInt J Pharm20134561314023994363
- ZhangYChanHFLeongKWAdvanced materials and processing for drug delivery: the past and the futureAdv Drug Deliv Rev201365110412023088863
- Luangtana-ananMOpanasopitPNgawhirunpatTEffect of chitosan salts and molecular weight on a nanoparticulate carrier for therapeutic proteinPharm Dev Technol20051018919615926667
- ChanHKKwokPCProduction methods for nanodrug particles using the bottom-up approachAdv Drug Deliv Rev20116340641621457742
- HenninkWEvan NostrumCFNovel crosslinking methods to design hydrogelsAdv Drug Deliv Rev2002541133611755704
- MaoSBakowskyUJintapattanakitAKisselTSelf-assembled polyelectrolyte nanocomplexes between chitosan derivatives and insulinJ Pharm Sci2006951035104816565978
- KoJAParkHJHwangSJParkJBLeeJSPreparation and characterization of chitosan microparticles intended for controlled drug deliveryInt J Pharm200224916517412433445
- VilaASánchezAJanesKLow molecular weight chitosan nanoparticles as new carriers for nasal vaccine delivery in miceEur J Pharm Biopharm200457112313114729088
- AmidiMRomeijnSGBorchardGJungingerHEHenninkWEJiskootWPreparation and characterization of protein-loaded N-trimethyl chitosan nanoparticles as nasal delivery systemJ Control Release20061111–210711616380189
- AmidiMRomeijnSGVerhoefJCN-trimethyl chitosan (TMC) nanoparticles loaded with influenza subunit antigen for intranasal vaccination: biological properties and immunogenicity in a mouse modelVaccine200725114415316973248
- Ozbas-TuranSAkbugaJAralCControlled release of interleukin-2 from chitosan microspheresJ Pharm Sci2002911245125111977100
- KizilayEKayitmazerABDubinPLComplexation and coacervation of polyelectrolytes with oppositely charged colloidsAdv Colloid Interface Sci20111671–2243721803318
- BozkirASakaOMChitosan nanoparticles for plasmid DNA delivery: effect of chitosan molecular structure on formulation and release characteristicsDrug Deliv200411210711215200009
- MoorenFCBertholdADomschkeWKreuterJInfluence of chitosan microspheres on the transport of prednisolone sodium phosphate across HT-29 cell monolayersPharm Res199815158659487547
- BasuSKKavithaKRupeshkumarMEvaluation of ketorolac tromethamine microspheres by chitosan/gelatin B complex coacervationSci Pharm201078799221179371
- SarmentoBRibeiroAVeigaFFerreiraDDevelopment and characterization of new insulin containing polysaccharide nanoparticlesColloids Surf B Biointerfaces20065319320217049214
- HuangMVitharanaSNPeekLJCoopTBerklandCPolyelectrolyte complexes stabilize and controllably release vascular endothelial growth factorBiomacromolecules200781607161417428030
- LinYHChungCKChenCTLiangHFChenSCSungHWPreparation of nanoparticles composed of chitosan/poly-γ-glutamic acid and evaluation of their permeability through Caco-2 cellsBiomacromolecules200561104111215762683
- Alonso-SandeMCunaMRemunan-LopezCFormation of new glucomannan–chitosan nanoparticles and study of their ability to associate and deliver proteinsMacromolecules20063941524158
- BoddohiSMooreNJohnsonPAKipperMJPolysaccharide-based polyelectrolyte complex nanoparticles from chitosan, heparin, and hyaluronanBiomacromolecules2009101402140919371056
- HuangMVitharanaSNPeekLJCoopTBerklandCPolyelectrolyte complexes stabilize and controllably release vascular endothelial growth factorBiomacromolecules200781607161417428030
- ZhuSYQianFZhangYTangCYinCHSynthesis and characterization of PEG modified N-trimethylaminoethylmethacrylate chitosan nanoparticlesEur Polym J20074322442253
- SchatzCBionazALucasJMFormation of polyelectrolyte complex particles from self-complexation of N-sulfated chitosanBiomacromolecules200561642164715877389
- OrientiIAiedehKGianasiEBertasiVZecchiVIndomethacin loaded chitosan microspheres. Correlation between the erosion process and release kineticsJ Microencapsul1996134634728808782
- BugamelliFRaggiMAOrientiIZecchiVControlled insulin release from chitosan microparticlesArch Pharm (Weinheim)19983311331389648521
- JameelaSRKumaryTVLalAVJayakrishnanAProgesterone-loaded chitosan microspheres: a long acting biodegradable controlled delivery systemJ Control Release19985217249685932
- AhmedTAEl-SayKMDevelopment of alginate-reinforced chitosan nanoparticles utilizing W/O nanoemulsification/internal crosslinking technique for transdermal delivery of rabeprazoleLife Sci2014110354324997393
- WangLYGuYHZhouQZMaGHWanYHSuZGPreparation and characterization of uniform-sized chitosan microspheres containing insulin by membrane emulsification and a two-step solidification processColloids Surf B Biointerfaces20065012613516787743
- WeiWYuanLHuGMonodisperse chitosan microspheres with interesting structures for protein drug deliveryAdv Mater20082022922296
- TokumitsuHIchikawaHFukumoriYChitosan–gadopentetic acid complex nanoparticles for gadolinium neutron capture therapy of cancer: preparation by novel emulsion droplet coalescence technique and characterizationPharm Res199916121830183510644070
- ChawlaATaylorKMGNewtonJMJohnsonMCRProduction of spray dried salbutamol sulphate for use in dry powder aerosol formulationsInt J Pharm1994108233240
- GanderBWehrliEAlderRMerkleHPQuality improvement of spray-dried, protein-loaded D,L-PLA microspheres by appropriate polymer solvent selectionJ Microencapsul19951283977730960
- LeeHKParkJHKwonKCDouble-walled microparticles for single shot vaccineJ Control Release199744283293
- BodmeierRChenHPreparation of biodegradable poly(±)-lactide microspheres using a spray-drying techniqueJ Pharm Pharmacol1988407547572907552
- YangMVelagaSYamamotoHCharacterisation of salmon calcitonin in spray-dried powder for inhalation. Effect of chitosanInt J Pharm200733117618117126507
- KusonwiriyawongCLipipunVVardhanabhutiNZhangQRitthidejGCSpray-dried chitosan microparticles for cellular delivery of an antigenic protein: physico-chemical properties and cellular uptake by dendritic cells and macrophagesPharm Res20133061677169723483441
- HePDavisSSIllumLChitosan microspheres prepared by spray dryingInt J Pharm1999187536510502613
- ShiXYTanTWPreparation of chitosan/ethylcellulose complex microcapsule and its application in controlled release of vitamin D-2Biomaterials2002234469447312322966
- HarshaSNAldhubiabBENairABNanoparticle formulation by Büchi B-90 Nano Spray Dryer for oral mucoadhesionDrug Des Devel Ther20159273282
- ReverchonEDagheroJMarroneCMatteaMPolettoMSupercritical fractional extraction of fennel seed oil and essential oil: experiments and mathematical modelingInd Eng Chem Res19993830693075
- AmidiMPellikaanHCde BoerAHCrommelinDJHenninkWEJiskootWPreparation and physicochemical characterization of supercritically dried insulin loaded microparticles for pulmonary deliveryEur J Pharm Biopharm200868219120017576056
- de DiegoYPPellikaanHCWubboltsFEBorchardGWitkampGJJansensPJOpening new operating windows for polymer and protein micronization using the PCA processJ Supercrit Fluids200636216224
- OkamotoHNishidaSTodoHSakakuraYIidaKDanjoKPulmonary gene delivery by chitosan–pDNA complex powder prepared by a supercritical carbon dioxide processJ Pharm Sci20039237138012532386
- MeloEPAires-BarrosMRCabralJMReverse micelles and protein biotechnologyBiotechnol Annu Rev200178712911686050
- KrishnaSAAmareshwarPPreparation of bovine serum albumin loaded chitosan nanoparticle using reverse micelle methodRes J Pharm Biol Chem Sci201123837846
- MitraSGaurUGhoshPCMaitraANTumor targeted delivery of encapsulated dextran–doxorubicin conjugate using chitosan nanoparticles as carrierJ Control Release20017431732311489513
- AgnihotriSAAminabhaviTMControlled release of clozapine through chitosan microparticles prepared by a novel methodJ Control Release20049624525915081216
- GarudNGarudAPreparation and in-vitro evaluation of metformin microspheres using non-aqueous solvent evaporation techniqueTrop J Pharm Res2012114577583
- Particle size distributions from sub-nanometer to millimeters Available from: http://www.malvern.com/en/products/measurement-type/particle-size/Accessed December 31, 2015
- El-SayKMEl-HelwAMAhmedOAAHosnyKMAhmedTANovel controlled release of antihypertensive drug: preparation, in-vitro and in-vivo evaluationAfr J Pharm Pharmacol201372517441756
- MerkusHGParticle Size Measurements: Fundamentals, Practice, QualityNew York, NYSpringer Science & Business Media200915
- AhmedTAEl-SayKMMahmoudMFSamyAMBadawiAAMiconazole nitrate oral disintegrating tablets: in vivo performance and stability studyAAPS PharmSciTech201213376077122585373
- MaZYeohHHLimLYFormulation pH modulates the interaction of insulin with chitosan nanoparticlesJ Pharm Sci20029161396140412115839
- GazoriTKhoshayandMRAziziEYazdizadePNomaniAHaririanIEvaluation of alginate/chitosan nanoparticles as antisense delivery vector: formulation, optimization and in vitro characterizationCarbohydr Polym200977599606
- CoverNFLai-YuenSParsonsAKKumarASynergetic effects of doxycycline-loaded chitosan nanoparticles for improving drug delivery and efficacyInt J Nanomedicine201272411241922811601
- RodriguesSda CostaAMGrenhaAChitosan/carrageenan nanoparticles: effect of cross-linking with tripolyphosphate and charge ratiosCarbohydr Polym201289128228924750635
- CasettariLCastagninoEStolnikSLewisAHowdleSMIllumLSurface characterisation of bioadhesive PLGA/chitosan microparticles produced by supercritical fluid technologyPharm Res20112871668168221394661
- KimKKimJHParkHTumor-homing multifunctional nanoparticles for cancer theragnosis: simultaneous diagnosis, drug delivery, and therapeutic monitoringJ Control Release2010146221922720403397
- NishimuraKNishimuraSNishiNAdjuvant activity of chitin derivatives in mice and guinea pigsVaccine198533793843936299
- NishimuraKIshiharaCUkeiSTokuraSAzumaIStimulation of cytokine production in mice using deacetylated chitinVaccine198641511562429471
- ZaharoffDARogersCJHanceKWSchlomJGreinerJWChitosan solution enhances the immunoadjuvant properties of GM-CSFVaccine2007258673868618037196
- ZaharoffDARogersCJHanceKWSchlomJGreinerJWChitosan solution enhances both humoral and cell-mediated immune responses to subcutaneous vaccinationVaccine2007252085209417258843
- ReadRCNaylorSCPotterCWEffective nasal influenza vaccine delivery using chitosanVaccine2005234367437415916838
- NagamotoTHattoriYTakayamaKMaitaniYNovel chitosan particles and chitosan-coated emulsions inducing immune response via intranasal vaccine deliveryPharm Res200421467167415139524
- JaganathanKSVyasSPStrong systemic and mucosal immune responses to surface-modified PLGA microspheres containing recombinant hepatitis B antigen administered intranasallyVaccine200624194201421116446012
- ZhaoHWuBWuHProtective immunity in rats by intranasal immunization with Streptococcus mutans glucan-binding protein D encapsulated into chitosan-coated poly(lactic-co-glycolic acid) microspheresBiotechnol Lett200628161299130416804764
- AmidiMMastrobattistaEJiskootWHenninkWEChitosan-based delivery systems for protein therapeutics and antigensAdv Drug Deliv Rev2010621598219925837
- JayakumarRChennazhiKPMuzzarelliRAATamuraHNairSVSelvamuruganNChitosan conjugated DNA nanoparticles in gene therapyCarbohydr Polym201079118
- KatasHAlparHDevelopment and characterization of chitosan nanoparticles for siRNA deliveryJ Control Release2006115221622516959358
- LiuXDChitosan-siRNA complex nanoparticles for gene silencingJ Biomed Engin201027197101
- GaoSChenJXuXGalactosylated low molecular weight chitosan as DNA carrier for hepatocyte-targetingInt J Pharm2003255576812672602
- Dautry-VarsatAReceptor-mediated endocytosis: the intracellular journey of transferrin and its receptorBiochimie1986683753812874839
- LeeDLockeyRMohapatraSFolate receptor-mediated cancer cell specific gene delivery using folic acid-conjugated oligochitosansJ Nanosci Nanotechnol200662860286617048492
- LavertuMMethotSTrankhanhNBuschmannMHigh efficiency gene transfer using chitosan/DNA nanoparticles with specific combinations of molecular weight and degree of deacetylationBiomaterials200627274815482416725196
- MansouriSCuieYWinnikFCharacterization of folate chitosan-DNA nanoparticles for gene therapyBiomaterials20062792060206516202449
- YousefpourPAtyabiFVasheghani-FarahaniEMovahediAADinarvandRTargeted delivery of doxorubicin-utilizing chitosan nanoparticles surface-functionalized with anti-Her2 trastuzumabInt J Nanomedicine201161977199021976974
- LinAChenJLiuYPreparation and evaluation of N-caproyl chitosan nanoparticles surface modified with glycyrrhizin for hepatocyte targetingDrug Dev Ind Pharm200935111348135519832635
- KimJHKimYSParkKAntitumor efficacy of cisplatin-loaded glycol chitosan nanoparticles in tumor-bearing miceJ Control Release20081271414918234388
- MinKHParkKKimYSHydrophobically modified glycol chitosan nanoparticles-encapsulated camptothecin enhance the drug stability and tumor targeting in cancer therapyJ Control Release2008127320821818336946
- YaoQStudy on the Two-Ligand Modified Chitosan Nanoparticles Actively Targeting to Malignant Liver Cells [PhD thesis]Chengdu, People’s Republic of ChinaSichuan University. Doctoral Paper of Sichuan University2006 Available from: http://www.globethesis.com/?t=1104360185994553Accessed December 31, 2015
- TabataYIkadaYMacrophage phagocytosis of biodegradable microspheres composed of L-lactic acid/glycolic acid homo- and copolymersJ Biomed Mater Res198822108378583220838
- TiyaboonchaiWChitosan nanoparticles: a promising system for drug deliveryNaresuan Univ J20031135166
- GuoSHuangLNanoparticles escaping RES and endosome: challenges for siRNA delivery for cancer therapyJ Nanomater2011201111221808638
- OwensDIIIPeppasNOpsonization, biodistribution, and pharmacokinetics of polymeric nanoparticlesInt J Pharm200630719310216303268
- PatelKShrimankerMDaveRModiHAnandJBhadaniSPreparation and in vivo study of doxorubicin HCl loaded chitosan nanoparticles prepared by W/O emulsion methodInt J Curr Res2012412438440
- HuoMZhangYZhouJSynthesis and characterization of low-toxic amphiphilic chitosan derivatives and their application as micelle carrier for antitumor drugInt J Pharm20103941–216217320457237
- AshfordMBiopharmaceutical principles of drug deliveryAultonMEPharmaceutics: The Science of Dosage Form Design2nd edNew York, NYChurchill Livingstone2001211253
- ChangCHLinYHYehCLNanoparticles incorporated in pH-sensitive hydrogels as amoxicillin delivery for eradication of Helicobacter pyloriBiomacromolecules201011113314219924885
- GisbertJPTorradoGTorradoSOlivaresDPajaresJMClinical trial evaluating amoxicillin and clarithromycin hydrogels (chitosan-polyacrylic acid polyionic complex) for H. pylori eradicationJ Clin Gastroenterol200640761862216917404
- DhaliwalSJainSSinghHPTiwaryAKMucoadhesive microspheres for gastroretentive delivery of acyclovir: in vitro and in vivo evaluationAAPS J200810232233018523891
- WeiWMaGHWangLYWuJSuZGHollow quaternized chitosan microspheres increase the therapeutic effect of orally administered insulinActa Biomater20106120520919505595
- HejaziRAmijiMChitosan-based gastrointestinal delivery systemsJ Control Release20038915116512711440
- VarshosazJJaffarian DehkordiAGolafshanSColon-specific delivery of mesalazine chitosan microspheresJ Microencapsul20062332933916801244
- MladenovskaKRaickiRSJanevikEIColon-specific delivery of 5-aminosalicylic acid from chitosan-Ca-alginate microparticlesInt J Pharm20073421–212413617590293
- OosegiTOnishiHMachidaYNovel preparation of enteric-coated chitosan–prednisolone conjugate microspheres and in vitro evaluation of their potential as a colonic delivery systemEur J Pharm Biopharm20086826026617703928
- JainSKJainAGuptaYAhirwarMDesign and development of hydrogel beads for targeted drug delivery to the colonAAPS PharmSciTech20078E5617915806
- ElzatahryAAEldinMSMPreparation and characterization of metronidazole loaded chitosan nanoparticles for drug delivery applicationPolym Adv Technol20081917871791
- KublikHVidgrenMTNasal delivery systems and their effect on deposition and absorptionAdv Drug Deliv Rev19982915717710837586
- CostantinoHRIllumLBrandtGJohnsonPHQuaySCIntranasal delivery: physicochemical and therapeutic aspectsInt J Pharm200733712417475423
- BaigAMKhanNANovel chemotherapeutic strategies in the management of primary amoebic meningoencephalitis due to Naegleria fowleriCNS Neurosci Ther201420328929024456292
- McMartinCHutchinsonLEHydeRPetersGEAnalysis of structural requirements for the absorption of drugs and macromolecules from the nasal cavityJ Pharm Sci1987765355402889824
- VarshosazJSadraiHAlinagariRNasal delivery of insulin using chitosan microspheresJ Microencapsul20042176177415799226
- Fernandez-UrrusunoRRomaniDCalvoPVila-JatoJLAlonsoMJDevelopment of a freeze-dried formulation of insulin-loaded chitosan nanoparticles intended for nasal administrationSTP Pharma Sci19999429436
- JiangHLKangMLQuanJSThe potential of mannosylated chitosan microspheres to target macrophage mannose receptors in an adjuvant-delivery system for intranasal immunizationBiomaterials200829121931193918221992
- KangMLJiangHLKangSGPluronic F127 enhances the effect as an adjuvant of chitosan microspheres in the intranasal delivery of Bordetella bronchiseptica antigens containing dermonecrotoxinVaccine200725234602461017485148
- HolmgrenJCzerkinskyCMucosal immunity and vaccinesNat Med2005114S45S5315812489
- de la FuenteMRaviñaMPaolicelliPSanchezASeijoBAlonsoMJChitosan-based nanostructures: a delivery platform for ocular therapeuticsAdv Drug Deliv Rev201062110011719958805
- De CamposAMSanchezAAlonsoMJChitosan nanoparticles: a new vehicle for the improvement of the delivery of drugs to the ocular surface. Application to cyclosporin AInt J Pharm20012241–215916811472825
- WadhwaSPaliwalRPaliwalSRVyasSPHyaluronic acid modified chitosan nanoparticles for effective management of glaucoma: development, characterization, and evaluationJ Drug Target201018429230219943753
- PurasGZarateJAcevesMLow molecular weight oligochitosans for non-viral retinal gene therapyEur J Pharm Biopharm201383213114023059418
- KlausnerEAZhangZChapmanRLMultackRFVolinMVUltrapure chitosan oligomers as carriers for corneal gene transferBiomaterials20103171814182019879644
- de la FuenteMSeijoBAlonsoMJBioadhesive hyaluronan-chitosan nanoparticles can transport genes across the ocular mucosa and transfect ocular tissueGene Ther200815966867618305575
- GronebergDAWittCWagnerUChungKFFischerAFundamentals of pulmonary drug deliveryRespir Med20039738238712693798
- PatilJSSarasijaSPulmonary drug delivery strategies: a concise, systematic reviewLung India2012291444922345913
- EvansCMKooJSAirway mucus: the good, the bad, the stickyPharmacol Ther200912133234819059283
- PalecandaAKobzikLReceptors for unopsonized particles: the role of alveolarmacrophages scavenger receptorsCurr Mol Med2001158959511899233
- ChonoSTaninoTSekiTMorimotoKInfluence of particle size on drug delivery to rat alveolar macrophages following pulmonary administration of ciprofloxacin incorporated into liposomesJ Drug Target20061455756617043040
- FerinJOberdorsterGSoderholmSCGeleinRPulmonary tissue access of ultrafine particlesJ Aerosol Med199145768
- GrenhaARemunan-LopezCCarvalhoELSeijoBMicrospheres containing lipid/chitosan nanoparticles complexes for pulmonary delivery of therapeutic proteinsEur J Pharm Biopharm2008691839318166446
- SungJCPadillaDJGarcia-ContrerasLFormulation and pharmacokinetics of self-assembled rifampicin nanoparticle systems for pulmonary deliveryPharm Res20092681847185519407933
- BharatwajBWuLWhittum-HudsonJAda RochaSRThe potential for the noninvasive delivery of polymeric nanocarriers using propellant-based inhalers in the treatment of Chlamydial respiratory infectionsBiomaterials201031287376738520615546
- GrenhaAGraingerCIDaileyLAChitosan nanoparticles are compatible with respiratory epithelial cells in vitroEur J Pharm Sci2007312738417408932
- HuangYCVieiraAHuangKLYehMKChiangCHPulmonary inflammation caused by chitosan microparticlesJ Biomed Mater Res A200575228328716059899
- ChoiMChoMHanBSChitosan nanoparticles show rapid extrapulmonary tissue distribution and excretion with mild pulmonary inflammation to miceToxicol Lett2010199214415220816729
- RodriguesSCordeiroCSeijoBRemunán-LópezCGrenhaAHybrid nanosystems based on natural polymers as protein carriers for respiratory delivery: stability and toxicological evaluationCarbohydr Polym201512336938025843870
- PaudelKSMilewskiMSwadleyCLBrogdenNKGhoshPStinchcombALChallenges and opportunities in dermal/transdermal deliveryTher Deliv2010110913121132122
- BosJDMeinardiMMThe 500 Dalton rule for the skin penetration of chemical compounds and drugsExp Dermatol2000916516910839713
- KimDGJeongYIChoiCRetinol-encapsulated low molecular water-soluble chitosan nanoparticlesInt J Pharm200631913013816713152
- HasanovicAZehlMReznicekGValentaCChitosan-tripolyphosphate nanoparticles as a possible skin drug delivery system for aciclovir with enhanced stabilityJ Pharm Pharmacol2009611609161619958582
- Ozbas-TuranSAkbugaJSezerADTopical application of antisense oligonucleotide-loaded chitosan nanoparticles to ratsOligonucleotides20102014715320180684
- Ozbas-TuranSAkbugaJPlasmid DNA-loaded chitosan/TPP nanoparticles for topical gene deliveryDrug Deliv20111821522221226549
- UsmanMSEl ZowalatyMEShameliKZainuddinNSalamaMIbrahimNASynthesis, characterization, and antimicrobial properties of copper nanoparticlesInt J Nanomedicine201384467447924293998
- SanpuiPMurugadossADurga PrasadPVGhoshSSChattopadhyayaAThe antibacterial properties of a novel chitosan–Ag nanoparticle compositeInt J Food Microbiol200812414214618433906
- HonarySGhajarKKhazaeliPShalchianPPreparation, characterization and antibacterial properties of silver-chitosan nanocomposites using different molecular weight grades of chitosanTrop J Pharm Res201110169
- BhumkarDRJoshiHMSastryMPokharkarVBChitosan reduced gold nanoparticles as novel carriers for transmucosal delivery of insulinPharm Res20072481415142617380266
- SalehizadehHHekmatianESadeghiMKennedyKSynthesis and characterization of core-shell Fe3O4-gold-chitosan nanostructureJ Nanobiotechnology201210310.1186/1477-3155-10-322221555
- QiLXuZJiangXHuCZouXPreparation and antibacterial activity of chitosan nanoparticlesCarbohydr Res2004339162693270015519328
- TokarekKHuesoJLKuśtrowskiPStochelGKyziołAGreen synthesis of chitosan-stabilized copper nanoparticlesEur J Inorg Chem201320132849404947
- ChattopadhyaySDashSKKar MahapatraSChitosan-modified cobalt oxide nanoparticles stimulate TNF-α-mediated apoptosis in human leukemic cellsJ Biol Inorg Chem201419339941424445996