Abstract
Graphene, a wonder material, has made far-reaching developments in many different fields such as materials science, electronics, condensed physics, quantum physics, energy systems, etc. Since its discovery in 2004, extensive studies have been done for understanding its physical and chemical properties. Owing to its unique characteristics, it has rapidly became a potential candidate for nano-bio researchers to explore its usage in biomedical applications. In the last decade, remarkable efforts have been devoted to investigating the biomedical utilization of graphene and graphene-based materials, especially in smart drug and gene delivery as well as cancer therapy. Inspired by a great number of successful graphene-based materials integrations into the biomedical area, here we summarize the most recent developments made about graphene applications in biomedicine. In this paper, we review the up-to-date advances of graphene-based materials in drug delivery applications, specifically targeted drug/ gene delivery, delivery of antitumor drugs, controlled and stimuli-responsive drug release, photodynamic therapy applications and optical imaging and theranostics, as well as investigating the future trends and succeeding challenges in this topic to provide an outlook for future researches.
Introduction
Several shortcomings of traditional therapeutics and low efficacy of drugs for in vivo administration have resulted in the progress of nanobiomedicine and modern drug delivery platforms. These drawbacks include abrupt metabolization and exertion of drugs from the body before operation on their targeted sites, poor solubility of some drugs in aqueous medium, non-specific targeting, low retention effectiveness on deceased regions and adverse effects on normal cells. However, recent progress of nanotechnology and nanomaterials have provided a bright prospect in the biomedicine area, namely a better bio-distribution of drugs, reduced adverse effects on healthy cells, specific selectivity, and higher local therapeutic absorption with the help of newly proposed drug delivery systems. During the last decades, many nanomaterials have been explored by researchers in the nanobiomedicine field including polymeric nanoparticles, micelles, liposomes, mesoporous inorganic nanoparticles, metal oxides, noble materials, carbon nanomaterials etc.Citation1–Citation9 Besides the mentioned nanomaterials, the newly proposed two-dimensional nanomaterials have attracted the attention of many scientists because of their unique properties like their very high surface area, biocompatibility, and easy modification.
Graphene, the so-called two-dimensional material is a uniformed flat monolayer of carbon atoms, packed tightly into a hexagonal, honeycomb structure. It is the basic structure of other zero-dimensional (fullerene, graphene quantum dots), one dimensional (CNTs) and three dimensional (Graphite) allotropes of carbon.Citation10 In this material, each carbon atom is attached to three other carbon atoms by making three hybridized spCitation2 bonds while one out-of-plane p orbital provides the electron delocalization network.Citation3 The extremely interesting properties of graphene such as its great mechanical strength, high electrical, thermal and magnetic properties, and unique optical properties have made it a potential candidate to be used in several fields of engineering.Citation2–Citation4,Citation11 Specifically, the acceptable biocompatibility, very large surface areas, great optical properties together with straightforward bio-functionalization of graphene and its derivatives (graphene oxide (GO) and reduced graphene oxide (rGO)) have engrossed several researchers in several fields of biomedical engineering like drug/ gene delivery and tissue engineering.Citation12,Citation13
In 2008, Liu et al14 for the first time explored the graphene capabilities in biological systems where they showed the successful non-covalent attachment of a water-insoluble, aromatic drug (SN38) to the PEGylated graphene oxide sheets by Van der Waals interaction.Citation14 Since then, the research on graphene-based materials for biomedical applications, especially drug delivery systems, has grown exponentially demonstrating their high potential in this field. However, being a water-insoluble material limits the biomedical applications of graphene, mostly cell works. Graphene oxide (GO) and reduced graphene oxide (rGO), are functional derivatives of graphene which possess attractive properties like being water-soluble, emitting visible and near-infrared fluorescence as well as having other graphene characteristics important in drug/gene delivery.Citation15
Nanoscale graphene-based materials have also been extensively used for designing targeted and stimuli-responsive drug delivery systems through either external stimulus (such as temperature, light, ultrasound waves, magnetic and electric fields), internal stimulus (such as pH, redox and enzymes) or multi-stimuli-responsive drug delivery systems bringing a controlled smart drug delivery with higher bioactivity, better temporal and spatial control at lower dosages of therapeutic agents, together with decreased toxicity and other adverse side effects.Citation16 Therefore, functionalized graphene-based materials are promising candidates to be considered for designing smart drug delivery systems and especially for cancer therapy. Furthermore, therapeutic agents like anticancer drugs, proteins, gene and photosensitizers demonstrate a higher efficiency both in diagnosis and treatment of diseases when combined with graphene-based materials.Citation17–Citation19
Recently, in a comprehensive study we could review and discuss developments in graphene and graphene oxide; properties, synthesis, and modifications.Citation10 In this review paper, we will investigate the most recent progress in biomedical usage of graphene and graphene-based materials, specifically targeted drug/gene delivery, delivery of antitumor drugs, smart and controlled stimuli-responsive drug release. Also, we will explore the developments in photodynamic therapy applications, optical imaging and theranostics based on the graphene materials with a perspective toward the upcoming challenges and trends in this area.
Drug Delivery Applications
The main goal of designing an optimized drug delivery system (DDS) is to deliver therapeutic agents inside the diseased region in a controllable manner while having fewer side effects on healthy tissues. Due to poor solubility of some chemotherapy drugs in water as well as lack of specificity in drug localization into a tumor, they often have drastic side effects on non-targeted tissues. In the past few decades, the development in nanotechnology has bypassed some difficulties in this area by introducing nano-sized drug carriers with efficient applications in DDS.Citation20 Among various nano-carriers, graphene and some derivatives particularly GO have been widely investigated in the field of nanomedicine as bacterial inhibitors,Citation21 imaging contrast agentsCitation22 and drug/gene delivery systems,Citation14,Citation23 due to appropriate expanded surface area, biocompatibility and easiness of surface functionalization.Citation24,Citation25 Moreover, the high near-infrared (NIR) wavelengths absorbance capacity of graphene nanostructures is providing an opportunity for utilizing them as photo-thermal therapy agents.Citation26,Citation27 Therefore, by merging both potentials of carrying drugs and enhanced photo-heat conversion, these graphene-based nanomaterials (GBNs) have a synergistic effect to be applied as heat-responsive nano-sized drug carriers in therapeutic applications like cancer.Citation28–Citation30 However, other types of modified graphene-based nanoplatforms loaded with therapeutic agents could be recognized as stimuli-responsive structures for efficient drug delivery.Citation31 In this regard, a number of studies about stimuli-responsive graphene-based nanoparticles are reviewed in the next section according to types of environmental stimulus (ie, pH, redox, temperature and magnetic field and light). For better judgment, a list of some remarkable studies with their pros and coins are presented in .
Table 1 Advantages and Limitations of Some Remarkable Published Studies About Functionalized Graphene Oxide Nanoparticles
Targeted Drug Delivery
At present, we are faced with numerous drug delivery systems as well as targeting methods presented through a wide variety of investigations.Citation39 Like other nano-systems which have been utilized for drug delivery, graphene-based materials have the potential to be applied for designing targeted DDSs. Zhang et al40 have used a novel strategy using histidine (His) for mediating the hydrothermal expansion of amorphous zinc oxide (a-ZnO) shells on nanoparticles (NPs) of gold (Au-His@a-ZnO). With the aid of carbodiimide crosslinker chemistry, they integrated Au-His@a-ZnO NPs onto the plane of PEGylated GO (PEG-GO).Citation40 In this work, Apt@GO@Au-His@a-ZnO@DOX (Apt is aptamer) nanocomposites were created and indicated high carrying capacity of doxorubicin (DOX) along with near-infrared/pH-sensitive release of drugs so that the metal-drug complex dissociated to free the anticancer ions of ZnCitation2+ in the low pH environment (endosome/lysosome of tumor cell). Moreover, they demonstrated fine biostability and aptamer-promoted attaching to lung tumor cells. The specific binding promoted the cellular uptake into EGFR-mutated tumor site (EGFR is epidermal growth factor receptor) in comparison to non-targeted controls. This work employed A549 cells (human pulmonary adenocarcinoma cells) to turmeric mice revealing appreciable targeted drug delivery and high anti-cancer efficiency of Apt@GO@Au-His@a-ZnO nanocomposites in vivo. The multifunctional nanocomposites (Apt@GO@Au-His@a-ZnO@DOX) exploited in this research led to high efficiency in targeted, photo/chemotherapy to lung tumors.Citation40
In another effort, a quantum dot-based GO nanoparticle (GOQD) was prepared in such a manner that the nanoparticle can be a sound nanocarrier potently delivering a drug during cancer chemotherapy. To apply this DDS in cancer therapy, a nanocargo was flourished through conjugating GOQD with the chitosan functionalized folic acid (FA-CH). This group disclosed that the synthesized FA-CH-GOQD is a favorable biocompatible nanocarrier for targeted DOX delivery with high therapeutic efficiency.Citation41
A folic acid (FA) targeted system based on Go-coated Fe3O4 nanocomposites used is for MRI and Dox delivery. The system abbreviated as FA-Fe3O4@nGO-DOX could be fruitful and stable material for targeted drug delivery.Citation42 The cell viability investigations into FA-Fe3O4@nGO-DOX nanoplatforms were conducted and exhibited Cellosaurus cell line/MGC-803 selective uptake. Selective killing of tumor cells was verified as a result of pH-mediated drug release and selective uptake of the nanoparticles through MGC-803 cells using the folic acid receptor.
Also, it has been reported that in support of tumor cell detection plus targeted release of DOX towards HeLa cells, graphene quantum dots and Fe3O4 nanocomposites conjugated with lectin protein concanavalin A, might be an auspicious candidate. The whole system was abbreviated as GQD-ConA@Fe3O4. It has been shown that drug amounts in HeLa cells increase to more than twice when there is an external magnetic field, which is caused by Fe3O4 incorporation into the nanocarriers. Apart from that, the targeting role of Concanavalin-A in the nanoparticle was certified by proving the vulnerability of HeLa cells to the drug which was 13% more, compared to normal cells.Citation43
An aptamer-conjugated magnetic GO (MGO) nanocarrier was used for targeting delivery of paclitaxel. MGO nanocarriers had formed in consequence of Fe3O4 attachment on GO layer followed by joining aptamer as a targeting moiety. Furthermore, an anticancer drug, paclitaxel was loaded on the nanocarrier in such a way that this loading as well as in vitro release results unveiled excellent loading possessing high pH-responsive release and entrapment efficiency of 95.75%. By considering cellular toxicity assay, it was found that MGO nanocarriers were biocompatible with the cell death less than 20% for fibroblast L-929 cells. However, high cell death was reported for paclitaxel and paclitaxel-loaded MGO on MCF-7 tumor cells at different dosages of the drug. Based on the analysis of flow cytometry, it has been discerned that the nanocarrier is capable of binding to MCF-7 cancerous cells. It was deduced that the created superparamagnetic nanocarrier can be a propitious agent for cancer drug delivery.Citation44
An improved fluorinated GO (FGO) as an immensely significant drug carrier with the following properties: 1) high capacity of drug loading; 2) switchable fluorescence; 3) water-soluble; 4) controlled and pH-responsive drug release; 5) high NIR absorption; 6) optimized photothermal effects; 7) targeted drug delivery; and 8) nanoscale structure was prepared. At the outset, by applying designed oxygen introduction into the nanosheets, FGO was well modified with folic acid as a targeting agent. Additionally, through the adjustment of fluorinated graphene into a nanoscale structure with SP2 carbon, the photothermal performance was bettered. It is noteworthy that the infinitesimal size of FGO is an advantage for endocytosis into cells. DOX was promptly loaded onto FGO–FA (FA is folic acid). FA guarantees target delivery of the nanoparticle to cancer cells with FA receptor and the rest of the particle triggers release of the DOX under intracellular acid condition.Citation45
In a three-parted system named GONs−FITC−ATP (GON: graphene oxide nanosheets, FITC: fluorescein isothiocyanate, and ATP: adenosine-5′-triphosphate) energy transfer between GO, FITC and ATP were pointed out. In the light of this article, FITC is substantially quenched by ATP and through drawing a comparison between the quenching of FITC by GONs and FITC-ATP, it was understood that the latter is far weaker due to the formation of supramolecular structures formed by hydrogen bonds and π−π interactions. In this research, a model of chemical adsorption of ATP molecules on GON surfaces together with partial blocking of FITC quenching by exploring complex characteristic of the GONs−FITC−ATP ternary system was studied. Accordingly, it was stated that in the case of FITC quenching by GONs, ATP has the role of a moderator. Understanding the mechanism of interaction of FITC labeled probe with ATP could be recognized as key in designing targeted systems.Citation46
It was elaborated on a targeted drug delivery system based on graphene quantum dot (GQDs) linked to biotin as a targeting module, for the delivery of DOX to tumor cells. It was observed that GQDs were biocompatible and the biotin-linked GQDs loaded with DOX proved to have greater uptake rather than free DOX or GQDs-DOX in A549 cell line.Citation47
A system based on decorated magnetic nanoparticles onto GO layered by mesoporous silica was developed for camptothecin (CPT) delivery. The carriers have a capacity of 20% regarding drug loading and showed a controlled and pH-responsive drug release. In this study, it was realized that the synthesized structures were considerably successful against HeLa cells. Moreover, under suitable magnetic properties along with high adsorption capacity, it was deemed a beneficial and versatile nanoplatform with the aim of tumor chemotherapy using magnetic targeting.Citation47
Delivery of Anti-Cancer Drugs
Among the astonishing properties of graphene and GO nanomaterials, their high surface area makes them an appropriate selection for nanotherapy. Several reports are published on utilizing these materials, for delivery or co-delivery of anti-cancer drugs.
Naturally functionalized GO by chitosan is an appropriate candidate for being used in nanomedicine by good biocompatibility to co-deliver CPT and 3,3′-Diindolylmethane (DIM).Citation48 As these two drugs possess diverse performances, their co-delivery engenders combined influence upon breast cancer. Moreover, via anti-inflammatory assays and hemolysis, the biocompatibility was assayed. The cellular toxicity was determined by 3-(4, 5-dimethyl-2-thiazolyl) −2, 5-diphenyl-2H- tetrazolium bromide (MTT), sulforhodamine B (SRB) and cell death assays toward MCF-7 cell lines together with some other in vivo studies like evaluating the blood biochemical analysis, the biodistribution of the drug, and bioavailability of the drug. Accordingly, it was deduced that loading of the two drugs onto the nanocarrier in conjunction with each other causes the anticancer activity to grow considerably. Besides, in vivo assessment showed that DIM masked the toxic impacts arising from CPT very well.
In another report, Ginsenoside Rh2 (Rh2) which is a new natural-based anticancer agent was treated with amino-acid modified GO (ie, lysine-Go & arginine-GO). The MTT assay on cancer cells (ie, MDA-MB/breast cancer, OVCAR3/ovarian cancer, and A375/human melanoma) and MSCs/human mesenchymal stem cells were carried out and showed promising anti-cancer activity on cancer cell lines and lower toxicity on MSCs.Citation49
Functionalized GO is introduced as a suitable carrier for DDSs. It was displayed that polyvinyl pyrrolidone (PVP) and ß-cyclodextrin modified GO (ie, PVP-GO and ß-CD-GO) could preserve SN-38, as an anti-cancer drug. The cytotoxic assay on breast cancer/MCF-7 cells showed that the cytotoxicity of free SN-38 was lower than SN-38 loaded on these structures. In comparative studies, PVP-GO/SN-38 showed higher masking action rather than ß-CD-GO/SN-38 and free SN-38.
Embedded graphene quantum dots/GQDs on CMC (carboxymethyl cellulose) hydrogel film showed high capacity in loading DOX and a long release pattern. Further, these films have demonstrated minor toxicity against blood cancer cells (K562) while it was asserted that DOX-loaded CMC/GQD nanocomposite could be exploited as a proper anti-cancer material.Citation50
In a noteworthy strategy, P-glycoprotein expression and multidrug resistance/MDR problems involved in cancers, specifically breast cancer, were treated by a multiplexed gene silencing system tailored by two molecular beacons (MBs) embedded in GO and loaded with DOX. The nanosystem can release DOX by the low pH endosomes while up-taken by MDR/breast cancer cells and could silence the MDR1 gene and erythroblastosis virus E26 oncogene homolog 1 gene by MBs hybridizing with target sequences, plus it is capable of hindering DOX efflux transporter of P-glycoprotein expression and causing the progression of MDR breast cancer to desist.Citation51
A novel nanosystem of drug delivery comprising GO-PRM/SA (PRM is an abbreviation for natural peptide protamine sulfate and SA represents sodium alginate) has been synthesized using layer-by-layer self-assembly technique and electrostatic attraction to make DOX delivery more efficacious. The employed modification resulted in the improvement of GO nanosheets to be more stable and dispersible under pH of the physiological environment. GO-PRM/SA-DOX indicated successful pH-responsive drug delivery behavior. GO-PRM/SA nanocomposites could enter into the cytoplasm of MCF-7 cells because of two features; minute particle size and high water dispersibility.Citation52
In controlling mass diffusion drug delivery via hydrogels, GO could be properly utilized. In an experiment, the diffusion of a peptide through a network of GO membranes and the so-called GO-embedded hydrogels were assessed. They posit that size and density of graphene oxide effect on the drug release rate in a way that their optimization could lead to the peptide release rates possessing high efficiency.Citation53
CMC-modified graphene acts as a pH-sensitive carrier for DOX delivery. The platform could interact with drug via hydrogen bonding and π–π stacking. The system showed high antitumor activity while in vitro assessment was performed on cervical cancer/Hela and fibroblast/NIH-3T3 cells.Citation54
Another synthesized drug delivery system (GO-PEG-FA-CPT) has been announced consisting of GO nanomaterials, an anticancer drug (CPT), polyethylene glycol (PEG), and folic acid (FA). This nanosystem disclosed a pH-responsive CPT delivery. By MTT assay on MCF-7 breast cancer cell lines, the antitumor efficacy of the drug delivery system was monitored which was satisfactory for being undertaken.Citation55 In a similar strategy, CPT loaded GO-PEG-FA. The system demonstrated a high drug-loading capacity of 37.8%. In vitro investigations showed a lengthy drug release of 200 hours. It was found that the drug release was more gradual at acidic pH in comparison to physiological pH. Herein, two cell models of J774 (a high proliferation rate and macrophage phenotype tumor cell) and HepG2 (folate transporter containing human hepatocellular carcinoma cancer cell) were tested. The cytotoxicity of GO-FA varies on different cell types and did not show much cytotoxicity to J774, whereas there was substantial toxicity to HepG2. Moreover, GO-FA/CPT nanocarrier improved the killing of tumor cells by apoptosis.Citation56
Controlled and Stimulated Responsive Drug Release
pH-Responsive Graphene-Based DDSs
It is well-known that there is a somewhat broad range of pH values between various organs (from about 2 for the stomach to 7 for the mouth) in the body as well as between micro-organisms at subcellular level (lysosomes ~4 and cytosol~7.4). Particularly, due to the abnormal characteristics of cancer cells and subsequent accumulation of proton in the extracellular matrix of the cells, the regions around tumors have acidic pH which has motivated the researchers to design pH-sensitive DDSs.Citation57–Citation59 The main drug-loading mechanism on GO nanoplatforms is based on simple physisorption either by π-π stacking or hydrophobic interactions. After exposure to the acidic environment, such drug molecules and the graphene surface links are weakened owing to the protonation of hydrophobic drugs. As a result, drug release had occurred at lower pH due to hydrophilicity and solubility increment of a drug.Citation60
As the pioneering work in pH-responsive DDS with GNBs, Sun et al synthesized theranostic polyethylene glycol grafted GO (PEG-GO) as a nano-carrier of cancer therapy of DOX. They showed the PEG-GO nanostructure releases about 40% of loaded DOX in an acidic solution (pH=5.5) after a day while only 15% of the drug is released in a physiological pH=7.4 over 2 days. Moreover, they conjugated anti-CD20 (Rituxan) antibody to the PEG-GO for a specific bond to B-cells and achieved sharp NIR fluorescence images from the cells. Cytotoxicity assay results showed an increase in cell growth inhibition rate from 20% to 80% for non-Rituxan and Rituxan containing PEG-GO/DOX nanostructures.Citation22 In another study, poly (2-(diethylamino) ethyl methacrylate) (PDEMAEMA) as the pH-sensitive part was grafted on to GO and loaded by camptothecin (CPT) as an antitumor drug. The pH-triggered delivery of the drug was examined in buffers with pH 5.5, 7.4 and 9.0. The PDEMAEMA-GO nanocarriers had the maximum release of CPT in the pH of 5.5 over two days (~%60) while in higher pH solutions CPT release was very negligible. Additionally, the cytotoxicity test revealed that the PDEMAEMA-GO has no toxicity effect on N2a cells in contrast to PDEMAEMA-GO-CPT with ~%70 growth inhibitory effect.Citation60
To have both targeting therapy and imaging of hepatoma carcinoma cells simultaneously, cyclic arginyl-glycyl-aspartic acid (cRGD) and chitosan as a targeting moiety (RC) were conjugated and so coated on DOX-loaded GO as a drug carrier to form RC-GO-DOX (). It is found that the drug release is pH-dependent so that the release process was greater for lower pH than higher pH. In addition, targeting ability assay that functionalization of fluorescein isothiocyanate (FITC) conjugated RC-GO-DOX endowed it with an obvious enhancement of green fluorescence intensity in images of over-expressing integrin αvβ3 cell line, Bel-7402.Citation61
Figure 1 Schematic of (A) cRGD conjugated to chitosan (RC) and (B) RC-GO-Dox nanocarrier.
Note: Copyright ©2014. Elsevier. Reproduced from Wang C, Chen B, Zou M, Cheng G. Cyclic RGD-modified chitosan/graphene oxide polymers for drug delivery and cellular imaging. Colloids and Surfaces B: Biointerfaces. 2014; 122: 332–340.Citation61
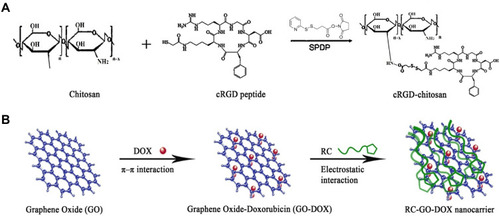
Recently, modified PEG with 2, 3-dimethylmaleic anhydride (DA)-PEG-GO nanocomposite was fabricated to carry and release DOX as a pH-dependent charge reversal DDS. They reported the uptake of PEG-GO-DA in MCF-7 cells elevates under acidic pH 6.8 because of nanocomposite charge switching to positive. Meanwhile, the cell-killing ability of PEG-GO-DA/Dox is improved under this pH. Taking advantage of high NIR absorbance of GO nanostructures, PEG-GO-DA/DOX in combination with 808 nm NIR laser exhibited more power to destroy the cancer cells.Citation62
A novel receptor-mediated nanoplatform of GO was functionalized by targeting and apoptosing activator agents; tumor necrosis factor-related apoptosis-inducing ligand (TRAIL), provided with DOX to achieve a synergistic antitumor effect.Citation63 As shown in , after gathering the nanoplatforms near the tumor site, they enveloped the cell surface via binding of TRAIL on the corresponding receptors. Afterward, the Furin cleaves the peptide linker and apoptosis trigger factor; TRAIL induces caspase-mediated apoptosis death. Finally, the DOX contained GOs are internalized into the cytoplasm and under low pH of endosomes, drug release is commenced.Citation63
Figure 2 Scheme of TRAIL/DOX-fGO nanoplatform. (A) Main components of TRAIL/DOX-fGO, and (B) Step by step activity of TRAIL/DOX-fGO, from vessel administration to drug release in the cell nucleus.
Note: Copyright ©2015. John Wiley and Sons. Reproduced from Jiang T, Sun W, Zhu Q, et al. Furin‐Mediated Sequential Delivery of Anticancer Cytokine and Small‐Molecule Drug Shuttled by Graphene. Advanced Materials. 2015; 27 (6):1021–1028.Citation63
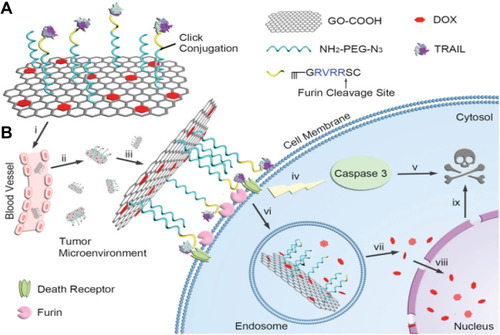
Using Pluronic F127 (PF127) as a hydrophilic surfactant, PF127/graphene nano-sheets were assembled which can load DOX up to 289% (W/W) also, release it 56% and 25% in acidic and basic conditions, respectively. The result of water-soluble tetrazolium salts (WST) assay showed that even for high concentrations of PF127/graphene nano-sheets, their toxicity is negligible while PF127/graphene loaded DOX nano-sheets with the same concentration brings the MCF-7 cell proliferation inhibitory up to 50%.Citation27
In another pH-responsive system, dual functionalized GO nanocomposite was fabricated as a carrier of DOX. The DDS was equipped by two well-known biostructures: carboxymethyl chitosan (CMC); with high biocompatibility and solubility in water as well as drug release sensitivity to low pH, and lactobionic acid (LA); a disaccharide containing galactose and gluconic acid to target hepatocytes. It was revealed that the drug release profile has pH dependency so that it reaches up to about 45% in pH 5.8 against 20% in phosphate buffer saline (PBS) with pH 7.4 after 72 hours. Moreover, by coupling FITC as a tracker of the nanocarrier, it was found that this DOX DDS platform can target hepatocellular carcinoma SMMC-7721 cells more than L929 cells.Citation64
RGD-linked GQDs containing DOX had proven to be a potent tool to carry the drug and monitor the drug release pattern into cells simultaneously. The RGD-GQD-DOX nano-carriers indicated acceptable biocompatibility, high drug release capability in acidic pH (60% vs 13% in 5 and 7.4 pH, respectively), cytotoxicity effects on DU-145 and PC-3 prostate tumor cells as well as photoluminescence ability to track drug release into the cells. The fluorescence images exhibited that DOX accumulation had occurred in the cell nucleus after 16 hours of incubation.Citation65
Application of the GQDs with fluorescent emission capability along with chitosan xerogel coating by loading sodium salicylate (SS) as a drug for wound healing and inflammation treatment has been well studied before.48 They revealed that the nano-xerogel has interesting fluorescent properties so that in vitro and in vivo bio-imagings by three different emission colors of blue, green and red which are excited by various wavelengths were well monitored by UV-Vis spectroscopy. In contrast to the previously introduced system related to DOX delivery, this system released more SS in higher pH owing to the negative charge of the drug.Citation64
By combining magnetic NPs, PEG, chitosan and GO nano-sheets (mGOC-PEG), a DDS with controllable and pH-responsive delivery of both irinotecan (CPT-11) and DOX have been achieved. Based on the position of the magnet under the U87 human glioblastoma cells, mGOC-PEG/DOX showed meaningful lethal effect.Citation66
Exploiting the anticancer effect and pH responsiveness of poly(vinylpyrrolidone) nanoparticles, the exfoliated GO nanosheets were prepared. The GO-PVP-NPs affected the mitochondrial enzymatic activities, proliferation of the cells which may dominantly be attributed to the generation of ROSs. Additionally, the higher release of DOX from the exfoliated GO nanosheet in a low pH and hypoxic environment demonstrated a great potential to treat the tumors.Citation67 In another study, it is shown that modifications of GO by peptide protamine sulfate (PRM) and sodium alginate (SA) lead to better water dispersibility and stability and also lower protein absorption. A pH-responsive release pattern of DOX and higher cytotoxicity on MCF-7 cells than GO-DOX are the remarkable properties of the novel nanocarriers.Citation52
The nanocomposite of carboxymethyl cellulose (CMC) hydrogel and graphene quantum dots (GQD) with the improved ability for swelling in vitro and pH-sensitive release of DOX have been introduced as a theranostic nanoagent for the K562 cancer cells.Citation50 In a similar work, the GQD-cross-linked chitosan was loaded by a model drug (sodium salicylate) and encapsulated in CMC. The nanocomposite in the gastrointestinal tract conditions showed high stability and controlled release pattern of the drug.Citation68 Focusing on the fluorescent properties of GQDs, the DOX-GQD-RGD was developed to real-time screening of the cellular uptake process as well as drug release location vs the time. The nano-DDS caused the delivery of the DOX into the nucleus of the U251 glioma cells which was followed by increases in cell death.Citation69
In the field of bone cancer treatment, graphene oxide (GO)/hydroxyapatite (HAP)/chitosan (CS) composite was loaded with cisplatin (CDDP). This structure was applied to hinder osteosarcoma cells development and enhance osteoblast cells growth. The GO/HAP/CS-3/CDDP nanocomposites revealed higher cytotoxicity against cancer cells while they showed high viability on osteoblast-like cells and a promising application as a replacement for cancer-affected bone tissues.Citation70 In breast cancer therapy, camptothecin (CPT) chemo drug and magnetic nanoparticles were attached to reduced graphene oxide surface, which was cross-linked with 4-hydroxycoumarin (4-HC) photosensitizer through an allylamine (AA) linker. The CPT-loaded MrGO-AA-g-4-HC exhibited superior toxicity effect towards the breast cancer cells in comparison with normal cell lines, resulting in remarkable cancer cell death.Citation71 Another study used modified graphene oxide-methyl acrylate (GO-g-MA) conjugated with folic acid in which paclitaxel (PTX) was attached to GO-g-MA/FA nanocarrier by hydrophobic interaction and π-π stacking. GO-g-MA/FA-PTX nanocarrier revealed 39% cytotoxic effect that inhibited breast cancer cell growth and decreased size of the tumor. In addition, this system effectively alleviated the mitochondrial dysfunction during breast cancer.Citation72 In the cervical cancer field, κ-Carrageenan-loaded graphene oxide in conjunction with biotin was applied for cervical cancer treatment. The anticancer drug doxorubicin was grafted on GO-κ-car-biotin and demonstrated substantial cell death of cancer cells.Citation73 In order to improve the curative and permeability of bioactive materials in a drug delivery system, protein-polymeric carriers have been used. In a recent study graphene oxide was functionalized by means of egg white protein of ovalbumin (OVA) and polymethyl methacrylate (PMMA) and was loaded with doxorubicin on this DDS. The OVA–PMMA–GO–DOX system revealed successful loading and controllable release of the drug.Citation74
In a recent study, ultra-small SPIONs were grown on the GO nanosheets and finally were loaded by a modified form of DOX (CAD). The nano-sized theranostic agent proved a high antitumor effect and high-resolution MR imaging in-vivo.Citation75
More recently, PEG4000 as a hydrophilic molecule was grafted on the GO nanosheets to elevate the biocompatibility of GO. The DOX release efficiency of the nanosheets was higher for lower pH than neutral pH due to H-bonding changes between the drug and nanocarrier.Citation76 Beside DOX and CPT, indomethacinCitation77 and 5-fluorouracilCitation34 are also loaded in GBNs.
Redox-Triggered Drug Release of Graphene
Exploiting of hydrophilic structures such as PEG and poly(vinyl alcohol) (PVA) to attain further biocompatibility and prolong circulation time in blood have been extensively explored by many investigators.Citation78 Nevertheless, the application of these polymers as coating structures can disturb the drug delivery rate due to surface diffusion barriersCitation79 and so cause a decline in the release efficiency. On the other hand, the concentration of glutathione (GSH) as the main intracellular redox regulator, is about 2 µM and 10 mM for extra- and intracellular environments, respectively. Interestingly, reduced glutathione (GSH) level inside some types of cancer cells rises at least fourfold compared to normal cells.Citation80 Therefore, it seems that this significant difference in GSH level can be an appropriate factor to design DDSs. A combination of DDS nanoplatform by coating with biocompatible polymers and redox-sensitive linkage provided a system with ideal criteria of such systems. Redox responsive NGBs have also interested in designing DDSs. In an effort, PEG was linked to GO nanocarrier via disulfide (SS) linkage (NGO-SS-mPEG) while antitumor drug DOX was attached (). Disulfide linkage is susceptible to cleavage due to the high intracellular level of GSH which induces drugs to release at a higher rate in the cell. In the absence of GSH, less than 35% (over 48 hours) and only 5% (over 72 hours) of DOX left the carrier surface at pH 5.5 and 7.4, respectively, while the drug release percentage increased up to 55 and 10% upon adding 10 mM GSH. Furthermore, enhanced drug release due to S-S bond cleavage induced more growth inhibitory effect on HeLa cells.Citation81
Figure 3 (A) NGO-SS-mPEG in extracellular environment (low GSH level), (B) it is internalized into the tumor cell (high GSH level), (C) linkage is cleaved, and (D) DOX is released.
Note: Copyright ©2012. John Wiley and Sons. Reproduced from Wen H, Dong C, Dong H, et al. Engineered redox‐responsive PEG detachment mechanism in PEGylated nano‐graphene oxide for intracellular drug delivery. Small. 2012; 8 (5): 760–769.Citation81
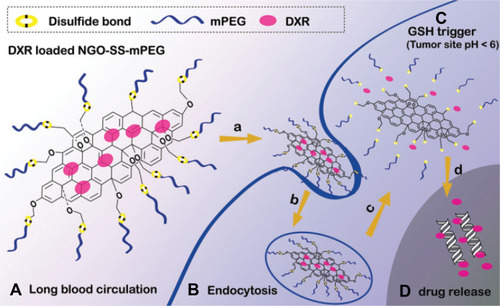
Another PEI-modified GO was applied in redox stimulated delivery by introducing disulfide linkage to PEI and conjugating DOX to this moiety. Furthermore, silver nanoparticles were loaded by GO surface. The intracellular GSH further stimulated drug release by cleavage disulfide bond. Moreover, the presence of silver nanoparticles made monitoring of this material uptake in HeLa cells possible via surface-enhanced raman scattering (SERS) technique.Citation82
A novel PEGylated GO, grafted by poly(methacrylic acid) (PMAA) and crosslinked with cystamine as disulfide linkage was introduced to carry DOX. They found PMMA crosslinking on PEG-GO can significantly prevent premature drug release until the SS bond is cleaved by GSH. In pH 5.0 and 10 mM GSH condition, the releasing rate was six times faster than pH 7.0.Citation83 Emphasizing on biocompatibility and controllable drug release of nano-carriers, GO was reduced by 2-chloro-3´,4´-dihydroxyacetophenone quaternized poly(ethylene glycol)-g-poly(dimethylaminoethyl methacrylate) (rGO/QC-PEG). Then, rGO/QC-PEG was loaded with DOX and the complex was fenced by disulfide cross-linking of thiol grafted pluronic (Plu-SH). A schematic of the release mechanism of the prepared nanocarrier is presented in .
Figure 4 The mechanism of DOX release process in acidic and high GSH level conditions from rGO/QC-PEG and rGO/QC-PEG/Plu-SH.
Note: Copyright ©2013. Elsevier. Reproduced from Al-Nahain A, Lee SY, In I, et al. Triggered pH/redox responsive release of doxorubicin from prepared highly stable graphene with thiol grafted pluronic. Int J Pharm. 2013; 450 (1): 208–217.Citation84
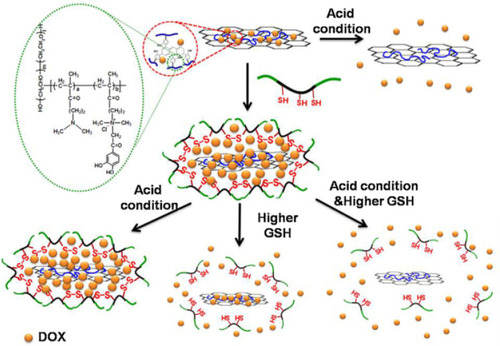
As shown in , under acidic conditions DOX is detached from rGO/QC-PEG uncontrollably. By adding redox-sensitive Plu-SH to rGO/QC-PEG, the drug is embayed by Plu-SH and is not released even in the acidic condition. Once the complex is faced with high GSH conditions, disulfide bonds of Plu-SH are broken and so entrapped drugs are released into the environment. This can be resonated in the presence of both acidic and high GSH level simultaneously.Citation84 In order to exploit the GO nano-sheets as a powerful theranostic agent activated by redox stimuli, a photosensitizer; chlorine6 (Ce6) was conjugated to GO nano-sheets via a disulfide linker to obtain GO-SS-Ce6. They declared that GO-SS-Ce6 has notable Ce6-related fluorescence intensity while disulfide bonds were cleaved by reductive agent dithiothreitol (DTT). The flow cytometry and confocal laser scanning microscopy results proved a high intracellular penetration of GO-SS-Ce6 nanostructures into A549 cell line compared with free Ce6. The GO-SS-Ce6 was able to kill about 80% of A549 cells exposure with 670 nm diode laser irradiation while free Ce6 has smaller lethal effect in the same condition.Citation85
Active targeting of GO nanosheets by hyaluronic acid (HA) via a redox-sensitive linkage imparts simultaneously some extraordinary properties to the nanosheets such as selective accumulation, higher stability in the bio-environment, drug-loading capacity, NIR absorption and better response to the glutathione presence.Citation86
By conjugating Ce6 as a photodynamic therapy agent to amine group of GO‐(NSSNH2)‐PEG, a nanocomposite was achieved which could transport Ce6 into the cells more than free Ce6. Moreover, it showed a redox-sensitive release pattern in the presence of glutathione. The animals treated by the nanocomposite had a great accumulation of Ce6 in the tumor while the liver of other groups exhibited the highest uptake of it.Citation87
In a more recent study, gefitinib (Gef) as a drug for lung cancer was linked to HA functionalized GO nanosheets. The NGO-SS-HA-Gef nanocarrier showed significant responsiveness to the high level of GSH in A549 lung cancer cells due to the disulfide linkage.Citation88 In another study, a redox responsive prodrug (PEG-PCL-SS-DOX) was coupled to the GO nanosheets to elevate bio-stability and accelerate the drug release into the cancer cells. In vivo results exhibited lower systematic toxicity and higher antitumor effect for groups treated by the nanohybrids.Citation89
Thermo-Sensitive Drug Delivery by Graphene
Another strategy to design an efficient DDS can be based on response to temperature changes, particularly for polymeric nanoplatforms.Citation90 In this regard, poly(N-isopropylacrylamide) (PNIPAM) has been known as a suitable thermo-sensitive polymer whose lower critical solution temperature (LCST) is about 32°C in water.Citation91 For instance, GO-PNIPAM was reported as ibuprofen (IBU) contained nanocomposite responsive to changes of the environment temperature.Citation92 They reached a large drug loading percentage of 280 wt% which could be caused by the interaction between a carboxylic part of IBU and –NH of PNIPAM. Additionally, a long polymeric chain of PNIPAM could keep the drug in its porous structure.
Introducing polymeric NPs including PNIPAM-g-PEO on GO was performed to have a thermo-sensitive nanoplatform working as a protein inhibitor and platelet for adhesion of therapeutic agents in which DOX is tested within. Other than thermoresponsivenesss this nano-hybrid showed high lethal effect on HepG2 cells.Citation93
In another study drug loading and release profile of CPT were assessed on PNIPAM-g-GO which exhibited a temperature-dependent manner because a phase transition occurred from hydrophilic to lipophilic at 33°C. The PNIPAM homopolymer synthesized by the ATRP method was grafted to GO via click chemistry. A noticeable anti-cancer effect was observed while CPT-loaded PNIPAM-g-GO were assessed in vitro with A5RT3 cancer cell line.Citation94
A salep modified GO (SMGO) comprising of branched PNIPAM copolymerized with acrylic acid (AA) P(NIPAM-co-AA) as a thermo/pH responding to DOX nanocarriers was introduced. Release tests of DOX-loaded nanostructures also showed that maximum drug release happens in acidic pH of 5.0 and temperature at 42°C which confirmed the thermo/pH sensitivity of the nanostructures. In vitro cytotoxicity test of HeLa cells uncovered that SMGO modified P(NIPAM-co-AA) is biocompatible as well and can have a significant role in cancer cell killing followed by DOX loading.Citation95
Chemically reduced GO nanosheets were functionalized by chitosan followed by copolymerization with NIPAM and PEG-diacrylate as a crosslinker. The synthesized nanogel was loaded by DOX and released the drug in a thermo-responding manner by near infra-red (NIR) laser 808 nm. Heat production due to NIR absorption caused the water to squeeze out of nanogels which resulted in shrinkage and size reduction and stimulate the thermo-responsive nanogels to release DOX in the temperature range at 37–42°C.Citation96
In a more recent study, the quadruple-responsive nanocarrier was developed which could be stimulated by multiple factors like pH, temperature, redox, and NIR irradiation. The smart nanocarrier was loaded by rhodamine B and for various circumstances it was found that remarkable release occurred after applying multiple stimuli.Citation97 As a dual drug nanocarrier, two-faced GO nanosheets grafted by PNIPAM/PCL were prepared. The novel nanoparticles had the potential to load simultaneously hydrophobic (quercetin) and hydrophilic (5-FU) drugs and showed a thermos-responsive release profile.Citation98
Recently, it is found that Poly(N,N-diethyl acrylamide)/functionalized GQD hydrogels have a smart behavior against the temperature changes so that the release of the DOX remarkably increases near the physiological temperature. In-vitro and In-vivo results revealed that the smart nanohydrogel has great potential in the treatment of invasive tumor cells like melanoma.Citation99
Magnetically Released Drug from Graphene Nanocarriers
Magnetic nanoparticles have received great attention for drug delivery purposes.Citation100 Owing to suitable permeability of electromagnetic fields into the body, they can be used as external stimuli to release drug in DDSs. In order to accumulate and deliver a drug locally, a magnet is located near the desired site of treatment such as tumors by special tools like a conical piece of iron or typical pole shoes. Indeed, the magnetic NPs can indirectly enhance the therapeutic efficiency of GO-based DDSs by further accumulation of drug-loaded NPs in the targeted areaCitation101,Citation102 or synergistically improvement of cell killing due to magnetic hyperthermia effect.Citation103
It has been shown that iron oxide NPs-decorated PEG-GOs (IONP-GO-PEG) congregate where they are affected by an external magnetic field. From the other point of view, DOX loaded in this NPs can be released in greater quantities near tumor pH (~5.0). In addition to utilizing magnetic properties of IONPs for magnetic resonance imaging (MRI), they caused the death of more 4T1 cells located close to the magnet where IONP-GO-PEGs/DOX was more accumulated compared to regions far from the magnet.Citation104
A novel anticancer drug carrier has been presented; polyvinylpyrrolidone (PVP) and PVA stabilized iron oxide graphene whose release manner was sensitive to low pH and AC magnetic field (ACMF). Although both PVP and PVA stabilized iron oxide graphene containing DOX and paclitaxel had a lower killing effect on HeLa cells than free DOX or paclitaxel, exposure of the cells to ACMF and PVP and PVA stabilized iron oxide graphene DOX or paclitaxel simultaneously could increase the cell death up to 90 and 93%, respectively.Citation103
To combine gene therapy with anticancer drug delivery, chitosan functionalized magnetic graphene (CMG) NPs were synthesizedCitation105 and reported that these biocompatible NPs were capable of releasing DOX under pH 5.1 at a higher rate than 7.4. Besides high potential use as an MRI contrast agent, a green fluorescent protein (GFP) encoder plasmid was effectively transfected into cells in in-vitro and in-vivo in addition to DOX release.Citation106
It has been demonstrated that β-cyclodextrin modified magnetic (Fe3O4) graphene oxide (MGC) not only could be used in local drug delivery owing to superparamagnetic properties but is able to load and pH-dependently release DOX and epirubicin in MCF-7 cells. The toxicity test on the cells indicated that MGC has a lower toxic effect than MG whereas MGC/epirubicin showed a stronger lethal effect on MCF-7 cells.Citation107
In a recent work, it was proved that the decoration of GO nanosheets by magnetic iron nanoparticles followed by functionalization by chitosan/sodium alginate (CS/SA) leads to the formation of a versatile nanocarrier which can load a high amount of DOX, release it in a low pH environment and enhance cancer cell killing via photo-thermal therapy (PTT).Citation108 In a similar study, the triple responsive (pH, thermal and magnetic) GO nanohydrogel was developed and its DOX release ability was examined in the presence and absence of the magnet.Citation109
To overcome the brain-blood barrier (BBB), the functionalized GO/SPION/PLGA loaded by anticancer drug 5-iodo-2-deoxyuridine (IUdR) was exposed to an external magnet (0.5 T) and its elevated accumulation in the glioma-bearing rats was monitored by MRI. The fantastic targeted nanocarrier led to prolong median survival time of the tumor-bearing animals to 38 days.Citation110 In order to achieve an efficient chemo-thermotherapy, the Fe3O4 nanoparticles were localized into the layered rGo and finally loaded by DOX. Minimum cell survival under hyperthermia induced by a magnetic field occurred in the presence of the nanohybrid.Citation111
Graphene-Based Light Responding in DDSs
As another external stimulus, light can be used to deliver drugs in DDSs for treatment of unhealthy tissues. The DDSs based on light stimuli can benefit from user-controllable options like intensity and exposure time to deliver the drug in appropriate time and location. Over the whole electromagnetic spectrum, NIR region because of high transparency into the body as well as low damage to healthy tissues located in the beam path, was served as an excellent stimulator. In this regard, PTT using light absorber nanostructures was extensively explored for the ability of cancer cell ablation at high temperatures. Fortunately, GO nanostructures have a high tendency to absorb NIR radiation and convert it to heat.Citation112 On the other hand, photosensitizers loaded on nanocarriers have been utilized for photodynamic therapy (PDT) of cancers. Irradiation of photosensitizers by suitable wavelengths causes the production reactive oxygen species (ROSs) which induce necrotic or apoptotic cells’ death.Citation113,Citation114
PTT Using Graphene-Based Nanomaterials
Results of a study showed that peptide targeted mesoporous silica-coated graphene nano-sheet (GSPI) loaded with DOX, could release the drug in acidic pH and NIR irradiation of 3-fold higher than the non-irradiation condition. Besides targeting ability, chemo-photothermally cell killing of the nanocomposite was more effective than PTT or chemotherapy alone.Citation115 Similarly, the high death of MDA-MB-231 breast cancer cells due to treatment with dual Dox/irinotecan attached to GO NPs as well as NIR exposure has been reported.Citation116
In another study, GO and silver were utilized simultaneously for PTT and synthesized DOX loaded GO@Ag targeted with 1,2-distearoyl-sn-glycero-3-phosphoethanolamine (DSPE)-PEG2000-Asn-Gly-Arg (NGR) (GO@Ag-DOX-NGR).Citation117 In addition to imaging contrast-enhancing capability of the nanoplatforms in the animal model, they achieved highest tumor growth inhibition of 83.9% under irradiation of NIR laser. The same trend in tumor growth was observed using resveratrol (RV)-loaded PEGylated reduced GO (rGO/PEG-RV).Citation118
Recently, a novel design for rGO-based DDS has been proposed where rGO-DOX was entrapped in gold nanorods shell-coated vesicle (rGO-AuNRVe) (TEM image, ) in order to prevent contact of rGO-DOX with the bio-environment directly. As depicted in , the rGO-AuNRVe-Dox drug release mechanism into the cell was initiated upon NIR irradiation of it followed by disruption of the vesicle due to heat production and drug detachment from rGO nano-sheets in a pH-dependent manner. Under low pH and 808 nm laser irradiation, the nanoplatform showed a unique release profile so that about 70% of drug is rapidly released over 2 hours. Furthermore, U87MG cells suffered an intensive lethal effect in the presence of rGO-AuNRVe-DOX and laser irradiation simultaneously.Citation119 Surprisingly, this multifunctional nanostructure has the ability to improve photoacoustic signals in animal models.Citation119 Prodrug Pt(IV) as an analog of cisplatin and a FITC conjugated caspase-3 detector probe, were incorporated on the GO nano-sheets surface via amine groups of the PEG chains. In this work, the drug release pattern, PTT effect and potential of apoptosis identification were investigated. They expressed under high levels of GSH-like intracellular environment, Pt(IV) dihydroxy groups are removed and release Pt(II). In addition, followed by caspase-3 activation the florescent quenching of the probe will be halted. However, drug release exhibited GSH concentration dependency whilst PEG-NGO-Pt along with 785 nm NIR laser irradiation has the highest growth inhibitory effect on 4T1 cells (<5% cell viability for 100 µM). Remarkably, all PEG-NGO-Pt-treated and laser-irradiated tumors of animal models disappeared after 2 days.Citation103
Figure 5 (A) rGO-AuNRVe morphology by TEM, (B) Dox release process from rGO-AuNRVe in the cell (Reproduced with permission).
Note: Copyright ©2015. American Chemical Society. Reproduced from Song J, Yang X, Jacobson O, et al. Sequential drug release and enhanced photothermal and photoacoustic effect of hybrid reduced graphene oxide-loaded ultrasmall gold nanorod vesicles for cancer therapy. ACS nano. 2015; 9 (9): 9199–9209.Citation119
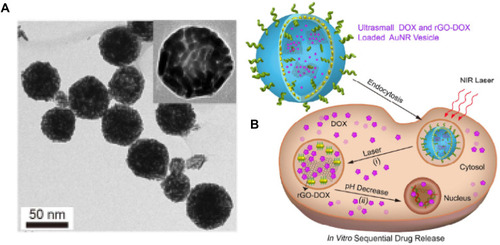
A multipurpose nanocarrier using rGO was developed to carry radionuclide 131I attaining nuclear imaging, brachytherapy and PTT concurrently. The rGO-PEG has adequate compatibility with 4T1 cells whereas 131I-rGO-PEG induced cell death in concentrations that free 131I was unable to kill them. It originates from significant accumulation of 131I in the cells by rGO-PEG nanocarrier in comparison to free 131I. Moreover, cell counting kit-8 (CCK8) assay proved 4T1 cells suffer great growth inhabitation after being exposed to 131I-rGO-PEG nano-sheet and 808 nm laser. In contrast to free 131I, the 131I-rGO-PEG was mainly taken up into the tumor rather than the other organs. In vivo tumor growth results showed that tumors were eradicated after 18 days for 4 out of 5 laser -rradiated mice and also treated by 131I-rGO-PEG.Citation120
Moreover for gene therapy, it has been demonstrated the GO functionalized with PEG and PEI can reach to a high plasmid DNA transfection as well as intracellular delivery of small interfering RNA (siRNA) utilizing 808 nm laser. They reported that heating produced from NIR exposure can elevate permeability of the cell membrane and consequently lead to further delivery of the cargo into the cells.Citation121
The synergistic therapeutic effect of a novel light (NIR)- and pH-responsive rGo functionalized by carboxymethyl chitosan and PEG has been reported. Under irradiation of NIR light and low pH, the DOX-oaded nanocarrier triggered high-performance PTT and drug release rate.Citation122 Similarly, a photothermal agent IR820 (new indocyanine green)-LA (lactobionic acid) was linked to the DOX-GO nanosheets so that in addition to pH-dependent release and photothermal capability, it exhibited fluorescent properties for imaging.Citation123
In another work, DOX release pattern from modified rGO nanosheets was improved under NIR laser irradiation and in an acidic medium. The controllable release manner under laser irradiation can enhance the therapeutic gain by selective light exposure of the target.Citation124 In order to load a remarkable amount of indocyanine green (ICG) as a PTT agent, a positive charged magnetic rGO was modified by PEI. As a result, the cancerous cells experience more heat due to irradiation of laser in the presence of the nanocomposite as well as inducing a significant tumor inhibition in the animal model after laser irradiation. Moreover, as a theranostic agent, the nanocomposite can be utilized in MR and thermal imaging modalities.Citation125
Graphene-Based Nanostructure-Mediated PDT
Considering the overexpression of some receptors such as CD44 on the surface of different tumor cells, hyaluronic acid (HA) was attached to GO nano-sheets as a targeting moiety. HA-GO-Ce6 nano-hybrid with a loading content of 115% extremely quenched Ce6 fluorescence and reduced the generation of 1O2 in comparison with free Ce6. It was concluded after Ce6 release from HA-GO-Ce6 into HeLa cells that it can boost the therapeutic gain of PDT 10 times compared to free Ce6.Citation115
Recently, a new nanoplatform was prepared with great potential in synergistic PTT, PDT, and chemotherapy. By Spinach extract (SE) as photosensitizer and contributor in hydrogel formation, rGO and gold nanocages (AuNCs) as NIR absorbers, rGO/AuNCs/SE hydrogel was fabricated to load anticancer drug 5-FU. The 5-FU loaded hydrogel declined the survival rate of HeLa cells to 1.2% under laser irradiation.Citation126
Similarly, methylene blue (MB)-linked GO coated with pluronic copolymer was fabricated to enhance bio-stability. During protonation of the GO surface and weakening interaction between MB and GO due to acidic pH, about 80% of MB was released in pH 5.0. Based on the toxicity results, in the form of combined PDT and PTT, the GO-MB nano-photosensitizer persuades HeLa cancer cells to expire more unlike NIH-3T3 normal cells.Citation127
It has been shown that a combination of core-shell upconversion NPs (UCNPs), PEGylated GO and photosensitizer medicine of phthalocyanine (ZnPc) forms a nanostructure with luminescence (UCL) emission, PTT and PDT capability. The cytotoxicity test indicated that not only the UCNP-NGO is almost nontoxic for HeLa and KB cells, but UCNP-NGO/ZnPc obviously halted the cell growth by heating and singlet oxygen production due to 808 and 630 nm laser irradiation, respectively.Citation115
GO nano-sheets conjugated with the integrin αvβ3 mAb were synthesized to transfer pyropheophorbide-a (PPa) photosensitizer into the cell mitochondria as the final target organelle. As expected, uptake test of U87-MG (positive) and MCF-7 (negative) cells proved mAb has acted as a proper targeting agent. Moreover, the confocal images clearly showed the PPa-GO-mAb was dominantly accumulated into the mitochondria over 8 h. Once the PPa-GO-mAb nanoplatforms arrived in the mitochondria successfully, a 633 nm laser can kill cancer cells noticeably.Citation128
The difference between GSH levels of intra- and extra-cellular environment motivated designing of a novel QGD nanocarrier which releases more Ce6 followed by disulfide link cleavage of GQD-SS-PEG/Ce6 by GSH. They reached drug release percentage 36% only over 10 hours in contrast to 3% over 82 hours for 10 mM and 2 µM GSH, respectively. The dual stimuli sensitive nanostructure considerably suppressed mice bearing HeLa tumor growth.Citation129
In another study, microRNA (miRNA) responding dextran-coated GO nano-colloid was attached with Ce6 conjugated peptide nucleic acid (PNA). They demonstrated that, in the presence of miR-21, the Ce6-PNA regain its fluorescent and photosensitization ability. Generally, cytotoxicity and tumor growth assay suggested the Ce6-PNA21/Dex-rGO as an appropriate agent for cellular imaging as well as PDT.Citation130
In a more recent work, a pH-sensitive link between a photosensitizer agent (Pu18) and GO caused the efficient release of the agent in a weak acidic environment. The authors have claimed that the design can trigger severe damage in the HepG-2 cells via singlet oxygen generation after light irradiation of the photosensitizer agent.Citation124 Combining the high tendency of GO to absorb the laser irradiation during PTT, high potential of protoporphyrin IX (PpIX) as a PDT agent and anticancer properties of DOX, led to the synthesis of DOX/GO (PEG-PpIX) nanocomposite. The nano-sized multimodal structure caused a reduction in tumor size of the animal model by a combination of PDT, PTT, and chemotherapy.Citation131
Recently, a convenient GQD for using as a photosensitizer was achieved by alternating the number of doped nitrogen atoms. In addition to targeting the cell nucleus the GQDs were functionalized by charge‐reversal (3‐Aminopropyl) triethoxysilane (APTES) followed by loading DOX. The fluorescent images confirmed the uptake of the nanoplatform into the nucleus where they can significantly harm it by releasing the drug and producing ROS concomitantly.Citation132
Graphene in Smart Gene Delivery System
Smart gene delivery system (SGDS) is simply transferring a foreign DNA to a cell for gene therapy or genetic research. Based on the mechanism of entry of the gene into the cytosol, SGDSs are classified as chemical (like lipids or nanoparticle carriers), biological (like viral or bacterial vectors), or mechanical (like electroporation, microinjection or biolistics).Citation133,Citation134
Chemical methods are a more common approach in which genes are delivered via such organic complexes of nucleic acid as lipid, cationic polymer, and calcium phosphate. However, biological methods are based on developing a viral gene delivery, in which the target cells are infected by a specific gene(s). Nevertheless, in the mechanical methods, naked nucleic acids are transferred directly to cells by means of electroporation, microinjection, and phototransfection.Citation135,Citation136
As far as the efficiency of a system is concerned, the satisfactory results of a SGDS directly depend on the transport of DNA to selected organs. The advent of nanotechnology is a boom time for different areas of science and industry focus in the 21st century.Citation57,Citation137 Moreover, nanotechnology has an admirable effect in realizing cost-effective and efficient diagnostic systems like SGDSs.Citation138 With this in mind, novel gene delivery systems for gene therapy can be made with several nanomaterials, including carbon nanotubes (CNTs),Citation139 nano-graphene oxide,Citation140 nanoscale polymeric materials, as well as peptide and protein-based nanoparticles.Citation141
In recent years, the advances of graphene and its various derivatives continue to accelerate as scientists produce a great number of incredible new SGDSs by taking such advantages as flexibility in design, high mechanical strength, low cytotoxicity, high surface area, easy functionalization with targeting ligand, and high intrinsic mobility.Citation142 This part highlights the recent advances of graphene and its derivatives like GOs, reduced-GOs (rGOs), and also graphene composites in GDSs and looks forward to the future of graphene-based GDSs.
One of the biggest challenges in using graphene-based nanomaterials for gene delivery is minimizing the side effects of GDS, especially their cytotoxicity. In this regard, several studies have modified the graphene derivatives by polymers such as polyethylenimine (PEI),Citation143–Citation146 lactosylated chitosan oligosaccharide (LCO), chitosan (CS),Citation147–Citation149 and polyamidoamine (PAMAM).Citation150,Citation151 For instance, the results of Zhang’s studiesCitation113 clearly showed that the use of PEI-conjugated GO to deliver DOX and siRNA does not only lead to improved therapy efficiency but also to patient safety increment. In a similar study, Feng et alCitation152 explored the effects of two molecular weights of PEI (1.2 kDa and 10 kDa) on the cytotoxicity of the PEI-GO complex. In a valuable study, Cao et alCitation153 highlighted the major role of LCO functionalized graphene oxides in removing the toxicity, and also increasing the loading capacity of FAM-DNA to human hepatic carcinoma cells (QGY-7703).
A GDS was developed to transfer plasmid DNA (pDNA). The electrostatic self-assembly method to prepare folate-conjugated trimethyl chitosan (FTMC/GO) nanoplatforms was applied. Their results showed that pDNA could be loaded into FTMC/GO, in a way that the migration of 31.1% pDNA could be retarded within 72 hours. They have also observed no cytotoxicity of FTMC/GO in both Hela cells and A549 cells. Accordingly, FTMC/GO can be noted as being a great candidate for targeted gene delivery.Citation147
Liu et alCitation150 designed an efficient graphene-based gene vector using graphene-oleate-polyamidoamine (PAMAM) dendrimer hybrids mainly because of their good dispersion and stability in water solutions. They developed this system by means of oleic acid and covalent binding of PAMAM dendrimers. Moreover, they investigated plasmid DNA transfection capacity of the hybrids and the cytotoxicity to HeLa and MG-63 cells. The results of biocompatibility showed that such hybrids are biocompatible to HeLa cells so that up to 100 μg/mL of hybrid, the cellular viability retains about 80%. However, they showed clear cytotoxicity to MG-63 cells at concentrations greater than 20 mg/mL. In optimal condition, the synthesized hybrids enjoy satisfying transfection capacity and biocompatibility.
Based on conjugation of GO and PEI, PAMAM, and polypropylenimine (PPI), three groups of vectors have been fabricated.Citation145 They have also used glycine, surface carboxyl group, and spermidine as linkers in their study. Their invaluable results showed that the synthesized vectors generally have lower cytotoxicity in comparison with the intact polymer. Moreover, they evaluated the transfection efficiency of such three groups by a plasmid encoding green fluorescent protein (GFP) using both quantitative and qualitative approaches. The best results were obtained for PEI-based vectors, especially for PEI-GO conjugates with glycine linkers in different carrier to plasmid (C/P) weight ratios. In addition, these vectors were highly efficient with the best transfection enhancement of 9 fold over that of neat PEI.
Optical Imaging and Theranostics
The American Cancer Society has predicted that one out of four deaths will be as a result of cancer. Cancer mortality mostly occurs when cancer cells metastasize via the bloodstream. Therefore, early stage detection is a key factor in cancer treatment. Accordingly, recently nanomaterials have been investigated broadly for imaging applications. The right drug for the right patient at the right moment is what nanotechnology-aided theranostics (diagnostic imaging + therapeutic) was born for, which can deliver a noninvasive as well as selective cancer therapy.Citation154 PTT/PTD and imaging for diagnosing diseases have been receiving attention due to the fact that unlike most drugs and dyes used for diagnostics they provide no toxicity as well as no side effects like anticancer drugs.
Nano-theranostic therapy has been used through various pathways namely shell-magnetic core star-shaped gold nanoparticles in which the tumor cells were recognized using whole blood sample,Citation155 Fe3O4@Au core/shell nanostars attacking at CD44 receptor-overexpressing cancer cells,Citation156 nickel ferrite and carbon targeting melanoma cancer,Citation157 or materials such as black phosphorous (BP), covalent organic framework (COF) which are known as graphene-like metal free 2D nanosheets,Citation158 and etc. Incorporating graphene in this process has been considered as a novelty of theranostic studies. This led to research on nanocomposites with Fe2O3/Au core-shell nanoparticle-graphene,Citation159 PEGylated nanoGO-platine,Citation156 nanocomplexes of GO- aptamer,Citation160 graphene nanosheets-magnetic nanoparticles,Citation161 acting as theranostic agents. Therefore, graphene-involved theranostic agents have provided a respectable dye-free recognition and treatment procedure.
The unique physical properties of graphene and its derivatives have made them potent nanomaterials to be considered and utilized in several forms of bioimaging systems including: optical imaging, radionuclide-based imaging, MRI (magnetic resonance imaging), Raman imaging and photoacoustic imaging, single-photon emission computed tomography, positron emission tomography, and multimodal imaging.Citation75,Citation162 In this part, we only investigate the use of graphene-based nanomaterials in optical imaging which comprises fluorescent imaging (FL), and two-photon fluorescent imaging (TPFL). Non-expensive availability, non-ionizing energy, imaging in real-time mode, short-range free-space optical (FSO) communication and multiplexing ability, acceptable photothermal conversion in both first near-infrared window (650–950nm) and second near-infrared window (1000–1350) in biological tissue are amongst capabilities of GO- and rGO-based nanomaterials which have resulted in their extensive exploration in the field of bioimaging and theranostics. However, several shortcomings such as low penetration in tissue, high spreading of photons in the visible region by tissue, fluorescent quenching, photobleaching, autofluorescent of background tissue (mainly light-absorbing moieties like water molecules, macro-nutrients and oxygen-containing groups) have challenged scientists using graphene in theranostic platforms for the last decade begetting future developments in this field.Citation163–Citation166 A pH-dependent fluorescence emission of GO flakes in vitro in green (550 nm) versus red (630 nm) using 480 nm laser excitation was explored. Having in mind the acidic environment of cancerous cells (about pH=6), the ratio of green to red emission intensities can be used as a pH sensor for distinguishing healthy cells against tumor cells.Citation15 A triple light/enzyme-activated chemotherapy/PDT/PTT theranostics system (HG-GNCs/GO-5FU) using hyaluronic acid-glutathione (HG), gold nanoclusters (GNCs), graphene oxide (GO) and the antitumor drug, 5-fluorouracil (5FU) was reported. In this study, it was first shown that HG-GNCs are capable of red fluorescence emission, tumor-targeting function (due to the presence of hyaluronic acid and its ability to target activated CD44 receptor on tumor cells' surfaces) as well as generation of singlet oxygen 1O2 while irradiated by laser. Then the HG-GNCs were combined with 5FU and supported with GO in order to obtain a controlled, targeted fluorescence on/off switch for imaging and synergetic tumor therapy. This was achieved because the fluorescent HG-GNCs served as energy donors and GO as energy acceptor so that the HG-GNCs fluorescence and 1O2 generation would be quenched and inhibited by GO. Interestingly, in the presence of hyaluronidase (HAdase), and under laser irradiation, the HG-GNCs and 5FU tended release from GO surface (due to the cleavage of glycosidic bindings) resulting in the restoration of HG-GNCs fluorescent and 1O2 generation which were combined with photothermal conversion of GO and cancer chemotherapy of 5FU drug.Citation167
In another study a red fluorescence, biodegradable, stimuli-responsive nano theranostic platform (GOF-Lip-FA) was presented based on graphene oxide flakes (GOF) supporting self-assembly liposomes (Lip) loaded with DOX and functionalized with FA ().Citation168
Figure 6 (A) Preparation of Dox loaded GOF-Lip-FA nano theranostic system in a schematic view, (B) Targeted cellular uptake of the GOF-Lip-FA nanohybrid and DOX release in cells body (red particles), (C) In vivo biodistribution of the platform by IV injection and cancer cells uptake, and (D) near-infrared 4T1 breast tumor cells death.
Note: Copyright ©2019. American Chemical Society. Reproduced from Prasad R, Yadav AS, Gorain M, et al. Graphene Oxide Supported Liposomes as Red Emissive Theranostics for Phototriggered Tissue Visualization and Tumor Regression. ACS Applied Bio Materials. 2019; 2 (8): 3312–3320.Citation168
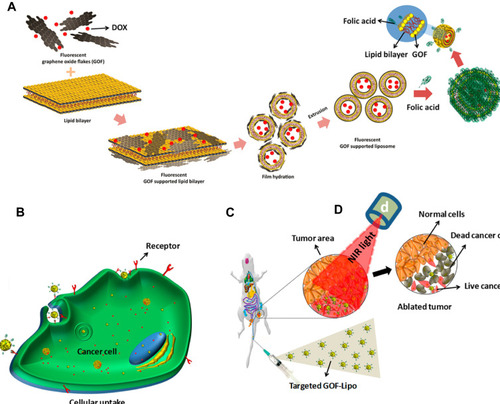
This theranostic platform was successful both in in vivo diagnosis and treatment of 4T1 breast cancer cells by dual-stimuli (pH and near-infrared light) of GOF-Lip-FA. The GOF was uniformly attached to the liposome bilayer by electrostatic and hydrophilic interactions of anionic GOF surface and cationic dipalmitoylphosphatidylcholine lipid. The combined chemotherapy and PTT of 4T1 breast tumor regression in mice under 800 nm laser irradiation and in acidic environments were successfully reported. The drug release was attributed to both protonation (due to the low pH of cancerous environment) as well as the increased temperature resulting from laser irradiation ().Citation168
Figure 7 In vivo performance of GOF-Lip-FA nano theranostic platform. (A–C) in vivo 4T1 cancer diagnosis and distribution of nano theranostic systems by near-infrared fluorescence imaging. (D–E) images of the tumor-bearing animal under laser irradiation and tumor size shrinkage shown in dotted circles. (F) Tumor size after different therapeutic paths. (G) The decrease in tumor size after 21 days. (H) near-infrared fluorescence images of tumor regression after various treatments.
Note: Copyright ©2019. American Chemical Society. Reproduced from Prasad R, Yadav AS, Gorain M, et al. Graphene Oxide Supported Liposomes as Red Emissive Theranostics for Phototriggered Tissue Visualization and Tumor Regression. ACS Applied Bio Materials. 2019; 2 (8): 3312–3320.Citation168
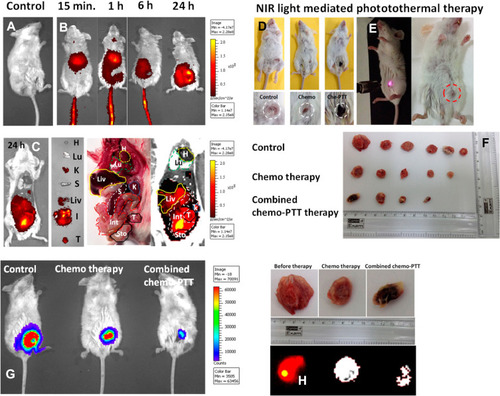
Although graphene nanomaterials can bring dye-free theranostics, it has been shown that graphene-based oxides are not intrinsically strong fluorophores, making them needful to other imaging probes or organic dyes to bind with so that they can provide acceptable contrast in bioimaging.Citation16 Recently, a GO/GQD hybrid nanomaterial was prepared as a stable theranostics agent capable of both FL and PTT. In this study, GQDs, as fluorescent agents, were electrostatically bound to GO sheets using PEI as a bridge and also, a fluorescent enhancer (preventing fluorescent quenching of GQDs by GO) leading to GO-PEI-GQDs nanomaterials. This theranostics complex showed great bioimaging and cancer therapy capabilities on MDA-MB-231 cells (breast cancer cells) as well as L292 (healthy murine fibroblast cells) effectively at low dosages and low power density (50 μg/mL and 0.5 W.cm−2 respectively) of laser (808 nm) exposure. The synergetic effects of PTT and PDT in this research resulted in treatment of cancer cells by both hyperthermia and oxidative stress.Citation169
Having an ideal photothermal sensitizer is a crucial tool in ablating destructive cells. Recently scientists have not been able to keep an eye away from graphene in this regard. The most important factor for any material to be used in optical applications (here optical imaging, photothermal treatment) is bandgap because without it emitting light is not possible. This issue is a highlight for graphene since it has no bandgap. What happens in bandgap-less graphene is that via fast electron-electron and electron-photon interactions, carriers relax making it impossible for light emission.Citation170 Nevertheless, the good news is graphene in nanosized, GO and rGO forms has a bandgap.
Bandgap opening in graphene is a long fascinating story and it has been categorized into two approaches summarized into 1) chemical engineering (chemical functionalization (hydrogenation, fluorination, and other chemical ways); and 2) physical engineering creating defects, doping).Citation171 It has to be noted that photoluminescent property of graphene-based theranostic platforms is induced by laser to produce heat for malfunction cell destruction.
Angiogenesis and Anti-Angiogenesis Ability of Graphene
In addition to the high capability of GO nanoplatforms in drug/gene delivery, some studies paid attention to angiogenesis or anti-angiogenesis properties of them. During angiogenesis new vessels are formed in the primary vessel channels to provide important nutrition and oxygen for rapid proliferative cells and to take away useless biochemical products. As stated in many studies, it plays a key role in wound healing, treatment of cardiovascular diseases as well as tumor growth.Citation172–Citation174
99As a booster of angiogenesis for the treatment of cardiovascular diseases, GO nanostructures can act via inducing the intracellular formation of reactive oxygen species (ROS) and reactive nitrogen species (RNS). It has been found that GO and rGo can trigger angiogenesis by a pathway in which ROS could affect the phosphorylation of Akt followed by upregulation of p-eNOS to elevate the intracellular release of NO. This process of angiogenesis can be inverted to anti-angiogenesis based on the concentration of GO and rGO as well as the amount of ROS in the cell.Citation175 One study has shown that GO/PCL nanoscaffold can induce angiogenesis during sciatic nerve regeneration of rats. According to their result, AKT‐eNOS‐VEGF signaling was the most probable pathway of the angiogenesis process.Citation176 Exploiting the remarkable role of vascular endothelial growth factor (VEGF) in the angiogenesis process, the VEGF-IR800-GO nanotheranostic was developed to monitor and deliver more VEGF into the ischemic tissues concurrently.Citation177 Incorporating the nanocomplex of PEI functionalized GO and VEGF gene in a hydrogel caused the effective delivery of the pro-angiogenic gene without any acute toxicity. The myocardial infarction bearing rats were intramyocardially injected by the nanohydrogel. They showed a higher myocardial capillary density and cardiac performance in comparison to other groups.Citation178 Recently, as important factors for bone regeneration the capability of GO to stimulate angiogenesis and osteogenesis has been evaluated. The authors revealed GO can induce angiogenesis of HUVACs via VEGF pathway as well as osteogenic differentiation in BMSCs via OSM and NF-κB pathways.Citation179 Besides, gelatin-methacryloyl (GelMA) hydrogel loaded by rGO exhibited an acceptable ability of wound healing in endothelial (EA.hy926), Hacat keratinocyte, and 3T3 fibroblast cells. Furthermore, the result of chicken embryo angiogenesis (CEA) assay demonstrated the high potential of the nanocomposite in angiogenesis.Citation180
Apart from the angiogenesis properties, the great capacity of GO and its derivatives in carrying and delivering anti-angiogenesis drugs has been extensively investigated by many researchers. By administration of rGO/Dox nanosheets coated by an anti-angiogenic agent (LHT7) into KB tumor-bearing mice, they significantly accumulated into the tumor cells compared to rGO/Dox nanosheets which led to diminishing tumor size after 25 days.Citation181 In another study, it has been proven that BSA-GO has a robust binding affinity to VEGF-A165 as the main receptor for triggering angiogenesis. Therefore, the binding can compete with VEGFs and leads to an anti-angiogenesis effect. The treatment of HUVECs by BSA-GO nanosheets caused a notable reduction in proliferation and tube formation of the cells. Additionally, In-vivo results showed the BSA-GO disturbs the angiogenesis process in chick chorioallantoic membrane as well as the formation of blood vessels in rabbit corneal neovascularization.Citation182 Similarly, in a more recent study, GO showed an anti-angiogenesis effect on HUVECs through the induction of oxidative stress and the manipulation of some metabolic pathways.Citation183 Focusing on the strong correlation between the level of ROS and NF-κB activation, the NF-κB related anti-angiogenesis effect of graphite nanoparticles and graphene oxide nanoplatelets (nGO) was assessed on both p53 mutant and wild type of glioblastoma cancer cells. The treatment of HUVECs co-cultured with p53 wild type of glioblastoma (U87) attenuated their strength for angiogenesis but not for p53 mutant group (U118). The predicted mechanism of anti-angiogenesis of the nanostructure had been attributed to a decrease in the level of ROS and RNS in the cells followed by downregulating of NF-κB activation which is dependent on p53 status.Citation184 Recently, a GO-based nanocarrier was synthesized to deliver a siRNA to silence the VEGF gene. GRcR/VEGF-siRNA downregulated the expression of VEGF in HeLa cells as well as in the animal model which again proves the great potential of GO nanosheets in anti-angiogenic approach.Citation185
Toxicity and Biocompatibility of Graphene and GO
Without a doubt, exhaustive investigations using cells and animal models must be conducted in a manner that everything about the biocompatibility and toxicity of graphene and GO is specified, afterward we will be able to talk about their practical clinical applications. Currently, scholars endeavor to assess the toxicity and biocompatibility of GBNs-type structures in order to tackle the problems which arise with the safety of these materials in biological applications.
From the biochemical point of view, the cytotoxicity effect of some synthesized nanomaterials could be expressed via production of ROSs through oxidative stress in treated cells.Citation186 There is speculation that oxidative stress is the base of toxicity in GNBs materials considering their analogy to materials that are derived from carbon such as CNTs.Citation187 Fibrous-type nanomaterials which include CNTs and GNBs, have been proven to cause adverse health impacts. CNTs and carbon nanofiber have physical similarity to asbestos while exposure to asbestos by inhalation could implement pulmonary diseases such as lung cancer.Citation188 Besides, examinations state that the more the dispersibility or solubility of graphene rises, the more its biocompatibility increases, GO sheets encompass plenty of hydrophilic groups such as carboxyl, hydroxyl and epoxy groups on the edges or basal planes, and their hydrophilicity is considerably raised.Citation189 Additionally, it has been asserted that the surface functionalization of both graphene and GO with hydrophilic agents, tremendously enhance their biocompatibility. It was reported that gelatin-modified graphene nanosheets were biocompatible while assessed in vitro by MCF-7 cell line, and while loaded with DOX showed high anticancer property.Citation187 Other modified types of GO including structures with folic acid conjugation and also sulfonic acid groups modification had proved to be biocompatible.Citation38 In other similar researches, PEG as a hydrophilic biopolymer has been employed for functionalizing graphene and GO in order to enhance biocompatibility, decrease non-specific binding, and improve in vivo pharmacokinetics for tumor targeting.Citation14 Latterly, exploitation of Pluronic F-127 which is another amphiphilic polymer has been reported to functionalize rGOCitation190 and GOCitation191 respectively, both for clinical utilization. The results exhibited that F-127 enhanced stability and solubility of rGO and GO engendering improved biocompatibility and drug performance. In another research, graphene modified by COOH+ ion and COOH were compared in vitro. Better cellular viability was observed for COOH+ implemented graphene.Citation192 Silanization of GO nanosheet led to a reduction in cytotoxicity of GO examined on three cell lines namely human dermal fibroblast, murine embryonic fibroblast, and human osteosarcoma. Furthermore, the SiGO showed a lower immune activation of macrophages as well as a less hepatotoxic effect than GO in the same concentration on the murine model.Citation193
With respect to the interaction between distinct forms of graphene and cell membranes, two underlying factors are highly influential. Different cell types as well as morphology of graphene, bringing various materials varying in the number of layers, lateral dimensions, shapes, and chemistry impact on the interaction. To elaborate on this point, it has been disclosed that graphene sheets with a thickness of 10 μm could enter the cells by edge-first penetration through the cell membranes and were captured by the lung epithelial cells and macrophages.Citation115
Taking all matters into account, in accordance with all of the explorations into biocompatibility, it is unmistakably inferred that there is a strong correlation between the two sides; the toxicity of graphene and GO on one side, and their surface functionalization and morphology on the other. Drawing a comparison between functionalized and non-functionalized GBNs determines that the latter are primarily far more toxic. It is crucial to carry out long-term and comprehensive toxicity assessment of the effects of GBNs on people, although such tests have been performed on rodents there is no guarantee that the outcomes can be exploited for humankind as well.Citation194 Therefore, prior applying DDSs based on graphene and GO nano-carriers for clinical purposes, numerous in vitro and in vivo toxicity researches are needed.
In terms of biodistribution, multiple pieces of researches have been carried out to evaluate distribution of graphene family materials in living organisms. In 2011, distribution of GO was investigated in mice after intravenous injection.Citation195 The results revealed that although several organs had taken up the GO (188Re–GO) during 48 hours, it predominantly accumulated in the lungs, liver, and spleen, with the minimum amount of deposition in the brain, heart, and bone. Over the next few hours, the concentration of GO decreased gradually in most organs, except for the liver and spleen. Another in-vivo research was conducted to investigate the long-term circulation of carboxylated GQDs in treated mice.Citation196 The results showed the intravenously administrated nanoparticles mainly are distributed in the liver, lung, spleen, kidney, and tumor sites. Similarly, analogous results of biodistribution were obtained by injecting nanographene sheets into mice.Citation197
All in all, when we contemplate the existing available data and information attained in consequence of research works, we become pretty hopeful about the promising entrance of these materials into the world of medical treatment, but still, surely even more endeavors must be made in this regard.
Future Trends
It is confirmed that over the past few years, the role of graphene and GO in drug/gene delivery has been promoted significantly in view of the unique and unequaled structure and properties ascribed to them. Past researches manifestly demonstrated that the awesome advancements made in this area convey the huge capability of these developing biomaterials to be used in biomedical applications. It is worth noting that the conducted preclinical studies are not sufficient at all and a great deal of preparatory study still needs to be performed. To date, graphene and its derivatives have been profoundly analyzed as a carrier having a large surface area in favor of drug-carrying and particular stress has been laid to characterize their bio-properties in proper cell lines. Notwithstanding, what occurs inside the body after loading the graphene carriers, has not been adequately noticed. The new papers considering the interactions of graphene and cells, plus in vivo effectiveness of these carriers in animals are not sufficient. They have mainly focused on the ability of GBNs to deliver their burden in the body while the attention to their removal is very scarce. In addition, in order to fully appraise their actual capacity, clearance mechanisms, long-term cytotoxicity, tissue biodistribution, and intracellular uptake trends must be studied. Apart from that, having high proficiency in and in-depth knowledge about any type of material based on graphene is essential because each form of graphene-based material possesses its own exclusive properties and surface functionalities, so it should be kept in mind that this does matter in the behavior of the material inside the body. Incidentally, in relation to gene transfection, there is the prospect that its efficiency heightens due to GBNs creation which is at the cutting edge. Unfortunately, the current trend in the studying of therapeutics is not acceptable and should alter as the total concentration is on cancer therapeutics, whereas the therapeutics such as AIDS, neurological disorders, and cardiovascular diseases are not covered in investigations. We are hopful that the present review smooths the path for further and in particular much more comprehensive research works in this field.
Although many efforts have been made to reduce the toxicity of the graphene and graphene-based materials, the use of these compounds is currently associated with high risk. Recently, these materials were put into the list of hazardous agents by The European Scientific Committee on Emerging and Newly Identified Health Risks (SCENIHR).Citation198 Unlike simple molecules, when studying a nanomaterial for biomedical research, some critical factors such as shape, size, exposure type and time, agglomeration state and concentration must be considered as a yardstick of bio-safe materials. Talking about graphene family nanomaterials, no unquestionable explanation is available for determining the safety of the materials. However, to clarify the risk-to-benefit balance of graphene-based materials, the main parameters that strongly affect the safety of the materials are necessary to summarize. To put it simply, the interaction between graphene nanomaterials and cells/tissues is the main toxicity issue. Retrospectively, when a graphene-based device is supposed to be as a scaffold in tissue engineering, long time interaction between the device and tissue would be expected. To reduce this concern, efficient synthetic and purification methods must be carefully examined. In significant studies, toxicity is attributed to the contaminants present in the sample.Citation199 Along with this consideration, rigorous cleaning processes are undeniably needed during top-down strategies for producing graphene materials. For example, during the exfoliation of graphene, amphiphilic substances are usually used that, if not properly cleaned, tissue inflammation might occur. In addition, the morphological characteristics of graphene are very effective in the toxicity of these materials. As an example, although both carbon nanotubes and GO generated using carbon nanomaterials, it has been previously indicated that GO materials have different toxicity for neurons.Citation200 As concluded, the difference recognized principally due to the altered shape of the materials. To think about graphene as a bio-grade substance, some other factors such as agglomeration and size must also be considered. Of late, it has been studied that different graphenes in lateral size have different effects on the toxicity of the lung. To be specific, smaller flakes showed less toxicity. 47 Considering the size of graphene is also important to think about the mechanism by which the material internalizes into a target cell.Citation16
Overall, regarding the presented information, it is worthwhile noting that examining a study concerned with graphene-based materials, many factors and properties including size of the material, shape, functionalization, functionalizing groups and percent of these reactions, in-vivo concentration, pharmacokinetic and pharmacodynamics of the materials must carefully be considered.
Disclosure
The authors report no conflicts of interest in this work.
References
- Liu J, Cui L, Losic D. Graphene and graphene oxide as new nanocarriers for drug delivery applications. Acta Biomater. 2013;9(12):9243–9257. doi:10.1016/j.actbio.2013.08.01623958782
- Li Z, Fan J, Tong C, et al. A smart drug-delivery nanosystem based on carboxylated graphene quantum dots for tumor-targeted chemotherapy. Nanomedicine. 2019;14(15):2011–2025. doi:10.2217/nnm-2018-037831355696
- Novoselov KS, Geim A. The rise of graphene. Nat Mater. 2007;6(3):183–191. doi:10.1038/nmat184917330084
- Pinto AM, Goncalves IC, Magalhães FD. Graphene-based materials biocompatibility: a review. Colloids Surf B Biointerfaces. 2013;111:188–202. doi:10.1016/j.colsurfb.2013.05.02223810824
- Zangabad PS, Mirkiani S, Shahsavari S, et al. Stimulus-responsive liposomes as smart nanoplatforms for drug delivery applications. Nanotechnol Rev. 2018;7(1):95–122.29404233
- Farjadian F, Ghasemi A, Gohari O, Roointan A, Karimi M, Hamblin MR. Nanopharmaceuticals and nanomedicines currently on the market: challenges and opportunities. Nanomedicine. 2019;14(1):93–126. doi:10.2217/nnm-2018-012030451076
- Farjadian F, Roointan A, Mohammadi-Samani S, Hosseini M. Mesoporous silica nanoparticles: synthesis, pharmaceutical applications, biodistribution, and biosafety assessment. Chem Eng J. 2019;359:684–705. doi:10.1016/j.cej.2018.11.156
- Entezar-Almahdi E, Mohammadi-Samani S, Tayebi L, Farjadian F. Recent advances in designing 5-fluorouracil delivery systems: a stepping stone in the safe treatment of colorectal cancer. Int J Nanomedicine. 2020;15:5445. doi:10.2147/IJN.S25770032801699
- Karimi M, Ghasemi A, Mirkiani S, Basri SMM, Hamblin MR. Carbon Nanotubes in Drug and Gene Delivery. San Rafael, CA: Morgan & Claypool Publishers; 2017.
- Fatemeh Farjadian SA, Sadatlu MAA, Mirkiani S, et al. Recent developments in graphene and graphene oxide: properties, synthesis, and modifications: a review chemistryselect. ChemistrySelect. 2020;5(33):10200–10219. doi:10.1002/slct.202002501
- А F, Prischepa S, Fedotova J, et al. Electrical conductivity and magnetoresistance in twisted graphene electrochemically decorated with Co particles. Physica E Low Dimens Syst Nanostruct. 2020;117:113790. doi:10.1016/j.physe.2019.113790
- Jiang J-H, Pi J, Jin H, Cai J-Y. Functional graphene oxide as cancer-targeted drug delivery system to selectively induce oesophageal cancer cell apoptosis. Arti Cells Nanomed Biotechnol. 2018;46(sup3):S297–S307. doi:10.1080/21691401.2018.1492418
- Muñoz R, Singh DP, Kumar R, Matsuda A. Graphene oxide for drug delivery and cancer therapy In: Swain SK, Jawaid M, editors. Nanostructured Polymer Composites for Biomedical Applications.. Elsevier; 2019:447–488.
- Liu Z, Robinson JT, Sun X, Dai H. PEGylated nanographene oxide for delivery of water-insoluble cancer drugs. J Am Chem Soc. 2008;130(33):10876–10877. doi:10.1021/ja803688x18661992
- Campbell E, Hasan MT, Pho C, Callaghan K, Akkaraju G, Naumov A. Graphene oxide as a multifunctional platform for intracellular delivery, imaging, and cancer sensing. Sci Rep. 2019;9(1):1–9. doi:10.1038/s41598-018-36617-430626917
- McCallion C, Burthem J, Rees-Unwin K, Golovanov A, Pluen A. Graphene in therapeutics delivery: problems, solutions and future opportunities. Eur J Pharm Biopharm. 2016;104:235–250. doi:10.1016/j.ejpb.2016.04.01527113141
- Singh N, Kushwaha P, Gupta A, Prakash O. Recent advances of novel therapeutic agents from botanicals for prevention and therapy of breast cancer: an updated review. Curr Cancer Ther Rev. 2020;16(1):5–18. doi:10.2174/1573394715666181129101502
- Choi J-S, Joo SH. Recent trends in cyclic peptides as therapeutic agents and biochemical tools. Biomol Ther (Seoul). 2020;28(1):18. doi:10.4062/biomolther.2019.08231597413
- Kovalchuk O, Kovalchuk I. Cannabinoids as anticancer therapeutic agents. Cell Cycle. 2020;19(9):961–989. doi:10.1080/15384101.2020.174295232249682
- Hosseini M, Farjadian F, Makhlouf ASH. Smart stimuli-responsive nano-sized hosts for drug delivery In: Hosseini, Makhlouf M, Hamdy AS, editors. Industrial Applications for Intelligent Polymers and Coatings.. Springer; 2016:1–26.
- Abdelhamid HN, Khan MS, Wu H-F. Graphene oxide as a nanocarrier for gramicidin (GOGD) for high antibacterial performance. RSC Adv. 2014;4(91):50035–50046. doi:10.1039/C4RA07250B
- Sun X, Liu Z, Welsher K, et al. Nano-graphene oxide for cellular imaging and drug delivery. Nano Res. 2008;1(3):203–212. doi:10.1007/s12274-008-8021-820216934
- Goenka S, Sant V, Sant S. Graphene-based nanomaterials for drug delivery and tissue engineering. J Controlled Release. 2014;173:75–88. doi:10.1016/j.jconrel.2013.10.017
- Lu CH, Yang HH, Zhu CL, Chen X, Chen GN. A graphene platform for sensing biomolecules. Angewandte Chemie. 2009;121(26):4879–4881. doi:10.1002/ange.200901479
- Ahmadi S, Rabiee N, Bagherzadeh M, et al. Stimulus-responsive sequential release systems for drug and gene delivery. Nano Today. 2020;34:100914. doi:10.1016/j.nantod.2020.10091432788923
- Robinson JT, Tabakman SM, Liang Y, et al. Ultrasmall reduced graphene oxide with high near-infrared absorbance for photothermal therapy. J Am Chem Soc. 2011;133(17):6825–6831. doi:10.1021/ja201017521476500
- Yang K, Wan J, Zhang S, Tian B, Zhang Y, Liu Z. The influence of surface chemistry and size of nanoscale graphene oxide on photothermal therapy of cancer using ultra-low laser power. Biomaterials. 2012;33(7):2206–2214. doi:10.1016/j.biomaterials.2011.11.06422169821
- Wang A, Long L, Zhao W, et al. Increased optical nonlinearities of graphene nanohybrids covalently functionalized by axially-coordinated porphyrins. Carbon. 2013;53:327–338. doi:10.1016/j.carbon.2012.11.019
- Chen Y-W, Chen P-J, Hu S-H, Chen IW, S-Y C. NIR-triggered synergic photo-chemothermal therapy delivered by reduced graphene oxide/carbon/mesoporous silica nanocookies. Adv Funct Mater. 2014;24(4):451–459.
- Tang Y, Hu H, Zhang MG, et al. An aptamer-targeting photoresponsive drug delivery system using “off-on” graphene oxide wrapped mesoporous silica nanoparticles. Nanoscale. 2015;7(14):6304–6310. doi:10.1039/C4NR07493A25782595
- Yang K, Feng L, Liu Z. Stimuli responsive drug delivery systems based on nano-graphene for cancer therapy. Adv Drug Deliv Rev. 2016;105(Part):B:228–241. doi:10.1016/j.addr.2016.05.015
- Yang Y, Zhang YM, Chen Y, Zhao D, Chen JT, Liu Y. Construction of a graphene oxide based noncovalent multiple nanosupramolecular assembly as a scaffold for drug delivery. Chem Eur J. 2012;18(14):4208–4215. doi:10.1002/chem.20110344522374621
- Wu J, Y-S W, X-Y Y, et al. Graphene oxide used as a carrier for adriamycin can reverse drug resistance in breast cancer cells. Nanotechnology. 2012;23(35):355101. doi:10.1088/0957-4484/23/35/35510122875697
- Fan X, Jiao G, Gao L, Jin P, Li X. The preparation and drug delivery of a graphene–carbon nanotube–Fe 3 O 4 nanoparticle hybrid. J Mater Chem B. 2013;1(20):2658–2664. doi:10.1039/c3tb00493g32260953
- Zhang L, Lu Z, Zhao Q, Huang J, Shen H, Zhang Z. Enhanced chemotherapy efficacy by sequential delivery of siRNA and anticancer drugs using PEI‐grafted graphene oxide. Small. 2011;7(4):460–464. doi:10.1002/smll.20100152221360803
- Shen H, Liu M, He H, et al. PEGylated graphene oxide-mediated protein delivery for cell function regulation. ACS Appl Mater Interfaces. 2012;4(11):6317–6323. doi:10.1021/am301936723106794
- La WG, Park S, Yoon HH, et al. Delivery of a therapeutic protein for bone regeneration from a substrate coated with graphene oxide. Small. 2013;9(23):4051–4060. doi:10.1002/smll.20130057123839958
- Zhang L, Xia J, Zhao Q, Liu L, Zhang Z. Functional graphene oxide as a nanocarrier for controlled loading and targeted delivery of mixed anticancer drugs. Small. 2010;6(4):537–544. doi:10.1002/smll.20090168020033930
- Öztürk-Atar K, Eroğlu H, Çalış S. Novel advances in targeted drug delivery. J Drug Target. 2018;26(8):633–642. doi:10.1080/1061186X.2017.140107629096554
- Zhang M, Wu F, Wang W, Shen J, Zhou N, Wu C. Multifunctional nanocomposites for targeted, photothermal, and chemotherapy. Chemi Mater. 2019;31(6):1847–1859. doi:10.1021/acs.chemmater.8b00934
- De S, Patra K, Ghosh D, et al. Tailoring the efficacy of multifunctional biopolymeric graphene oxide quantum dot-based nanomaterial as nanocargo in cancer therapeutic application. ACS Biomater Sci Eng. 2018;4(2):514–531.33418741
- Li D, Deng M, Yu Z, et al. Biocompatible and stable GO-coated Fe3O4 nanocomposite: a robust drug delivery carrier for simultaneous tumor MR imaging and targeted therapy. ACS Biomater Sci Eng. 2018;4(6):2143–2154. doi:10.1021/acsbiomaterials.8b0002933435038
- Chowdhury AD, Ganganboina AB, Y-C T, H-C C, R-A D. Multifunctional GQDs-Concanavalin A@ Fe3O4 nanocomposites for cancer cells detection and targeted drug delivery. Anal Chim Acta. 2018;1027:109–120. doi:10.1016/j.aca.2018.04.02929866260
- Hussien NA, Işıklan N, Türk M. Aptamer-functionalized magnetic graphene oxide nanocarrier for targeted drug delivery of paclitaxel. Mater Chem Phys. 2018;211:479–488. doi:10.1016/j.matchemphys.2018.03.015
- Gong P, Ji S, Wang J, et al. Fluorescence-switchable ultrasmall fluorinated graphene oxide with high near-infrared absorption for controlled and targeted drug delivery. Chem Eng J. 2018;348:438–446. doi:10.1016/j.cej.2018.04.193
- Ratajczak K, Stobiecka M. Ternary interactions and energy transfer between fluorescein isothiocyanate, adenosine triphosphate, and graphene oxide nanocarriers. J Phys Chem B. 2017;121(28):6822–6830. doi:10.1021/acs.jpcb.7b0429528650635
- Itatahine A, Mehdi YA, Fizir M, Qi M, Dramou P, He H. Multifunctional carbon nanomateriels for camptothecine low-water soluble anticancer drug delivery. N J Chem. 2018;42(2):1326–1336. doi:10.1039/C7NJ04609J
- Deb A, Andrews NG, Raghavan V. Natural polymer functionalized graphene oxide for co-delivery of anticancer drugs: in-vitro and in-vivo. Int J Biol Macromol. 2018;113:515–525. doi:10.1016/j.ijbiomac.2018.02.15329496437
- Zare-Zardini H, Taheri-Kafrani A, Amiri A, Bordbar AK. New generation of drug delivery systems based on ginsenoside Rh2-, Lysine- and Arginine-treated highly porous graphene for improving anticancer activity. Sci Rep. 2018;8(1):1–15. doi:10.1038/s41598-017-18938-y29311619
- Javanbakht S, Namazi H. Doxorubicin loaded carboxymethyl cellulose/graphene quantum dot nanocomposite hydrogel films as a potential anticancer drug delivery system. Mater Sci Eng C. 2018;87:50–59. doi:10.1016/j.msec.2018.02.010
- Li Y, Gao X, Yu Z, et al. Reversing multidrug resistance by multiplexed gene silencing for enhanced breast cancer chemotherapy. ACS Appl Mater Interfaces. 2018;10(18):15461–15466. doi:10.1021/acsami.8b0280029663807
- Xie M, Zhang F, Liu L, et al. Surface modification of graphene oxide nanosheets by protamine sulfate/sodium alginate for anti-cancer drug delivery application. Appl Surf Sci. 2018;440:853–860. doi:10.1016/j.apsusc.2018.01.175
- Puvirajesinghe TM, Zhi Z, Craster R, Guenneau S. Tailoring drug release rates in hydrogel-based therapeutic delivery applications using graphene oxide. J R Soc Interface. 2018;15(139):20170949. doi:10.1098/rsif.2017.094929445040
- Rao Z, Ge H, Liu L, et al. Carboxymethyl cellulose modified graphene oxide as pH-sensitive drug delivery system. Int J Biol Macromol. 2018;107:1184–1192. doi:10.1016/j.ijbiomac.2017.09.09628951302
- Deb A, Vimala R. Camptothecin loaded graphene oxide nanoparticle functionalized with polyethylene glycol and folic acid for anticancer drug delivery. J Drug Deliv Sci Technol. 2018;43(October2017):333–342. doi:10.1016/j.jddst.2017.10.025
- de Sousa M, Visani de Luna LA, Fonseca LC, Giorgio S, Alves OL. Folic-acid-functionalized graphene oxide nanocarrier: synthetic approaches, characterization, drug delivery study, and antitumor screening. ACS Appl Nano Mater. 2018;1(2):922–932. doi:10.1021/acsanm.7b00324
- Karimi M, Eslami M, Sahandi‐Zangabad P, et al. pH‐Sensitive stimulus‐responsive nanocarriers for targeted delivery of therapeutic agents. Wiley Interdiscip Rev Nanomed Nanobiotechnol. 2016;8(5):696–716.26762467
- Roointan A, Farzanfar J, Mohammadi-Samani S, Behzad-Behbahani A, Farjadian F. Smart pH responsive drug delivery system based on poly (HEMA-co-DMAEMA) nanohydrogel. Int J Pharm. 2018;552(1–2):301–311. doi:10.1016/j.ijpharm.2018.10.00130291961
- Mozafari N, Farjadian F, Samani SM, Azadi S, Azadi A. Simvastatin-chitosan-citicoline conjugates nanoparticles as the co-delivery system in Alzheimer susceptible patients. Int J Biol Macromol. 2020;156:1396–1407. doi:10.1016/j.ijbiomac.2019.11.18031760027
- Kavitha T, Abdi SIH, Park S-Y. pH-sensitive nanocargo based on smart polymer functionalized graphene oxide for site-specific drug delivery. Phys Chem Chem Phys. 2013;15(14):5176–5185. doi:10.1039/c3cp00008g23454895
- Wang C, Chen B, Zou M, Cheng G. Cyclic RGD-modified chitosan/graphene oxide polymers for drug delivery and cellular imaging. Colloids Surf B Biointerfaces. 2014;122:332–340. doi:10.1016/j.colsurfb.2014.07.01825064484
- Feng L, Li K, Shi X, Gao M, Liu J, Liu Z. Smart pH‐responsive nanocarriers based on nano‐graphene oxide for combined chemo‐and photothermal therapy overcoming drug resistance. Adv Healthcare Mater. 2014;3(8):1261–1271. doi:10.1002/adhm.201300549
- Jiang T, Sun W, Zhu Q, et al. Furin‐mediated sequential delivery of anticancer cytokine and small‐molecule drug shuttled by graphene. Adv Mater. 2015;27(6):1021–1028. doi:10.1002/adma.20140449825504623
- Lv O, Tao Y, Qin Y, et al. Highly fluorescent and morphology-controllable graphene quantum dots-chitosan hybrid xerogels for in vivo imaging and pH-sensitive drug carrier. Mater Sci Eng C. 2016;67:478–485. doi:10.1016/j.msec.2016.05.031
- Qiu J, Zhang R, Li J, et al. Fluorescent graphene quantum dots as traceable, pH-sensitive drug delivery systems. Int J Nanomedicine. 2015;10:6709.26604747
- Huang Y-S, Lu Y-J, Chen J-P. Magnetic graphene oxide as a carrier for targeted delivery of chemotherapy drugs in cancer therapy. J Magn Magn Mater. 2016.
- Tyagi N, Attia NF, Geckeler KE. Exfoliated graphene nanosheets: pH-sensitive drug carrier and anti-cancer activity. J Colloid Interface Sci. 2017;498:364–377. doi:10.1016/j.jcis.2017.03.05728343134
- Javanbakht S, Shaabani A. Encapsulation of graphene quantum dot-crosslinked chitosan by carboxymethylcellulose hydrogel beads as a pH-responsive bio-nanocomposite for the oral delivery agent. Int J Biol Macromol. 2019;123:389–397. doi:10.1016/j.ijbiomac.2018.11.11830445077
- Dong J, Wang K, Sun L, et al. Application of graphene quantum dots for simultaneous fluorescence imaging and tumor-targeted drug delivery. Sens Actuators B Chem. 2018;256:616–623. doi:10.1016/j.snb.2017.09.200
- Sumathra M, Sadasivuni KK, Kumar SS, Rajan M. Cisplatin-Loaded graphene oxide/chitosan/hydroxyapatite composite as a promising tool for osteosarcoma-affected bone regeneration. ACS Omega. 2018;3(11):14620–14633. doi:10.1021/acsomega.8b0209030555982
- Vinothini K, Rajendran NK, Rajan M, Ramu A, Marraiki N, Elgorban AM. A magnetic nanoparticle functionalized reduced graphene oxide-based drug carrier system for a chemo-photodynamic cancer therapy. N J Chem. 2020;44(14):5265–5277. doi:10.1039/D0NJ00049C
- Vinothini K, Rajendran NK, Ramu A, Elumalai N, Rajan M. Folate receptor targeted delivery of paclitaxel to breast cancer cells via folic acid conjugated graphene oxide grafted methyl acrylate nanocarrier. Biomedicine Pharmacother. 2019;110:906–917. doi:10.1016/j.biopha.2018.12.008
- Vinothini K, Rajendran NK, Munusamy MA, Alarfaj AA, Rajan M. Development of biotin molecule targeted cancer cell drug delivery of doxorubicin loaded κ-carrageenan grafted graphene oxide nanocarrier. Mater Sci Eng C. 2019;100:676–687. doi:10.1016/j.msec.2019.03.011
- Prabakaran S, Jeyaraj M, Nagaraj A, Sadasivuni KK, Rajan M. Polymethyl methacrylate–ovalbumin@ graphene oxide drug carrier system for high anti-proliferative cancer drug delivery. Applied Nanosci. 2019;9(7):1487–1500. doi:10.1007/s13204-019-00950-5
- Luo Y, Tang Y, Liu T, et al. Engineering graphene oxide with ultrasmall SPIONs and smart drug release for cancer theranostics. Chem Commun. 2019;55(13):1963–1966. doi:10.1039/C8CC09185D
- Kazempour M, Namazi H, Akbarzadeh A, Kabiri R. Synthesis and characterization of PEG-functionalized graphene oxide as an effective pH-sensitive drug carrier. Artif Cells Nanomed Biotechnol. 2019;47(1):90–94. doi:10.1080/21691401.2018.154319630663418
- Kundu A, Nandi S, Das P, Nandi AK. Fluorescent graphene oxide via polymer grafting: an efficient nanocarrier for both hydrophilic and hydrophobic drugs. ACS Appl Mater Interfaces. 2015;7(6):3512–3523. doi:10.1021/am507110r25612470
- Onuki Y, Bhardwaj U, Papadimitrakopoulos F, Burgess DJ. A Review of the Biocompatibility of Implantable Devices: Current Challenges to Overcome Foreign Body Response. SAGE Publications; 2008.
- Romberg B, Hennink WE, Storm G. Sheddable coatings for long-circulating nanoparticles. Pharm Res. 2008;25(1):55–71. doi:10.1007/s11095-007-9348-717551809
- Meng F, Hennink WE, Zhong Z. Reduction-sensitive polymers and bioconjugates for biomedical applications. Biomaterials. 2009;30(12):2180–2198. doi:10.1016/j.biomaterials.2009.01.02619200596
- Wen H, Dong C, Dong H, et al. Engineered redox‐responsive PEG detachment mechanism in PEGylated nano‐graphene oxide for intracellular drug delivery. Small. 2012;8(5):760–769. doi:10.1002/smll.20110161322228696
- Chen H, Wang Z, Zong S, et al. SERS-fluorescence monitored drug release of a redox-responsive nanocarrier based on graphene oxide in tumor cells. ACS Appl Mater Interfaces. 2014;6(20):17526–17533. doi:10.1021/am505160v25272041
- Zhao X, Yang L, Li X, et al. Functionalized graphene oxide nanoparticles for cancer cell-specific delivery of antitumor drug. Bioconjug Chem. 2015;26(1):128–136. doi:10.1021/bc500513725525819
- Al-Nahain A, Lee SY, In I, Lee KD, Park SY. Triggered pH/redox responsive release of doxorubicin from prepared highly stable graphene with thiol grafted pluronic. Int J Pharm. 2013;450(1):208–217. doi:10.1016/j.ijpharm.2013.04.05323624082
- Cho Y, Choi Y. Graphene oxide–photosensitizer conjugate as a redox-responsive theranostic agent. Chem Commun. 2012;48(79):9912–9914. doi:10.1039/c2cc35197h
- Yin T, Liu J, Zhao Z, et al. Redox sensitive hyaluronic acid-decorated graphene oxide for photothermally controlled tumor-cytoplasm-selective rapid drug delivery. Adv Funct Mater. 2017;27(14):1604620. doi:10.1002/adfm.201604620
- Kim DJ, Kim J, Lee HL, et al. Redox-responsive nanocomposites composed of graphene oxide and chlorin e6 for photodynamic treatment of cholangiocarcinoma. Bull Korean Chem Soc. 2018;39(9):1073–1082. doi:10.1002/bkcs.11552
- Liu J, Zhang D, Lian S, et al. Redox-responsive hyaluronic acid-functionalized graphene oxide nanosheets for targeted delivery of water-insoluble cancer drugs. Int J Nanomedicine. 2018;13:7457. doi:10.2147/IJN.S17388930532533
- Ma N, Song A, Li Z, Luan Y. Redox-sensitive prodrug molecules meet graphene oxide: an efficient graphene oxide-based nanovehicle toward cancer therapy. ACS Biomater Sci Eng. 2019;5(3):1384–1391. doi:10.1021/acsbiomaterials.9b0011433405614
- Farjadian F, Rezaeifard S, Naeimi M, et al. Temperature and pH-responsive nano-hydrogel drug delivery system based on lysine-modified poly (vinylcaprolactam). Int J Nanomedicine. 2019;14:6901. doi:10.2147/IJN.S21446731564860
- Farjadian F, Ghasemi S, Andami Z, Tamami B. Thermo-responsive nanocarrier based on poly (N-isopropylacrylamide) serving as a smart doxorubicin delivery system. Iranian Polymer J. 2020;29(3):197–207. doi:10.1007/s13726-020-00785-w
- Zhu S, Li J, Chen Y, et al. Grafting of graphene oxide with stimuli-responsive polymers by using ATRP for drug release. J Nanoparticle Res. 2012;14(9):1–11. doi:10.1007/s11051-012-1132-x
- Wang H, Sun D, Zhao N, et al. Thermo-sensitive graphene oxide–polymer nanoparticle hybrids: synthesis, characterization, biocompatibility and drug delivery. J Mater Chem B. 2014;2(10):1362–1370.32261451
- Pan Y, Bao H, Sahoo NG, Wu T, Water‐Soluble Poly LL. (N‐isopropylacrylamide)–graphene sheets synthesized via click chemistry for drug delivery. Adv Funct Mater. 2011;21(14):2754–2763. doi:10.1002/adfm.201100078
- Bardajee GR, Hooshyar Z, Farsi M, Mobini A, Sang G. Synthesis of a novel thermo/pH sensitive nanogel based on salep modified graphene oxide for drug release. Mater Sci Eng C. 2016.
- Wang C, Mallela J, Garapati USet al., A chitosan-modified graphene nanogel for noninvasive controlled drug release. Nanomedicine. 2013;9(7):903–911. doi:10.1016/j.nano.2013.01.00323352802
- Wang P, Chen S, Cao Z, Wang G, Light- NIR. Temperature-, pH-, and redox-responsive polymer-modified reduced graphene oxide/mesoporous silica sandwich-like nanocomposites for controlled release. ACS Appl Mater Interfaces. 2017;9(34):29055–29062. doi:10.1021/acsami.7b0746828795557
- Khoee S, Karimi MR. Dual-drug loaded Janus graphene oxide-based thermoresponsive nanoparticles for targeted therapy. Polymer. 2018;142:80–98. doi:10.1016/j.polymer.2018.03.022
- Havanur S, Batish I, Cheruku SP, Gourishetti K, Kumar PEJ. N. Poly(N,N-diethyl acrylamide)/functionalized graphene quantum dots hydrogels loaded with doxorubicin as a nano-drug carrier for metastatic lung cancer in mice. Mater Sci Eng C. 2019;105:110094. doi:10.1016/j.msec.2019.110094
- Farjadian F, Ghasemi S, Mohammadi-Samani S. Hydroxyl-modified magnetite nanoparticles as novel carrier for delivery of methotrexate. Int J Pharm. 2016;504(1–2):110–116. doi:10.1016/j.ijpharm.2016.03.02226994523
- Yang X, Wang Y, Huang X, et al. Multi-functionalized graphene oxide based anticancer drug-carrier with dual-targeting function and pH-sensitivity. J Mater Chem. 2011;21(10):3448–3454. doi:10.1039/C0JM02494E
- Wang Z, Zhou C, Xia J, et al. Fabrication and characterization of a triple functionalization of graphene oxide with Fe 3 O 4, folic acid and doxorubicin as dual-targeted drug nanocarrier. Colloids Surf B Biointerfaces. 2013;106:60–65. doi:10.1016/j.colsurfb.2013.01.03223434692
- Swain AK, Pradhan L, Bahadur D. Polymer stabilized Fe3O4-graphene as an amphiphilic drug carrier for thermo-chemotherapy of cancer. ACS Appl Mater Interfaces. 2015;7(15):8013–8022. doi:10.1021/acsami.5b0253625821899
- Ma X, Tao H, Yang K, et al. A functionalized graphene oxide-iron oxide nanocomposite for magnetically targeted drug delivery, photothermal therapy, and magnetic resonance imaging. Nano Res. 2012;5(3):199–212. doi:10.1007/s12274-012-0200-y
- Wang C, Ravi S, Garapati USet al., Multifunctional chitosan magnetic-graphene (CMG) nanoparticles: a theranostic platform for tumor-targeted co-delivery of drugs, genes and MRI contrast agents. J Mater Chem B. 2013;1(35):4396–4405. doi:10.1039/c3tb20452a24883188
- Guo L, Shi H, Wu H, et al. Prostate cancer targeted multifunctionalized graphene oxide for magnetic resonance imaging and drug delivery. Carbon. 2016;107:87–99. doi:10.1016/j.carbon.2016.05.054
- Wang C, Li B, Niu W, et al. β-Cyclodextrin modified graphene oxide–magnetic nanocomposite for targeted delivery and pH-sensitive release of stereoisomeric anti-cancer drugs. RSC Adv. 2015;5(108):89299–89308. doi:10.1039/C5RA13082D
- Xie M, Zhang F, Peng H, et al. Layer-by-layer modification of magnetic graphene oxide by chitosan and sodium alginate with enhanced dispersibility for targeted drug delivery and photothermal therapy. Colloids Surf B Biointerfaces. 2019;176:462–470. doi:10.1016/j.colsurfb.2019.01.02830682619
- Bardajee GR, Hooshyar Z. Thermo/pH/magnetic-triple sensitive poly(N-isopropylacrylamide-co-2-dimethylaminoethyl) methacrylate)/sodium alginate modified magnetic graphene oxide nanogel for anticancer drug delivery. Polymer Bull. 2018;75(12):5403–5419. doi:10.1007/s00289-018-2329-7
- Shirvalilou S, Khoei S, Khoee S, Raoufi NJ, Karimi MR, Shakeri-Zadeh A. Development of a magnetic nano-graphene oxide carrier for improved glioma-targeted drug delivery and imaging: in vitro and in vivo evaluations. Chem Biol Interact. 2018;295:97–108. doi:10.1016/j.cbi.2018.08.02730170108
- Gupta J, Prakash A, Jaiswal MK, Agarrwal A, Bahadur D. Superparamagnetic iron oxide-reduced graphene oxide nanohybrid-a vehicle for targeted drug delivery and hyperthermia treatment of cancer. J Magn Magn Mater. 2018;448:332–338. doi:10.1016/j.jmmm.2017.05.084
- Bao Z, Liu X, Liu Y, Liu H, Zhao K. Near-infrared light-responsive inorganic nanomaterials for photothermal therapy. Asian J Pharm Sci. 2016;11(3):349–364. doi:10.1016/j.ajps.2015.11.123
- Tian B, Wang C, Zhang S, Feng L, Liu Z. Photothermally enhanced photodynamic therapy delivered by nano-graphene oxide. ACS Nano. 2011;5(9):7000–7009. doi:10.1021/nn201560b21815655
- Zhou L, Jiang H, Wei S, Ge X, Zhou J, Shen J. High-efficiency loading of hypocrellin B on graphene oxide for photodynamic therapy. Carbon. 2012;50(15):5594–5604. doi:10.1016/j.carbon.2012.08.013
- Wang Y, Wang H, Liu D, Song S, Wang X, Zhang H. Graphene oxide covalently grafted upconversion nanoparticles for combined NIR mediated imaging and photothermal/photodynamic cancer therapy. Biomaterials. 2013;34(31):7715–7724. doi:10.1016/j.biomaterials.2013.06.04523859660
- Tran TH, Nguyen HT, Pham TT, et al. Development of a graphene oxide nanocarrier for dual-drug chemo-phototherapy to overcome drug resistance in cancer. ACS Appl Mater Interfaces. 2015;7(51):28647–28655. doi:10.1021/acsami.5b1042626641922
- Shi J, Wang L, Zhang J, et al. A tumor-targeting near-infrared laser-triggered drug delivery system based on GO@ Ag nanoparticles for chemo-photothermal therapy and X-ray imaging. Biomaterials. 2014;35(22):5847–5861. doi:10.1016/j.biomaterials.2014.03.04224746963
- Chen J, Liu H, Zhao C, et al. One-step reduction and PEGylation of graphene oxide for photothermally controlled drug delivery. Biomaterials. 2014;35(18):4986–4995. doi:10.1016/j.biomaterials.2014.02.03224656608
- Song J, Yang X, Jacobson O, et al. Sequential drug release and enhanced photothermal and photoacoustic effect of hybrid reduced graphene oxide-loaded ultrasmall gold nanorod vesicles for cancer therapy. ACS Nano. 2015;9(9):9199–9209. doi:10.1021/acsnano.5b0380426308265
- Chen L, Zhong X, Yi X, et al. Radionuclide 131 I labeled reduced graphene oxide for nuclear imaging guided combined radio-and photothermal therapy of cancer. Biomaterials. 2015;66:21–28. doi:10.1016/j.biomaterials.2015.06.04326188609
- Feng L, Yang X, Shi X, et al. Polyethylene glycol and polyethylenimine dual‐functionalized nano‐graphene oxide for photothermally enhanced gene delivery. Small. 2013;9(11):1989–1997. doi:10.1002/smll.20120253823292791
- Liu W, Zhang X, Zhou L, Shang L, Su Z. Reduced graphene oxide (rGO) hybridized hydrogel as a near-infrared (NIR)/pH dual-responsive platform for combined chemo-photothermal therapy. J Colloid Interface Sci. 2019;536:160–170. doi:10.1016/j.jcis.2018.10.05030366181
- Huang C, Hu X, Hou Z, Ji J, Li Z, Luan Y. Tailored graphene oxide-doxorubicin nanovehicles via near-infrared dye-lactobionic acid conjugates for chemo-photothermal therapy. J Colloid Interface Sci. 2019;545:172–183. doi:10.1016/j.jcis.2019.03.01930878783
- Zhang Y, Zhang H, Wang Z, Jin Y. pH-Sensitive graphene oxide conjugate purpurin-18 methyl ester photosensitizer nanocomplex in photodynamic therapy. N J Chem. 2018;42(16):13272–13284. doi:10.1039/C8NJ00439K
- Du B, Liu J, Ding Get al., Positively charged graphene/Fe3O4/polyethylenimine with enhanced drug loading and cellular uptake for magnetic resonance imaging and magnet-responsive cancer therapy. Nano Res. 2017;10(7):2280–2295. doi:10.1007/s12274-016-1418-x
- Chang G, Li S, Huang F, Zhang X, Shen Y, Xie A. Multifunctional reduced graphene oxide hydrogel as drug carrier for localized and synergic photothermal/photodynamics/chemo therapy. J Mater Sci Technol. 2016;32(8):753–762. doi:10.1016/j.jmst.2016.06.014
- Sahu A, Choi WI, Lee JH, Tae G. Graphene oxide mediated delivery of methylene blue for combined photodynamic and photothermal therapy. Biomaterials. 2013;34(26):6239–6248. doi:10.1016/j.biomaterials.2013.04.06623706688
- Wei Y, Zhou F, Zhang D, Chen Q, Xing D. A graphene oxide based smart drug delivery system for tumor mitochondria-targeting photodynamic therapy. Nanoscale. 2016;8(6):3530–3538. doi:10.1039/C5NR07785K26799192
- Li Y, Wu Z, Du D, Dong H, Shi D, Li Y. A graphene quantum dot (GQD) nanosystem with redox-triggered cleavable PEG shell facilitating selective activation of the photosensitiser for photodynamic therapy. RSC Adv. 2016;6(8):6516–6522. doi:10.1039/C5RA23622C
- Lee JS, Kim S, Na HK, Min DH. MicroRNA‐responsive drug release system for selective fluorescence imaging and photodynamic therapy in vivo. Adv Healthcare Mater. 2016;5(18):2386–2395. doi:10.1002/adhm.201600328
- Su T, Cheng F, Yan J, et al. Hierarchical nanocomposites of graphene oxide and PEGylated protoporphyrin as carriers to load doxorubicin hydrochloride for trimodal synergistic therapy. J Mater Chem B. 2018;6(28):4687–4696. doi:10.1039/C8TB00733K32254413
- Ju J, Regmi S, Fu A, Lim S, Liu Q. Graphene quantum dot based charge-reversal nanomaterial for nucleus-targeted drug delivery and efficiency controllable photodynamic therapy. J Biophoton. 2019;12(6):e201800367. doi:10.1002/jbio.201800367
- Pack DW, Hoffman AS, Pun S, Stayton PS. Design and development of polymers for gene delivery. Nat Rev Drug Discov. 2005;4(7):581. doi:10.1038/nrd177516052241
- Panyam J, Labhasetwar V. Biodegradable nanoparticles for drug and gene delivery to cells and tissue. Adv Drug Deliv Rev. 2003;55(3):329–347. doi:10.1016/S0169-409X(02)00228-412628320
- Xiang Y, Oo NNL, Lee JP, Li Z, Loh XJ. Recent development of synthetic nonviral systems for sustained gene delivery. Drug Discov Today. 2017;22(9):1318–1335. doi:10.1016/j.drudis.2017.04.00128428056
- Midoux P, Pichon C, Yaouanc JJ, Jaffrès PA. Chemical vectors for gene delivery: a current review on polymers, peptides and lipids containing histidine or imidazole as nucleic acids carriers. Br J Pharmacol. 2009;157(2):166–178. doi:10.1111/j.1476-5381.2009.00288.x19459843
- Farjadian F, Moghoofei M, Mirkiani S, et al. Bacterial components as naturally inspired nano-carriers for drug/gene delivery and immunization: set the bugs to work? Biotechnol Adv. 2018;36(4):968–985. doi:10.1016/j.biotechadv.2018.02.01629499341
- Wong JK, Mohseni R, Hamidieh AA, MacLaren RE, Habib N, Seifalian AM. Will nanotechnology bring new hope for gene delivery? Trends Biotechnol. 2017;35(5):434–451. doi:10.1016/j.tibtech.2016.12.00928108036
- Karimi M, Solati N, Ghasemi A, et al. Carbon nanotubes part II: a remarkable carrier for drug and gene delivery. Expert Opin Drug Deliv. 2015;12(7):1089–1105. doi:10.1517/17425247.2015.100430925613837
- Chandrasekhar P. Medical and pharmaceutical applications of graphene In: Dalton, editor. Conducting Polymers, Fundamentals and Applications. Springer; 2018:149–150.
- Zhi F, Dong H, Jia X, et al. Functionalized graphene oxide mediated adriamycin delivery and miR-21 gene silencing to overcome tumor multidrug resistance in vitro. PLoS One. 2013;8(3):e60034. doi:10.1371/journal.pone.006003423527297
- Goncalves G, Marques P, Vila M. Graphene-Based Materials in Health and Environment. Springer; 2017.
- Jian H-J, Wu R-S, Lin T-Y, et al. Super-cationic carbon quantum dots synthesized from spermidine as an eye drop formulation for topical treatment of bacterial keratitis. ACS Nano. 2017;11(7):6703–6716. doi:10.1021/acsnano.7b0102328677399
- Lam P-L, Wong W-Y, Bian Z, Chui C-H GR. Recent advances in green nanoparticulate systems for drug delivery: efficient delivery and safety concern. Nanomedicine. 2017;12(4):357–385. doi:10.2217/nnm-2016-030528078952
- Teimouri M, Nia AH, Abnous K, Eshghi H, Ramezani M. Graphene oxide–cationic polymer conjugates: synthesis and application as gene delivery vectors. Plasmid. 2016;84:51–60. doi:10.1016/j.plasmid.2016.03.00227072918
- Zhou X, Laroche F, Lamers GE, et al. Ultra-small graphene oxide functionalized with polyethylenimine (PEI) for very efficient gene delivery in cell and zebrafish embryos. Nano Res. 2012;5(10):703–709. doi:10.1007/s12274-012-0254-x
- Hu H, Tang C, Yin C. Folate conjugated trimethyl chitosan/graphene oxide nanocomplexes as potential carriers for drug and gene delivery. Mater Lett. 2014;125:82–85. doi:10.1016/j.matlet.2014.03.133
- Zhu X, Zhang Y, Huang H, Zhang H, Hou L, Zhang Z. Functionalized graphene oxide-based thermosensitive hydrogel for near-infrared chemo-photothermal therapy on tumor. J Biomater Appl. 2016;30(8):1230–1241. doi:10.1177/088532821561958326759390
- Bao H, Pan Y, Ping Yet al., Chitosan‐functionalized graphene oxide as a nanocarrier for drug and gene delivery. Small. 2011;7(11):1569–1578. doi:10.1002/smll.20110019121538871
- Liu X, Ma D, Tang H, et al. Polyamidoamine dendrimer and oleic acid-functionalized graphene as biocompatible and efficient gene delivery vectors. ACS Appl Mater Interfaces. 2014;6(11):8173–8183. doi:10.1021/am500812h24836601
- Kou Z, Wang X, Yuan R, et al. A promising gene delivery system developed from PEGylated MoS 2 nanosheets for gene therapy. Nanoscale Res Lett. 2014;9(1):587. doi:10.1186/1556-276X-9-58725386104
- Feng L, Zhang S, Liu Z. Graphene based gene transfection. Nanoscale. 2011;3(3):1252–1257. doi:10.1039/c0nr00680g21270989
- Cao X, Zheng S, Zhang S, et al. Functionalized graphene oxide with hepatocyte targeting as anti-tumor drug and gene intracellular transporters. J Nanosci Nanotechnol. 2015;15(3):2052–2059. doi:10.1166/jnn.2015.914526413620
- Fan Z, Fu PP, Yu H, Ray PC. Theranostic nanomedicine for cancer detection and treatment. J Food Drug Anal. 2014;22(1):3–17. doi:10.1016/j.jfda.2014.01.00124673900
- Fan Z, Senapati D, Singh AK, Ray PC. Theranostic magnetic core-plasmonic shell star shape nanoparticle for the isolation of targeted rare tumor cells from whole blood, fluorescence imaging, and photothermal destruction of cancer. Mol Pharm. 2013;10(3):857–866. doi:10.1021/mp300468q23110457
- Li J, Lyv Z, Li Y, et al. A theranostic prodrug delivery system based on Pt (IV) conjugated nano-graphene oxide with synergistic effect to enhance the therapeutic efficacy of Pt drug. Biomaterials. 2015;51:12–21. doi:10.1016/j.biomaterials.2015.01.07425770993
- Gorgizadeh M, Azarpira N, Sattarahmady N. In vitro and in vivo tumor annihilation by near-infrared photothermal effect of a NiFe2O4/C nanocomposite. Colloids Surf B Biointerfaces. 2018;170:393–400.29945051
- Luo Y, Li Z, Zhu C, et al. Graphene-like metal-free 2D nanosheets for cancer imaging and theranostics. Trends Biotechnol. 2018;36(11):1145–1156. doi:10.1016/j.tibtech.2018.05.01229954613
- Chen H, Liu F, Lei Z, Ma L, Wang Z. Fe2O3@Au core@shell nanoparticle–graphene nanocomposites as theranostic agents for bioimaging and chemo-photothermal synergistic therapy. RSC Adv. 2015;5(103):84980–84987. doi:10.1039/C5RA17143A
- Bahreyni A, Yazdian-Robati R, Hashemitabar S, et al. A new chemotherapy agent-free theranostic system composed of graphene oxide nano-complex and aptamers for treatment of cancer cells. Int J Pharm. 2017;526(1–2):391–399. doi:10.1016/j.ijpharm.2017.05.01428495579
- Yang K, Hu L, Ma X, et al. Multimodal imaging guided photothermal therapy using functionalized graphene nanosheets anchored with magnetic nanoparticles. Adv Mater. 2012;24(14):1868–1872. doi:10.1002/adma.20110496422378564
- Lamb J, Fischer E, Rosillo-Lopez M, Salzmann CG, Holland JP. Multi-functionalised graphene nanoflakes as tumour-targeting theranostic drug-delivery vehicles. Chem Sci. 2019;10(38):8880–8888. doi:10.1039/C9SC03736E32874485
- Karki N, Rana A, Tiwari H, Negi P, Sahoo NG. Theranostics application of graphene-based materials in cancer imaging, targeting and treatment In: Lasfar A, Cohen-Solal K, editors. Tumor Progression and Metastasis. IntechOpen; 2020.
- Janib SM, Moses AS, MacKay JA. Imaging and drug delivery using theranostic nanoparticles. Adv Drug Deliv Rev. 2010;62(11):1052–1063. doi:10.1016/j.addr.2010.08.00420709124
- Bao X, Yuan Y, Chen J, et al. In vivo theranostics with near-infrared-emitting carbon dots—highly efficient photothermal therapy based on passive targeting after intravenous administration. Light. 2018;7(1):1–11. doi:10.1038/s41377-018-0090-1
- Pramanik A, Chavva SR, Fan Z, Sinha SS, Nellore BPV, Ray PC. Extremely high two-photon absorbing graphene oxide for imaging of tumor cells in the second biological window. J Phys Chem Lett. 2014;5(12):2150–2154. doi:10.1021/jz500985626270507
- Yang Y, Wang S, Wang C, Tian C, Shen Y, Zhu M. Engineered targeted hyaluronic acid–glutathione‐stabilized gold nanoclusters/graphene oxide–5‐fluorouracil as a smart theranostic platform for stimulus‐controlled fluorescence imaging‐assisted synergetic chemo/phototherapy. Chem Asian J. 2019;14(9):1418–1423. doi:10.1002/asia.20190015330829452
- Prasad R, Yadav AS, Gorain M, et al. Graphene oxide supported liposomes as red emissive theranostics for phototriggered tissue visualization and tumor regression. ACS Appl Bio Mater. 2019;2(8):3312–3320. doi:10.1021/acsabm.9b00335
- Kumawat MK, Thakur M, Bahadur R, et al. Preparation of graphene oxide-graphene quantum dots hybrid and its application in cancer theranostics. Mater Sci Eng C. 2019;103:109774. doi:10.1016/j.msec.2019.109774
- Lui CH, Mak KF, Shan J, Heinz TF. Ultrafast photoluminescence from graphene. Phys Rev Lett. 2010;105(12):127404. doi:10.1103/PhysRevLett.105.12740420867672
- Xu X, Liu C, Sun Z, et al. Interfacial engineering in graphene bandgap. Chem Soc Rev. 2018;47(9):3059–3099.29513306
- Xiong J, Yang Q, Li J, Zhou S. Effects of MDM2 inhibitors on vascular endothelial growth factor-mediated tumor angiogenesis in human breast cancer. Angiogenesis. 2014;17(1):37–50. doi:10.1007/s10456-013-9376-323907365
- Khurana R, Simons M, Martin JF, Zachary IC. Role of angiogenesis in cardiovascular disease: a critical appraisal. Circulation. 2005;112(12):1813–1824. doi:10.1161/CIRCULATIONAHA.105.53529416172288
- Carmeliet P, Jain RK. Angiogenesis in cancer and other diseases. nature. 2000;407(6801):249–257. doi:10.1038/3502522011001068
- Mukherjee S, Sriram P, Barui AK, et al. Graphene oxides show angiogenic properties. Adv Healthcare Mater. 2015;4(11):1722–1732. doi:10.1002/adhm.201500155
- Qian Y, Song J, Zhao X, et al. 3D fabrication with integration molding of a graphene oxide/polycaprolactone nanoscaffold for neurite regeneration and angiogenesis. Advan Sci. 2018;5(4):1700499. doi:10.1002/advs.201700499
- Sun Z, Huang P, Tong G, et al. VEGF-loaded graphene oxide as theranostics for multi-modality imaging-monitored targeting therapeutic angiogenesis of ischemic muscle. Nanoscale. 2013;5(15):6857–6866. doi:10.1039/c3nr01573d23770832
- Paul A, Hasan A, Kindi HA, et al. Injectable graphene oxide/hydrogel-based angiogenic gene delivery system for vasculogenesis and cardiac repair. ACS Nano. 2014;8(8):8050–8062. doi:10.1021/nn502078724988275
- Xue D, Chen E, Zhong H, et al. Immunomodulatory properties of graphene oxide for osteogenesis and angiogenesis. Int J Nanomedicine. 2018;13:5799. doi:10.2147/IJN.S17030530310282
- Ur Rehman SR, Augustine R, Zahid AA, et al. Reduced graphene oxide incorporated GelMA hydrogel promotes angiogenesis for wound healing applications. Int J Nanomedicine. 2019;14(9603):9603–9617. doi:10.2147/IJN.S21812031824154
- Shim G, Kim J-Y, Han J, et al. Reduced graphene oxide nanosheets coated with an anti-angiogenic anticancer low-molecular-weight heparin derivative for delivery of anticancer drugs. J Controlled Release. 2014;189:80–89. doi:10.1016/j.jconrel.2014.06.026
- Lai P-X, Chen C-W, Wei S-C, et al. Ultrastrong trapping of VEGF by graphene oxide: anti-angiogenesis application. Biomaterials. 2016;109:12–22. doi:10.1016/j.biomaterials.2016.09.00527639528
- Cibecchini G, Veronesi M, Catelani T, Bandiera T, Guarnieri D, Pompa PP. Antiangiogenic effect of graphene oxide in primary human endothelial cells. ACS Appl Mater Interfaces. 2020;12(20):22507–22518.32255338
- Wierzbicki M, Sawosz E, Strojny B, Jaworski S, Grodzik M, Chwalibog A. NF-κB-related decrease of glioma angiogenic potential by graphite nanoparticles and graphene oxide nanoplatelets. Sci Rep. 2018;8(1):1–9. doi:10.1038/s41598-018-33179-329311619
- Li J, Ge X, Cui C, et al. Preparation and characterization of functionalized graphene oxide carrier for siRNA delivery. Int J Mol Sci. 2018;19(10):3202. doi:10.3390/ijms19103202
- Stone V, Johnston H, Schins RPF. Development of in vitro systems for nanotoxicology: methodological considerations. Crit Rev Toxicol. 2009;39(7):613–626. doi:10.1080/1040844090312097519650720
- Liu K, Zhang -J-J, Cheng -F-F, Zheng -T-T, Wang C, Zhu -J-J. Green and facile synthesis of highly biocompatible graphene nanosheets and its application for cellular imaging and drug delivery. J Mater Chem. 2011;21(32):12034–12040. doi:10.1039/c1jm10749f
- Sanchez VC, Pietruska JR, Miselis NR, Hurt RH, Kane AB. Biopersistence and potential adverse health impacts of fibrous nanomaterials: what have we learned from asbestos? Wiley Interdiscip Rev Nanomed Nanobiotechnol. 2009;1(5):511–529.20049814
- Dreyer DR, Park S, Bielawski CW, Ruoff RS. The chemistry of graphene oxide. Chem Soc Rev. 2010;39(1):228–240. doi:10.1039/B917103G20023850
- Muthoosamy K, Abubakar IB, Bai RG, Loh H-S, Manickam S. Exceedingly higher co-loading of curcumin and paclitaxel onto polymer-functionalized reduced graphene oxide for highly potent synergistic anticancer treatment. Sci Rep. 2016;6:32808. doi:10.1038/srep3280827597657
- Cho YC, Pak PJ, Joo YH, Lee H-S CN. In vitro and in vivo comparison of the immunotoxicity of single- and multi-layered graphene oxides with or without pluronic F-127. Sci Rep. 2016;6:38884. doi:10.1038/srep3888427941848
- Zhao M-L, Liu X-Q, Cao Y, et al. Enhancement of interaction of L-929 cells with functionalized graphene via COOH+ ion implantation vs. chemical method. Sci Rep. 2016;6:37112. doi:10.1038/srep3711227845420
- Vuppaladadium SSR, Agarwal T, Kulanthaivel S, et al. Silanization improves biocompatibility of graphene oxide. Mater Sci Eng C. 2020;110:110647. doi:10.1016/j.msec.2020.110647
- Sydlik SA, Jhunjhunwala S, Webber MJ, Anderson DG, Langer R. In vivo compatibility of graphene oxide with differing oxidation states. ACS Nano. 2015;9(4):3866–3874. doi:10.1021/acsnano.5b0129025849074
- Zhang X, Yin J, Peng C, et al. Distribution and biocompatibility studies of graphene oxide in mice after intravenous administration. carbon. 2011;49(3):986–995. doi:10.1016/j.carbon.2010.11.005
- Nurunnabi M, Khatun Z, Huh KM, et al. In vivo biodistribution and toxicology of carboxylated graphene quantum dots. ACS Nano. 2013;7(8):6858–6867. doi:10.1021/nn402043c23829293
- Yang K, Zhang S, Zhang G, Sun X, Lee S-T LZ. Graphene in mice: ultrahigh in vivo tumor uptake and efficient photothermal therapy. Nano Lett. 2010;10(9):3318–3323. doi:10.1021/nl100996u20684528
- Reina G, González-Domínguez JM, Criado A, Vázquez E, Bianco A, Prato M. Promises, facts and challenges for graphene in biomedical applications. Chem Soc Rev. 2017;46(15):4400–4416. doi:10.1039/C7CS00363C28722038
- Bianco A. Graphene: safe or toxic? The two faces of the medal. Angewandte Chemie Int Ed. 2013;52(19):4986–4997. doi:10.1002/anie.201209099
- Zhang Y, Ali SF, Dervishi E, et al. Cytotoxicity effects of graphene and single-wall carbon nanotubes in neural phaeochromocytoma-derived PC12 cells. ACS Nano. 2010;4(6):3181–3186. doi:10.1021/nn100717620481456