Abstract
Diabetic wound shows delayed and incomplete healing processes, which in turn exposes patients to an environment with a high risk of infection. This article has summarized current developments of nanoparticles/hydrogels and nanotechnology used for promoting the wound healing process in either diabetic animal models or patients with diabetes mellitus. These nanoparticles/hydrogels promote diabetic wound healing by loading bioactive molecules (such as growth factors, genes, proteins/peptides, stem cells/exosomes, etc.) and non-bioactive substances (metal ions, oxygen, nitric oxide, etc.). Among them, smart hydrogels (a very promising method for loading many types of bioactive components) are currently favored by researchers. In addition, nanoparticles/hydrogels can be combined with some technology (including PTT, LBL self-assembly technique and 3D-printing technology) to treat diabetic wound repair. By reviewing the recent literatures, we also proposed new strategies for improving multifunctional treatment of diabetic wounds in the future.
Introduction
Diabetes mellitus is a complex metabolic disorder that affects the health of millions of people around the world. The world of diabetes among adults (aged 20–79 years) are 285 million adults, which will increase to 439 million adults by 2030.Citation1,Citation2 Diabetics will lead to high glucose condition as well as a variety of complications, including cardiovascular disease, nerve damage (neuropathy), kidney damage (kidney disease), eye damage (retinopathy), hearing impairment, dementia, and especial the delayed wound healing which is one of the most serious complications of diabetes impaired wound healing.Citation3–Citation9 Severe diabetic wounds could lead to amputation.Citation10 Diabetes can be divided into four main types: Type I diabetes is caused by the autoimmune be destroyed of β-cells in the pancreas, eventually leading to reduction of insulin production;Citation11,Citation12 Type II diabetes is closely associated with insulin resistance and subsequent decompensation of pancreatic β-cells (including pancreatic β-cell mass loss and β-cell dysfunction);Citation13 Gestational diabetes occurs during pregnancy and causes glucose intolerance;Citation14 other diabetes are resulted by specific genetic defects of beta-cell function, illness of the pancreas, drugs or chemicals, etc.Citation15
Normal Wound Healing
Wound healing is a complex and ordered biological process, including four classical stages: hemostasis, inflammation, proliferation and remodeling,Citation16–Citation18 involving different cell types releasing many cytokines and growth factors (GFs).Citation19,Citation20 Hemostasis lasts 2–3 hours, the fibrin plug is formed, and aggressive platelets release pro-inflammatory mediators such as cytokines and growth factors. Cytokines recruit neutrophils and monocytes to the wound area, triggering an inflammatory period of wound healing that lasts from hours to days.Citation21 The inflammatory phase begins immediately after injury and can last from hours to days in acute wounds, while in chronic wounds it can last for weeks or even months due to the effects of the underlying disease (eg, diabetic foot ulcers).Citation16 Injury results in the rupture of vessels, and form clots and temporary extracellular matrix (ECM), which closes the wound, reduces blood loss, and helps guide cell migration.Citation22 Platelets secrete and activate cellular mediators that attract inflammatory cells (multinucleated cells and macrophages), fibroblasts, and endothelial cells.Citation23 In the proliferation, endothelial cell and fibroblast proliferation and migration promote angiogenesis and new ECM formation.Citation16 As the new ECM is reconstructed, the old matrix is degraded by proteases (matrix metalloproteinases, MMPs), MMPs promote autolytic debridement and cell migration in wounds.Citation16,Citation17 The level of MMPs in wounds increases after tissue damage and decreases with remission of inflammation, but increases abnormally in chronic wounds.Citation17 Epithelial cells migrate from the edge of the wound, initiating epithelialization.Citation24 Keratinocyte differentiation helps restore the barrier function of the epidermis.Citation25 Remodeling can last for months and eventually an eschar (scab) has formed on the surface of the wound.Citation17 The matrix is constantly reconstructed by myofibroblasts.Citation16 The microfilaments attached to the ECM densify the collagen network and contract the wound.Citation26 At the same time, new components are secreted to increase matrix density and stability.Citation26,Citation27 The proportion of different types of collagen began to change: the proportion of type I collagen increased (80%–90%) and the proportion of type III collagen decreased (10%–20%).Citation28 Apoptosis reduces the density of myofibroblasts, making room for fibroblasts, further strengthening the ECM, and increasing its resistance to mechanical forces.Citation26
Diabetic Wound Healing
Under normal physiological conditions, the injured tissue will initiate the acute wound healing process.Citation16–Citation18 However, when the healing process is disturbed by the underlying pathological mechanism or microbial invasion, the wound cannot heal and become a chronic wound (such as diabetic wound)Citation29 (). Hypoxia is a major cause of diabetic wound damage caused by two factors: limited oxygen supply and high oxygen consumption in the wound.Citation30 In diabetic patients, oxygen supply to the wound is limited due to vascular dysfunction and neuropathy.Citation30 In addition to inadequate oxygen supply, high oxygen consumption by wound cells during inflammation also induces hypoxia in wounds.Citation30 In diabetic patients, the imbalance between angiogenic factors (eg, transforming growth factor-β, TGF-β; fibroblast growth factor 2, FGF2; vascular endothelial growth factor, VEGF; angiopoietins) and angiostatic factors (eg, thrombospondins, endostatin, angiostatin) may lead to angiogenic imbalance and aggravate wound hypoxia.Citation31 Likewise, hypoxia can amplify the inflammatory response, thereby prolonging injury by increasing the levels of oxygen radicals.Citation32 Two main events for effective wound healing involve an inflammatory response and migration of keratinocytes, fibroblasts, and endothelial cells. However, diabetic wounds did not undergo a normal healing process rather trapped into a chronic inflammatory stage characterized by excessive accumulation of uninhibited M1 macrophages.Citation33 Moreover, the fibroblast proliferation, function, and differentiation into myofibroblast also significantly reduced with suppressed expression of tumor growth factor β type II receptor and decreased collagen synthesis, which hindered the tissue remodeling stage.Citation34 The high glucose levels mediated-induction of matrix metalloproteases-9 (which is responsible for collagen degradation and regulates keratinocytes migration) overexpression in diabetic models impaired keratinocyte migration.Citation35,Citation36 High glucose could also reduce the activity of VEGF and hypoxia-inducible factor 1α (HIF-1α), and increases the non-enzymatic glycation of many important proteins, leading to abnormal cellular and ECM function, thereby inhibiting angiogenesis in diabetic wounds.Citation37
Figure 1 The physiological process of normal wound and diabetic wound. Unlike normal wounds, diabetic wounds are characterized by impaired angiogenesis, excessive inflammatory macrophages. Excessive production of matrix metalloproteinases (MMPs) at the wound site, and hyperglycemia leads to an increase ROS that prevent the formation of healthy tissue.
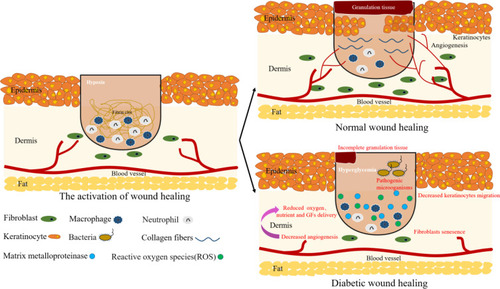
General wound clinical therapies include restoration of skin perfusion, treatment of infection, metabolic control, treatment of co-morbidity and local wound care.Citation38 Although these standard treatments may achieve the goal of symptom control, but the effective treatment of diabetic wound healing remains limited. Moreover, traditional treatment mainly use dressings, the treatment process is long, easy to cause secondary injury, psychological and physiological adverse effect on diabetic patients. Currently, there are some technologies of wound healing treatment for diabetes, namely topical drug treatment (eg, drugs, peptides and growth factors), cellular therapies (eg, stem cells and fibroblasts) and biomaterial-based treatment.Citation39 Biomaterials with controlled-release of signaling molecules can be combined with other therapeutic methods, which is a promising treatment method for diabetic wound healing.Citation40 In this review, we will summarize biomaterials and their potential applications in diabetic wound repair. In addition, we have also reviewed the challenges and application prospects of biomaterials in diabetic wound healing.
Substances Applied in Diabetic Wound Healing
Diabetic wounds often require a longer healing period due to persistent inflammation, bacterial infections, and degradation or diminished expression of growth factors.Citation41 In addition, chronic hyperglycemia makes new vessel formation is difficult, thus limiting the access of oxygen and nutrients to the wound site.Citation42 Therefore, some substances need to be delivered from the outside to promote the healing of diabetic wounds. At present, a variety of bioactive molecules (such as growth factors, genes/proteins/peptides, stem cells/exosomes, etc.) and non-bioactive substances (metal ions, oxygen, nitric oxide, etc.) are widely used to promote diabetic wound healing ().
Figure 2 Schematic illustration of the categories of biomaterials used on diabetic wounds. Biomaterials are loaded with bioactive molecules (including GFs, genes/proteins/peptides, stem cells/exosomes, etc.) and non-bioactive substances (including metal ions, oxygen, etc.) to promote diabetic wound healing.
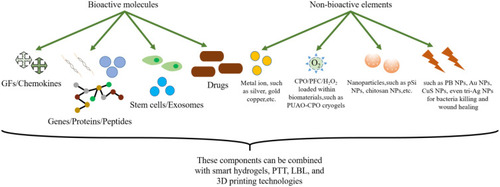
Bioactive Molecules
Signaling Molecules
It is well known that chemokines can directly promote angiogenesis, ECM remodeling or formation and re-epithelialization.Citation43,Citation44 These chemokines play a crucial role in the migration of inflammatory cells and mesenchymal stem cells.Citation45 Moreover, the chemokines (interleukin-8, IL-8; macrophage inflammatory protein-3α, MIP-3α) that possess the ability to recruit bone marrow-derived mesenchymal stem cells (BMSCs) for articular cartilage repair.Citation46 In addition, IL-8 is also known to be a potent promoter of angiogenesis.Citation47 Previous studies have shown that horseradish peroxidase triggered in-situ cross-linked gelatin-hydroxyphenyl propionic acid (GH) hydrogels can be used as an injectable carriers for tissue engineering and regenerative medicine.Citation48,Citation49 Therefore, two types of chemokines (IL-8 and MIP-3α) could be loaded into GH hydrogel.Citation50 IL-8/MIP-3α was released through GH hydrogel within 7 days, and endogenous cells were able to attract chemokines to the wound. The incorporation of chemokines did not affect hydrogels' properties (including swelling ratio and mechanical stiffness), and the bioactivities of IL-8 and MIP-3α were stably maintained in GH hydrogel. GH/IL-8 and GH/MIP-3α hydrogel dressings promote diabetic wound regeneration, enhance re-epithelialization/neovascularization and collagen deposition.Citation50 GH hydrogel can be used as a delivery platform for various therapeutic proteins for wound healing in the future.
Various growth factors are important function in mediating, coordinating and controlling cellular interactions during normal wound healing.Citation51 But in diabetic wounds, the balance of many growth factors is upset, damaging angiogenesis, disrupting the ECM, and ultimately delaying wound healing (). A strategy of mediating the diabetic wound cell signaling is to locally administering endogenous therapeutic growth factors.Citation52 However, repeated high doses of growth factors are needed to achieve therapeutic effects.Citation53 And the proteases in the cells cause growth factors to degrade rapidly. Therefore, a delivery system is required not only to maintain growth factor activity, but also to enable sustained and controlled release of growth factors to the target. Currently, various systems (including nanoparticles, hydrogels and nanofibers) have been used for growth factors delivery in diabetic woundCitation54–Citation61 ().
Table 1 Growth Factors in Nanoparticles/Hydrogels/Scaffolds Used in Experimental Diabetic Wound Healing Studies
Figure 3 Changes in GFs in diabetic wounds. (A) Changes in growth factors in diabetic wounds and their effects on angiogenesis and ECM. (B) Hyperglycemia leads to the production of oxygen free radicals.
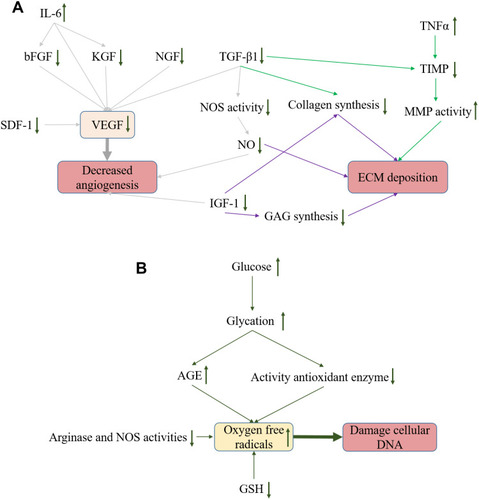
Previous studies have shown that VEGF-A is the primary pro-angiogenic factor in normal healing wounds.Citation62 Its expression reached a peak at 2–3 days after injury and continued to rise for about a week.Citation63 However, compared to normal mice, the increase of VEGF in db/db diabetes mice is transient, rather than sustained, and rapidly decreases to almost undetectable levels when granulation tissue is formed.Citation64 The results of clinical trials showed that single-dose application of VEGF to wounds alone has limited success due to its short half-life.Citation65 Repeated local delivery of VEGF-165 promoted rapid re-epithelialization and enhanced angiogenesis of diabetic wounds.Citation66–Citation68 In order to overcome the disadvantages of short half-life and repeated delivery of VEGF, delivering VEGF by gene activation strategy should be effective. Physical encapsulation of nucleic acid-carrier complexes in a hydrogel can protect the carrier from degradation and provide more sustained, localized transfection compared with rapid delivery of growth factors or genes.Citation69 Hyaluronic acid (HA), the main component of the ECM, is a highly biocompatible biomaterial, which can also promote angiogenesis.Citation70 Porous HA hydrogel with encapsulated proangiogenic (pVEGF) plasmids for local gene therapy in diabetic wound healing.Citation70 These researches have shown that porous hydrogels did not degrade and provided a mechanical barrier to wound healing. However, transfection levels of pVEGF did not appear to be high enough to enhance angiogenesis by increasing vascular density or size.Citation70 Devalliere et al recombined keratinocyte growth factor (KGF) and cytoprotective peptides into a protein polymer with the aim of increasing their activity in vivo (enhancing anti-proteolytic ability), thereby accelerating chronic wound healing (increasing wound bed angiogenesis).Citation71 Previous studies have shown that two or more growth factors are more effective at stimulating angiogenesis and subsequent tissue repair than a single growth factor.Citation72,Citation73 For example, co-stimulation of VEGF-A and fibroblast growth factor 2 (FGF-2) significantly improves cell migration and angiogenesis in vivo compared to single angiogenic growth factor.Citation74 In addition, the delivery of bFGF and VEGF as dual factors immediately restored the vascular network.Citation75
In the future, local controlled delivery of multiple GFs by the combination of nanoparticles and hydrogels. At the same time, determine the optimal degradation rate of the hydrogel and reduce the toxicity of the nanoparticles to allow for overall faster wound closure.
Genes/Proteins/Peptides
Targeting disease-related miRNA may be an effective therapeutic strategy in comparison with single-target angiogenic growth factors, since an individual miRNA with its pleiotropic effects can regulate multiple different genes and processesCitation76 (). Recently, miR-26a has been identified as a key negative regulator of angiogenesis in diabetic wounds, inhibition of this miRNA may be an effective treatment for diabetes.Citation76 Wu et al developed a redox-modulatory ceria nanozyme-reinforced self-protecting hydrogel (PCN-miR/Col).Citation77 PCN-miR/Col not only remodeled the oxidative wound microenvironment, but also ensured the structural integrity of the encapsulated pro-angiogenic miRNA in the oxidative microenvironment.Citation77 The design adopted the “seed-and-soil” concept in the regenerative medicine field with the aim to reshaping the oxidative wound microenvironment into a proregenerative one (the “soil”), and providing proangiogenic miRNA cues for diabetic wound repair and regeneration (the “seed”). The proposed “seed-and-soil” strategy is applicable to the repair and regeneration of a wide range of damaged tissues, which exposed to highly oxidative diseased microenvironments and dysfunctional biomacromolecules.Citation77 So, it is a new direction of treatment for diabetic wounds. Li et al had developed a β-CD-(D3)7 as the gene carrier to carry siRNA, which effectively interfered with the expression of MMP-9, accelerated wound healing and could not cause organ damage and accumulation.Citation78 The results suggest that this gene carrier might be developed as a novel topical agent for the diabetic wound treatment. See () for other systems.Citation79–Citation81
Table 2 Genes/Proteins/Peptides Used in Diabetic Wound Healing are Summarized. Most of the Genes are miRNA, and There are Both Natural and Synthetic Peptides
Although genes and growth factors are intended to improve angiogenesis and re-epithelialization, cost and safety issues remain in their application.Citation82 Introduction of peptides into hydrogels or scaffolds has been widely used to confer these tissue-engineering substrates with bioactivityCitation83–Citation90 (). Carrejo et al prepared a multidomain peptide hydrogel that can rapid cell infiltration and elicit a mild inflammatory response, thereby promoting angiogenesis and accelerating wound closure in diabetes.Citation91 A therapeutic integrin-binding pro-survival peptide-engineered silk fibroin nanosheet that can regulate angiogenesis and promote diabetic ulcer healing.Citation92 Nanofibrous mats of Antheraea assama silkworm silk fibroin are coated with various recombinant spider silk fusion proteins through silk–silk interactions to fabricate multifunctional wound dressings.Citation93
We recently reviewed the roles of peptides in diabetic wound healing. Research spans the use of native proteins, recombinant proteins, and engineered peptides with integration in a diverse set of substrates from conjugation to hydrogel matrices. While the current studies have demonstrated the benefits of these strategies, the key consideration should be how to promote diabetic wound repair. A large number of clinical trials should be carried out to conduct an overall evaluation of the efficiency and safety of peptides in diabetic wound healing.
Stem Cells/Exosomes
Stem cells can produce various bioactive substances (such as growth factors) to restore tissue/organ function, so stem cell therapy is one of the most promising methods for diabetic woundsCitation94–Citation101 (). Epidermal growth factor (EGF)-loaded microcapsules and human adipose-derived stem cells (ADSCs) are integrated into the collagen hydrogel and facilitate tissue regeneration and effectively restore blood perfusion.Citation102 Moreover, acrylated hyaluronic acid (AHA) hydrogels load pluripotent stem cells (hiPSCs) treat type-1 diabetic wounds.Citation103 In addition, gingival mesenchymal stem cells (GMSCs) loaded into the chitosan/silk hydrogel sponge effectively promote the skin wound healing.Citation104 With the capacity to protect and regulate immune function during the healing process, macrophages (MΦ) especially MΦ2, contribute to reduce inflammation and promote proliferation and angiogenesis by releasing anti-inflammatory cytokines and growth factors (such as TGF-β and VEGF).Citation97 Although the pullulan−collagen composite hydrogel has been reported to deliver monocytes or macrophages to the wound bed, the interaction between immune cells and the material is unclear. Liu et al have reported a 0.5Cu-HHA/PVA@MΦ2 hydrogel to provide and regulate MΦ2 for synergistic improvement of immunocompromise and impaired angiogenesis to accelerate the diabetic chronic wound healing phase transition from inflammation to proliferation and remodeling.Citation97 Hydrogels facilitate the adhesion, growth, migration and regeneration of immune cells.
Table 3 Summary of Stem Cell/Exosomes Towards Effective Control of Diabetic Wounds
Exosomes are nanoscale membrane vesicles (30–150nm in diameter) that can be identified by the expression of exosome-related markers (such as Alix, Tsg101, CD9, CD63 and CD81) and carry functional complexes of proteins, lipids and nucleic acids.Citation105–Citation108 Therefore, exosomes are considered as drug delivery carriers, and natural RNA carriers for the treatment of diseases.Citation109–Citation111 And exosomes are considered as one of the most important secretory products of bone marrow mesenchymal stem cells, which can mediate intercellular communication and promote wound healing.Citation112–Citation115 Polypeptide-based FHE hydrogel (F127/OHA-EPL) contains adipose-derived mesenchymal stem cell exosomes (AMSCs-exo),Citation116 FHE@exo hydrogel has multifunctional properties of biological activity, including injectability, self-healing, antibacterial activity, and exosome release. And it can significantly improve the proliferation, migration and angiogenesis of human umbilical vein endothelial cells (HUVECs). FHE@exo hydrogel promotes neovascularization and cell proliferation, leading to faster granulation tissue formation, re-epithelialization, and collagen remodeling at wound sites, thus accelerating the healing process of diabetic wounds.Citation116 Wang et al developed an injectable thermosensitive multifunctional polysaccharide-based dressing (FEP) with sustained pH-responsive exosome release that promotes angiogenesis and diabetic wound healing.Citation117 Moreover, hydroxyapatite/chitosan or chitosan hydrogel incorporating microRNA-126-overexpressing synovium mesenchymal stem cells (SMSC-126-Exos) can accelerate re-epithelialization, stimulate the proliferation of human dermal fibroblasts, and activate angiogenesis.Citation118,Citation119 Exosome-based hydrogels hold great promise in the treatment of chronic wounds (especially diabetic wounds) and skin regeneration. Therefore, it may become a treatment means in the future.
Drugs
Under the framework of pharmaceutical and clinical challenges of drug delivery in diabetic wound infections, an ideal drug delivery system must deliver the drug in deep layers of skin. The nanoscale local drug delivery system, combined with hydrogel/nanoparticles properties, can stabilize the long-term release of drugs to the wound and promote healing.Citation120 At present, a variety of complex delivery systems have been developed to extend drug delivery timeCitation121–Citation126 (). A multi-responsive composite polydopamine/nanocellulose hydrogel with the ability of drug (tetracycline hydrochloride) release and wound healing.Citation127 The drug can be released continuously for more than 24 hours, and no explosive drug release occurs at the beginning of the release process. The maximum drug release ratio reached 77%, with long-term drug delivery properties.Citation127 Desferrioxamine (DFO) is used as a hypoxic-mimetic agent, and has been used for the induction of HIF-1α accumulation.Citation128–Citation130 And HIF-1α has been shown to play an important role during the wound healing.Citation131 DFO-loaded hydrogel/scaffolds by upregulating HIF-1α that rapidly promote angiogenesis for diabetic skin regenerationCitation132–Citation134 (). In addition, mixed DFO and bioglass (BG) in sodium alginate hydrogel, BG and DFO could synergistically upregulate HIF-1α and VEGF expression, and subsequently vascularization in the wound sites, and better facilitate diabetic skin wound healing.Citation135 Insulin is a universal drug for treating diabetes because it is a physiological glucose-lowering agent. The use of topical insulin became of greater interest as a healing agent in diabetic foot ulcers.Citation136 However, the use of topical insulin presents a great challenge due to the instability of the molecule. In order to ensure molecular stability of insulin, it is incorporated into hydrogels to maximize its effect. Recently, the drug- and cell-loaded hydrogels have promising potential in wound healing. Protein drugs (insulin) and live cells (fibroblasts L929) can be encapsulated in the pH and glucose dual-responsive injectable hydrogels, this hydrogel dressing could promote neovascularization and collagen deposition and enhance the wound-healing process of diabetic wounds.Citation137 The combination of drugs and cell/growth factors hydrogel may be a promising approach to enhance wound healing and can also be used for the regeneration of other vascularized tissues. A kind of aligned porous poly (L-lactic acid) electrospun fibrous membranes loaded mesoporous silica nanoparticles that release dimethyloxalylglycine for diabetic wound healing.Citation138 Encapsulation of curcumin nanoparticles with MMP9-responsive and thermos-sensitive gelatin microspheres hydrogel improves diabetic wound healing.Citation139–Citation141
Table 4 Delivery of Drug/Natural Macromolecular Bioactive Substances Systems with Effective Control of Chronic/Diabetic Wounds
Figure 4 Development of a transdermal drug delivery system for DFO. (A) DFO patch is administered through transdermal drug delivery system into the dermis to perform its functions. (B) Functional diagram of DFO and its regulation in the HIF-1a signaling pathway.
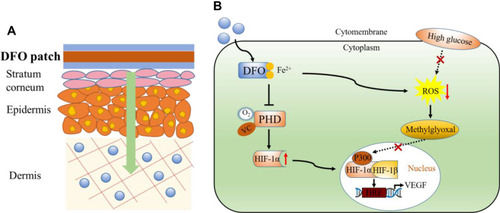
Improving some properties of composite scaffolds/hydrogels can increase drug load and control drug release. When scaffolds/hydrogels are subjected to certain stimulation (near infrared laser irradiation or pH), drugs are released in “on-off” mode without explosive drug release at the beginning of the release process, and has long-term drug delivery performance. In the future, our research direction should be precise administration, which can be achieved gradually at different stages of wound healing. Wound-healing research will need to incorporate hydrogels, which can deliver more DNA, growth factors, peptides and drugs, increasing angiogenesis and wound healing. It is also important to determine the optimal hydrogel degradation rate and water content for faster wound healing, while maintaining complex release and mechanical support to the wound bed. However, emerging drug resistance and physicochemical characteristics require the design of more accurate topical drug delivery systems which could be combined with 3D technology to achieve high functional efficiencies in terms of permeability, stability and therapeutic efficacy.
Non-Bioactive Elements
Metal Ion
Currently, the antibacterial nanoparticles used in wound healing are silver nanoparticles, gold nanoparticles, copper nanoparticles, nano-bioactive glass particles, etc.Citation142–Citation147 Among different metal nanoparticles, AgNP is the most active nanoparticle due to its unique anti-inflammatory properties and antibacterial activity against natural and nosocomial strains of multidrug-resistant (MDR) microorganisms, promoting wound healing.Citation148 The mechanisms of antimicrobial action of AgNPs are of two types, (a) the inhibitory action and (b) the bactericidal action.Citation15 Such as NIR laser-excited silver triangular nanoparticles (Tri-Ag) can eradicate multidrug-resistant bacteria and promote wound healing.Citation149 Tong et al constructed a combinational antibacterial system by loading AgNPs on the polydopamine-modified prussian blue NPs(PB@PDA@Ag), the bactericidal mechanism of this system can be attributed by damaging cell integrity, producing ROS, the reducing ATP and disrupting bacterial metabolism.Citation150 Likewise, Zhao et al used polydopamine decorated silver nanoparticles, and then loaded into conductive hydrogel to inhibit bacterial growth and control diabetic wound infection.Citation151 Gold NPs (AuNPs) can perform gene transfer, drug delivery, as biosensors and cancer cell imaging, angiogenesis as well as wound healing.Citation152–Citation155 AuNPs could inhibit the lipid from peroxidation and prevents the formation of ROS to restores antioxidant discrepancies.Citation15 AuNP combines with epigallocatechin gallate (EGCG) or alpha-lipoic acid (ALA) or both (EA) to achieve synergistic effects and enhance diabetic wound healing by modulating angiogenesis and anti-inflammatory effects.Citation156,Citation157 Wang et al optimized a novel gene delivery system based on antimicrobial peptide (LL37) grafted ultra-small gold nanoparticles for the topical treatment of diabetic wounds with or without bacterial infection.Citation158 Copper nanoparticles (CuNPs) have been gained increasing attention due to its antibacterial activity in diabetic foot ulcer infectionsCitation159,Citation160 (). Bhadauriya et al focused on the synthesis of the yeast extract-immobilized and copper nanoparticle-dispersed carbon nanofibers as a potential diabetic wound dressing material.Citation161 Copper-based metal−organic framework nanoparticles can be modified to slowly release Cu2+, which reduces toxicity and improves wound healing in diabetes.Citation147,Citation159,Citation160 However, rapid oxidation and agglomeration of copper nanoparticles are key problems during their use, and needs to control the stability of CuNPs by using biocompatible stabilizer such as chitosan and folic acid.Citation159–Citation161
Table 5 Metallic and Metal Oxide Nanomaterials are Used in Diabetic Wound Repair
In recent years, bimetallic/polymetallic composite nanomaterials have great potential in diabetic wound repair.Citation162–Citation164 Das et al reported a bimetallic (Fe-Cu) wound healing dressing material that exhibited antimicrobial activity against methicillin-resistant Staphylococcus aureus and displayed in vivo diabetic wound healing property.Citation165 Bioactive glass (BG), which is typically composed of Na2O, SiO2, CaO and P2O5, is considered to be a classical material for hard tissue regeneration.Citation166 The ionic products of bioglass dissolution stimulate macrophages to secrete anti-inflammatory factors.Citation167 Zeng et al combined bioglass and sodium alginate for diabetic wound healing.Citation168 Jiang et al found that the Si ions released by bioactive glass up-regulated the expression of VEGF.Citation169
In the future, the reversible swelling-shrinking transition of hydrogels can be utilized to achieve controllable and sustained release of Ag+, Cu2+ and Si2+ etc., so as to avoid the explosive ion release causing damage to cells. This system has great potential in tissue repair and antibacterial application.
Oxygen
Chronic diabetic wounds are limited in oxygen supply due to vascular dysfunction and neuropathy.Citation170 In addition, high oxygen consumption by cells during inflammation also leads to hypoxia in the wounds. Due to the increased utilization of oxygen in some regenerated tissues, there are inevitable differences between the supply and demand of oxygen, and the result is a hypoxic environment with high oxidative stress.Citation171 In addition, increased oxidative stress in diabetic ulcers is caused by macrophages and neutrophils producing more ROS in a hyperglycemic response.Citation172,Citation173 Hence the need for multifaceted biomaterials that will simultaneously reduce the oxidative stress, provide oxygen, and induce angiogenesis. To reduce oxidative stress, Zhu et al developed a thermoresponsive antioxidant poly(polyethylene glycol co-citric acid-co-N-isopropylacrylamide) hydrogel (PPCN) that uses the laminin-derived dodecapeptide A5G81 (PPCN-A5G81). A5G81 peptide conjugation to PPCN via the cross-linker N-β-maleimidopropionic acid hydrazide. A5G81 has unique receptor-mediated and antioxidant properties and is beneficial to the diabetic wound repair.Citation174 Novel nanofibrous mats (chitosan/poly (vinyl alcohol)/ZnO nanofibrous) with antibacterial and antioxidant properties for diabetic wound healing,Citation175 but the mechanism of the healing of diabetic wounds caused by nanofiber mats has not been clarified in this study.
Oxygen Containing Nanocarriers
At present, oxygen-producing materials are mainly delivered through some nanoscale systems to relieve wound hypoxia. The main oxygen-producing materials are sodium percarbonate (SPO), calcium peroxide (CaO2), magnesium peroxide (MgO2), hydrogen peroxide (H2O2), and fluorinated materials.Citation176–Citation180 It has been shown that the combination of nanomedicine and some oxygen-producing agents can improve the wound healing of diabetes.Citation30 Recently, Shiekh et al developed a porous cryogels (polyurethane polymeric material-calcium peroxide, PUAO-CPO), PUAO-CPO cryogels can not only continuously release oxygen, but also supplement with adipose-derived stem cell (ADSCs) exosomesCitation181 (). Nanoperfluorocarbon (nano-PFC) has been widely studied as an oxygen-carrying system to overcome hypoxia-associated resistance in cancer therapies due to its high oxygen affinity and good biocompatibility.Citation182–Citation184 In addition, PFC has been approved by the USA Food and Drug Administration (FDA) to improve myocardial oxygenation and prevent ventricular dysfunction.Citation185,Citation186 Therefore, nano-PFC can be used as a nano-drug delivery system (NDDS) to deliver molecules, such as drugs and oxygen to target tissues, and release the contents under the stimulus of external conditions.Citation187,Citation188 Wang et al combined the radial extracorporeal shock wave therapy (rESW) with oxygen-carrying nano-PFC to provide targeted oxygen supply, improving blood microcirculation of DFUs and accelerating wound healing.Citation189 And when nano-PFC is injected into the blood circulation, the nanodroplets triggered by rESW can reversibly release oxygen within the tumor tissueCitation189 (). This strategy offered a great potential for further clinical trials. However, the potential safety problems of PFC-based micro/nanomaterials cannot be ignored. Therefore, a large number of experiments are needed to further prove its reliability. In addition, Zehra et al developed a polycaprolactone (PCL)-based oxygen-releasing electrospun wound dressings. The dressing can produce oxygen continuously for up to 10 days and stimulate angiogenesis.Citation190 These oxygen-loading nanomaterials can improve wound healing efficiency. Therefore, oxygen-producing biomaterials are essential to cure chronic diabetes wounds in the futureCitation191–Citation194 ().
Table 6 Summary of Current O2 Delivery Systems
Hydrogen Sulfide Containing Nanocarriers
Besides, the synthesis and levels of circulating hydrogen sulfide (H2S) are reduced in diabetic mellitus.Citation195 Studies have shown that H2S can stimulate cell proliferation and migration and regulate ECs assembly into capillary structures.Citation196 Therefore, exogenous H2S supplementation is a promising treatment method to promote refractory wound healings in diabetic. Lin et al used emulsion technique to prepare NaHS particles (NaHS@MPs), which could be used as in situ depot for continuous release of exogenous H2S under physiological conditions.Citation197 The sustained release of H2S from NaHS@MPs promotes several cell behaviors, including epidermal/endothelial cell proliferation and migration, as well as angiogenesis, by extending the activation of cellular ERK1/2 and p38, accelerating the healing of full-thickness wounds in diabetic mice.Citation197
Nitric Oxide Containing Nanocarriers
NO is an antibacterial agent effective against a broad range of bacteria, including biofilm forming microorganisms, through an oxidation process involving free radical superoxide (O2*−) to form peroxynitrite (−OONO).Citation198 Nitric oxide (NO) plays a key role in the physiological regulation of vascular function, but in diabetic patients, NO synthesis and bioavailability decrease as well as NO consumption increases.Citation199,Citation200 A direct and effective strategy for promoting diabetic skin ulcer healing is exogenous supplement of NO. Chen et al activated the NO-sGC-cGMP pathway by inducing long-term NO release (t= 27.4±0.5 h at 25°C and 16.8±1.8 h at 37°C) and maintaining the angiogenesis process.Citation201
Technology
Nanoparticles
Nanoparticle (NP) is a basic component of nanostructure and has its unique size and characteristics.Citation202 NPs applications mainly include drug and gene delivery, tissue engineering and fluorescent biological labels, etc.Citation203–Citation206 (). Currently, the NPs used in diabetics wound healing mainly include metallic and metal oxide nanomaterials, nonmetallic nanomaterials (). NPs and nanotechnology allow them to achieve high local drug concentrations with relatively few side effects compared to traditional drug delivery systems, so drug therapy is more effective. There are two main criteria of nanomaterials used in wound healing (1) nanomaterials that are beneficial to wound healing; (2) nanomaterials as delivery vehicles.
Table 7 Other Approaches for Diabetic Wound Repair
Metallic and Metal Oxide Nanomaterials
The antibacterial mechanism of silver is realized by blocking the respiratory enzyme pathways and altering the microbial DNA and cell wall.Citation15 Tong et al promoted diabetic wound healing through the antibacterial of silver ions.Citation150 Copper ions can also stimulate angiogenesis and collagen deposition processes in addition to antibacterial effects, thus improving diabetic wound healing.Citation159–Citation161 Rubidium (Rb) is an important microelement for the human body. Rb+ has been reported to inhibit or kill bacteria by affecting membrane potential.Citation207 He et al loaded rubidium into calcium alginate hydrogel to achieve antibacterial and promote diabetic skin wound healing.Citation208 The intrinsic antibacterial properties of zinc oxide nano-particles (nZnO) prompt the use of these nanomaterials in several hydrogel-based wound dressings.Citation209 Cerium oxide nanoparticle incorporated electrospun membranes for diabetic wound healing.Citation210 However, despite the high potential of metallic nanoparticles in treating drug-resistant bacteria, the high toxicity of these materials limits their use in wound healing.Citation211 In addition, Gao et al have reported a new near-infrared 808nm laser-mediated nitric oxide-releasing nanovehicle (MoS2-BNN6), MoS2-BNN6 can effectively inhibit the growth of ampicillin-resistant Escherichia coli, heat-resistant Escherichia faecalis, and pathogen Staphylococcus aureus.Citation212 Zhao et al used bovine serum albumin stabilized-CuS (BSA-CuS) NPs to propose that PTT could kill bacteria in the field of diabetic wound infectionCitation213 (). See for detailed description of metal ion nanoparticles.
Figure 6 Schematic diagram of BSA-CuS antibacterial therapy. Reprinted with permission from Zhao Y, Cai Q, Qi W, et al. BSA-CuS nanoparticles for photother- mal therapy of diabetic wound infection in vivo. Biol Chem ChemBiol. 2018;3:9510–9516. Copyright 2018, John Wiley and Sons.Citation213 (A) HRTEM image of the BSA-CuS nanoparticles. (B) Schematic illustration of BSA-CuS nanoparticles as photothermal agent for photothermal antibacterial therapy in vitro and in vivo.
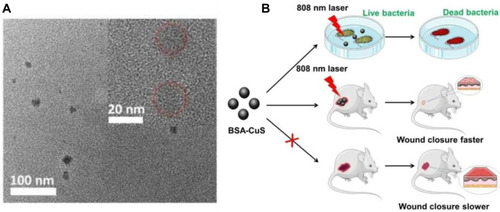
Nonmetallic Nanomaterials
A recent study reported that the use of graphene oxide (rGO)-isabgol nanocomposite dressings for enhanced vascularization and accelerated wound healing in normal and diabetic rats.Citation214 Furthermore, a study has shown that the different adhesion and bioactivity properties of GO can prevent bacterial adhesion and biofilm formation. Therefore, there is a growing interest in studying the potential of graphene-based materials in biomedical applications, such as drug delivery, tissue engineering, imaging, biosensing and wound healing.Citation125,Citation215,Citation216 Porous silicon (pSi) is a biological material widely used in vivo and in vitro.Citation217 pSi has the ability to store and release a variety of small molecular drugs, oligonucleotides, and even protein therapeutics.Citation218,Citation219 The use of porous silicon nanoparticles (pSi NPs) is demonstrated for the controlled release of Flii neutralizing antibodies (FnAb) to diabetic wounds.Citation220 The use of nanotherapeutic drugs alone may cause rapid degradation and cannot reach the target tissue quickly and effectively, thus reducing the biological effect.Citation58 Xie et al used a dual-growth factor releasing nanoparticle-in-nanofiber system, encapsulated platelet derived growth factor in NP, embedded into VEGF nanofiber, and delivered VEGF quickly and PDGF in a relayed manner. Nanofiber/nanoparticle scaffolds significantly accelerate wound healing by promoting angiogenesis, increasing reepithelialization, and controlling granulation tissue formation.Citation221
Naturally occurring polymers, such as chitosan nanoparticles, have been studied for their antibacterial activity and pro-wound healing properties.Citation222,Citation223 Correa et al reported a melatonin loaded lecithin-chitosan nanoparticles improved the wound healing in diabetic rats.Citation224 Chitosan composites usually exhibit unique properties that are not individually displayed by chitosan or the incorporated materials.Citation175,Citation225
Currently, nanocarriers have been validated in vitro and offered potential therapeutic applications that require to be further tested in vivo. At the same time, several nano-therapeutic agents are used in combination with NPs (loaded copper, which is helpful for wound healing) to provide synergistic effect and accelerate wound healing.
Smart Hydrogels
Hydrogels are considered to be three-dimensional nanofiber materials composed of cross-linked hydrophilic polymer networks.Citation46 Due to the presence of chemical or physical cross-links, they are able to swell and retain large amounts of water, and preserving their structural and dimensional constrained integrity.Citation47 Hydrogels are biocompatible and biodegradable materials and have been used in cell therapy, drug delivery, biosensing, tissue engineering and wound healingCitation226–Citation229 ().
Table 8 To Summarize the Role of Multifunctional Hydrogel in Diabetic Wound Healing
Injectable Hydrogels
In recent years, injectable hydrogels have favored among researchers due to its nonsurgical treatment to the patients for the purpose of mini-invasive medicine, especially for deep, irregular injuries.Citation230,Citation231 To enable injection, most in situ forming hydrogels are delivered in a liquid form that will subsequently solidify in the body.Citation232,Citation233 Typically, the injected precursor gel solution forms a hydrogel via chemical (eg, michael-type addition reaction, disulfide bond formation, click chemistry, radical polymerization) or physical (eg, ionic interactions, hydrogen bonding, hydrophobic interactions) crosslinking.Citation234,Citation235 Chen et al developed injectable self-healing and antibacterial hydrogel, the multi-functional hydrogel featured manageable, resistant to mechanical irritation, antibacterial and angiogenic properties. Hydrogel would show great promise in the physiological dysfunction and bacterial infection wounds.Citation236 However, further studies are needed on the release and cytotoxicity of silver ions. Wang et al developed multifunctional hydrogel (injectable, self-healing, and adhesive) that simultaneously eliminated MRSA infection, reduced hyperglycemia, improved oxidative stress, and continuously provided oxygen.Citation237 In addition, Kong et al loaded desferrioxamine and bioglass into injectable sodium alginate hydrogel to synergistic promote diabetic wound healing.Citation135
There have been many reports on wound dressing with bioactive/non-bioactive substances (growth factors, stem cells/exosomes and oxygen, etc.) for diabetic wound, but few studies have considered the specific physiological environment (such as acidic pH, ROS and high glucose levels) of diabetic wounds. Li et al reported a pH and glucose dual-responsive injectable hydrogel by in situ crosslinking of modified chitosan and oxidized dextran, and then Zhao et al incorporated insulin and fibroblasts into the hydrogel, which could not only respond to pH and glucose, but also promote wound healing in diabetic wounds.Citation137,Citation238 Zhu et al used zwitterionic hydrogel to monitor pH value and glucose concentration in diabetic wounds, and these two parameters are converted into visible images, which were collected by smartphones and monitor changes in wounds at any timeCitation239 (). This multifunctional wound dressing may open vistas in chronic wound management and guide the diabetes treatment in clinical applications.
Figure 7 Schematic diagram of hydrogel synthesis. (A) Scheme of poly-carboxybetaine (PCB) hydrogel dressing for the detection of pH value and glucose concentration in wound exudate. Reprinted with permission from Zhu YN, Zhang JM, Song JY, et al. A multifunctional pro-healingzwitterionic hydrogel for simultaneous optical monitoring of pH and glucose in diabetic wound treatment. Adv Funct Mater.2019:1905493. Copyright 2019, John Wiley and Sons.Citation239 (B) The formation of OxOBand from PUAO-CPO cryogels with ADSC-exos. Reprinted with permission from Shiekh PA, Singh A, Kumar A. Exosome laden oxygen releasing antioxidant and antibacterial cryogel wound dressing OxOBandalleviate diabetic and infectious wound healing. Biomaterials.2020;249:120020. Copyright 2020, Elsevier.Citation181
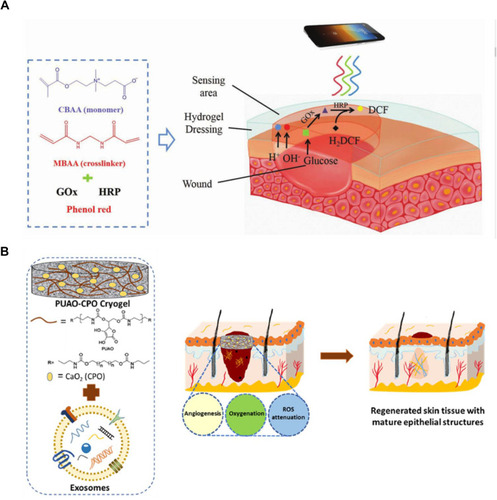
Conductive Hydrogels
In recent years, conductive hydrogels have also been widely used in health recording electrodes, biomedical patches, wearable/implantable bio-devices, and electronic skin.Citation240–Citation244 Conductive hydrogels are stimulated by external electrical signals, which are converted to bioelectrical stimulation after reaching the skin to achieve the purpose of treatment. Zhao et al developed a conductive hydrogels (good self-healing ability as well as repeatable adhesiveness), which can promote angiogenesis, collagen deposition, inhibit bacterial growth and control diabetic wound closure.Citation151 Zhang et al designed conductive hydrogels based on polyvinyl alcohol and chitosan, the conductivity can enable hydrogels to perceive temperature and strain.Citation245 The hydrogels are expected to build flexible sensory systems and the next generation of intelligent biomedical products in the future.Citation245 Researchers are now focusing on developing smarter hydrogels that not only contain “sensor” moieties that can respond to wound environmental pH, ROS levels, glucose concentrations, etc., but also are easy to operate and safe, this smart drug delivery system can promote diabetic wound healing.Citation246,Citation247
Although significant progress has been made in injectable hydrogels, more research is needed to address some of the current technical challenges. A major limitation of injectable gels is the rapid release of low molecular weight compounds such as drugs and biomolecules. One way to slow down the kinetics of drug release is to hybrid the hydrogel with drug-loaded nanoparticles. Furthermore, the interaction between cells-matrix should be enhanced. All of these methods in combination with 3D cryoprinting. Additionally, the size of injectable hydrogels could be a barrier when moving from preclinical studies into clinical practice, where larger scaffolds are often required for humans. In the future, designing more compressible or self-healing injectable intelligent hydrogels should broaden their biomedical applications and accelerate their clinical translatability.
Other technology
Currently, various technologies, especially multifunctional systems (including photothermal therapy (PTT), layer-by-layer (LBL) self-assembly technique and 3D-printing technology), are widely used in diabetic wound repair.Citation248–Citation251
Photothermal Therapy
PTT is based on near infrared (NIR) laser triggered therapy, widely used in cancer treatment. It combines near-infrared laser and light-absorbing nanomaterials to achieve local high temperature around NPs, leading to cancer cell death. Huang et al synthesized BSA-CuS nanoparticles by biomineralization method of bovine serum albumin (BSA) and copper sulfide (CuS), it showed strong killing bacterial ability under NIR.Citation213,Citation252 PB@PDA@Ag NPs can accelerate the healing of diabetic wounds under NIR.Citation150 In addition, MoS2-BNN6 can effectively inhibit the growth of ampicillin-resistant Escherichia coli, heat-resistant Escherichia faecalis, and pathogen Staphylococcus aureus.Citation212 Although nanoparticles based PTT has great potential for treating diabetic wound infections, local heat can also severely damage surrounding healthy tissue, so precise research and specific clinical trials are needed for PTT therapy.
Layer-by-Layer Self-Assembly Technique
Some biomaterials can improve their biomedical properties through many simple methods, such as layer-by-layer (LBL) self-assembly technique.Citation253 LBL self-assembly technique is widely used in biomedical for delivery from a broad range of material surfaces.Citation254 And LBL modified composite material has good stability, mechanical properties and hydrophilicity.Citation255,Citation256 LBL self-assembly technique is favored by many people because it can alternately deposit the electrostatic force with opposite charge on the surface of polyelectrolyte matrix, improving the continuous release of drugs, and is easy to operate, controllable and economical without potential complications.Citation257 Natural rubber latex (NRL) can be used to treat chronic skin wounds, but because of their low integration, most applications of NRL biomembranes are external, short-term implants, or as delivery matrices.Citation258,Citation259 Davi et al can increase the membrane formation speed by 10 times by spraying LBL technology.Citation260 In addition, self-assembled nanometer-scale coatings can incorporate and release therapeutically relevant quantities of siRNA in a controlled fashion to yield rapid diabetic wound closureCitation79 (). Thus, the use of LBL to alter localized protein expression levels has significant implications for the treatment of site-specific diseases, including cardiovascular disease, DFUs, cancers, and transplant rejection.
Figure 8 Schematic diagram of material synthesis. (A) Schematic of the hierarchical structure of LbL films into a single coating. The first (X) film is a hydrolytically degradable undercoating, while the second (Y) film contains the siRNA to be delivered. (B) Side-on schematic of hierarchical LBL film architecture. Reprinted with permission from Castleberry SA, Almquist BD, Li W, et al. Self-assembled wounddressings silenceMMP-9 and improve diabetic wound healing in vivo. Adv Mater. 2016;28:1809–1817. Copyright 2016, John Wiley and Sons.Citation79 (C) Schematic illustration of the synthesis procedure and rESW-responsive oxygen release from Nano-PFC. Adapted from Wang S, Yin C, Han X, et al. Improved healing of diabetic footulcer upon oxygenation therapeutics through oxygen-loading nanoperfluorocarbontriggered by radial extracorporeal shock wave. Oxid Med Cell Longev. 2019;2019:5738368. Creative Commons license and disclaimer available from: http://creativecommons.org/licenses/by/4.0/legalcode.Citation189
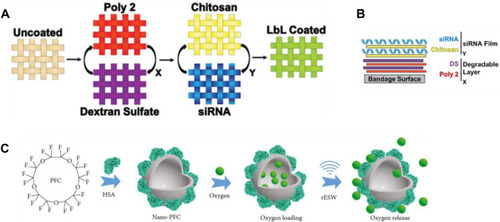
3D-Printed
3D-printed scaffolds for wound dressings have many advantages, such as the ability to adjust the dimensional characteristics of wound dressings (such as area, thickness, or pore size), simple drug loading, the use of a variety of materials, and oxygen penetration due to pore design.Citation261 Sodium alginate/polyethylene glycol (SA/PEG) scaffolds were prepared by adding different concentrations (1, 3 and 5 wt.%) of PEG to SA using 3D-printing technology. 3D-printing scaffolds had good antibacterial effect, especially against gram-positive bacteria. In addition, using 3D-printing technology, ideal porosity and properties were obtained, enabling cells to grow on/within the scaffold.Citation262 Wu et al used extrusion-based 3D printing and post stretching to fabricate a microneedle patch system for minimally invasive and glucose-responsive insulin delivery for diabetes treatment.Citation263
Conclusions and Perspectives
The treatment of diabetic wounds is complex and challenging due to its pathophysiology, resulting in impaired function of different cells and in unbalanced levels of key biochemical healing mediators. Based on the characteristics of diabetic wounds and the mechanism of tissue repair, nanoparticles/hydrogels loaded with bioactive molecules (such as growth factors, genes, proteins/peptides, stem cells/exosomes) and non-bioactive substances (metal ions, oxygen and nitric oxide), as well as nanotechnology (eg, PTT, LBL self-assembly technique and 3D printing) have been applied to diabetic wound healing (). The etiopathogenesis of diabetic ulcers is too complex, one or two substances are not enough to accelerate wound healing, so a variety of substances can be combined to release in different stages of wounds to accelerate diabetic wound healing. Overall, the future direction may be the development of new biomaterials with multiple roles (including improve hypoxia, enhance angiogenesis, reduce oxidative stress and prevent infection) that may regulate wound healing at all stages and provide a balanced environment throughout the wound healing process, thereby reducing potential complications.
Figure 9 Potential therapies for diabetic wound repair. Strategies for manipulating the regeneration of diabetic wounds include the use of hydrogels (loaded with small molecules and stem cells, etc.), photothermal therapy, and materials that release oxygen. All of these elements have been demonstrated to have an effect on in vitro and in vivo models of wound healing. These repair mechanisms include vascularization, less ROS production, oxygen release, and antimicrobial resistance. Therefore, combining these strategies will undoubtedly change the result of diabetic wound healing.
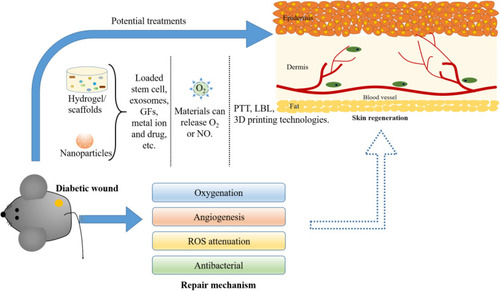
In recent years, people are interested in using various technologies to prepare some multifunctional nano-systems for diabetic wound healing. However, enough information about the physicochemical properties of nanoscale systems and their expected behavior and toxicity in human body remains unclear. In the long term, further studies are indispensable to provide insights into how research findings about technology-based therapies can be applied in the clinical arena. In the future, we are sure to design exciting intelligent nanotechnology platforms for the diagnosis and treatment of various chronic diseases.
Abbreviations
IL-6, Interleukin 6; TGF- β1, Transforming growth factor-β1; NGF, Nerve growth factor; KGF, Keratinocyte growth factor; VEGF, Vascular endothelial growth factor; IGF-1, Insulin-like growth factor; bFGF, basic Fibroblast growth factors b; AGE, Advanced glycation end-product; GF, Growth factor; LBL, Layer-by-layer; NO, Nitric oxide; NOS, Nitric oxide synthase; ECM, Extracellular matrix; MMP, Matrix metalloproteinases; TIMP, Tissue inhibitors of metalloproteinases; TNFα, Tumour necrosis factor α; GAG, Glycosaminoglycan; SDF-1, Stromal cell-derived factor 1; MIP-3α, Macrophage inflammatory protein-3α; DFO, Desferrioxamine; PB, Prussian blue; NP, Nanoparticle; ADSCs, Adipose derived stem cells; GSH, Reduced glutathione; PTT, Photothermal therapy; HIF-1α, Hypoxia-inducible factor 1α; MΦ, Macrophages; MRSA, Methicillin-resistant staphylococcus aureus; BG, Bioglass; nano-PFC, Nanoperfluorocarbon.
Acknowledgments
We acknowledge funding by the Innovation Capability Support Program of Shanxi (Program No. 2020TD-042), Jointly funded by Department of Science and Technology of Shaanxi Province and Northwestern Polytechnical University (No. 2020GXLH-Z-021), the National Natural Science Foundation of China (No. 81803491), the Natural Science Foundation of Shaanxi Province (No. 2019JQ-320), and the Fundamental Research Funds for the Central Universities (No. 3102019smxy002). We would like to thank the Analytical & Testing Center of Northwestern Polytechnical University for supporting our research on diabetic wound repair materials.
Disclosure
The authors declare that there are no competing interests.
References
- Shaw JE, Sicree RA, Zimmet PZ. Global estimates of the prevalence of diabetes for 2010 and 2030. Diabetes Res Clin Pract. 2010;87:4–14. doi:10.1016/j.diabres.2009.10.00719896746
- Wang X, Sng MK, Foo S, et al. Early controlled release of peroxisome proliferator-activated receptor beta/delta agonist GW501516 improves diabetic wound healing through redox modulation of wound microenvironment. J Control Release. 2015;197:138–147. doi:10.1016/j.jconrel.2014.11.00125449811
- Ahmed N. Advanced glycation endproducts—role in pathology of diabetic complications. Diabetes Res Clin Pract. 2005;67:3–21. doi:10.1016/j.diabres.2004.09.00415620429
- McCance DR. Pregnancy and diabetes. Best Pract Res Clin Endocrinol Metab. 2011;25:945–958. doi:10.1016/j.beem.2011.07.00922115168
- Mohammedi K, Maimaitiming S, Emery N, et al. Allelic variations in superoxide dismutase-1 (SOD1) gene are associated with increased risk of diabetic nephropathy in type 1 diabetic subjects. Mol Genet Metab. 2011;104(4):654–660. doi:10.1016/j.ymgme.2011.08.03321963083
- Zhang S, Zhang Y, Wei X, et al. Expression and regulation of a novel identified TNFAIP8 family is associated with diabetic nephropathy. BBA Mol Basis Dis. 2010;1802:1078–1086. doi:10.1016/j.bbadis.2010.08.003
- Kim BY, Kim CH, Jung CH, et al. Association between subclinical hypothyroidism and severe diabetic retinopathy in Korean patients with type 2 diabetes. Endocr J. 2011;58:1065–1070. doi:10.1507/endocrj.EJ11-028121931224
- Silva L, Carvalho E, Cruz MT. Role of neuropeptides in skin inflammation and its involvement in diabetic wound healing. Expert Opin Biol Ther. 2010;10:1427–1439. doi:10.1517/14712598.2010.51520720738210
- Kim SK, Lee KJ, Hahm JR, et al. Clinical significance of the presence of autonomic and vestibular dysfunction in diabetic patients with peripheral neuropathy. Diab Metab J. 2012;36:64–69. doi:10.4093/dmj.2012.36.1.64
- Sunkari VG, Lind F, Botusan IR, et al. Hyperbaric oxygen therapy activates hypoxia-inducible factor 1 (HIF-1), which contributes to improved wound healing in diabetic mice. Wound Repair Regen. 2015;23:98–103. doi:10.1111/wrr.1225325532619
- Zamboni F, Collins MN. Cell based therapeutics in type 1 diabetes mellitus. Int J Pharm. 2017;521(1–2):346–356. doi:10.1016/j.ijpharm.2017.02.06328242376
- Cahill D, Zamboni F, Collins MN. Radiological advances in pancreatic islet transplantation. Acad Radiol. 2019;26:1536–1543. doi:10.1016/j.acra.2019.01.00630709732
- Sun YX, Shi H, Yin SQ, et al. Human medenchymal stem cell derived exosomes alleviate type 2 diabetes mellitus by reversing peripheral insulin resistance and relieving β-cell destruction. ACS Nano. 2018;12:7613–7628. doi:10.1021/acsnano.7b0764330052036
- Patel P, Macerollo A. Diabetes mellitus: diagnosis and screening. Diabetes. 2010;107:213–240. doi:10.3238/arztebl.2010.0231
- Vijayakumar V, Samal SK, Mohanty S, et al. Recent advancements in biopolymer and metal nanoparticle-based materials in diabetic wound healing management. Int J Biol Macromol. 2019;122:137–148. doi:10.1016/j.ijbiomac.2018.10.12030342131
- Gurtner GC, Werner S, Barrandon Y, et al. Wound repair and regeneration. Nature. 2008;453:314–321. doi:10.1038/nature0703918480812
- Schreml S, Szeimies RM, Prantl L, et al. Oxygen in acute and chronic wound healing. Br J Dermatol. 2010:1–12. doi:10.1111/j.1365-2133.2010.09804.x
- Broughton GII, Janis JE, Attinger CE. Wound healing: an overview. Plast Reconstr Surg. 2006;117:1e-S-32e-S. doi:10.1097/01.prs.0000222562.60260.f9
- Robson MC, Steed DL, Franz MG. Wound healing: biologic features and approaches to maximize healing trajectories. Curr Probl Surg. 2016;38(2):72–140. doi:10.1067/msg.2001.111167
- Chellappan DK, Yenese Y, Wei CC, et al. Nanotechnology and diabetic wound healing: a review. Endocr Metab Immune Disord Drug Targets. 2017;17:87–95. doi:10.2174/187153031766617042112120228427246
- Falanga V. Wound healing and its impairment in the diabetic foot. Lancet. 2005;366:1736–1743. doi:10.1007/978-1-62703-505-7_616291068
- Delavary BM, Veer WMVD, Egmond MV, et al. Macrophages in skin injury and repair. Immunobiology. 2011;216(7):753–762. doi:10.1016/j.imbio.2011.01.00121281986
- Martin P, Leibovich SJ. Inflammatory cells during wound repair: the good, the bad and the ugly. Trends Cell Biol. 2005;15(11):599–607. doi:10.1016/j.tcb.2005.09.00216202600
- Guo SA, DiPietro LA. Factors affecting wound healing. J Dent Res. 2010;89(3):219–229. doi:10.1177/002203450935912520139336
- O’Toole EA. Extracellular matrix and keratinocyte migration. Clin Exp Dermatol. 2001;26(6):525–530. doi:10.1046/j.1365-2230.2001.00891.x11678882
- Hinz B. Formation and function of the myofibroblast during tissue repair. J Investig Dermatol. 2007;127(3):526–537. doi:10.1038/sj.jid.570061317299435
- Reinke JM, Sorg H. Wound repair and regeneration. Eur Surg Res. 2012;49:35–43. doi:10.1159/00033961322797712
- Gonzalez AC, Costa TF, Andrade ZA, et al. Wound healing – a literature review. An Bras Dermatol. 2016;91:614–620. doi:10.1590/abd1806-4841.2016474127828635
- Lazarus GS, Cooper DM, Knighton DR, et al. Definitions and guidelines for assessment of wounds and evaluation of healing. Wound Repair Regen. 1994;2:165–170. doi:10.1046/j.1524-475X.1994.20305.x17156107
- Desmeta CM, Préat V, Gallez B. Nanomedicines and gene therapy for the delivery of growth factors to improve perfusion and oxygenation in wound healing. Adv Drug Deliv Rev. 2018;129:262–284. doi:10.1016/j.addr.2018.02.00129448035
- Choudhury H, Pandey M, Lim YQ, et al. Silver nanoparticles: advanced and promising technology in diabetic wound therapy. Mater Sci Eng C. 2020;112:11092. doi:10.1016/j.msec.2020.110925
- Woo K, Ayello EA, Sibbald RG. The edge effect: current therapeutic options to advance the wound edge. Adv Skin Wound Care. 2007;20:99–117. doi:10.1097/00129334-200702000-0000917287621
- Eming SA, Krieg T, Davidson JM. Inflammation in wound repair: molecular and cellular mechanisms. J Invest Dermatol. 2007;127:514–525. doi:10.1038/sj.jid.570070117299434
- Margadant C, Sonnenberg A. Integrin–TGF-β crosstalk in fibrosis, cancer and wound healing. EMBO Reports. 2010;11(2):97–105. doi:10.1038/embor.2009.27620075988
- Yang L, Zheng Z, Zhou Q, et al. miR-155 promotes cutaneous wound healing through enhanced keratinocytes migration by MMP-2. J Mol Histol. 2017;48:147–155. doi:10.1007/s10735-017-9713-828247149
- Prabhakar PK, Singh K, Kabra D, et al. Natural SIRT1 modifiers as promising therapeutic agents for improving diabetic wound healing. Phytomedicine. 2020;76:153252. doi:10.1016/j.phymed.2020.15325232505916
- Thangarajah H, Yao D, Chang EI, et al. The molecular basis for impaired hypoxia-induced VEGF expression in diabetic tissues. PNAS. 2009;106:13505–13510. doi:10.1073/pnas.090667010619666581
- Schaper NC, Van Netten JJ, Apelqvist J, et al. Prevention and management of foot problems in diabetes: a summary guidance for daily practice 2015, based on the IWGDF guidance documents. Diabetes/Metab Res Rev. 2016;32:7–15. doi:10.1016/j.diabres.2016.12.007
- Ezhilarasu H, Vishalli D, Dheen ST, et al. Nanoparticle-based therapeutic approach for diabetic wound healing. Nanomaterials. 2020;10:1234. doi:10.3390/nano10061234
- Chen L, Zheng Q, Liu YP, et al. Adipose-derived stem cells promote diabetic wound healing via the recruitment and differentiation of endothelial progenitor cells into endothelial cells mediated by the VEGF-PLC gamma-ERK pathway. Arch Biochem Biophys. 2020;692:108531. doi:10.1016/j.abb.2020.10853132745464
- Armstrong DG, Boulton AJ, Bus SA. Diabetic foot ulcers and their recurrence. N Engl J Med. 2017;376:2367–2375. doi:10.1056/NEJMra161543928614678
- Dinh T, Tecilazich F, Kafanas A, et al. Mechanisms involved in the development and healing of diabetic foot ulceration. Diabetes. 2012;61:2937–2947. doi:10.2337/db12-022722688339
- Charo IF, Ransohoff RM. The many roles of chemokines and chemokine receptors in inflammation. N Engl J Med. 2006;354:610–621. doi:10.1056/NEJMra05272316467548
- Rosenkilde MM, Schwartz TW. The chemokine system – a major regulator of angiogenesis in health and disease. APMIS. 2004;112:481–495. doi:10.1111/j.1600-0463.2004.apm11207-0808.x15563311
- Jiang BC, Liu T, Gao YJ. Chemokines in chronic pain: cellular and molecular mechanisms and therapeutic potential. Pharmacol Ther. 2020;212:107581. doi:10.1016/j.pharmthera.2020.10758132450191
- Peppas NA, Hilt JZ, Khademhosseini A, et al. Hydrogels in biology and medicine: from molecular principles to bionanotechnology. Adv Mater. 2006;18(11):1345–1360. doi:10.1002/adma.200501612
- Chai QY, Jiao Y, Yu XJ. Hydrogels for biomedical applications: their characteristics and the mechanisms behind them. Gels. 2017;3(1):6. doi:10.3390/gels3010006
- Lee Y, Bae JW, Lee JW, et al. Enzyme-catalyzed in situ forming gelatin hydrogels as bioactive wound dressings: effects of fibroblast delivery on wound healing efficacy. J Mater Chem B. 2014;2:7712–7718. doi:10.1039/c4tb01111b32261906
- Lee SH, Lee Y, Chun YW, et al. In situ crosslinkable gelatin hydrogels for vasculogenic induction and delivery of mesenchymal stem cells. Adv Funct Mater. 2014;24:6771–6781. doi:10.1002/adfm.20140111026327818
- Dong SY, Lee YK, Ryu HA, et al. Cell recruiting chemokine-loaded sprayable gelatin hydrogel dressings for diabetic wound healing. Acta Biomater. 2016;38:59–68. doi:10.1016/j.actbio.2016.04.03027109762
- Greenhalgh DG. The role of growth factors in wound healing. J Trauma. 1996;41:159–167. doi:10.1097/00005373-199607000-000298676414
- Badillo AT, Chung S, Zhang L, et al. Lentiviral gene transfer of SDF-1alpha to wounds improves diabetic wound healing. J Surg Res. 2007;143:35–42. doi:10.1016/j.jss.2007.03.05117950070
- Gainza G, Villullas S, Pedraz JL, et al. Advances in drug delivery systems (DDSs) to release growth factors for wound healing and skin regeneration. Nanomedicine. 2015;11:1551–1573. doi:10.1016/j.nano.2015.03.00225804415
- Rui L, Yiyang L, Yanqing W, et al. Heparin-poloxamer thermosensitive hydrogel loaded with bFGF and NGF enhances peripheral nerve regeneration in diabetic rats. Biomaterials. 2018;168:24–37. doi:10.1016/j.biomaterials.2018.03.04429609091
- Zhu Y, Hoshi R, Chen S, et al. Sustained release of stromal cell derived factor-1 from an antioxidant thermoresponsive hydrogel enhances dermal wound healing in diabetes. J Control Release. 2016;238:114–122. doi:10.1016/j.jconrel.2016.07.04327473766
- Chereddy KK, Lopes A, Koussoroplis S, et al. Combined effects of PLGA and vascular endothelial growth factor promote the healing of non-diabetic and diabetic wounds. Nanomedicine: NBM. 2015;11:1975–1984. doi:10.1016/j.nano.2015.07.006
- Hui Q, Zhang L, Yang XX, et al. Higher biostability of rh-aFGF-carbomer 940 hydrogel and its effect on wound healing in a diabetic rat model. ACS Biomater Sci Eng. 2018;4:1661–1668. doi:10.1021/acsbiomaterials.8b00011
- Losi P, Briganti E, Errico C, et al. Fibrin-based scaffold incorporating VEGF- and bFGF-loaded nanoparticles stimulates wound healing in diabetic mice. Acta Biomater. 2013;9:7814–7821. doi:10.1016/j.actbio.2013.04.01923603001
- Lai HJ, Kuan CH, Wu HC, et al. Tailor design electrospun composite nanofibers with staged release of multiple angiogenic growth factors for chronic wound healing. Acta Biomater. 2014;10:4156–4166. doi:10.1016/j.actbio.2014.05.00124814882
- Lee CH, Liu KS, Cheng CW, et al. Co-delivery of sustainable anti-microbial agents and platelet-derived growth factor via biodegradable nanofibers for repair of diabetic infectious wounds. ACS Infect Dis. 2020. doi:10.1021/acsinfecdis.0c00321
- Zhu JY, Jiang GH, Hong WJ, et al. Rapid gelation of oxidized hyaluronic acid and succinyl chitosan for integration with insulin-loaded micelles and epidermal growth factor on diabetic wound healing. Mater Sci Eng C. 2020;117:111273. doi:10.1016/j.msec.2020.111273
- Nissen NN, Polverini PJ, Koch AE, et al. Vascular endothelial growth factor mediates angiogenic activity during the proliferative phase of wound healing. Am J Pathol. 1998;152:1445–1452. doi:10.1097/00000433-199806000-000229626049
- Brown LF, Yeo KT, Berse B, et al. Expression of vascular permeability factor (vascular endothelial growth factor) by epidermal keratinocytes during wound healing. J Exp Med. 1992;176:1375–1379. doi:10.1084/jem.176.5.13751402682
- Peters KG, De Vries C, Williams LT. Vascular endothelial growth factor receptor expression during embryogenesis and tissue repair suggests a role in endothelial differentiation and blood vessel growth. Proc Natl Acad Sci. 1993;90:8915–8919. doi:10.1073/pnas.90.19.89157692439
- Galiano RD, Tepper OM, Pelo CR, et al. Topical vascular endothelial growth factor accelerates diabetic wound healing through increased angiogenesis and by mobilizing and recruiting bone marrow-derived cells. Am J Pathol. 2004;164:1935–1947. doi:10.1016/S0002-9440(10)63754-615161630
- Guo R, Xu S, Ma L, et al. Enhanced angiogenesis of gene-activated dermal equivalent for treatment of full thickness incisional wounds in a porcine model. Biomaterials. 2010;31:7308–7320. doi:10.1016/j.biomaterials.2010.06.01320598366
- Guo R, Xu S, Ma L, et al. The healing of full-thickness burns treated by using plasmid DNA encoding VEGF-165 activated collagen-chitosan dermal equivalents. Biomaterials. 2011;32:1019–1031. doi:10.1016/j.biomaterials.2010.08.08721071076
- Tokatlian T, Cam C, Segura T. Non-viral DNA delivery from porous hyaluronic acid hydrogels in mice. Biomaterials. 2014;35:825–835. doi:10.1016/j.biomaterials.2013.10.01424210142
- Prestwich GD. Clinical biomaterials for scar-free healing and localized delivery of cells and growth factors. Adv Wound Care. 2010;1:394–399. doi:10.1089/awc.2009.0124
- Tokatlian T, Cam C, Segura T. Porous hyaluronic acid hydrogels for localized nonviral DNA delivery in a diabetic wound healing model. Adv Healthcare Mater. 2015;4:1084–1091. doi:10.1002/adhm.201400783
- Devalliere J, Dooley K, Hu Y, et al. Co-delivery of a growth factor and a tissue-protective molecule using elastin biopolymers accelerates wound healing in diabetic mice. Biomaterials. 2017;141:149–160. doi:10.1016/j.biomaterials.2017.06.04328688286
- Kempen DHR, Lu L, Heijink A, et al. Effect of local sequential VEGF and BMP-2 delivery on ectopic and orthotopic bone regeneration. Biomaterials. 2009;30:2816–2825. doi:10.1016/j.biomaterials.2009.01.03119232714
- Roberts JJ, Farrugia BL, Green RA, et al. In situ formation of poly(vinyl alcohol)-heparin hydrogels for mild encapsulation and prolonged release of basic fibroblast growth factor and vascular endothelial growth factor. J Tissue Eng. 2016;7:1–10. doi:10.1177/2041731416677132
- Kano MR, Morishita Y, Iwata C, et al. VEGF-A and FGF-2 synergistically promote neoangiogenesis through enhancement of endogenous PDGF-B-PDGFRbeta signaling. J Cell Sci. 2005;118:3759–3768. doi:10.1242/jcs.0248316105884
- Jiang X, Lin H, Jiang D, et al. Co-delivery of VEGF and bFGF via a PLGA nanoparticle-modified BAM for effective contracture inhibition of regenerated bladder tissue in rabbits. Sci Rep. 2016;6:20784. doi:10.1038/srep2078426854200
- Icli B, Nabzdyk CS, Lujan-Hernandez J, et al. Regulation of impaired angiogenesis in diabetic dermal wound healing by microRNA-26a. J Mol Cell Cardiol. 2016;91:151–159. doi:10.1016/j.yjmcc.2016.01.00726776318
- Wu H, Li F, Shao W, et al. Promoting angiogenesis in oxidative diabetic wound microenvironment using a nanozyme-reinforced self-protecting hydrogel. ACS Cent Sci. 2019;5:477–485. doi:10.1021/acscentsci.8b0085030937375
- Li N, Luo HC, Ren M, et al. Efficiency and safety of β‑CD-(D3)7 as siRNA carrier for decreasing matrix metalloproteinase-9 expression and improving wound healing in diabetic rats. ACS Appl Mater Interfaces. 2017;9:17417–17426. doi:10.1021/acsami.7b0280928447455
- Castleberry SA, Almquist BD, Li W, et al. Self-assembled wound dressings silence MMP-9 and improve diabetic wound healing in vivo. Adv Mater. 2016;28:1809–1817. doi:10.1002/adma.20150356526695434
- Lou D, Luo Y, Pang Q, et al. Gene-activated dermal equivalents to accelerate healing of diabetic chronic wounds by regulating inflammation and promoting angiogenesis. Bioact Mater. 2020;5:667–679. doi:10.1016/j.bioactmat.2020.04.01832420517
- Rabbani PS, Zhou A, Borab ZM, et al. Novel lipoproteoplex delivers Keap1 siRNA based gene therapy to accelerate diabetic wound healing. Biomaterials. 2017;132:1–15. doi:10.1016/j.biomaterials.2017.04.00128391065
- Turner N, Grose R. Fibroblast growth factor signalling: from development to cancer. Nat Rev Cancer. 2010;10:116–129. doi:10.1038/nrc278020094046
- Chen J, Wu C, Oupicky D. Bioreducible hyperbranched poly(amido amine)s for gene delivery. Biomacromolecules. 2009;10:2921–2927. doi:10.1021/bm900724c19743843
- Senturk B, Mercan S, Delibasi T, et al. Angiogenic peptide nanofibers improve wound healing in STZ-induced diabetic rats. ACS Biomater Sci Eng. 2016;2:1180–1189. doi:10.1021/acsbiomaterials.6b00238
- Wang JH, Chen XY, Zhao Y, et al. pH-switchable antimicrobial nanofiber networks of hydrogel eradicate biofilm and rescue stalled healing in chronic wounds. ACS NANO. 2019;10:11686–11697. doi:10.1021/acsnano.9b05608
- Zhang QK, Oh JH, Park CH, et al. Effects of dimethyloxalylglycine-embedded poly(ε-caprolactone) fiber meshes on wound healing in diabetic rats. ACS Appl Mater Interfaces. 2017;9:7950–7963. doi:10.1021/acsami.6b1581528211272
- Cifuentes A, Gomez-Gil V, Ortega MA, et al. Chitosan hydrogels functionalized with either unfractionated heparin or bemiparin improve diabetic wound healing. Biomed Pharmacother. 2020;129:110498. doi:10.1016/j.biopha.2020.11049832768973
- Lin SY, Zhang Q, Li SH, et al. Antioxidative and angiogenesis-promoting effects of tetrahedral framework nucleic acids in diabetic wound healing with activation of the Akt/Nrf2/HO-1 pathway. ACS Appl Mater Interfaces. 2020;12:11397–11408. doi:10.1021/acsami.0c0087432083455
- Chen SX, Zhang M, Shao XB, et al. Laminin mimetic peptide SIKVAV-chitosan hydrogel promoting wound healing by enhancing angiogenesis, re-epithelialization and collagen deposition. J Mater Chem B. 2015;3:6798–6804. doi:10.1039/c5tb00842e32262473
- Peng ZH, Nguyen TT, Song W, et al. Selective MMP‑9 inhibitor (R)‑ND-336 alone or in combination with linezolid accelerates wound healing in infected diabetic mice. ACS Pharmacol Transl Sci. 2020. doi:10.1021/acsptsci.0c00104
- Carrejo NC, Moore AN, Silva TLL, et al. Hartgerink, multidomain peptide hydrogel accelerates healing of full-thickness wounds in diabetic mice. ACS Biomater Sci Eng. 2018;4:1386–1396. doi:10.1021/acsbiomaterials.8b0003129687080
- Yang HS, Lai C, Xuan CK, et al. Integrin-binding pro-survival peptide engineered silk fibroin nanosheets for diabetic wound healing and skin regeneration. Chem Eng J. 2020;398:125617. doi:10.1016/j.cej.2020.125617
- Chouhan D, Das P, Thatikonda N, et al. Silkworm silk matrices coated with functionalized spider silk accelerate healing of diabetic wounds. ACS Biomater Sci Eng. 2019;5:3537–3548. doi:10.1021/acsbiomaterials.9b00514
- Mizuno H, Tobita M, Uysal AC. Concise review: adipose-derived stem cells as a novel tool for future regenerative medicine. Stem Cells. 2012;30:804–810. doi:10.1002/stem.107622415904
- Dong YX, Rodrigues M, Kwon SH, et al. Acceleration of diabetic wound regeneration using an in situ–formed stem-cell-based skin substitute. Adv Healthcare Mater. 2018;7:1800432. doi:10.1002/adhm.201800432
- Fu JP, Zhang Y, Chu J, et al. Reduced graphene oxide incorporated acellular dermal composite scaffold enables efficient local delivery of mesenchymal stem cells for accelerating diabetic wound healing. ACS Biomater Sci Eng. 2019;5:4054–4066. doi:10.1021/acsbiomaterials.9b00485
- Liu S, Yu J, Zhang QF, et al. Dual cross-linked HHA hydrogel supplies and regulates MΦ2 for synergistic improvement of immunocompromise and impaired angiogenesis to enhance diabetic chronic wound healing. Biomacromolecules. 2020;21:3795–3806. doi:10.1021/acs.biomac.0c0089132786521
- Tyeb S, Shiekh PA, Verma V, et al. Adipose-derived stem cells (ADSCs) loaded gelatin-sericin-laminin cryogels for tissue regeneration in diabetic wounds. Biomacromolecules. 2020;21:294–304. doi:10.1021/acs.biomac.9b0135531771325
- Gao WD, Jin WW, Li YN, et al. A highly bioactive bone extracellular matrix-biomimetic nanofibrous system with rapid angiogenesis promotes diabetic wound healing. J Mater Chem B. 2017;5:7285–7296. doi:10.1039/c7tb01484h32264178
- Morris AH, Lee H, Xing H, et al. Tunable hydrogels derived from genetically engineered extracellular matrix accelerate diabetic wound healing. ACS Appl Mater Interfaces. 2018;10:41892–41901. doi:10.1021/acsami.8b0892030424595
- Morris AH, Stamer DK, Kunkemoeller B, et al. Decellularized materials derived from TSP2-KO mice promote enhanced neovascularization and integration in diabetic wounds. Biomaterials. 2018;169:61–71. doi:10.1016/j.biomaterials.2018.03.04929631168
- Wang H, Agarwal P, Xiao Y, et al. A nano-in-micro system for enhanced stem cell therapy of ischemic diseases. ACS Cent Sci. 2017;3:875–885. doi:10.1021/acscentsci.7b0021328852702
- Shen YI, Cho H, Papa AE, et al. Engineered human vascularized constructs accelerate diabetic wound healing. Biomaterials. 2016;102:107–119. doi:10.1016/j.biomaterials.2016.06.00927328431
- Shi Q, Qian Z, Liu D, et al. GMSC-derived exosomes combined with a chitosan/silk hydrogel sponge accelerates wound healing in a diabetic rat skin defect model. Front Physiol. 2017;8:904. doi:10.3389/fphys.2017.0090429163228
- Johnstone RM, Adam M, Hammond JR, et al. Vesicle formation during reticulocyte maturation. Association of plasma membrane activities with released vesicles (exosomes). J Biol Chem. 1987;262:9412–9420. doi:10.1557/PROC-0928-GG08-043597417
- de Gassart A, Geminard C, Fevrier B, et al. Lipid raft-associated protein sorting in exosomes. Blood. 2003;102:4336–4344. doi:10.1182/blood-2003-03-087112881314
- Wubbolts R, Leckie RS, Veenhuizen PT, et al. Proteomic and biochemical analyses of human B cell-derived exosomes, potential implications for their function and multivesicular body formation. J Biol Chem. 2003;278:10963–10972. doi:10.1074/jbc.M20755020012519789
- Lai RC, Yeo RW, Lim SK. Mesenchymal stem cell exosomes. Semin Cell Dev Biol. 2015;40:82–88. doi:10.1016/j.semcdb.2015.03.00125765629
- Haney MJ, Klyachko NL, Zhao Y, et al. Exosomes as drug delivery vehicles for Parkinson’s disease therapy. J Control Release. 2015;207:18–30. doi:10.1016/j.jconrel.2015.03.03325836593
- Batrakova EV, Kim MS. Using exosomes, naturally-equipped nanocarriers, for drug delivery. J Control Release. 2015;219:396–405. doi:10.1016/j.jconrel.2015.07.03026241750
- Shtam TA, Kovalev RA, Varfolomeeva EY, et al. Exosomes are natural carriers of exogenous siRNA to human cells in vitro. Cell Commun Signal. 2013;11:88. doi:10.1186/1478-811X-11-8824245560
- Li X, Chen C, Wei L, et al. Exosomes derived from endothelial progenitor cells attenuate vascular repair and accelerate reendothelialization by enhancing endothelial function. Cytotherapy. 2016;18:253–262. doi:10.1016/j.jcyt.2015.11.00926794715
- Rani S, Ritter T. The exosome – a naturally secreted nanoparticle and its application to wound healing. Adv Mater. 2016;28:5542–5552. doi:10.1002/adma.20150400926678528
- Yu MY, Liu W, Li JX, et al. Exosomes derived from atorvastatin-pretreated MSC accelerate diabetic wound repair by enhancing angiogenesis via AKT/eNOS pathway. Stem Cell Res Ther. 2020;1:350. doi:10.1186/s13287-020-01824-2
- Lv QJ, Deng JF, Chen Y, et al. Engineered human adipose stem-cell-derived exosomes loaded with miR-21-5p to promote diabetic cutaneous wound healing. Mol Pharm. 2020;17:1723–1733. doi:10.1021/acs.molpharmaceut.0c0017732233440
- Wang CG, Wang M, Xu TZ, et al. Engineering bioactive self-healing antibacterial exosomes hydrogel for promoting chronic diabetic wound healing and complete skin regeneration. Theranostics. 2019;9(1):65–76. doi:10.7150/thno.2976630662554
- Wang M, Wang CG, Chen M, et al. Efficient angiogenesis-based diabetic wound healing/skin reconstruction through bioactive antibacterial adhesive ultraviolet shielding nanodressing with exosome release. ACS Nano. 2019;13:10279–10293. doi:10.1021/acsnano.9b0365631483606
- Tao SC, Guo SC, Min L, et al. Chitosan wound dressings incorporating exosomes derived from microRNA-126-overexpressing synovium mesenchymal stem cells provide sustained release of exosomes and heal full-thickness skin defects in a diabetic rat model. Stem Cells Transl Med. 2017;6:736–747. doi:10.5966/sctm.2016-027528297576
- Li M, Ke QF, Tao SC, et al. Fabrication of hydroxyapatite/chitosan composite hydrogels loaded with exosomes derived from miR-126-3p overexpressed synovium mesenchymal stem cells for diabetic chronic wound healing. J Name. 2013:1–11. doi:10.1039/C6TB01560C
- Jyoti K, Malik G, Chaudhary M, et al. Chitosan and phospholipid assisted topical fusidic acid drug delivery in burn wound: strategies to conquer pharmaceutical and clinical challenges, opportunities and future panorama. Int J Biol Macromol. 2020;161:325–335. doi:10.1016/j.ijbiomac.2020.05.23032485249
- Yang Y, Yin DK, Wang F, et al. In situ eNOS/NO up-regulation – a simple and effective therapeutic strategy for diabetic skin ulcer. Sci Rep. 2016;6:30326. doi:10.1038/srep3032627453476
- Amiel AG, Durand CP, Maton M, et al. Designed sponges based on chitosan and cyclodextrin polymer for a local release of ciprofloxacin in diabetic foot infections. Int J Pharm. 2020;587:119677. doi:10.1016/j.ijpharm.2020.11967732717280
- Wu YB, Zhou ZP, Luo L, et al. A non-anticoagulant heparin-like snail glycosaminoglycan promotes healing of diabetic wound. Carbohydr Polym. 2020;247:116682. doi:10.1016/j.carbpol.2020.11668232829810
- Ren J, Yang MJ, Chen JW, et al. Anti-inflammatory and wound healing potential of kirenol in diabetic rats through the suppression of inflammatory markers and matrix metalloproteinase expressions. Biomed Pharmacother. 2020;129:110475. doi:10.1016/j.biopha.2020.11047532768960
- Chu J, Shi PP, Yan WX, et al. PEGylated graphene oxide-mediated quercetin-modified collagen hybrid scaffold for enhancement of MSCs differentiation potential and diabetic wound healing. Nanoscale. 2018;10:9547–9560. doi:10.1039/c8nr02538j29745944
- Mittal AK, Bhardwaj R, Arora R, et al. Acceleration of wound healing in diabetic rats through poly dimethylaminoethyl acrylate−hyaluronic acid polymeric hydrogel impregnated with a didymocarpus pedicellatus plant extract. ACS Omega. 2020. doi:10.1021/acsomega.0c02040
- Liu YY, Sui YL, Liu C, et al. A physically crosslinked polydopamine/nanocellulose hydrogel as potential versatile vehicles for drug delivery and wound healing. Carbohydrate Polymersn. 2018;188:27–36. doi:10.1016/j.carbpol.2018.01.093
- Martı´nez-Romero R, Martı´nez-Lara E, Aguilar-Quesada R, et al. PARP-1 modulates deferoxamine-induced HIF-1alpha accumulation through the regulation of nitric oxide and oxidative stress. J Cell Biochem. 2008;104:2248. doi:10.1002/jcb.2178118459142
- Wu Y, Li X, Xie W, et al. Neuroprotection of deferoxamine on rotenone-induced injury via accumulation of HIF-1 alpha and induction of autophagy in SH-SY5Y cells. Neurochem Int. 2010;57:198–205. doi:10.1016/j.neuint.2010.05.00820546814
- Hou ZJ, Nie CL, Si ZX, et al. Deferoxamine enhances neovascularization and accelerates wound healing in diabetic rats via the accumulation of hypoxia-inducible factor-1α. Diabetes Res Clin Pract. 2013;101:62–71. doi:10.1016/j.diabres.2013.04.01223726275
- Xing D, Liu L, Marti GP, et al. Hypoxia and hypoxia-inducible factor in the burn wound. Wound Repair Regen. 2011;19:205. doi:10.1111/j.1524-475X.2010.00656.x21362088
- Chen H, Jia P, Kang H, et al. Upregulating Hif-1α by hydrogel nanofi- brous scaffolds for rapidly recruiting angiogenesis relative cells in diabetic wound. Adv Healthcare Mater. 2016;5:907–918. doi:10.1002/adhm.201501018
- Chen H, Guo L, Wicks J, et al. Quickly promoting angiogenesis by using a DFO-loaded photo-crosslinked gelatin hydrogel for diabetic skin regeneration. J Mater Chem B. 2016;4:3770–3781. doi:10.1039/c6tb00065g32263315
- Duscher D, Neofytou E, Wong VW, et al. Transdermal deferoxamine prevents pressure-induced diabetic ulcers. PNAS. 2015;112:94–99. doi:10.1073/pnas.141344511225535360
- Kong L, Zhi W, Zhao H, et al. Bioactive injectable hydrogels containing desferrioxamine and bioglass for diabetic wound healing. ACS Appl Mater Interfaces. 2018;10:30103–30114. doi:10.1021/acsami.8b0919130113159
- Emanuelli T, Burgeiro A, Carvalho E. Effects of insulin on the skin: possible healing benefits for diabetic foot ulcers. Arch Dermatol Res. 2016;308:677–694. doi:10.1007/s00403-016-1686-z27655635
- Zhao LL, Niu LJ, Liang HZ, et al. pH and glucose dual-responsive injectable hydrogels with insulin and fibroblasts as bioactive dressings for diabetic wound healing. ACS Appl Mater Interfaces. 2017;9:37563–37574. doi:10.1021/acsami.7b0939528994281
- Ren XZ, Han YM, Wang J, et al. An aligned porous electrospun fibrous membrane with controlled drug delivery – an efficient strategy to accelerate diabetic wound healing with improved angiogenesis. Acta Biomater. 2018;70:140–153. doi:10.1016/j.actbio.2018.02.01029454159
- Liu J, Chen ZQ, Wang J, et al. Encapsulation of curcumin nanoparticles with MMP9-responsive and thermos-sensitive hydrogel improves diabetic wound healing. ACS Appl Mater Interfaces. 2018;10:16315–16326. doi:10.1021/acsami.8b0386829687718
- Ghufran H, Mehmood A, Azam M, et al. Curcumin preconditioned human adipose derived stem cells co-transplanted with platelet rich plasma improve wound healing in diabetic rats. Life Sci. 2020;257:118091. doi:10.1016/j.lfs.2020.11809132668325
- Karri VVSR, Kuppusamy G, Talluri SV, et al. Curcumin loaded chitosan nanoparticles impregnated into collagen-alginate scaffolds for diabetic wound healing. Int J Biol Macromol. 2020;257:118091. doi:10.1016/j.ijbiomac.2016.05.038
- Mendes C, Haupenthal DPD, Zaccaron RP, et al. Effects of the association between photobiomodulation and hyaluronic acid linked gold nanoparticles in wound healing. ACS Biomater Sci Eng. 2020;6:5132–5144. doi:10.1021/acsbiomaterials.0c00294
- Edwards JV, Prevost NT, Santiago M, et al. Hydrogen peroxide generation of copper/ascorbate formulations on cotton: effect on antibacterial and fibroblast activity for wound healing application. Molecules. 2018;23:2399. doi:10.3390/molecules23092399
- Maity GN, Maity P, Choudhuri I, et al. Green synthesis, characterization, antimicrobial and cytotoxic effect of silver nanoparticles using arabinoxylan isolated from Kalmegh. Int J Biol Macromol. 2002;162:1025–1034. doi:10.1016/j.ijbiomac.2020.06.215
- Mao CY, Xiang YM, Liu XM, et al. Photo-inspired antibacterial activity and wound healing acceleration by hydrogel embedded with Ag/Ag@AgCl/ZnO nanostructures. ACS Nano. 2017;11:9010–9021. doi:10.1021/acsnano.7b0351328825807
- Shi LY, Zhao YN, Xie QF, et al. Moldable hyaluronan hydrogel enabled by dynamic metal–bisphosphonate coordination chemistry for wound healing. Adv Healthcare Mater. 2018;7:1700973. doi:10.1002/adhm.201700973
- Zhang PJ, Li Y, Tang YH, et al. Copper-based metal−organic framework as a controllable nitric oxide-releasing vehicle for enhanced diabetic wound healing. ACS Appl Mater Interfaces. 2020;12:18319–18331. doi:10.1021/acsami.0c0179232216291
- Salomoni R, Léo P, Montemor AF, et al. Antibacterial effect of silver nanoparticles in Pseudomonas aeruginosa. Nanotechnol Sci Appl. 2017;10:115–121. doi:10.2147/NSA.S13341528721025
- Qiao Y, Fei M, Liu C, et al. Near-infrared laser-excited nanoparticles to eradicate multidrug-resistant bacteria and promote wound healing. ACS Appl Mater Interfaces. 2018;10(1):193–206. doi:10.1021/acsami.7b1525129215863
- Tong CY, Zhong XH, Yang YJ, et al. PB@PDA@Ag nanosystem for synergistically eradicating MRSA and accelerating diabetic wound healing assisted with laser irradiation. Biomaterials. 2020;243:119936. doi:10.1016/j.biomaterials.2020.11993632171103
- Zhao Y, Li ZH, Song SL, et al. Skin-inspired antibacterial conductive hydrogels for epidermal sensors and diabetic foot wound dressings. Adv Funct Mater. 2019;29:1901474. doi:10.1002/adfm.201901474
- Pankhurst QA, Connolly J, Jones SK, et al. Applications of magnetic nanoparticles in biomedicine. J Phys D Appl Phys. 2003;36(13):167–181. doi:10.1088/0022-3727/36/13/201
- Anker JN, Hall WP, Lyandres O, et al. Biosensing with plasmonic nanosensors. Nat Mater. 2008;7(6):442–453. doi:10.1038/nmat216218497851
- Wrobel JS, Najafi B. Diabetic foot biomechanics and gait dysfunction. J Diabetes Sci Technol. 2010;4(4):833–845. doi:10.1177/19322968100040041120663446
- Pissuwan D, Valenzuela SM, Cortie MB. Therapeutic possibilities of plasmonically heated gold nanoparticles. Trends Biotechnol. 2006;24(2):62–67. doi:10.1016/j.tibtech.2005.12.00416380179
- Kim H, Kawazoe T, Han DW, et al. Enhanced wound healing by an epigallocatechin gallate-incorporated collagen sponge in diabetic mice. Wound Repair Regen. 2008;16(5):714–720. doi:10.1111/j.1524-475X.2008.00422.x19128267
- Leu J-G, Chen S-A, Chen H-M, et al. The effects of gold nanoparticles in wound healing with antioxidant epigallocatechin gallate and α-lipoic acid. Nanomedicine. 2012;8(5):767–775. doi:10.1016/j.nano.2011.08.01321906577
- Wang S, Yan C, Zhang XM, et al. Antimicrobial peptide modification enhances the gene delivery and bactericidal efficiency of gold nanoparticles for accelerating diabetic wound healing. Biomater Sci. 2018;6:2757–2772. doi:10.1039/c8bm00807h30187036
- Xiao JS, Zhu YX, Huddleston S, et al. Copper metal−organic framework nanoparticles stabilized with folic acid improve wound healing in diabetes. ACS Nano. 2018;12:1023–1032. doi:10.1021/acsnano.7b0185029406741
- Xiao JS, Chen S, Yi J, et al. A cooperative copper metal–organic framework-hydrogel system improves wound healing in diabetes. Adv Funct Mater. 2017;27:1604872. doi:10.1002/adfm.20160487228729818
- Bhadauriya P, Mamtani H, Ashfaq M, et al. Synthesis of yeast-immobilized and copper nanoparticle-dispersed carbon nanofiber-based diabetic wound dressing material: simultaneous control of glucose and bacterial infections. ACS Appl Bio Mater. 2018;1:246–258. doi:10.1021/acsabm.8b00018
- Lv F, Wang J, Xu P, et al. A conducive bioceramic/polymer composite biomaterial for diabetic wound healing. Acta Biomater. 2017;60:128–143. doi:10.1016/j.actbio.2017.07.02028713016
- Jiang YQ, Li Y, Li JK, et al. A mussel-inspired extracellular matrix-mimicking composite scaffold for diabetic wound healing. ACS Appl Bio Mater. 2020;3:4052–4061. doi:10.1021/acsabm.0c00143
- Li JY, Lv F, Xu H, et al. A patterned nanocomposite membrane for high-efficiency healing of diabetic wound. J Mater Chem B. 2017;5:1926–1934. doi:10.1039/c7tb00124j32263946
- Das M, Goswami U, Kandimalla R, et al. Iron−copper bimetallic nanocomposite reinforced dressing materials for infection control and healing of diabetic wound. ACS Appl Bio Mater. 2019;2:5434–5445. doi:10.1021/acsabm.9b00870
- Raucci MG, Demitri C, Soriente A, et al. Gelatin/nano-hydroxyapatite hydrogel scaffold prepared by sol-gel technology as filler to repair bone defects. J Biomed Mater Res Part A. 2018;106:2007–2019. doi:10.1002/jbm.a.36395
- Xie WH, Fu XL, Tang FL, et al. Dose-dependent modulation effects of bioactive glass particles on macrophages and diabetic wound healing. J Mater Chem B. 2019;7:940. doi:10.1039/c8tb02938e32255099
- Zeng QY, Han Y, Li HY, et al. Design of thermosensitive bioglass/agarose-alginate composite hydrogel for chronic wound healing. J Mater Chem B. 2015;3:8856–8864. doi:10.1039/c5tb01758k32263479
- Jiang YQ, Han YM, Wang J, et al. Space-oriented nanofibrous scaffold with silicon-doped amorphous calcium phosphate nanocoating for diabetic wound healing. ACS Appl Bio Mater. 2019;2:787–795. doi:10.1021/acsabm.8b00657
- Nunan R, Harding KG, Martin P. Clinical challenges of chronic wounds: searching for an optimal animal model to recapitulate their complexity. Dis Model Mech. 2014;7:1205–1213. doi:10.1242/dmm.01678225359790
- Sen CK. Wound healing essentials: let there be oxygen. Wound Repair Regen. 2009;17:1–18. doi:10.1111/j.1524-475X.2008.00436.x19152646
- Giacco F, Brownlee M. Oxidative stress and diabetic complications. Circ Res. 2010;107:1058–1070. doi:10.1161/CIRCRESAHA.110.22354521030723
- Lan CCE, Wu CS, Huang SM, et al. High-glucose environment enhanced oxidative stress and increased interleukin-8 secretion from keratinocytes: new insights into impaired diabetic wound healing. Diabetes. 2013;62:2530–2538. doi:10.2337/db12-171423423570
- Zhu Y, Cankova Z, Iwanaszko M, et al. Potent laminin-inspired antioxidant regenerative dressing accelerates wound healing in diabetes. Proc Natl Acad Sci. 2018;115(26):6816–6821. doi:10.1073/pnas.180426211529891655
- Ahmed R, Tariq M, Ali I, et al. Novel electrospun chitosan/polyvinylalcohol/zinc oxide nanofibrous mats with antibacterial and antioxidant properties for diabetic wound healing. Int J Biol Macromol. 2018;120:385–393. doi:10.1016/j.ijbiomac.2018.08.05730110603
- Nureddin A, Ali DM, Seda KN, et al. Advances in controlled oxygen generating biomaterials for tissue engineering and regenerative therapy. Biomaterials. 2020;21(1):56–72. doi:10.1021/acs.biomac.9b00546
- Eileen P, Coronel MM, Fraker CA, et al. Preventing hypoxia-induced cell death in beta cells and islets via hydrolytically activated, oxygen-generating biomaterials. Proc Natl Acad Sci. 2012;109(11):4245–4250. doi:10.1073/pnas.111356010922371586
- Harrison BS, Eberli D, Lee SJ, et al. Oxygen producing biomaterials for tissue regeneration. Biomaterials. 2007;28:4628–4634. doi:10.1016/j.biomaterials.2007.07.00317681597
- Abdi SIH, Ng SM, Lim JO. An enzyme-modulated oxygen-producing micro-system for regenerative therapeutics. Int J Pharm. 2011;409:203–205. doi:10.1016/j.ijpharm.2011.02.04121356297
- White JC, Stoppel WL, Susan C, et al. Addition of perfluorocarbons to alginate hydrogels significantly impacts molecular transport and fracture stress. J Biomed Mater Res A. 2013;101(2):438–446. doi:10.1002/jbm.a.3434422865503
- Shiekh PA, Singh A, Kumar A. Exosome laden oxygen releasing antioxidant and antibacterial cryogel wound dressing OxOBand alleviate diabetic and infectious wound healing. Biomaterials. 2020;249:120020. doi:10.1016/j.biomaterials.2020.12002032305816
- Kim HJ, Matsuda H, Zhou H, et al. Ultrasound–triggered smart drug release from a poly (dimethylsiloxane)–mesoporous silica composite. Adv Mater. 2006;18(23):3083–3088. doi:10.1002/adma.200600387
- Song X, Feng L, Liang C, et al. Ultrasound triggered tumor oxygenation with oxygen-shuttle nanoperfluorocarbon to overcome hypoxia-associated resistance in cancer therapies. Nano Lett. 2016;16(10):6145–6153. doi:10.1021/acs.nanolett.6b0236527622835
- Song G, Liang C, Yi X, et al. Cancer therapy: perfluorocarbon-loaded hollow Bi2Se3 nanoparticles for timely supply of oxygen under near-infrared light to enhance the radiotherapy of cancer. Adv Mater. 2016;28(14):2654. doi:10.1002/adma.201504617
- Castro CI, Briceno JC. Perfluorocarbon–based oxygen carriers: review of products and trials. Artif Organs. 2010;34(8):622–634. doi:10.1111/j.1525-1594.2009.00944.x20698841
- Wang W, Cheng Y, Yu P, et al. Perfluorocarbon regulates the intratumoural environment to enhance hypoxia-based agent efficacy. Nat Commun. 2019;10(1):1580. doi:10.1038/s41467-019-09389-230952842
- Marano F, Argenziano M, Frairia R, et al. Doxorubicin-loaded nanobubbles combined with extracorporeal shock waves: basis for a new drug delivery tool in anaplastic thyroid cancer. Thyroid. 2016;26(5):705–716. doi:10.1089/thy.2015.034226906083
- Marano F, Frairia R, Rinella L, et al. Combining doxorubicin-nanobubbles and shockwaves for anaplastic thyroid cancer treatment: preclinical study in a xenograft mouse model. Endocr Relat Cancer. 2017;24(6):275–286. doi:10.1530/ERC-17-004528487350
- Wang S, Yin C, Han X, et al. Improved healing of diabetic foot ulcer upon oxygenation therapeutics through oxygen-loading nanoperfluorocarbon triggered by radial extracorporeal shock wave. Oxid Med Cell Longev. 2019;2019:5738368. doi:10.1155/2019/573836831485296
- Zehra M, Zubairi W, Hasan A, et al. Oxygen generating polymeric nano fibers that stimulate angiogenesis and show efficient wound healing in a diabetic wound model. Int J Nanomedicine. 2020;15:3511–3522. doi:10.2147/IJN.S24891132547010
- Zhang XX, Chen GP, Liu YX, et al. Black phosphorus-loaded separable microneedles as responsive oxygen delivery carriers for wound healing. ACS Nano. 2020;14:5901–5908. doi:10.1021/acsnano.0c0105932315159
- Abudula T, Gauthaman K, Hammad AH, et al. Oxygen-releasing antibacterial nanofibrous scaffolds for tissue engineering applications. Polymers. 2020;12:1233. doi:10.3390/polym12061233
- Kang JI, Park KM, Park KD. Oxygen-generating alginate hydrogels as a bioactive acellular matrix for facilitating wound healing. J Ind Eng Chem. 2019;69:397–404. doi:10.1016/j.jiec.2018.09.048
- Zhang T, Han ZY, Zhang W, et al. Cyanoacrylate-encapsulated calcium peroxide achieved oxygen-sustained release and promoted wound healing. Int J Polym Mater Poly Biomater. 2020;69:703–708. doi:10.1080/00914037.2019.1600518
- Durante W. Hydrogen sulfide therapy in diabetes-accelerated atherosclerosis: awhiff of success. Diabetes. 2016;65:2832–2834. doi:10.2337/dbi16-004227659227
- Coletta C, Papapetropoulos A, Erdelyi K, et al. Hydrogen sulfide and nitric oxide are mutually dependent in the regulation of angiogenesis and endothelium-dependent vasorelaxation. PNAS. 2012;109:9161–9166. doi:10.1073/pnas.120291610922570497
- Lin WC, Huang CC, Lina SJ, et al. In situ depot comprising phase-change materials that can sustainably release a gasotransmitter H2S to treat diabetic wounds. Biomaterials. 2017;145:1–8. doi:10.1016/j.biomaterials.2017.08.02328843063
- Hamdan S, Pastar I, Drakulich S, et al. Nanotechnology-driven therapeutic interventions in wound healing: potential uses and applications. ACS Central Science. 2017;3:163–175. doi:10.1021/acscentsci.6b0037128386594
- Zhao YZ, Vanhoutte PM, Leung SWS. Vascular nitric oxide: beyond eNOS. J Pharmacol Sci. 2015;129:83–94. doi:10.1016/j.jphs.2015.09.00226499181
- Balakumar P, Chakkarwar VA, Krishan P, et al. Vascular endothelial dysfunction: a tug of war in diabetic nephropathy? Biomed Pharmacother. 2009;63:171–179. doi:10.1016/j.biopha.2008.08.00818823739
- Chen YJ, Wu SC, Wang HC, et al. Activation of angiogenesis and wound healing in diabetic mice using NO-delivery dinitrosyl iron complexes. Mol Pharmaceutics. 2019;16:4241–4251. doi:10.1021/acs.molpharmaceut.9b00586
- Horikoshi S, Serpone N. Introduction to nanoparticles. Micro-Waves Nanoparticle Synth Fundam Appl. 2013;1–24. doi:10.1002/9783527648122.ch1
- Pantarotto D, Partidos CD, Hoebeke J, et al. Immunization with peptide-functionalized carbon nanotubes enhances virus-specific neutralizing antibody responses. Chem Biol. 2016;10(10):961–966. doi:10.1016/j.chembiol.2003.09.09.011
- Salata O. Applications of nanoparticles in biology and medicine. J Nanobiotechnology. 2004;2(1):3. doi:10.1186/1477-3155-2-315119954
- Bruchez Jr. JM. Semiconductor nanocrystals as fluorescent biological labels. Science. 1998;281(5385):2013–2016. doi:10.1126/science.281.5385.20139748157
- Wang S, Mamedova N, Kotov NA, et al. Antigen/antibody immunocomplex from CdTe nanoparticle bioconjugates. Nano Lett. 2002;2(8):817–822. doi:10.1021/nl0255193
- Tong Z, Dong L, Zhou L, et al. Nisin inhibits dental caries-associated microorganism in vitro. Peptides. 2010;31:2003–2008. doi:10.1021/acsbiomaterials.9b0054720688123
- He X, Ding YF, Xie WJ, et al. Rubidium-containing calcium alginate hydrogel for antibacterial and diabetic skin wound healing applications. ACS Biomater Sci Eng. 2019;5:4726–4738. doi:10.1021/acsbiomaterials.9b00547
- Kumar S, Lakshmanan VK, Raj M, et al. Evaluation of wound healing potential of β-chitin hydrogel/nano zinc oxide composite bandage. Pharm Res. 2013;30:523–537. doi:10.1007/s11095-012-0898-y23135816
- Augustine R, Hasan A, Patan NK, et al. Cerium oxide nanoparticle incorporated electrospun poly(3-hydroxybutyrate-co-3-hydroxyvalerate) membranes for diabetic wound healing applications. ACS Biomater Sci Eng. 2020;6:58–70. doi:10.1021/acsbiomaterials.8b01352
- Soenen SJ, Paak WJ, Rejman J, et al. (Inter)Cellular stability of inorganic nanoparticles: effects on cytotoxicity, particle functionality, and biomedical applications. Chem Rev. 2015;115:2109–2135. doi:10.1021/cr400714j25757742
- Gao Q, Zhang X, Yin WY, et al. Functionalized MoS2 nanovehicle with near-infrared laser-mediated nitric oxide release and photothermal activities for advanced bacteria-infected wound therapy. Small. 2018;14(45):1802290. doi:10.1002/smll.201802290
- Zhao Y, Cai Q, Qi W, et al. BSA-CuS nanoparticles for photothermal therapy of diabetic wound infection in vivo. Biol Chem Chem Biol. 2018;3:9510–9516. doi:10.1002/slct.201802069
- Thangavel P, Kannan R, Ramachandran B, et al. Development of reduced graphene oxide (rGO)-isabgol nanocomposite dressings for enhanced vascularization and accelerated wound healing in normal and diabetic rats. J Colloid Interface Sci. 2018;517:251–264. doi:10.1016/j.jcis.2018.01.11029428812
- Sun L, Zhang Y, Wang Y, et al. Real-time subcellular imaging based on graphene biosensors. Nanoscale. 2018;10(4):1759–1765. doi:10.1039/c7nr07479d29308810
- Jing X, Mi HY, Napiwocki BN, et al. Mussel-inspired electroactive chitosan/graphene oxide composite hydrogel with rapid self-healing and recovery behavior for tissue engineering. Carbon. 2017;125:557–570. doi:10.1016/j.carbon.2017.09.071
- Tessmar JK, Göpferich AM. Matrices and scaffolds for protein delivery in tissue engineering. Adv Drug Delivery Rev. 2007;59:274. doi:10.1016/j.addr.2007.03.020
- Andrew JS, Anglin EJ, Wu EC, et al. Sustained release of a monoclonal antibody from electrochemically prepared mesoporous silicon oxide. Adv Funct Mater. 2011;20:4168. doi:10.1002/adfm.201190106
- Rytkönen J, Arukuusk P, Xu W, et al. Porous silicon–cell penetrating peptide hybrid nanocarrier for intracellular delivery of oligonucleotides. Mol Pharmaceutics. 2014;11:382–390. doi:10.1021/mp4002624
- Christopher TT, Steven JPM, Elizabeth M, et al. Delivery of flightless I neutralizing antibody from porous silicon nanoparticles improves wound healing in diabetic mice. Adv Healthcare Mater. 2017;6:1600707. doi:10.1002/adhm.201600707
- Xie Z, Paras CB, Weng H, et al. Dual growth factor releasing multi-functional nanofibers for wound healing. Acta Biomater. 2013;9(12):9351–9359. doi:10.1016/j.actbio.2013.07.03023917148
- Majd SA, Khorasgani MR, Moshtaghian SJ, et al. Application of chitosan/PVA nano fiber as a potential wound dressing for streptozotocin-induced diabetic rats. Int J Biol Macromol. 2016;92:1162–1168. doi:10.1016/j.ijbiomac.2016.06.03527492559
- Xia GX, Liu Y, Tian MP, et al. Nanoparticles/thermosensitive hydrogel reinforced with chitin whiskers as wound dressing for treating chronic wounds. J Mater Chem B. 2017;5:3172–3185. doi:10.1039/c7tb00479f32263715
- Correa VLR, Martins JA, Souza TR, et al. Melatonin loaded lecithin-chitosan nanoparticles improved the wound healing in diabetic rats. Int J Biol Macromol. 2020;162:1465–1475. doi:10.1016/j.ijbiomac.2020.08.02732781118
- Patrulea V, Ostafe V, Borchard G, et al. Chitosan as a starting material for wound healing applications. Eur J Pharm Biopharm. 2015;97:417–426. doi:10.1016/j.ejpb.2015.08.00426614560
- Ali L, Ahmad M, Aamir MN, et al. Venlafaxine-loaded sustained-release poly (hydroxyethyl methacrylate-co-itaconic acid) hydrogel composites: their synthesis and in vitro/in vivo attributes. Iran Polym J. 2019:1–8. doi:10.1007/s13726-019-00697-4
- Paterson SM, Shadforth AMA, Brown DH, et al. The synthesis and degradation of collagenase-degradable poly(2-hydroxyethyl methacrylate)-based hydrogels and sponges for potential applications as scaffolds in tissue engineering. Mater Sci Eng C Mater Biol Appl. 2012;32(8):2536–2544. doi:10.1016/j.msec.2012.07.037
- Lin YS, Ming LJ, Peng JS, et al. Radical annihilation of γ-ray-irradiated contact lens blanks made of a 2-hydroxyethyl methacrylate copolymer at elevated temperatures. J Appl Polym Sci. 2010;117(6):3114–3120. doi:10.1002/app.31947
- Shah SA, Sohail M, Minhas MU, et al. pH-responsive CAP-co-poly (methacrylic acid)-based hydrogel as an efficient platform for controlled gastrointestinal delivery: fabrication, characterization, in vitro and in vivo toxicity evaluation. Drug Deliv Transl Res. 2019;9(2):555–577. doi:10.1007/s13346-018-0486-829450805
- Yu L, Ding J. Injectable hydrogels as unique biomedical materials. Chem Soc Rev. 2008;37:1473–1481. doi:10.1039/b713009k18648673
- Zheng ZQ, Bian SQ, Li ZQ, et al. Catechol modified quaternized chitosan enhanced wet adhesive and antibacterial properties of injectable thermo-sensitive hydrogel for wound healing. Carbohydr Polym. 2020;249:116826. doi:10.1016/j.carbpol.2020.11682632933673
- Cai L, Dewi RE, Heilshorn SC. Injectable hydrogels with in situ double network formation enhance retention of transplanted stem cells. Adv Funct Mater. 2015;25:1344–1351. doi:10.1002/adfm.20140363126273242
- Truong VX, Ablett MP, Richardson SM, et al. Simultaneous orthogonal dual-click approach to tough, in-situ-forming hydrogels for cell encapsulation. J Am Chem Soc. 2015;137:1618–1622. doi:10.1021/ja511681s25590670
- Yang JA, Yeom J, Hwang BW, et al. In situ-forming injectable hydrogels for regenerative medicine. Prog Polym Sci. 2014;39:1973–1986. doi:10.1016/j.progpolymsci.2014.07.006
- Eggermont LJ, Rogers ZJ, Colombani T, et al. Injectable cryogels for biomedical applications. Trends Biotechnol. 2020;38:418–431. doi:10.1016/j.tibtech.2019.09.00831699534
- Chen H, Cheng RY, Zhao X, et al. An injectable self-healing coordinative hydrogel with antibacterial and angiogenic properties for diabetic skin wound repair. NPG Asia Mater. 2019;11:3. doi:10.1038/s41427-018-0103-9
- Wang SQ, Zheng H, Zhou L, et al. Nanoenzyme-reinforced injectable hydrogel for healing diabetic wounds infected with multidrug resistant bacteria. Nano Lett. 2020;20:5149–5158. doi:10.1021/acs.nanolett.0c0137132574064
- Li J, Hu WQ, Zhang YJ, et al. pH and glucose dually responsive injectable hydrogel prepared by in situ crosslinking of phenylboronic modified chitosan and oxidized dextran. J Polym Sci Part A: Polym Chem. 2015;53:1235–1244. doi:10.1002/pola.27556
- Zhu YN, Zhang JM, Song JY, et al. A multifunctional pro-healing zwitterionic hydrogel for simultaneous optical monitoring of pH and glucose in diabetic wound treatment. Adv Funct Mater. 2019:1905493. doi:10.1002/adfm.201905493
- Liu Y, Pharr M, Salvatore GA. Lab-on-skin: a review of flexible and stretchable electronics for wearable health monitoring. ACS Nano. 2017;11:9614–9635. doi:10.1021/acsnano.7b0489828901746
- Liang S, Zhang Y, Wang H, et al. Paintable and rapidly bondable conductive hydrogels as therapeutic cardiac patches. Adv Mater. 2018;30:1704235. doi:10.1002/adma.201704235
- Liao M, Wan P, Wen J, et al. Wearable, healable, and adhesive epidermal sensors assembled from mussel-inspired conductive hybrid hydrogel framework. Adv Funct Mater. 2017;27:1703852. doi:10.1002/adfm.201703852
- Zhou Y, Wan CJ, Yang YS, et al. Highly stretchable, elastic, and ionic conductive hydrogel for artificial soft electronics. Adv Funct Mater. 2019;29:1806220. doi:10.1002/adfm.201806220
- Hua DW, Gao ST, Zhang MJ, et al. A novel xanthan gum-based conductive hydrogel with excellent mechanical, biocompatible, and self-healing performances. Carbohydr Polym. 2020;247:116743. doi:10.1016/j.carbpol.2020.11674332829862
- Zhang JJ, Wu C, Xu YY, et al. A highly stretchable and conductive self-healing hydrogel for temperature and strain sensing and chronic wound treatment. ACS Appl Mater Interfaces. 2020;12:40990–40999. doi:10.1021/acsami.0c0829132808753
- Zhao H, Huang J, Li Y, et al. ROS-scavenging hydrogel to promote healing of bacteria infected diabetic wounds. Biomaterials. 2020;258:120286. doi:10.1016/j.biomaterials.2020.12028632798744
- Ma HS, Zhou Q, Chang J, et al. Grape seed-inspired smart hydrogel scaffolds for melanoma therapy and wound healing. ACS Nano. 2019;13:4302–4311. doi:10.1021/acsnano.8b0949630925040
- Wan WB, Cai F, Huang JY, et al. A skin-inspired 3D bilayer scaffold enhances granulation tissue formation and anti-infection for diabetic wound healing. J Mater Chem B. 2019;7:2954–2961. doi:10.1039/c8tb03341b
- Chen SX, Wang HJ, Su YJ, et al. Mesenchymal stem cell-laden, personalized 3D scaffolds with controlled structure and fiber alignment promote diabetic wound healing. Acta Biomater. 2020;108:153–167. doi:10.1016/j.actbio.2020.03.03532268240
- Yu BR, He CH, Wang WB, et al. Asymmetric wettable composite wound dressing prepared by electrospinning with bioinspired micropatterning enhances diabetic wound healing. ACS Appl Bio Mater. 2020;3:5383–5394. doi:10.1021/acsabm.0c00695
- Bao F, Pei G, Wu ZC, et al. Bioactive self-pumping composite wound dressings with micropore array modified janus membrane for enhanced diabetic wound healing. Adv Funct Mater. 2020:2005422. doi:10.1002/adfm.202005422
- Huang JL, Zhou JF, Zhuang JY, et al. Strong near-infrared absorbing and biocompatible CuS nanoparticles for rapid and efficient photothermal ablation of gram-positive and -negative bacteria. ACS Appl Mater Interfaces. 2017;9:36606–36614. doi:10.1021/acsami.7b1106228976189
- Chen J, Cheng G, Liu R, et al. Enhanced physical and biological properties of silk fibroin nanofibers by layer-by-layer deposition of chitosan and rectorite. J Colloid Interface Sci. 2018;523:208–216. doi:10.1016/j.jcis.2018.03.09329625323
- Tang ZY, Wang Y, Podsiadlo P, et al. Biomedical applications of layer-by-layer assembly: from biomimetics to tissue engineering. Adv Mater. 2006;18:3203. doi:10.1002/adma.200790025
- Shi YH, Wan DJ, Huang JH, et al. Stable LBL self-assembly coating porous membrane with 3D heterostructure for enhanced water treatment under visible light irradiation. Chemosphere. 2020;252:126581. doi:10.1016/j.chemosphere.2020.12658132222517
- Wu G, Ma X, Fan L, et al. Accelerating dermal wound healing and mitigating excessive scar formation using LBL modified nanofibrous mats. Mater Des. 2020;185:108265. doi:10.1016/j.matdes.2019.108265
- Oroojalian F, Jahanafrooz Z, Chogan F, et al. Synthesis and evaluation of injectable thermosensitive penta-block copolymer hydrogel (PNIPAAm-PCL-PEG-PCL-PNIPAAm) and star-shaped poly(CLCOLA)-b-PEG for wound healing applications. J Cell Biochem. 2019;120(10):17194–17207. doi:10.1002/jcb.2898031104319
- Andrade TAM, Iyer A, Das PK, et al. The inflammatory stimulus of a natural latex biomembrane improves healing in mice. Braz J Med Biol Res. 2011;44(10):1036–1047. doi:10.1590/S0100-879X201100750011621915475
- de Barros NR, Miranda MCR, Borges FA, et al. Oxytocin sustained release using natural rubber latex membranes. Int J Pept Res Ther. 2016;22(4):435–444. doi:10.1007/s10989-016-9523-y
- Davi CP, Lombello CB, Ferreira M. Higher cellular interaction and faster production of natural rubber latex LbL films by spraying method. Int J Adv Manuf Tech. 2019;100:999–1005. doi:10.1007/s00170-018-2778-z
- Long J, Etxeberria AE, Nand AV, et al. A 3D printed chitosan-pectin hydrogel wound dressing for lidocaine hydrochloride delivery. Mater Sci Eng C. 2019;104:109873. doi:10.1016/j.msec.2019.109873
- Ilhan E, Cesur S, Guler E, et al. Development of Satureja cuneifolia-loaded sodium alginate/polyethylene glycol scaffolds produced by 3D-printing technology as a diabetic wound dressing material. Int J Biol Macromol. 2020;161:1040–1054. doi:10.1016/j.ijbiomac.2020.06.08632544577
- Wu MX, Zhang YJ, Huang H, et al. Assisted 3D printing of microneedle patches for minimally invasive glucose control in diabetes. Mater Sci Eng C. 2020;117:111299. doi:10.1016/j.msec.2020.111299