Abstract
Superparamagnetic iron oxide nanoparticles (SPIONs) are an exciting advancement in the field of nanotechnology. They expand the possibilities of noninvasive analysis and have many useful properties, making them potential candidates for numerous novel applications. Notably, they have been shown that they can be tracked by magnetic resonance imaging (MRI) and are capable of conjugation with various cell types, including stem cells. In-depth research has been undertaken to establish these benefits, so that a deeper level of understanding of stem cell migratory pathways and differentiation, tumor migration, and improved drug delivery can be achieved. Stem cells have the ability to treat and cure many debilitating diseases with limited side effects, but a main problem that arises is in the noninvasive tracking and analysis of these stem cells. Recently, researchers have acknowledged the use of SPIONs for this purpose and have set out to establish suitable protocols for coating and attachment, so as to bring MRI tracking of SPION-labeled stem cells into common practice. This review paper explains the manner in which SPIONs are produced, conjugated, and tracked using MRI, as well as a discussion on their limitations. A concise summary of recently researched magnetic particle coatings is provided, and the effects of SPIONs on stem cells are evaluated, while animal and human studies investigating the role of SPIONs in stem cell tracking will be explored.
Keywords:
Introduction
Stem cells are omnipotent or pluripotent cells that are characterized by their self-renewal abilities through mitotic cell division, as well as by their potential for differentiation into a range of specialized cell types.Citation1 Their unique properties have prompted a rapidly emerging field of regenerative medicineCitation2 whereby damaged tissues are replaced with newly derived tissues constructed or seeded from appropriately differentiated stem cells.Citation3 In order to secure the success of this form of stem cell therapy,Citation4 it is essential that there is a safe way to track the movement of the implanted stem cells around the body, ensuring they reach their target tissue. Recent studies have reported the imaging of stem cells using varied cell labeling techniques for the treatment of serious, debilitating diseases including ischemic stroke,Citation5 skeletal dysplasia,Citation6 spinal cord injury,Citation7 and myocardial infarction.Citation8
A popular form of high spatial resolution imaging used in stem cell studies is magnetic resonance imaging (MRI). It is non-ionizing, noninvasive, and has the ability to produce three-dimensional images. Increased accuracy in image analysis can be achieved by using contrast agents. A popular contrast agent is superparamagnetic iron oxide nanoparticles (SPIONs). SPIONs are small crystalline magnetite structures ranging in size from 5 nm to 150 nm9 which, when appropriately coated, become biocompatible and are readily endocytosed into the cell. They act as good contrast agents in MRI, enhancing the contrast between different tissues present by inducing a darker area (negative contrast). This is demonstrated in , where 600 nm microgel iron oxide (M600) particles endocytosed into human fetal mesenchymal stem cells are imaged using transmission electron microscopy. With no cellular toxicity and a high sensitivity for MRI, M600 particles are a good candidate for use as a contrast agent in cell tracking using MRI. Additionally, SPIONs can be used for drug delivery and diagnostic purposes. Theoretically, SPIONs appear to be perfect for clinical use; however, their stability, biocompatibility,Citation10 and ability to locate and label the cellCitation11 must be controlled and enhanced. In this review, we explain the means of production of SPIONs and the different ways in which they are modified for specific stem cell tracking using MRI. We have also chosen to touch upon current research, both in animal and human studies that have utilized this technology, whilst discussing the potential limitations that exist.
Figure 1 Magnetic nanoparticles imaged in the cytoplasm of a stem cell.
Notes: A transmission electron microscopy image of a human fetal mesenchymal stem cell containing endocytosed microgel iron oxide particles (600 nm). Copyright © 2009 AlphaMed Press. Reproduced from Lee ES, Chan J, Shuter B, et al. Microgel iron oxide nanoparticles for tracking human fetal mesenchymal stem cells through magnetic resonance imaging. Stem Cells. 2009;27(8):1921–1931.Citation72
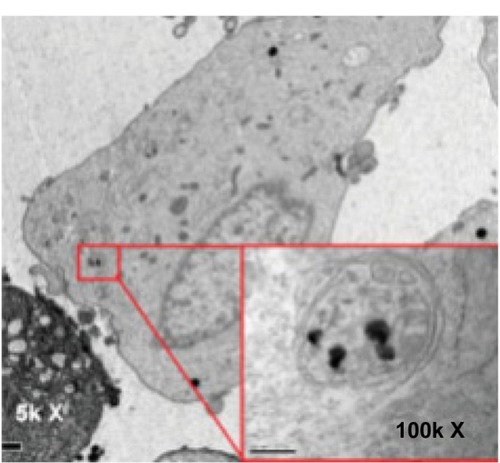
What is a superparamagnetic iron oxide nanoparticle?
SPIONs are a form of iron oxide nanoparticle that exhibit superparamagnetism,Citation12 a property similar to paramagnetism where a material shows magnetic properties only when under the influence of an external magnetic field. When such a field is present, as is produced by an MRI machine, the magnetic moments of the nanoparticle align in the direction of the applied field. No permanent magnetism is observed, and once the magnetic field is removed, the magnetic moments of the nanoparticle returns to being randomized by Brownian motion. Superparamagnetism is a property that is favorable for imaging, as it provides a higher tendency for the material to align with an applied magnetic field and a higher magnetization of a material in response to the applied magnetic field.Citation13 Several forms of iron oxide exist but, magnetite (Fe3O4) and maghemite (γ-Fe2O3) SPIONs are particularly common in the literature.Citation14 Numerous studies have been conducted concerning these structures, leading to the development of many new possible applications and improvements on existing technology using SPIONs, particularly in the field of biomedicine. These ideas exploit the rare superparamagnetic properties displayed by SPIONs for imaging with MRI.
Using SPIONs in magnetic resonance imaging (MRI)
MRI is a popular noninvasive, non-ionizing imaging technique that can be used to differentiate between pathological and healthy tissues. It is a powerful imaging method that is capable of providing insight into anatomical, physiological, and molecular processes, and it is often used for the diagnosis of diseases, the study of biological functions, and to identify cancer metastasis and inflammation.Citation13 MRI is based upon the relaxation properties of hydrogen atoms in water. The human body is largely composed of water molecules, with each molecule containing two hydrogen nuclei (protons). When a patient is subjected to the powerful magnetic field generated by an MRI scanner, the magnetic moments of the protons in the water molecules align with the direction of the field. A radio frequency electromagnetic field is then briefly turned on, causing the magnetic moment of the protons to differ from that of the applied magnetic field. The electric field is then switched off and the protons “relax”, returning to their original, random alignment via Brownian motion; in doing so, the protons create a radio frequency signal detected by the scanner. The technique allows for the detection of pathological tissues, such as tumors, because the protons in different tissues return to their equilibrium states at different rates. Optimizing scanner parameters or using MRI contrast agents can enhance contrast between tissues of interest, allowing for clearer imaging of specific molecules, cells, or tissues. In fact, although MRI has become one of the main imaging techniques used in oncology, its resolution is mostly insufficient at a molecular and cellular scale, unless magnetic contrast agents are employed.Citation15
SPIONs are effective contrast agents, owing to their superparamagnetic properties, high relaxivity,Citation16 and high sensitivity in MRI, which leads to the use of very low concentrations, reducing their side effects.Citation17 When SPIONs are present, they disturb the local magnetic field homogeneity and the large susceptibility differences between the iron oxide crystals and nearby protons, leading to a rapid dephasing of surrounding protons, resulting in a decrease in transverse (T2)Citation18 and translational (T1) relaxation times. The shorter transverse relaxation time, in particular, results in a darker image being observed in the vicinity of the SPIONs; this is referred to as negative contrast. SPIONs have been shown to be more efficient and longer lasting than many other agents, and they importantly exhibit long blood retention times, biodegradability, and low toxicity.Citation19 The magnetic properties of SPIONs can be manipulated by controlling the size of their core and coatings.Citation20 The basic morphology of a SPION is a single-domain iron oxide core that is <10 nm in size with a coating, which is most commonly dextran or a carboxy-dextran polymer.Citation21 Nanoparticle aggregation can be prevented and biocompatibility improved by varying the nature of the coating applied to the core. The coating is thick compared to the core and is, therefore, the main contributor to the hydrodynamic size. The size of the particle will inevitably determine the overall chemical and physiological properties of the nanoparticle.Citation22 A diagram of the basic structure of a magnetic nanoparticle (MNP) can be seen in . The figure details a magnetic core which, in the context of this article, is comprised of iron oxide, a protective coating, and active molecules, bound to the coating by organic linkers. Bound active molecules (a form of surface modification) are used to tailor the nanoparticle for specific applications.
Stem cell tracking
The localization of stem cells has attracted increasing amounts of interest in the field of biomedical science in recent years due to the vast potential to revolutionize treatment for an extensive range of pathologies. To ensure that these treatments are a success, the fate of the stem cells, their functional capabilities, and the role that they play biologically must be determined. Labeling stem or progenitor cells with SPIONs allow their migration pattern to be non-invasively monitored in vivo with MRI. This has the possibility to help monitor stem cell therapy in the treatment of diseases such as myocardial infarctions,Citation23 neurological diseases, and cancer.Citation24 Success has recently been reported in a rat model of Huntington’s disease.Citation24 shows an MRI of SPION-labeled mesenchymal stem cells (MSCs) in vivo, which could be imaged 60 days post-implantation. The labeled MSCs showed no sign of reduced cell viability, proliferation, or differentiation and, importantly, the number of degenerating neurons was reduced. This was ascertained by using techniques developed in previous research by Jasmin et al,Citation25 which included use of a live/dead viability/toxicity kit. The results showed that while, on its own, MRI imaging can only really highlight the position of stem cells in the body, combing techniques such as computed tomography, positron emission tomography,Citation26 single-photon emission computed tomography, and optical imaging provide a wide array of data, which could be gathered to present a clearer picture of biodistribution differentiation, cell viability, and function.Citation27
Figure 3 Magnetic resonance imaging (MRI) of rats with neurodegenerative disease injected by mesenchymal stem cells labeled with magnetic nanoparticles.
Notes: In both MRI images (sagittal A and horizontal B) a dark area, identified by a white arrow, is present. This indicates a large concentration of SPION-labeled mesenchymal stem cells. Reprinted from Stem Cell Res, 9(2), Moraes L, Vasconcelos-dos-Santos A, Santana FC, et al, Neuroprotective effects and magnetic resonance imaging of mesenchymal stem cells labeled with SPION in a rat model of Huntington’s disease, 143–155, Copyright (2012), with permission from Elsevier.Citation21
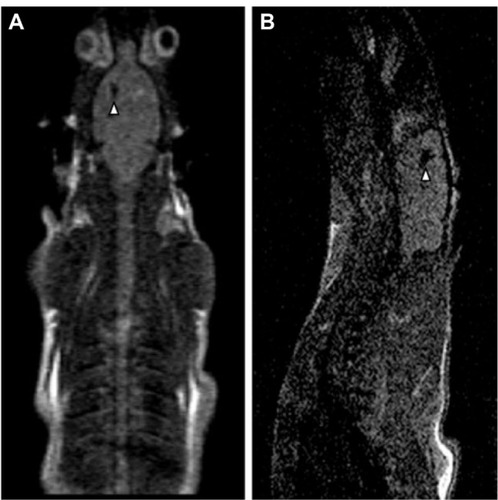
The future for the use of SPIONs in stem cell tracking is hopeful. In theory, information gained from stem cell tracking could lead to optimized dosages, preferred sites of engraftment, and specialized timing of stem cell injections,Citation28 allowing for the tailoring of treatments to individual patients.
Although the future is promising, if the tracking of SPION-labeled stem cells is to gain widespread use in clinical cellular therapies, it is essential to explore:
different coatings used to form biocompatible and long-lived SPIONs;
the synthesis of the chosen coated SPIONs;
uptake of SPIONs into specific stem cells; and
the limitations involved in SPION stem cell tracking.
In this review, we will cover these topics, providing a clear understanding of the processes involved in stem cell tracking using SPIONs.
Synthesis and labeling of magnetic iron oxide particles
Many different chemical methods for synthesizing MNPs are available, including thermal decomposition, hyperthermal, coprecipitation, solvo–thermal route, and sol–gel methods. Most commonly used is the precipitation-based approach, which is achieved either by coprecipitation or reverse micelle synthesis. This is shown in , which outlines the various research groups who showed that decomposition methods can produce MNPs with more favorable magnetic properties when compared to those formed by coprecipitation. The choice of the core of the MNP is an important factor when producing MNPs. The majority of the studies detailed in use iron oxide, due to its biocompatibility and low toxicity. Iron oxide nanoparticles without a surface coating are not viable in aqueous medium and, therefore, will readily aggregate and precipitate.
Table 1 Summary of the most common techniques used for the production of MNPs
In vivo experimental results show that particles will form aggregates in the blood, resulting in unwanted sequestration by macrophages.Citation29 In order to counteract this effect, the surface of SPIONs has been functionalized to enhance biocompatibility. details many of the coatings used in the literature and the effect that they have on the nanoparticle. The process of nanoparticle coating can be achieved by different mechanisms. Two common methods of doing so include:
Table 2 An analysis of the MNPs for their use in stem cell tracking using MRI
in situ coatings whereby the SPION is coated during the process of synthesis and post-synthesis;Citation28 and
liposome encapsulation to create magnetoliposomes, adding a more hydrophobic nature to the particle.Citation30
After coating, the SPION can be used to label the cells. This is achieved by one of two methods:
Attaching the nanoparticle to the surface of the stem cell.
Internalizing the biocompatible SPION by endocytosis or phagocytosis.
It is important to note that the first method, surface labeling, is limited to in vitro settings, as the method is generally unsuitable for use in vivo models due to clearance of the labeled cells from rapid reticuloendothelial recognition.Citation31 In the internalization method, the SPION can enter the cell via either receptor-mediated interactions or nonspecific internalization pathways.
In both cases, the nanomaterials become entrapped within the endosomes and are released into the cytoplasm. Human stem cells, such as mesenchymal stem cells, can internalize nanoparticles, even in the absence of transfection agents.Citation32 In most cases, however, the internalization of the SPION requires the use of an inactive substance that can be used as a carrier for an active ingredient, also known as an excipient.Citation15
A comparison of different coats for SPIONs
The success of labeling stem cells with SPIONs is usually advanced by encasing the nanoparticle in a biocompatible coating. The correct choice of coating will ensure stability, solubility, and prevent aggregation of the SPIONs. Often a transfection agent is used, which promotes a quicker and significantly more efficient uptake of the label into the cell. Some common examples are poly-L-lysine (PLL), sulfate, and protamine.Citation29 It is important to not only choose the correct coating, but also to use the correct concentration of the transfection agent. This is highlighted in , in which protamine is used as a transfection agent for the MNP, Feridex (Ad-Vance Magnetics Inc, Rochester, IN, USA). At a low concentration (1 μg/mL) of protamine, a lower percentage of mesenchymal stem cells are labeled than at the recommended concentration of protamine (5 μg/mL), in which 95% of the cells are labeled. After a transfection agent has been applied, the nanoparticles are incubated overnight in the appropriate stem cell medium, which facilitates a passive uptake of the nanoparticles. Notable and interesting exceptions are epithelial cell adhesion molecule beads, which utilize antigens that are specifically present on the human hepatic stem cells. Exterior attachment of the label is achieved by specific antibodies manufactured on the surface of the nanoparticle, which are complimentary to the antigen on the human hepatic stem cells. The usual process of passive uptake is often close to being 100% successful, meaning that in general, the labels are evaluated by the effect that they have on stem cells, their relaxivity, and the time in which they remain detectable by MRI.Citation33
Figure 4 Application of transfection agents to encourage a swift uptake of magnetic nanoparticles by stem cells.
Notes: Conjugating protamine to magnetic nanoparticles (Feridex; Ad-Vance Magnetics Inc, Rochester, IN, USA) significantly improved the uptake of the nanoparticles by the mesenchymal stem cells. The nuclei of the stem cells are labeled in blue, with white arrows identifying some of the magnetically labeled cells; the superparamagnetic iron oxide nanoparticles were detected using anti-dextran antibodies. The image shows the mesenchymal stem cells with protamine only (A), with magnetic nanoparticles in the absence of protamine (B), and with magnetic nanoparticles of a protamine at concentrations of 1 μg/mL (C) and 5 μg/mL (D). Scale bar is 50 μm. Springer and Methods Mol Biol, 906, 2012, 239–252, Labeling stem cells with superparamagnetic iron oxide nanoparticles: analysis of the labeling efficacy by microscopy and magnetic resonance imaging, Jasmin, Torres AL, Jelicks L, de Carvalho AC, Spray DC, Mendez-Otero R, .Citation29 With kind permission from Springer Science and Business Media.
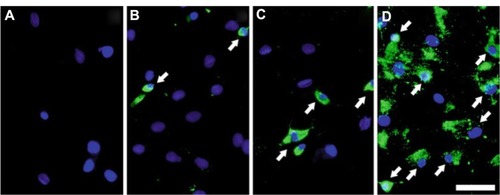
A wide array of different nanoparticles and coatings has been reported in the literature with impressive results.Citation33 For the most part, ferumoxide, ferucarbotran, and Feridex SPIONs coated with different materials (such as dextran and carboxydextran) are used, as they are approved for human use. A specific example of human use is shown in , in which neural stem cells are labeled by the nanoparticle, Feridex, which is coupled to the transfection reagent, Effectene® (Qiagen, Venlo, the Netherlands). A lesion in the left temporal lobe is highlighted with an asterisk and no signal is seen () on the MRI image before the implantation of the labeled stem cell. One day later, implantation signals were observed (), indicating the presence of the labeled neural stem cells at the legion site. A clear, concise summary of the effect of some commonly used magnetic nanoparticles is given in . The method of labeling, the percentage of the cells labeled, how long the label remains in the cell, and the effect of the presence of the label in the cell is detailed. Other nanoparticles have been tested and evaluated as MRI contrast agents (for example, gold, MnO, and CoPt); however the sheer volume of literature available regarding coated SPIONs confirms their superiority as a contrast agent in the field of stem cell tracking.
Figure 5 Magnetic resonance imaging scan of the damaged temporal lobe of a human that received neural stem cells labeled with magnetic nanoparticles.
Notes: The magnetic resonance images show pre- (A) and post-implantation (B) of neural stem cells into the temporal lobe. The stem cells are labeled with the magnetic nanoparticle, Feridex (Ad-Vance Magnetics Inc, Rochester, IN, USA), coupled to a transfection reagent. No signal was detected at the lesion, labeled with an asterisk, before the injection; however, 1 day post-injection, a dark area was seen around the lesion, indicating the presence of the magnetic-labeled neural stem cells. Copyright © 2011. John Wiley and Sons, Inc. Reproduced from Cromer Berman SM, Walczak P, Bulte JW. Tracking stem cells using magnetic nanoparticles. Wiley Interdiscip Rev Nanomed Nanobiotechnol. 2011;3(4):343–355.Citation33
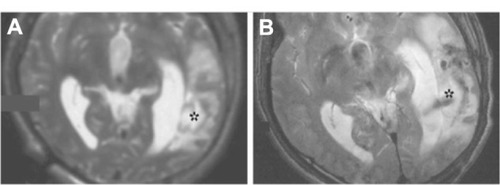
In vivo animal studies
The in vivo tracking of stem cells using SPIONs has already a markedly hypointense area in the region transplanted with SPION-labeled human umbilical cord mesenchymal stem cells and, more importantly, that the transplanted labeled cells were found to be more hypointense than non-labeled cells. It was also determined that the stem cells were accurately detectable 2 weeks after the initial transplant. It is, furthermore, important to note that both Jing et alCitation34 and Hu et alCitation35 identified that the extent of the signal intensity changes expressed by SPION-labeled stem cells was dependent on the number of labeled stem cells at any one time, and that this number could decrease significantly over time. The role of stem cells in myocardial repair following myocardial infarction or insult has yet been established.
A study by Amsalem et alCitation47 used SPIONs, coated with ferumoxide and complexed to PLL, to track MSC after being injected into the rats, 7 days after inducing a myocardial infarction. The authors found that after 1 week, 2 weeks, and 4 weeks, the MRI demonstrated well-defined hypointense areas in the rats that were injected with SPION-labeled MSCs, while control specimens injected with saline or unlabeled MSCs showed no areas of hypointensities. There was retention of the magnetic signal throughout the 4-week period, and this was comparable among both groups of rats (those that had suffered a myocardial infarction, and those that had not). Another group of researchers, Chapon et al,Citation36 used dextran-coated SPIONs to track bone marrow-derived mesenchymal stem cells to determine their effect in host cardiac tissue after a myocardial infarction. It was shown that the SPION-labeled stem cells could be tracked for up to 6 weeks after the initial stem cell injection using MRI. In addition, the study also demonstrated that there was a larger uptake of stem cells in infarcted tissue, as shown by the positron emission tomography study, in which radiola-beled glucose (2-deoxy-2-[F-18] fluoro-D-glucose [FDG]) was used to determine glucose uptake of the cells at the infarcted areas. While the study showed that despite the increased uptake of bone marrow-derived mesenchymal stem cells in the infarcted areas there was no improved left ventricular function in the rats, it did highlight the ability to track the stem cells. Although promising, some limitations and questions arose from the research concerning the best medium and cell surface coating to use in order to optimize stem cell tracking, and as such, further in vivo work would need to be conducted.
Further research conducted by Delcroix et alCitation37 in 2009 investigated how stem cell tracking in neural migratory pathways could be potentially used for clinical therapy. The researchers used SPIONs, which were coated with 1-hydroxyethylidene-1.1-bisphosphonic acid to label rat mesenchymal stem cells.Citation37 It had already been established that in an appropriate environment, which contains particular matrix molecules and growth factors, mesenchymal stem cells were able to transdifferentiate into neural cells in vitro, though very little work had been done in vivo. Delcroix et alCitation37 incubated the rat mesenchymal stem cells (rMSCs) for 48 hours with 50 ug/mL of 1-hydroxyethylidene-1. 1-bisphosphonic acid (HEDP)-coated SPIONs. They established that there was no effect or change in the morphology or viability of the rMSCs. At this point, it was discovered that by using an MRI, there was a long distance of migration in rMSC from the subventricular zone to the olfactory bulb via the rostral migratory system. The value of the research conducted by Delcroix et alCitation37 demonstrated for the first time that neural migratory pathways can be established and mapped out using SPIONs. The importance of identifying stem cell migratory mechanisms in response to injury could eventually lead to the use of MSCs in brain cell therapy, and it further establishes that additional research is required as to why, despite in vitro models suggesting that transdifferentiation of MSCs into neural cells, this is not the case in vivo. There is considerable promise and established research that demonstrates that SPIONs have an exciting role in stem cell tracking by non-invasive mechanisms which could, in time, lead to deeper appreciation of tissue regeneration pathways and future clinical applications.
Human studies
There is a great potential role for the in vivo, non-invasive tracking of transplanted human stem cells, as there is scope for obtaining real-time insights into underlying physiological and cellular responses of stem cells in both normal functioning and pathological injury. As was discussed with the animal studies, understanding migratory pathways and the manner in which different stem cells differentiate to site-specific cells can be crucial in the development of clinical therapies. In order for long-term clinical therapies to be introduced, there is a necessary evolution in research that has to occur, as animal models need to progress to human models before clinical trials can take place.
It is important to take into account the variable factors that make clinical trials on humans more challenging than simply repeating an experiment, as deduced from research on animal models. For example, the organism’s weight, blood volume, cardiac output, and intra- as well as inter-cellular microenvironments are markedly different from one animal to another; as such, human studies would require careful planning and assurance of safe testing before moving ahead.Citation38 Although only a few studies have been done, research has indeed showed that there is effective non-invasive tracking of labeled human embryonic stem cells using MRI imaging. The first study that attempted clinical MRI tracking of therapeutic cells in humans was first reported in 2005.Citation39 The researchers co-cultured immature dendritic cells with 200 μg/ml SPION.Citation39 They confirmed that the dendritic cells had taken up a significant amount of SPION before the end of the culture period. Following intranodal injection into eight grade 3 melanoma patients, 3T MRI along with scintigraphy was used to image the injected cells. The results showed that MRI imaging was at least as sensitive an imaging modality as scintigraphy, and it also managed to track the cells with a higher degree of accuracy due to its superior spatial resolution. de Vries et alCitation39 noted in their work that 50% of the ultrasound-guided injections of stem cells were misadministered into nodes adjacent to the target lymph node and, importantly, this was only revealed on subsequent MRI imaging. Identifying this error, however, showed that SPION-labeled cells could be tracked using MRI, and they could also be used to ensure that cells are injected or delivered to the correct target site. In 2008, Zhu et alCitation40 conducted a case study on two patients: one of which was a 34-year-old man who had brain trauma in his left temporal lobe in 2004; and a control 42-year-old male who had brain trauma in his right temporal lobe. Neural tissue from both patients was taken during emergency operations, and the test patient had his neural tissue incubated with Feridex SPIONs and a lipofection transfection agent.Citation40 The SPION-labeled neural stem cells were then injected around the region of the brain damage and 3T MRI was performed at 24 hours, then every 7 days after transplantation for 10 weeks. Before implantation, upon T2 weighted MRI imaging, no pronounced hypointense signals were found, although the injection sites themselves produced a circular area of dark tissue on imaging. This hypointense signal at the injec tion sites faded after a week or so. In terms of the injected cells themselves, a hypointense signal was seen around the border of the damaged tissue in the temporal lobe, and it intensified at around weeks 2 and 3, before eventually fading after 7 weeks, most likely due to dilution of the SPIONs in response to cell proliferation around the site of the lesion. In the control patient, however, there was no change in the signal on MRI during the observation over the trial period, although there was a slight hypointense signal at the injection sites. The researchers concluded that stem cell migration after implantation could indeed be detected via non-invasive methods using SPIONs and MRI.
As of yet, not many human subject studies have been done using SPIONs, but even from the brief research that has been conducted, there seems to be legitimate and effective results showing the promising use of SPIONs in stem cell tracking using non-invasive mechanisms, such as MRI. Of course, dependent on the progress of work in animal models, human studies can progress. Much will still need to be done to obviate the use of transfection agents, which may be attributed to cell death, and to assess the most appropriate and suitable method of labeling stem cells.
The limitations of MRI and the use of SPIONs
The limitations of MRI are well illustrated in their use of stem cell tracking. First, due to cell division, the SPION-labeled cells become diluted in a localized site and, consequently, the generated image is weakened or distorted. This has been seen in neural stem cells where the concentration of SPIONs was shown to decrease by 50% every 3 days in vitro. Second, due to the in vivo migration of SPION-labeled stem cells, the density of the cells is considerably reduced over time, again leading to a weakening of the MRI signal.Citation24 Third, SPION cell labeling itself cannot determine if or what the SPION-labeled stem cell differentiates into, although through the use of combined imaging techniques such as immunohistochemistry and confocal microscopy, the function of the stem cell can be ascertained.Citation41 Fourth, most studies utilizing MRI are conducted with a 1.5T MRI unit, which has a limited spatial resolution and does not allow for deep imaging. An improvement in spatial resolution can only be achieved through the use of stronger magnetic fields, and it is still unknown what effect this would have upon the cells.Citation42 Fifth, SPIONs are not detected directly; rather, they are detected through indirect microscopic disturbances in the magnetic field. As a result of this, it proves harder to correlate the magnetic resonance signal with the number of cells present.Citation43 Another commonly encountered problem is that if cells die, they will still be imaged by MRI, as the SPIONs will remain inside or attached to the cell. This invariably leads to an inaccurate analysis of the MRI image, especially since it is likely that these dead cells are engulfed by macrophages, which would lead to contrast agents being present in the lymph nodes.
SPIONs come with an inherent risk of toxicity, as is the case with any foreign particle or chemical that is injected or administered within an organism. SPIONs are composed of biodegradable iron, which could potentially be recycled by cells within the body that use biochemical pathways for iron metabolism. This would lead to a situation of iron-mediated tive oxygen species.Citation44 The cytotoxicity of SPIONs has been explored in human mesenchymal stem cells,Citation42 mouse embryonic stem cells,Citation23 and neural stem cellsCitation45,Citation46 by a variety of researchers. It was discovered that on the whole, the internalization of the nanoparticle into these stem cells did not affect cell growth or differentiation or, indeed, its viability to survive. However, there has been evidence to suggest that endocytic lifecycles can be affected due to nanoparticles residing in endosomes.Citation45 Studies were undertaken, which showed that it is the iron itself within the SPION that caused impairment in chondrogenic differentiation, as opposed to any transfection factors that were present;Citation46–Citation48 however, other research shows that it is the transfection agent, PLL, that causes this effect on differentiation (for more toxicity information, see ). The application of SPIONs in medicine brings a new dimension into monitoring stem cells in vitro and in vivo. It will allow for groundbreaking research or therapeutic achievements. However, it is important that with the huge potential of this ability to manipulate size, shape, charge, or surface modification, detailed studies of toxicity are carried out and cell damage with SPIONs checked before clinical application. For example, configuration of SPIONs into different shapes such as spheres, nanoworms, rod shapes, or beads for different functional applications may increase toxicity. This was demonstrated with mouse L929 fibroblast cells; there was significant increased toxicity, despite being at the same molarity as other configurations, when the cells were exposed to nanobeads, nanoworms, or nanospheres.Citation49 This helps to highlight different physicochemical properties such as final size, shape, and charge, which will affect the viability of SPIONs. As such, it is imperative that further detailed toxicity testing is undertaken to assess SPION toxicity and to be aware that new surface modification may alter the toxicity of the nanoparticles.
Conclusion
Stem cell therapy has boundless scope and prospects for therapeutic and clinical use. In this review, we have focused on stem cell tracking using SPIONs. We have discussed and evaluated the way in which SPIONS are synthesized, coated, and targeted for the use in stem cell tracking. Further to this, we have evaluated and discussed the current research published pertaining to stem cell tracking in both animal and human studies. Researchers across the globe are continuously working on ways to improve the techniques already in place, and to invent ways to combat limitations. It is, however, a new technology that requires further research and understanding. Many different types of peptides and different chemical coatings can be conjugated with SPIONs, and greater insights and understandings of the complex mechanisms that govern human functions are required to maximize the potential of the coatings. It is also still not fully clear which cytotoxic and hazardous effects the nanoparticles induce; consequentially, it will take time to allow them to be used in a clinical scenario. A greater understanding of the methods and strategies used to produce SPIONs with greater specificity and sensitivity are also needed. In summary, much work is still required in order to bring SPIONs into a clinical environment. Our understanding of many of the physiological mechanisms in the body has improved, our knowledge concerning the biology of stem cells has increased, and if research continues effectively, there is no doubt that nanoparticles, including SPIONS, will revolutionize clinical medicine.
Disclosure
The authors report no conflicts of interest in this work.
References
- BeckerAJMcCullochEATillJECytological demonstration of the clonal nature of spleen colonies derived from transplanted mouse marrow cellsNature196319745245413970094
- GhanbariHde MelASeifalianAMCardiovascular application of polyhedral oligomeric silsesquioxane nanomaterials: a glimpse into prospective horizonsInt J Nanomedicine2011677578621589645
- DeansRJMoseleyABMesenchymal stem cells: biology and potential clinical usesExp Hematol200028887588410989188
- de MelAMuradFSeifalianAMNitric oxide: a guardian for vascular grafts?Chem Rev201111195742576721663322
- BangOYLeeJSLeePHLeeGAutologous mesenchymal stem cell transplantation in stroke patientsAnn Neurol200557687488215929052
- HorwitzEMProckopDJGordonPLClinical responses to bone marrow transplantation in children with severe osteogenesis imperfectaBlood20019751227123111222364
- CalleraFde MeloCMMagnetic resonance tracking of magnetically labeled autologous bone marrow CD34+ cells transplanted into the spinal cord via lumbar puncture technique in patients with chronic spinal cord injury: CD34+ cells’ migration into the injured siteStem Cells Dev200716346146617610376
- WollertKCMeyerGPLotzJIntracoronary autologous bone-marrow cell transfer after myocardial infarction: the BOOST randomised controlled clinical trialLancet2004364942914114815246726
- PengXHQianXMaoHTargeted magnetic iron oxide nanoparticles for tumor imaging and therapyInt J Nanomedicine20083331132118990940
- MadaniSYNaderiNDissanayakeOTanASeifalianAMA new era of cancer treatment: carbon nanotubes as drug delivery toolsInt J Nanomedicine201162963297922162655
- TanAYildirimerLRajadasJDe La PeñaHPastorinGSeifalianAQuantum dots and carbon nanotubes in oncology: a review on emerging theranostic applications in nanomedicineNanomedicine (Lond)2011661101111421955079
- LaConteLNitinNBaoGMagnetic nanoparticle probesMater Today200585 Suppl 13238
- ReimerPBalzerTFerucarbotran (Resovist): a new clinically approved RES-specific contrast agent for contrast-enhanced MRI of the liver: properties, clinical development, and applicationsEur Radiol20031361266127612764641
- NeubergerTSchöpfBHofmannHHofmannMvon RechenbergBSuperparamagnetic nanoparticles for biomedical applications: possibilities and limitations of a new drug delivery systemJ Magn Magn Mater20052931483496
- LaiCHYenTCNgKKMolecular imaging in the management of cervical cancerJ Formos Med Assoc2012111841242022939658
- LinMMKim doKEl HajAJDobsonJDevelopment of superparamagnetic iron oxide nanoparticles (SPIONS) for translation to clinical applicationsIEEE Trans Nanobioscience20087429830519203873
- GindyMEPrud’hommeRKMultifunctional nanoparticles for imaging, delivery and targeting in cancer therapyExpert Opin Drug Deliv20096886587819637974
- RiceHEHsuEWShengHSuperparamagnetic iron oxide labeling and transplantation of adipose-derived stem cells in middle cerebral artery occlusion-injured miceAJR Am J Roentgenol200718841101110817377054
- HarisinghaniMGBarentszJHahnPFNoninvasive detection of clinically occult lymph-node metastases in prostate cancerN Engl J Med2003348252491249912815134
- RogersWJBasuPFactors regulating macrophage endocytosis of nanoparticles: implications for targeted magnetic resonance plaque imagingAtherosclerosis20051781677315585202
- MoraesLVasconcelos-dos-SantosASantanaFCNeuroprotective effects and magnetic resonance imaging of mesenchymal stem cells labeled with SPION in a rat model of Huntington’s diseaseStem Cell Res20129214315522742973
- JungCWJacobsPPhysical and chemical properties of superparamagnetic iron oxide MR contrast agents: ferumoxides, ferumoxtran, ferumoxsilMagn Reson Imaging19951356616748569441
- AraiTKofidisTBulteJWDual in vivo magnetic resonance evaluation of magnetically labeled mouse embryonic stem cells and cardiac function at 1.5 tMagn Reson Med200655120320916315206
- GuzmanRUchidaNBlissTMLong-term monitoring of transplanted human neural stem cells in developmental and pathological contexts with MRIProc Natl Acad Sci U S A200710424102111021617553967
- JasminTorresALNunesHMOptimized labeling of bone marrow mesenchymal cells with superparamagnetic iron oxide nanoparticles and in vivo visualization by magnetic resonance imagingJ Nanobiotechnology20119421542946
- HiguchiTAntonMDumlerKCombined reporter gene PET and iron oxide MRI for monitoring survival and localization of transplanted cells in the rat heartJ Nucl Med20095071088109419525455
- WellingMMDuijvesteinMSignoreAvan der WeerdLIn vivo biodistribution of stem cells using molecular nuclear medicine imagingJ Cell Physiol201122661444145221413018
- BerryCCWellsSCharlesSAitchisonGCurtisASCell response to dextran-derivatised iron oxide nanoparticles post internalisationBiomaterials200425235405541315130725
- JasminTorresALJelicksLde CarvalhoACSprayDCMendez-OteroRLabeling stem cells with superparamagnetic iron oxide nanoparticles: analysis of the labeling efficacy by microscopy and magnetic resonance imagingMethods Mol Biol201290623925222791437
- De CuyperMJoniauMMagnetoliposomes. Formation and structural characterizationEur Biophys J19881553113193366097
- SykováEJendelováPMagnetic resonance tracking of implanted adult and embryonic stem cells in injured brain and spinal cordAnn N Y Acad Sci2005104914616015965114
- LalandeCMirauxSDerkaouiSMMagnetic resonance imaging tracking of human adipose derived stromal cells within three-dimensional scaffolds for bone tissue engineeringEur Cell Mater20112134135421484704
- Cromer BermanSMWalczakPBulteJWTracking stem cells using magnetic nanoparticlesWiley Interdiscip Rev Nanomed Nanobiotechnol20113434335521472999
- JingXHYangLDuanXJIn vivo MR imaging tracking of magnetic iron oxide nanoparticle labeled, engineered, autologous bone marrow mesenchymal stem cells following intra-articular injectionJoint Bone Spine200875443243818448377
- HuSLLuPGZhangLJIn vivo magnetic resonance imaging tracking of SPIO-labeled human umbilical cord mesenchymal stem cellsJ Cell Biochem201211331005101222065605
- ChaponCJacksonJSAboagyeEOHerlihyAHJonesWABhakooKKAn in vivo multimodal imaging study using MRI and PET of stem cell transplantation after myocardial infarction in ratsMol Imaging Biol2009111313818773246
- DelcroixGJJacquartMLemaireLMesenchymal and neural stem cells labeled with HEDP-coated SPIO nanoparticles: in vitro characterization and migration potential in rat brainBrain Res20091255183119103182
- MahmoudiMHosseinkhaniHHosseinkhaniMMagnetic resonance imaging tracking of stem cells in vivo using iron oxide nanoparticles as a tool for the advancement of clinical regenerative medicineChem Rev2011111225328021077606
- de VriesIJLesterhuisWJBarentszJOMagnetic resonance tracking of dendritic cells in melanoma patients for monitoring of cellular therapyNat Biotechnol200523111407141316258544
- ZhuJZhouLXingWuFTracking neural stem cells in patients with brain traumaN Engl J Med2006355222376237817135597
- ArbabASPanditSDAndersonSAMagnetic resonance imaging and confocal microscopy studies of magnetically labeled endothelial progenitor cells trafficking to sites of tumor angiogenesisStem Cells200624367167816179427
- HsiaoJKTaiMFChuHHMagnetic nanoparticle labeling of mesenchymal stem cells without transfection agent: cellular behavior and capability of detection with clinical 1.5 T magnetic resonance at the single cell levelMagn Reson Med200758471772417899592
- BulteJWHot spot MRI emerges from the backgroundNat Biotechnol200523894594616082363
- HoepkenHHKortenTRobinsonSRDringenRIron accumulation, iron-mediated toxicity and altered levels of ferritin and transferrin receptor in cultured astrocytes during incubation with ferric ammonium citrateJ Neurochem20048851194120215009675
- ArbabASWilsonLBAshariPJordanEKLewisBKFrankJAA model of lysosomal metabolism of dextran coated superparamagnetic iron oxide (SPIO) nanoparticles: implications for cellular magnetic resonance imagingNMR Biomed200518638338916013087
- BulteJWKraitchmanDLMackayAMPittengerMFChondrogenic differentiation of mesenchymal stem cells is inhibited after magnetic labeling with ferumoxidesBlood20041041034103412 author reply 341215525839
- AmsalemYMardorYFeinbergMSIron-oxide labeling and outcome of transplanted mesenchymal stem cells in the infarcted myocardiumCirculation2007116Suppl 11I38I4517846324
- RiclesLMNamSYSokolovKEmelianovSYSuggsLJFunction of mesenchymal stem cells following loading of gold nanotracersInt J Nanomedicine2011640741621499430
- MahmoudiMSimchiAImaniMA new approach for the in vitro identification of the cytotoxicity of superparamagnetic iron oxide nanoparticlesColloids Surf B Biointerfaces201075130030919781921
- ZhaiYHanLWangPSuperparamagnetic plasmonic nanohybrids: shape-controlled synthesis, TEM-induced structure evolution, and efficient sunlight-driven inactivation of bacteriaACS Nano20115118562857021951020
- HariharanVRadhakrishnanSParthibavarmanMDhilipkumarRSekarCSynthesis of polyethylene glycol (PEG) assisted tungsten oxide (WO3) nanoparticles for L-dopa bio-sensing applicationsTalanta20118542166217421872074
- YasenevaPBowkerMHutchingsGStructural and magnetic properties of Zn-substituted cobalt ferrites prepared by co-precipitation methodPhys Chem Chem Phys20111341186091861421952718
- El-SherbinyIMSmythHDSmart magnetically responsive hydrogel nanoparticles prepared by a novel aerosol-assisted method for biomedical and drug delivery applicationsJ Nanomater20112011201111321808638
- KimHMLeeHHongKSSynthesis and high performance of magnetofluorescent polyelectrolyte nanocomposites as MR/near-infrared multimodal cellular imaging nanoprobesACS Nano20115108230824021932788
- RenJShenSPangZLuXDengCJiangXFacile synthesis of super-paramagnetic Fe3O4@Au nanoparticles for photothermal destruction of cancer cellsChem Commun (Camb)20114742116921169421952492
- ZhouSChenQSynthesis and characterization of bracelet-like magnetic nanorings consisting of Ag-Fe3O4 bi-component nanoparticlesDalton Trans201140348622862921799984
- NiuHZhangDZhangSZhangXMengZCaiYHumic acid coated Fe3O4 magnetic nanoparticles as highly efficient Fenton-like catalyst for complete mineralization of sulfathiazoleJ Hazard Mater20111901–355956521514993
- MoonJWRawnCJRondinoneAJLarge-scale production of magnetic nanoparticles using bacterial fermentationJ Ind Microbiol Biotechnol201037101023103120544257
- NidhinMIndumathyRSreeramKJNairBUSynthesis of iron oxide nanoparticles of narrow size distribution on polysaccharide templatesBulletin of Material Science20083119396
- DuLChenJQiYPreparation and biomedical application of a non-polymer coated superparamagnetic nanoparticleInt J Nanomedicine20072480581218203447
- YuWWFalknerJCYavuzCTColvinVLSynthesis of monodisperse iron oxide nanocrystals by thermal decomposition of iron carboxylate saltsChem Commun (Camb)2004202306230715489993
- JiangWYangHCYangSYPreparation and properties of superparamagnetic nanoparticles with narrow size distribution and biocompatibleJ Magn Magn Mater20042832–3210214
- GuptaAKWellsSSurface-modified superparamagnetic nanoparticles for drug delivery: preparation, characterization, and cytotoxicity studiesIEEE Trans Nanobioscience200431667315382647
- McClellandRWauthierETallhedenTReidLMHsuEIn situ labeling and magnetic resonance imaging of transplanted human hepatic stem cellsMol Imaging Biol201113591192220890665
- van BuulGMKotekGWielopolskiPAClinically translatable cell tracking and quantification by MRI in cartilage repair using superparamagnetic iron oxidesPLoS One201162e1700121373640
- KimTMominEChoiJMesoporous silica-coated hollow manganese oxide nanoparticles as positive T1 contrast agents for labeling and MRI tracking of adipose-derived mesenchymal stem cellsJ Am Chem Soc201113392955296121314118
- MengXSetonHCLu leTPriorIAThanhNTSongBMagnetic CoPt nanoparticles as MRI contrast agent for transplanted neural stem cells detectionNanoscale20113397798421293831
- HenningTDSuttonEJKimAThe influence of ferucarbotran on the chondrogenesis of human mesenchymal stem cellsContrast Media Mol Imaging20094416517319670250
- WangHHWangYXLeungKCDurable mesenchymal stem cell labelling by using polyhedral superparamagnetic iron oxide nanoparticlesChemistry20091545124171242519834937
- ReddyAMKwakBKShimHJIn vivo tracking of mesenchymal stem cells labeled with a novel chitosan-coated superparamagnetic iron oxide nanoparticles using 3.0T MRIJ Korean Med Sci201025221121920119572
- LoebingerMRKyrtatosPGTurmaineMMagnetic resonance imaging of mesenchymal stem cells homing to pulmonary metastases using biocompatible magnetic nanoparticlesCancer Res200969238862886719920196
- LeeESChanJShuterBMicrogel iron oxide nanoparticles for tracking human fetal mesenchymal stem cells through magnetic resonance imagingStem Cells20092781921193119544438