Abstract
Background
Long-term use of morphine induces antinociceptive tolerance and limits its clinical efficacy. Neuroinflammation in the spinal cord is thought to play a pivotal role in the development of morphine tolerance. Toll-like receptor 4 (TLR4) and P2X7 receptor (P2X7R) are key modulators of neuroinflammation. Recent studies show that the Nod-like receptor protein 3 (NLRP3) inflammasome play a crucial role in microglia-mediated neuroinflammation. Thus far, the mechanism underlying NLRP3 inflammasome activation during morphine-induced tolerance is not yet fully understood. Therefore, we sought to investigate the mechanisms of NLRP3 inflammasome activation and its role in the development of morphine-induced tolerance.
Methods
Repeated morphine treatment through intrathecal injection (15 μg once daily for 7 days) was given to establish antinociceptive tolerance in mice. Tail-flick latency was used to evaluate morphine-induced antinociception. NLRP3 knockout mice were used to assess the role of NLRP3 inflammasome in morphine tolerance. TLR4 knockout mice and A438079, a P2X7R antagonist, were used to assess the role of TLR4 and P2X7R in chronic morphine-induced NLRP3 inflammasome activation. Western blot and immunofluorescence were used for quantitative comparison.
Results
Repeated morphine treatment increased the expression of NLRP3. Knockout of NLRP3 attenuated morphine-induced tolerance and suppressed morphine-induced activation of microglia. Knockout of TLR4 alleviated morphine tolerance and chronic morphine-induced upregulation of spinal NLRP3. Inhibition of spinal P2X7R with A438079 not only prevented the development of morphine-induced tolerance but also inhibited repeated morphine treatment-induced upregulation of spinal NLRP3. Furthermore, spinal NLRP3, TLR4 and P2X7R were collectively colocalized with the microglia marker Iba1.
Conclusion
This study demonstrates that the NLRP3 inflammasome in microglia plays a crucial role in morphine tolerance and that both TLR4- and P2X7R-dependent pathways are required for NLRP3 inflammasome activation over the course of the development of morphine-induced tolerance. Our results provide a new perspective for the targeted treatment of morphine-induced tolerance.
Introduction
Morphine, as a classic opioid analgesic, is a mainstay in the management of moderate to severe pain throughout the world. However, the long-term use of morphine causes antinociception tolerance and reduces its clinical efficacy. The mechanisms associated with morphine tolerance are complicated, including the down-regulation and internalization of opioid receptors,Citation1 the ion channels,Citation2 and the activation of excitatory amino acid receptors,Citation3 and others. Recently, microglia have emerged as a key target for morphine-induced antinociceptive tolerance. Studies showed that microglia play an important role in the development and maintenance of morphine-induced antinociceptive tolerance,Citation4 and administration of microglial inhibitors may significantly reduce morphine-induced tolerance.Citation5–Citation7 However, the key cellular substrates and processes that increase microglial reactivity in response to opioid treatment remain an important unresolved question.
The Nod-like receptor protein 3 (NLRP3) inflammasome has been demonstrated to be crucial for the regulation of microglia-mediated neuroinflammation.Citation8,Citation9 It consists of a cytosolic multiprotein complex, composed of NLRP3, apoptosis-associated speck-like protein (ASC), and procaspase-1, and can be activated by a wide range of exogenous and endogenous stimuli.Citation10 The activation of the NLRP3 inflammasome involves a two-step process. The priming signal (signal 1) is at the transcriptional level. Stimuli such as toll-like receptors ligandsCitation11 activate the transcription factor NF-κB and enhance the transcription of NLRP3 and interleukin 1 βeta (IL-1β). The activation signal (signal 2) promotes the oligomerization of NLRP3 with ASC and pro-caspase 1, and subsequently leads to the conversion of pro-IL-1β to IL-1β. Adenosine triphosphate (ATP),Citation12 K+ ionophores,Citation13 and other factorsCitation14 are reported to participate in signal 2. It was reported that the inhibition of the NLRP3 inflammasome suppressed the activation of microglia and alleviated morphine antinociception tolerance.Citation15 However, the mechanism underlying NLRP3 inflammasome activation during morphine-induced antinociceptive tolerance is not yet fully understood.
Toll-like receptor 4 (TLR4), as an anti-inflammatory pattern-recognition receptor, contributes to the development of morphine tolerance.Citation16,Citation17 Both in vivo and in vitro studies have confirmed that the proinflammatory effects of repeated morphine were mediated by TLR4 in microglia and astrocytes.Citation1 Morphine binds to myeloid differentiation protein 2 (MD-2), an accessory protein of TLR4, and mediates the activation of microglia, resulting in the robust release of inflammatory cytokines, including tumor necrosis factor (TNF), IL-1β, and Interleukin 6 (IL-6).Citation18,Citation19 In addition, systemic TLR4 antagonists attenuate morphine-induced tolerance.Citation16 Furthermore, in other inflammatory disease models,Citation20,Citation21 TLR4 acts as the upstream of NLRP3 inflammasome. Therefore, we assessed whether the activation of the NLRP3 inflammasome is dependent on TLR4 during the development of morphine-induced antinociceptive tolerance.
The P2X7 receptor (P2X7R) is an ATP-gated nonselective cation channel receptor that has have been demonstrated to play key roles in numerous inflammatory diseases of the central nervous system.Citation22,Citation23 A recent study demonstrated that repeated morphine treatment potentiates P2X7R expression and function in microglia, and that the Y382-384 site in the P2X7R intracellular C-terminal domain gates morphine antinociception tolerance.Citation2 When the ATP binds to P2X7R, the ion channel on the cell membrane opens and leads the influx of Na+ and Ca2+ and the efflux of K+.Citation24 Interestingly, K+ efflux, Ca2+ mobilization, and Na+ influx are implicated in activating the NLRP3 inflammasome.Citation25 Hence, we sought here to investigate the relationship between P2X7R and NLRP3 inflammasome activation in the development of morphine-induced antinociceptive tolerance.
In this study, we investigated the role of the NLRP3 inflammasome in the development of morphine-induced antinociception tolerance. We also evaluated the effect of TLR4 and P2X7R in inflammasome activation during the development of morphine-induced antinociceptive tolerance.
Materials and Methods
Animals
Male C57BL/6 mice (8–10-weeks-old; 20–25 g) were purchased from Fudan University Medical Animal Center, Shanghai, China. NLRP3 knockout mice and TLR4 knockout mice were obtained from Dr. Xu at Shanghai East Hospital and bred in our lab. Control mice in each test consisted of age-matched littermates of the knockout animals. Mice were housed such that they were 5 per cage and maintained in animal care quarters at a temperature of 23 ± 2°C, with a relative humidity of 55 ± 5%, and according to a 12-hour light/dark cycle. Food and water were freely available. The protocol was approved by the Animal Care and Use Committee of the Shanghai Sixth People’s Hospital Affiliated to Shanghai Jiao Tong University [SYXK (Shanghai, China) 2011–0128, January 1, 2011] and followed the ARRIVE guidelines for reporting experiments involving animals.Citation26 All animals were treated humanely, and all efforts were made to minimize animal suffering and the number of experimental animals.
Drugs and Injections
Morphine hydrochloride (Shenyang First Pharmaceutical Factory, China) was diluted with saline (Baxter Healthcare, USA), and injected (15 μg in 5 μL, i.t.) daily for 7 days. To test the role of P2X7R in morphine-induced antinociceptive tolerance, A438079, the P2X7R antagonist (A9736, Sigma-Aldrich, USA), was dissolved with 25% dimethyl sulfoxide (DMSO) (Sigma-Aldrich, USA) and injected (80 mg/kg, i.p.) 30 min before each morphine administration.Citation27 Thirty minutes after the injection, morphine-induced antinociception was assessed. On day 8, the dose-responses of morphine were determined. Increasing doses of morphine (1, 2, 4, 8.16, 32 ug) were injected intrathecally every 1 h, and nociception was assessed 30min after drug administration using a tail-immersion test. When maximal anti-nociception was obtained, the process was stopped.
Behavioral Nociceptive Assay
A behavioral assay was performed 30 min after morphine administration by the tail-immersion test every morning during the treatment course. In brief, the mouse was gently restrained and 2–3 cm of the tip of the tail was submerged in the water bath, which was temperature at 52.5 ± 0.5 °C by a thermostatic regulator (Shanghai Thermo Factory Co., China). A 10-second cut-off value was set to avoid tissue damage. The latency of the tail withdrawal was recorded. Baseline latencies were measured before the daily treatment.
Western Blotting
After 7 consecutive days of drug treatment, mice were sacrificed under an overdose of pentobarbital intraperitoneal injection (50 mg/kg. i.p.), and the lumber section of the spinal cords were rapidly removed and homogenized in ice-cold RIPA buffer (Thermo Fisher Scientific, USA) supplemented with protease and phosphatase inhibitors (Thermo Fisher Scientific, USA). The lysates were then centrifuged at 12,000× g for 15 min at 4°C to isolate the proteins. The protein concentrations of the samples were determined with a BCA protein assay kit (Beyotime, China). Samples containing 50 μg protein were denatured by heating at 95°C for 5 min, separated on 10% SDS–polyacrylamide gels, and transferred to polyvinylidene fluoride (PVDF) membranes (Millipore, USA). The membranes were blocked with 5% non-fat milk in Tris-buffered saline with Tween (TBST) for 2 h at room temperature and incubated overnight at 4°C with the following primary antibodies: Recombinant Rabbit polyclonal NLRP3 antibody (ET1610-93, 1:1000, HuaBio, China); mouse polyclonal TLR4 antibody (sc-293,072,1:1000, Santa Cruz, USA); rabbit polyclonal P2X7 antibody (P8232, 1:500, Sigma-Aldrich, USA); mouse anti-β-actin (EM2001-07, 1:5000, HuaBio, China); mouse monoclonal GAPDH (EM1101, 1:5000, HuaBio, China). After washing with TBST, the membranes were incubated for 1 h at room temperature with horseradish peroxidase-conjugated goat anti-rabbit or anti-mouse IgG secondary antibody (1:5000, HuaBio, China). Next, the protein bands were detected by Image Quant Ai600 (General Electric Co., USA) with an enhanced ECL substrate (Thermo Fisher Scientific). The results were analyzed and quantified using ImageJ software (version 2.0.0, USA).
Immunofluorescence
After 7 consecutive days of drug treatment, the mice were anaesthetized with pentobarbital (50 mg/kg, i.p.) and transcardially perfused with 4% cold paraformaldehyde, after which the lumbar spinal cords were excised and fixed in 4% paraformaldehyde overnight. Next, the spinal cords were immersed in ascending sucrose solutions (10%, 2030%) overnight for dehydration, then cut into 15-μm sections with a cryostat. Next, frozen sections were incubated in blocking solution with 10% goat serum for 2 h at room temperature and incubated overnight at 4°C with the following primary antibodies: mouse polyclonal NLRP3 antibody (AG20B-0014-C100, 1:200, AdipoGen, USA); mouse polyclonal TLR4 antibody (sc-293,072, 1:200, Santa Cruz, USA); rabbit polyclonal P2X7 antibody (P8232, 1:50, Sigma-Aldrich, USA); rabbit polyclonal Iba1 Antibody (019–19,741, 1:200, Wako, Japan); mouse monoclonal Iba1 antibody (RT1316, 1:200, HuaBio, China) with the tissue sections in 1% normal goat serum and 0.01% Triton-X-100 (Sigma-Aldrich, USA). The sections were then washed and incubated with appropriate fluorescent secondary antibodies (1:500, Alexa Fluor 488 or 567; Invitrogen, USA) for 1 h at room temperature. Next, the sections were incubated with DAPI (F6057, Sigma-Aldrich, USA) for 5 min. Images were acquired using a confocal microscope (FV1000; Olympus, Tokyo, Japan).
Statistical Analysis
All data are presented as the mean ± SEM and were analyzed with GraphPad Prism 7 (GraphPad Software, San Diego, CA, USA). The behavioral latencies were expressed as a percentage of the maximum possible effect (%MPE), defined as: %MPE= 100×(response latency-baseline latency)/(cut-off time-baseline latency). Tail-flick latencies were analyzed using a two-way (time and treatment) analysis of variance (ANOVA), followed by Tukey’s test for multiple comparisons. Unpaired Student’s t-tests were used for comparisons between two groups. The analysis of immunoreactivity was carried out using a one-way ANOVA. For presentation of all statistical analyses, *** indicates p < 0.001, **p < 0.01, *p < 0.05, and p < 0.05 was considered to indicate statistical significance. Observers who performed the behavioral tests, protein quantitation and all data analysis were blinded to group allocation.
Results
Repeated Morphine Treatment Induced Antinociceptive Tolerance Accompanied by an Upregulation of NLRP3 and Microglial Activation
Repeated administration of morphine (15 μg, i.t.) daily for 7 days () induced an obvious antinociceptive tolerance (; ANOVA with Tukey’s test, F(1, 20) = 874.6; day 1:+30 min (MOR group) vs day 7:+30 min (MOR group); p < 0.0001). The tail-flick latency was tested before and 30 min after intrathecal injection. Treatment with morphine induced an acute increase in tail-flick latency. However, this antinociceptive effect was reduced after repeated intrathecal morphine injections (). In the morphine group, there was a significant increase in NLRP3 protein expression within the lumbar spinal cord (; unpaired t-test, t=3.4; p = 0.0068, compared with the control group). Additionally, the immunofluorescence staining data showed that the microglia were activated (with Iba1 as a microglia marker) in the lumbar spinal cord of the morphine group (; unpaired t-test, t=6.289; p <0.001, compared with the control group).
Figure 1 Repeated morphine treatment induced antinociceptive tolerance accompanied by an upregulation of NLRP3 and the activation of microglia. (A) Schematic representation of the experimental timeline. Mice received morphine (15 μg, i.t.) or normal saline (NS) (15 μL, i.t.) daily for 7 days. On each day, the baseline thermal threshold (BL) before treatment and a threshold of 30 min (+30 min) after treatment were tested by a tail-immersion test. (B) The withdrawal latency in the tail-immersion test before and 30 min after treatment. (C) The %MPE of mice treated with saline (CON) and morphine (MOR) on day 1 and day 7, ***p < 0.001. (D) Expression of NLRP3 of mice treated with saline and morphine. Columns and errors are presented as mean ± SEM. n = 6, **p<0.01. (E) Immunofluorescence of Iba1 (red) in the dorsal horn of the spinal cord of mice treated with saline (CON) and MOR. Scale bar = 50 μm. Columns and errors are presented as mean ± SEM; n = 7, ***p<0.001.
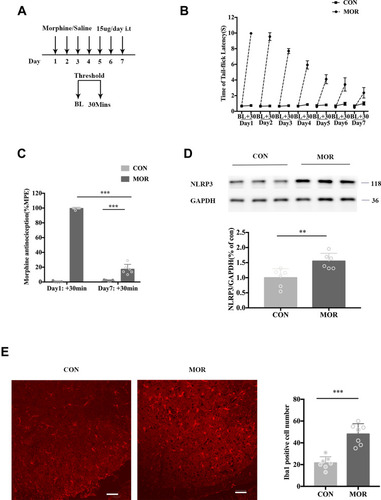
NLRP3 Depletion Prevented the Development of Tolerance Induced by Chronic Morphine Treatment and Suppressed the Activation of Microglia
NLRP3 knockout mice were used to assess the effect of NLRP3 inflammasome in morphine tolerance. Genotyping and Western blotting demonstrated the knockout efficacy of NLRP3 (A and ). Compared with the wild-type (WT) mice, an improved potent analgesic effect of morphine was observed in the NLRP3 KO mice throughout the 7 days of treatment (). On day 7, morphine antinociception was significantly attenuated in the WT group (; unpaired t-test, t = 29.78 p < 0.0001 compared with day 1), while the analgesic potency of morphine was retained on day 7 in NLRP3 KO mice (; unpaired t-test, t = 5.574, p=0.0003 compared with the WT+morphine group). In addition, the %∆MPE between days 1 and 7 showed that the morphine-induced tolerance was prevented in the NLRP3 KO group (; ANOVA with Tukey’s test, F(3,20) = 204.7; p < 0.0001, compared with the WT + morphine group).
Figure 2 Depletion of NLRP3 prevented the development of tolerance induced by chronic morphine treatment and suppressed the activation of microglia. (A) NLRP3 genotyping. (B) Western blot showing NLRP3 expression in the spinal cord in littermate controls and NLRP3 KO mice. (C) The withdrawal latencies of NLRP3 KO and wild-type (WT) mice before and 30 min after morphine treatment. (D) The %MPE of WT and KO mice treated with morphine on days 1 and 7. Columns and errors are presented as mean ± SEM; n = 6, **p<0.01. (E) The %ΔMPE of WT and KO mice treated with saline or morphine from days 1 to 7. **p < 0.01. (F and G) NLRP3 (red) colocalization with Iba1 (green) in the dorsal horn of the spinal cord of littermate controls and NLRP3 KO mice treated with saline or morphine. Scale bar = 50 μm. Columns and errors are presented as mean ± SEM; n = 7, ***p < 0.001.
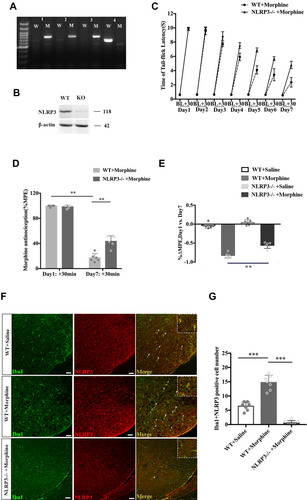
To further assess the effect of the NLRP3 inflammasome on microglial activation in morphine tolerance, we examined the co-localization of NLRP3 with Iba1 (an indicator of microglia activation) by immunofluorescence (). Double staining of NLRP3 and Iba1 predominantly confirmed this co-localization, thereby supporting the fact that NLRP3 was expressed in the activated microglia in the spinal cord of mice who had developed a certain degree of morphine tolerance (; ANOVA with Tukey’s test, F(2,18) = 111.9, p <0.0001 compared with the WT+saline group). Furthermore, in NLRP3 KO plus morphine treatment mice, the number of NLRP3- and Iba1-positive cells was significantly decreased (; ANOVA with Tukey’s test, F(2,18) = 111.9; p <0.0001 compared with the WT+morphine group).
TLR4 Knockout Decreased the Activation of the NLRP3 Inflammasome During the Development of Morphine Tolerance
To investigate the role of TLR4 in NLRP3 inflammasome activation during the development of morphine-induced antinociceptive tolerance, we used a line of TLR4 knockout mice. The knockout of TLR4 was confirmed by genomic polymerase chain reaction and Western blotting (). TLR4 depletion greatly suppressed the chronic morphine-induced expression of NLRP3 (; ANOVA with Tukey’s test, F(2,9) = 69.43, TLR4 KO + morphine vs WT + morphine: p = 0.0042), while TLR4 knockout alone did not induce NLRP3 expression changes (). TLR4 depletion also prevented the development of morphine-induced antinociceptive tolerance. Morphine showed a more potent analgesic effect in TLR4 KO mice than in WT mice throughout the 7 days (). On day 7, morphine-induced antinociception was significantly attenuated in WT mice (; unpaired t-test, t = 34.03; p < 0.0001 compared with day 1: +30 min), while the analgesic potency of morphine was still powerful in TLR4 KO mice (; unpaired t-test, t = 11.7; p<0.0001 compared with the WT + morphine group).
Figure 3 Depletion of TLR4 prevented the development of morphine antinociceptive tolerance and inhibited the morphine-tolerant-induced upregulation of NLRP3. (A) TLR4 genotyping. (B) Western blot results showing TLR4 expression in the spinal cord of littermate controls and TLR4 KO mice. (C) Western blot showing NLRP3 expression in the spinal cord of littermate controls and TLR4 KO mice treated with saline or morphine. Columns and errors are presented as mean ± SEM; n = 6, **p<0.01, ***p < 0.001. (D) The withdrawal latencies of TLR4 KO and wild-type (WT) mice before and 30 min after morphine treatment. (E) The %MPE of WT and KO mice treated with morphine on days 1 and 7. Columns and errors are presented as mean ± SEM; n = 6, ***p<0.001.

Spinal P2X7 Receptor Inhibition Alleviated the Activation of the NLRP3 Inflammasome During the Development of Morphine Tolerance
In order to assess the role of the P2X7 receptor in NLRP3 inflammasome activation during the development of morphine-induced antinociceptive tolerance, we used A438079, an antagonist of the P2X7 receptor, to inhibit P2X7 receptors. In the morphine group, chronic morphine induced an significant increase in P2X7 receptor protein expression within the lumbar spinal cord, and A438079 suppressed the morphine-induced expression of P2X7 receptors (A and ; ANOVA with Tukey’s test, F(3,20) = 35.5, CON vs MOR: p <0.0001; MOR vs A + MOR: p <0.0001). A438079 suppressed the morphine-induced expression of NLRP3 and A438079 alone did not alter NLRP3 expression (A and ; ANOVA with Tukey’s test, F(3,20) = 6.322, MOR vs A+MOR: p =0.006). Simultaneously, A438079 prevented the development of chronic morphine-induced anti-nociceptive tolerance. Morphine showed a more potent analgesic effect in the A+MOR group than in the MOR group (). On day 7, morphine-induced antinociception was significantly attenuated in the morphine group (; unpaired t-test, t = 43.28; p < 0.0001 compared with day 1:+30 min), while the analgesic potency of morphine was retained in the A+MOR group (; unpaired t-test, t = 4.79, p=0.0007 compared with the MOR group). On day 8, mice pretreated with A438079 showed elevated response to morphine and prevented the rightward shift of the morphine dose-response curve (; n=6). Double-labelling fluorescence staining demonstrated the colocalization of the P2X7 receptor with Iba1, which confirmed that, just like the NLRP3 inflammasome, the P2X7 receptor was also expressed in the activated microglia in the spinal cord of mice during the development of morphine-induced antinociceptive tolerance ().
Figure 4 A438079 prevented the development of morphine antinociceptive tolerance and inhibited the morphine-tolerant-induced upregulation of NLRP3. (A) Western blot showing the expression of P2X7R and NLRP3 in the spinal cord of mice treated with saline (CON), morphine (MOR), A438079+morphine (A+MOR) and A438079. (B) Columns and errors are presented as mean ± SEM; n = 6, **p<0.01, ***p < 0.001. (C) The withdrawal latencies of WT mice treated with morphine, A438079+morphine or 25%DMSO+Morphine before and 30 min after morphine treatment. (D) The %MPE of WT mice treated with morphine or A438079+morphine on days 1 and 7. Columns and errors are presented as mean ± SEM; n = 6, ***p<0.001. (E) Repeated morphine treatment produced a significant rightward shift in the morphine dose-response curve on day 8. A438079 pretreated mice showed elevated response to morphine, and morphine response curve was shifted to the left. The ID50 of morphine in mice treated with morphine and treated with A438079+morphine on day8 were 29.04 ug and 7.254ug (n=6). (F) P2X7R (red) colocalization with Iba1 (green) in the dorsal horn of spinal cord of WT mice treated with morphine.
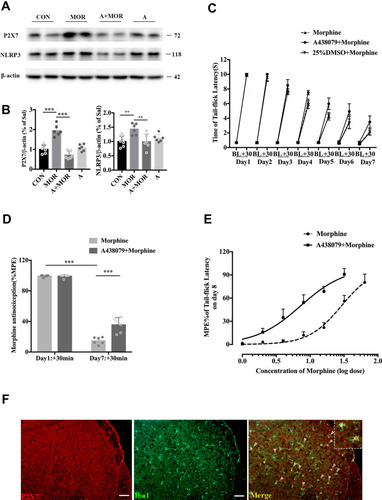
Discussion
In this study, we demonstrated that NLRP3 protein levels increased, accompanied by microglial activation, over the course of morphine tolerance development. NLRP3 knockout markedly potentiated the analgesic effects of morphine and inhibited the activation of microglia evoked by a chronic morphine treatment. These findings clearly indicated that the NLRP3 inflammasome in microglia plays a crucial role in morphine-induced antinociceptive tolerance. Next, TLR4 knockout was found to exert an inhibitory effect on the development of morphine-induced antinociceptive tolerance, as well as chronic morphine-induced spinal NLRP3 expression. Furthermore, we showed an increased expression of spinal P2X7 receptors after the development of chronic morphine-induced antinociceptive tolerance. Inhibition of spinal P2X7 receptors not only decreased chronic morphine-induced antinociception tolerance and the expression levels of P2X7 receptors but also rectified the increase in spinal NLRP3 expression levels during the development of morphine-induced antinociceptive tolerance. Spinal TLR4 or P2X7 receptor-dependent signaling-induced NLRP3 inflammasome activation in microglia contributes to the development of morphine-induced antinociceptive tolerance.
The NLRP3 inflammasome is abundantly expressed in the central nervous system (CNS) and acts to detect harmful substances or irregularities.Citation28,Citation29 An activated NLRP3 inflammasome leads to the activation of caspase-1, which subsequently promotes the maturation of IL-1β and interleukin-18 (IL-18). IL-1β and IL-18 further trigger inflammatory responses through a complex array of downstream signaling pathways, leading to a vicious cycle of neuroinflammation.Citation30,Citation31 Neuroinflammation has been heavily implicated in the formation of morphine-induced antinociceptive tolerance.Citation32 Inhibiting NLRP3 signaling to decrease neuroinflammation has been reported to alleviate morphine-induced antinociception tolerance in previous studiesCitation3,Citation5,Citation33,Citation34 Furthermore, with regard to neuropathic status, miR-223 has been reported to downregulate and suppress the activities of the NLRP3 inflammasome to relieve morphine-induced antinociception tolerance in rats.Citation35 Studies have shown that morphine can also modulate immune responses by promoting IL-1β release, while the inhibition of IL-1β prevents morphine-induced antinociception tolerance.Citation36 These findings point to an important role of NLRP3 in modulating neuroinflammation and in the development of morphine-induced antinociceptive tolerance.
In previous research, TLR4 signaling has been reported to contribute to the development of morphine tolerance in the spinal cordCitation37 and midbrain ventrolateral periaqueductal gray.Citation17 Morphine binds to MD-2, the accessory protein of TLR4, thereby activating TLR4 and triggering proinflammatory signaling,Citation18 such as by the release of IL-1βCitation38 and soluble TNF,Citation17 subsequently increasing robust neuroinflammatory responses, and ultimately increasing neuroexcitation. Systemic antagonism of TLR4 has been reported to attenuate the development of morphine-induced antinociception tolerance.Citation16 Nevertheless, apparently no attempt has been made to isolate the downstream signal of TLR4 that is responsible for opioid tolerance. As mentioned above, the activation of the NLRP3 inflammasome is a two-step process. Danger-associated cytokines, such as TNF-a and IL-1β binding to TLRs, may provide the initial signal and lead to the upregulation of NLRP3 and pro-IL-1β at the transcriptional level.Citation39 In this study, the protein expression of NLRP3 was inhibited in TLR4−/- mice after chronic morphine application, indicating that TLR4 induces the development of morphine-induced tolerance through the NLRP3 inflammasome pathway. Pro-IL-1β is not normally expressed, and the basal levels of NLRP3 are not sufficient for efficient inflammasome formation,Citation11 meaning that the failure of TLR4−/- mice to activate the NLRP3 inflammasome after morphine tolerance may be due to insufficient levels pro-IL-1β and NLRP3 levels.
Besides, we found that A438079, the antagonist of P2X7R, also inhibited the chronic morphine-induced expression of NLRP3, pointing to a key role of the ATP/P2X7R axis as an alternative signaling pathway in the activation of NLRP3 during the development of morphine-induced antinociceptive tolerance. P2X7R is an ATP-gated nonselective cationic channel which is vastly expressed in immune cells such as macrophagesCitation40 and microglial cells.Citation41 High concentrations of ATP, which occur under pathological circumstances, bind to P2X7R and induce the opening of cationic channels on cell membranes. Related studies showed that in the CNS, the influx of Ca2+ in the microglia through the activation of P2X7R can promote neuroinflammatory responses through p38/MAPKCitation42 or IL-1β signaling pathways.Citation43 Furthermore, Ca2+ influx may promote the alternative activation of NLRP3 inflammasome activation. Hence, we hypothesized that the action of the NLRP3 inflammasome and subsequent release of inflammatory cytokines is P2X7R-dependent during the development of morphine-induced antinociceptive tolerance. Findings demonstrating that the expression of P2X7R was increased in microglia in the morphine-tolerant mice and that A438079 markedly inhibited the tolerance and the upregulated expression of NLRP3 support our hypothesis and further suggest that the chronic morphine-induced activation of the NLRP3 inflammasome occurred mainly in the microglia. Some previous research has discussed the role of P2X7R in morphine-induced antinociceptive tolerance,Citation6,Citation44,Citation45 but to the best of our knowledge, this is the first study to confirm that P2X7R promotes morphine-induced antinociceptive tolerance through the NLRP3 inflammasome pathway in microglia.
Conclusions
In conclusion, this work demonstrates that the NLRP3 inflammasome in microglia plays a crucial role in morphine-induced antinociceptive tolerance and that both TLR4- and P2X7R- dependent pathways are alternative signaling pathways required for NLRP3 inflammasome activation during the development of morphine-induced antinociceptive tolerance (summarized in ). This work provides a new perspective to inform the targeted treatment of morphine-induced antinociception tolerance.
Abbreviations
NLRP, Nod-like receptor protein 3; ASC, apoptosis-associated speck-like protein; TLR4, toll-like receptor 4; TNF, tumor necrosis factor; IL-1β, interleukin 1 βeta; IL-6, interleukin 6; P2X7R, P2X7 receptor; TBST, Tris-buffered saline with Tween; ANOVA, analysis of variance; WT, wild-type; CNS, central nervous system; IL-18, interleukin-18.
Data Sharing Statement
All the data supporting the findings of this study are available within the article, and from the corresponding author upon reasonable request.
Ethics Approval and Consent to Participate
The protocol followed the NIH Guide for the Care and Use of Laboratory Animals (1996) and was approved by the Animal Care and Use Committee of the Sixth People’s Hospital Affiliated to Shanghai Jiao Tong University (SYXK [Shanghai, China] 2011-0128, 1 January 2011). All studies involving animals are reported in accordance with the ARRIVE guidelines. All efforts were made to minimize suffering and reduce the number of animals used.
Author Contributions
All authors made substantial contributions to conception and design, acquisition of data, or analysis and interpretation of data; took part in drafting the article or revising it critically for important intellectual content; agreed on the journal to which the article will be submitted; gave final approval of the version to be published; and agree to be accountable for all aspects of the work.
Acknowledgments
We want to thank Dr. Xu at Shanghai East Hospital for gifting TLR4 KO and NLRP3 KO mice.
Disclosure
The authors declare that they have no competing interests for this work.
References
- Williams JT, Ingram SL, Henderson G, et al. Regulation of mu-opioid receptors: desensitization,phosphorylation, internalization, and tolerance. Pharmacol Rev. 2013;65(1):223–254. doi:10.1124/pr.112.00594223321159
- Bagley EE, Chieng BC, Christie MJ, et al. Opioid tolerance in periaqueductal gray neurons isolated from mice chronically treated with morphine. Br J Pharmacol. 2005;146(1):68–76. doi:10.1038/sj.bjp.070631515980868
- Ueda H, Ueda M. Mechanisms underlying morphine analgesic tolerance and dependence. Front Biosci. 2009;14:5260–5272. doi:10.2741/3596
- Eidson LN, Murphy AZ. Inflammatory mediators of opioid tolerance: implications for dependency and addiction. Peptides. 2019;115:51–58. doi:10.1016/j.peptides.2019.01.00330890355
- Mika J, Wawrzczak-Bargiela A, Osikowicz M, et al. Attenuation of morphine tolerance by minocycline and pentoxifylline in naive and neuropathic mice. Brain Behav Immun. 2009;23(1):75–84. doi:10.1016/j.bbi.2008.07.00518684397
- Leduc-Pessah H, Weilinger NL, Fan CY, et al. Site-specific regulation of P2X7 receptor function in microglia gates morphine analgesic tolerance. J Neurosci. 2017;37(42):10154–10172. doi:10.1523/jneurosci.0852-17.201728924009
- Hameed H, Hameed M, Christo PJ. The effect of morphine on glial cells as a potential therapeutic target for pharmacological development of analgesic drugs. Curr Pain Headache Rep. 2010;14(2):96–104. doi:10.1007/s11916-010-0093-y20425198
- Haque ME, Akther M, Jakaria M, et al. Targeting the microglial NLRP3 inflammasome and its role in Parkinson’s disease. Mov Disord. 2020;35(1):20–33. doi:10.1002/mds.2787431680318
- Hung WL, Ho CT, Pan MH. Targeting the NLRP3 inflammasome in neuroinflammation: health promoting effects of dietary phytochemicals in neurological disorders. Mol Nutr Food Res. 2020;64(4):e1900550. doi:10.1002/mnfr.20190055031675164
- Song L, Pei L, Yao S, et al. NLRP3 inflammasome in neurological diseases, from functions to therapies. Front Cell Neurosci. 2017:11. doi: 10.3389/fncel.2017.00063.28217083
- Bauernfeind FG, Horvath G, Stutz A, et al. Cutting edge: NF-kappaB activating pattern recognition and cytokine receptors license NLRP3 inflammasome activation by regulating NLRP3 expression. J Immunol. 2009;183(2):787–791. doi:10.4049/jimmunol.090136319570822
- Mariathasan S, Weiss DS, Newton K, et al. Cryopyrin activates the inflammasome in response to toxins and ATP. Nature. 2006;440(7081):228–232. doi:10.1038/nature0451516407890
- Muñoz-Planillo R, Kuffa P, Martínez-Colón G, et al. K+ efflux is the common trigger of NLRP3 inflammasome activation by bacterial toxins and particulate matter. Immunity. 2013;38(6):1142–1153. doi:10.1016/j.immuni.2013.05.01623809161
- Heid ME, Keyel PA, Kamga C, et al. Mitochondrial reactive oxygen species induces NLRP3-dependent lysosomal damage and inflammasome activation. J Immunol. 2013;191(10):5230–5238. doi:10.4049/jimmunol.130149024089192
- Cai Y, Kong H, Pan YB, et al. Procyanidins alleviates morphine tolerance by inhibiting activation of NLRP3 inflammasome in microglia. J Neuroinflammation. 2016;13(1):53. doi:10.1186/s12974-016-0520-z26931361
- Eidson LN, Murphy AZ. Blockade of Toll-like receptor 4 attenuates morphine tolerance and facilitates the pain relieving properties of morphine. J Neurosci. 2013;33(40):15952–15963. doi:10.1523/JNEUROSCI.1609-13.201324089500
- Eidson LN, Inoue K, Young LJ, et al. Toll-like receptor 4 mediates morphine-induced neuroinflammation and tolerance via soluble tumor necrosis factor signaling. Neuropsychopharmacology. 2017;42(3):661–670. doi:10.1038/npp.2016.13127461080
- Wang X, Loram LC, Ramos K, et al. Morphine activates neuroinflammation in a manner parallel to endotoxin. Proc Natl Acad Sci U S A. 2012;109(16):6325–6330. doi:10.1073/pnas.120013010922474354
- Lewis SS, Hutchinson MR, Rezvani N, et al. Evidence that intrathecal morphine-3-glucuronide may cause pain enhancement via toll-like receptor 4/MD-2 and interleukin-1beta. Neuroscience. 2010;165(2):569–583. doi:10.1016/j.neuroscience.2009.10.01119833175
- Tavakoli Dargani Z, Singla DK. Embryonic stem cell-derived exosomes inhibit doxorubicin-induced TLR4-NLRP3-mediated cell death-pyroptosis. Am J Physiol Heart Circ Physiol. 2019;317(2):H460H71. doi:10.1152/ajpheart.00056.201931172809
- Fu S, Wang J, Hao C, et al. Tetramethylpyrazine ameliorates depression by inhibiting TLR4-NLRP3 inflammasome signal pathway in mice. Psychopharmacology. 2019;236(7):2173–2185. doi:10.1007/s00213-019-05210-630847567
- Bhattacharya A. Recent advances in CNS P2X7 physiology and pharmacology: focus on neuropsychiatric disorders. Front Pharmacol. 2018;9:30. doi:10.3389/fphar.2018.0003029449810
- Thawkar BS, Kaur G. Inhibitors of NF-kappaB and P2X7/NLRP3/Caspase 1 pathway in microglia: novel therapeutic opportunities in neuroinflammation induced early-stage Alzheimer’s disease. J Neuroimmunol. 2019;326:62–74. doi:10.1016/j.jneuroim.2018.11.01030502599
- Faria RX, Freitas HR, Reis RAM. P2X7 receptor large pore signaling in avian Muller glial cells. J Bioenerg Biomembr. 2017;49(3):215–229. doi:10.1007/s10863-017-9717-928573491
- Kelley N, Jeltema D, Duan Y, et al. The NLRP3 inflammasome: an overview of mechanisms of activation and regulation. Int J Mol Sci. 2019;20:13. doi:10.3390/ijms20133328
- Kilkenny C, Browne W, Cuthill IC, et al. Animal research: reporting in vivo experiments–the ARRIVE guidelines. J Cereb Blood Flow Metab. 2011;31(4):991–993. doi:10.1038/jcbfm.2010.22021206507
- Jiang W, Li M, He F, et al. Targeting the NLRP3 inflammasome to attenuate spinal cord injury in mice. J Neuroinflammation. 2017;14(1):207. doi:10.1186/s12974-017-0980-929070054
- Yang F, Wang Z, Wei X, et al. NLRP3 deficiency ameliorates neurovascular damage in experimental ischemic stroke. J Cereb Blood Flow Metab. 2014;34(4):660–667. doi:10.1038/jcbfm.2013.24224424382
- Geldhoff M, Mook-Kanamori BB, Brouwer MC, et al. Genetic variation in inflammasome genes is associated with outcome in bacterial meningitis. Immunogenetics. 2013;65(1):9–16. doi:10.1007/s00251-012-0653-x23053059
- Bossu P, Ciaramella A, Salani F, et al. Interleukin-18, from neuroinflammation to Alzheimer’s disease. Curr Pharm Des. 2010;16(38):4213–4224. doi:10.2174/13816121079451914721184660
- Wilms H, Sievers J, Rickert U, et al. Dimethylfumarate inhibits microglial and astrocytic inflammation by suppressing the synthesis of nitric oxide, IL-1beta, TNF-alpha and IL-6 in an in-vitro model of brain inflammation. J Neuroinflammation. 2010;7:30. doi:10.1186/1742-2094-7-3020482831
- DeLeo JA, Tanga FY, Tawfik VL. Neuroimmune activation and neuroinflammation in chronic pain and opioid tolerance/hyperalgesia. Neuroscientist. 2004;10(1):40–52. doi:10.1177/107385840325995014987447
- Qu J, Tao XY, Teng P, et al. Blocking ATP-sensitive potassium channel alleviates morphine tolerance by inhibiting HSP70-TLR4-NLRP3-mediated neuroinflammation. J Neuroinflammation. 2017;14(1):228. doi:10.1186/s12974-017-0997-029178967
- Liu Q, Su LY, Sun C, et al. Melatonin alleviates morphine analgesic tolerance in mice by decreasing NLRP3 inflammasome activation. Redox Biol. 2020;34:101560. doi:10.1016/j.redox.2020.10156032413745
- Xie XJ, Ma LG, Xi K, et al. Effects of microRNA-223 on morphine analgesic tolerance by targeting NLRP3 in a rat model of neuropathic pain. Mol Pain. 2017;13:1744806917706582. doi:10.1177/174480691770658228580822
- Zhang Y, Wang K, Lin M, et al. Inhibition of morphine tolerance by MrgC receptor via modulation of interleukin-1beta and matrix metalloproteinase 9 in dorsal root ganglia in rats. Eur J Pharmacol. 2017;815:10–17. doi:10.1016/j.ejphar.2017.10.01128993160
- Qian J, Zhu Y, Bai L, et al. Chronic morphine-mediated upregulation of high mobility group box 1 in the spinal cord contributes to analgesic tolerance and hyperalgesia in rats. Neurotherapeutics. 2019. doi:10.1007/s13311-019-00800-w
- Liang Y, Chu H, Jiang Y, et al. Morphine enhances IL-1β release through toll-like receptor 4-mediated endocytic pathway in microglia. Purinergic Signal. 2016;12(4):637–645. doi:10.1007/s11302-016-9525-427506813
- Sutterwala FS, Haasken S, Cassel SL. Mechanism of NLRP3 inflammasome activation. Ann N Y Acad Sci. 2014;1319:82–95. doi:10.1111/nyas.1245824840700
- Jiang S, Zhang Y, Zheng JH, et al. Potentiation of hepatic stellate cell activation by extracellular ATP is dependent on P2X7R-mediated NLRP3 inflammasome activation. Pharmacol Res. 2017;117:82–93. doi:10.1016/j.phrs.2016.11.04027940204
- He Y, Taylor N, Fourgeaud L, et al. The role of microglial P2X7: modulation of cell death and cytokine release. J Neuroinflammation. 2017;14(1):135. doi:10.1186/s12974-017-0904-828716092
- Kim EA, Cho CH, Kim J, et al. The azetidine derivative, KHG26792 protects against ATP-induced activation of NFAT and MAPK pathways through P2X7 receptor in microglia. Neurotoxicology. 2015;51:198–206. doi:10.1016/j.neuro.2015.10.01326522449
- Bhattacharya A, Jones DNC. Emerging role of the P2X7-NLRP3-IL1beta pathway in mood disorders. Psychoneuroendocrinology. 2018;98:95–100. doi:10.1016/j.psyneuen.2018.08.01530121550
- Chen ML, Cao H, Chu YX, et al. Role of P2X7 receptor-mediated IL-18/IL-18R signaling in morphine tolerance: multiple glial-neuronal dialogues in the rat spinal cord. J Pain. 2012;13(10):945–958. doi:10.1016/j.jpain.2012.06.00722968128
- Zhou D, Chen ML, Zhang YQ, et al. Involvement of spinal microglial P2X7 receptor in generation of tolerance to morphine analgesia in rats. J Neurosci. 2010;30(23):8042–8047. doi:10.1523/JNEUROSCI.5377-09.201020534852