Abstract
Mucus is an integral part of the respiratory physiology. It protects the respiratory tract by acting as a physical barrier against inhaled particles and microbes. Excessive inflammation in conditions such as COVID-19 can result in over-production of mucus which obstructs the airway. Build-up of mucus can also contribute to recurrent airway infection, causing further obstruction. This article summarizes the current understanding and knowledge of respiratory mucus production and proposes the role of cytokine storm in inducing sudden mucus hypersecretion in COVID-19. Based on these cascades, the active constituents that inhibit or activate several potential targets are outlined for further research. These may be explored for the discovery and design of drugs to combat cytokine storm and its ensuing complications.
Introduction
Nasal blockage or respiratory congestion is among the most common symptoms experienced in primary care as well as tertiary care. It can be particularly severe and even lethal in COVID-19 due to the formation of mucus plugs. Transmission of COVID-19 appears to occur primarily through dispersal of droplets generated from the respiratory tract when an infected person talks, coughs, or sneezes. Large amounts of the SARS-CoV-2 virus have been reported in sputum and nasal specimens, which account for the transmission through respiratory droplets. Numerous studies conclude that the recent coronavirus infection causes an allergic reaction in respiratory tract mucosa, which activates mucin secretion and modulates its chemical structure to enable the virus to enter the cells.Citation1–Citation3 Thereafter, SARS-CoV-2 initiates neutrophil and mucus-mediated inflammatory pathways.Citation4
SARS-CoV-2 is shed predominantly in upper and lower airway tract secretions.Citation5–Citation9 Patients with severe COVID-19 infections are likely to develop acute respiratory distress syndrome (ARDS), consisting of hypoxemic respiratory failure associated with neutrophilia, mucus deposition in bronchi, and bronchiectasis.Citation10 Therefore, better understanding is needed of the mechanisms underlying secretions, and how to control them. The increase in mucus production and secretion is likely due to mucus cell metaplasia since pulmonary inflammatory diseases are often associated with excessive mucus secretion. Computerized tomography (CT) images of COVID-19 depict the incidence of mucoid impaction in lungs. Studies of CT imaging in the pulmonary parenchymal region of COVID-19 patients have reported a 64% occurence of pathological fluid in the alveolar sacs which appears multifocal, patchy, or segmented and is distributed around sub-pleural areas or along broncho-vascular bundles.Citation11,Citation12,Citation13 Increase in sputum volume and mucus hypersecretion associated symptoms have been seen in up to 40% of patients. The mucus in these patients is also found to be more viscous than that in those with chronic obstructive pulmonary disease (COPD). Lastly, the formation of colloidal mucus plugs is more frequent in these patients.
The role of inflammatory stimuli in influencing mucus cell levels remains uncertain. Here we review recently published work which defines events in the immune system and downstream epithelial cascade related to continuous metaplasia of mucus cells.
Physiology of Airway Mucus Secretion
In the pulmonary system, mucus is a component of the epithelial lining fluid (ELF) or airway surface liquid (ASL). The major part of the respiratory tract is covered by it. The ASL comprises a sol layer and an overlying gel layer which are known as the perciliary liquid layer (PCL) and the mucus layer, respectively (see ).
Figure 1 Schematic illustration of a goblet cell, associated signalling for mucous secretion and microscopic images of goblet cells: (i) The gel on brush model describes mucus existing in two discrete layers, a more viscous gel layer on top and a periciliary layer (PCL) below. The gel layer contains the secreted mucins MUC5AC and MUC5B whilst the PCL contains the membrane-tethered mucins MUC1, MUC2 & MUC4; (ii) Viral infection induced signalling involved in mucus secretion; (iii) Lung tissue sections from a COPD-smoker showing goblet cells of large airway epithelium.Notes: Figure 1 (iii) reproduced with permission from Shukla SD, Mahmood MQ, Weston S, et al. The main rhinovirus respiratory tract adhesion site (ICAM-1) is upregulated in smokers and patients with chronic airflow limitation (CAL). Respir Res. 2017;18(1):6.Citation14
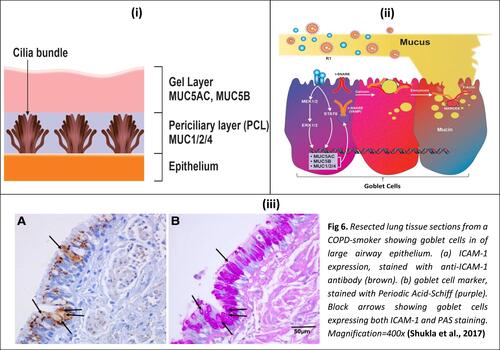
Mucus consists primarily of water (~95%). The major non-aqueous component is mucin, while proteoglycans, lipids, proteins, and DNA are also present in smaller quantities.Citation15 Mucin is secreted by goblet cells which are columnar epithelial cells present in the respiratory, gastrointestinal and reproductive tracts. Mucin-containing secretory vesicles are present at the upper surface of goblet cells. Short microvilli projections are present on the upper surface of goblet cells which give an increased surface area for secretion.Citation16,Citation17
Mucin, the main protein component of mucus, functions primarily as a barrier, and consists of MUC5AC and MUC5B as important secreted mucin genes. Their sizes range from 200 kDa to 200 MDa. Chains of carbohydrates make up around 80% of the weight of mucins.Citation18 Since mucin is quite large, it is packaged in secretory vesicles in a dehydrated state.Citation8 The release of mucin is governed by fusion proteins such as SNARE (N-ethyl-maleimide-sensitive factor attachment protein receptor) and MARCKS (Myristoylated Alanine-Rich C Kinase Substrate). The secretion occurs in the presence of high pH and low calcium concentration.Citation19–Citation22
Past studies have shown that cytokines IL4, IL5, IL9, and IL13 upregulate mucus gene expression and mucus cell hypersecretion.Citation23,Citation24 Apart from cytokines, various other stimuli such as smoking generate reactive oxygen species. These stimuli upregulate numerous downstream cascades, which trigger multiple signaling pathways via mitogen-activated protein kinase and other signaling cascades. These signaling cascades induce goblet cell differentiation in the airway, causing excessive synthesis and secretion of mucin.Citation25,Citation26 Such rampant hypersecretion leads to clinical conditions such as chronic obstructive pulmonary disease, asthma, bronchiectasis, and other respiratory disease conditions such as those observed in COVID-19 patients.
Association Between Viral Infection and Mucus Production
The inflammatory response that occurs after viral infection is similar to that observed in asthma and other respiratory conditions in which the role of mucus is profound. Viruses such as influenza, negative-strand RNA viruses such as respiratory syncytial virus (RSV) and rhinoviruses (RV) and lung colonization by pathogenic opportunistic bacteria have shown enhanced exacerbation in bronchial epithelial cells.Citation27,Citation28 For example, RSV infection in the upper respiratory tract is distinguished by inflammation and obstruction in the airways tract due to the formation of mucus plugs containing mucus, fibrin protein, cellular debris, and lymphocytes. Generally, these viruses activate the downstream signaling cascades of inflammatory markers through chemokines, as shown in . These in turn trigger multiple signaling pathways that result in goblet cell differentiation and hyperplasia in the airway, leading to the synthesis of MUC proteins, particularly MUC5AC, MUC5B, MUC1, MUC2, and MUC4 followed by their secretion.Citation29 Studies have shown that RSV and human metapneumovirus (hMPV) stimulate varying production of mucin in A549 cell line.Citation30 Despite similarities in the structure and pathogenicity of these two viruses, they cause different expressions of MUC2, MUC5AC, and MUC5B as well as membrane-bound mucins.Citation31 Thus, certain viral infections may uniquely alter the composition of mucus in respiratory epithelium.
Mucus Hypersecretion in COVID-19
Formation of mucus plugs has been observed in COVID 19 patients, causing airway obstruction and respiratory failure in a significant proportion of such patients. Severe mucoid tracheitis is detected in 33% of COVID-19 autopsies.Citation32
Association of Immune Response with Mucus Secretion
Sungnank et al. have stated that the nasal epithelial serves as the point of infection of SARS-CoV-2, from where it moves to the lower respiratory tract.Citation33 The respiratory mucosa functions as a defensive layer against pathogens. The layer has the ability to trap an invading pathogen through sticky secretions and then move it out via ciliary action.Citation34 Arumugham et al. suggest that SARS-CoV-2 overstimulates the mucosa in a pathophysiology similar to other viruses such as dengue virus. This leads to the activation of an inflammatory cascade and the release of various inflammatory cytokines and chemokines.Citation35 This is in line with other studies that show SARS-CoV-2 activates the inflammatory response and induces increased secretion of respiratory mucosa.Citation36
In an experiment by Cohn et al., the role of IL-4 and IL-5 in mucus production and cell recruitment mediated by TH2 cells is well described. Activation of CD4 T cells by IL-4 causes the differentiation of th0 cells to th2 cells which in turn activates IL-4 secretion, maintaining a positive feedback loop.Citation37 Interleukin 4 induces the transcription of MUC5AC by activation of the JAK3/STAT 6 pathway. STAT 6 is involved in the activation of CLCA1 (calcium activated chloride channel 1) which activates MAPK signaling ultimately resulting in mucin production. Th2 cells help in recruitment of lymphocytes and eosinophils into lungs causing the over-secretion of MUC5AC in the airway resulting in goblet cell hyperplasia and damage of the ciliary layer of epithelial cells.Citation38 Very-Late-Activation-Antigen-4 (VLA-4) is present on eosinophils and T lymphocytes which has the ability to bind with Vascular Cell Adhesion Molecule 1 (VCAM-1) and allows selective entry of eosinophils into injured tissues.Citation38
In various studies it has been found that defects in gene expression and function of Cystic Fibrosis Transmembrane Conductance Regulator (CFTR) is associated with airway mucus hypersecretion. CFTR acts as a cAMP-dependent chloride channel.Citation39 In an experiment performed on murine cell lines, tissue expression pattern of CLCA1 intersecting with that of CFTR confirms the fact that both genes participate in the pathogenesis of cystic fibrosis. The channel transports chloride ions, controlling the movement of water in tissues, which is necessary for the production of mucus.Citation40 Mutation in the CFTR gene disrupts the movement of water and chloride ions out of the cell causing the mucus to be thick and sticky. Trapped chloride ions in cells cannot attract the fluids necessary to hydrate the cell surface and in the absence of the fluids, mucus becomes dehydrated and takes on a viscous consistency.Citation41 CFTR modulator therapy proposed by Jarosz-Griffiths and co-workers could confer additional benefit to patients and may also contribute to improved clinical outcomes.Citation42
Role of Inflammation in Airway Mucus Hypersecretion
The symptoms in COVID-19 and elevated levels of inflammatory markers in patients indicate that a severe cytokine storm develops in this disease. Recent studies support that inflammation causes mucus hypersecretion. Studies have shown that most cases infected with SARS-CoV-2 have normal WBC counts or in some cases lymphocytopenia. Patients showing severe conditions have significant increases in neutrophil levels. Their blood urea and D-dimer levels are also significantly high, whereas there is a reduction in their lymphocyte count.Citation43 The levels of several pro-inflammatory cytokines such as IL6, IL10, and TNF-α are elevated. Moreover, the blood report of patients admitted to intensive care units (ICUs) have shown increases in IL-2, IL-7, and IL-10.Citation44–Citation46 The inflammatory response can induce mucus hypersecretion which can obstruct the respiratory tract, limiting airflow and thereby aggravating the already declining lung function.Citation47
Furthermore, the pro-inflammatory cascades alter the composition of mucus and compromise its clearance by cilia.Citation48 This leads to recurrent infection in airway tracts, causing more obstruction in the respiratory tract, thereby creating a vicious cycle. COVID-19 patients have higher levels of several pro-inflammatory markers, namely IL-1β, IL-6, IL-2, IL-13, and TNFα as shown in along with their crosstalk.Citation49
Figure 2 Crosstalk of interleukins, IL-4, IL-2, IL-6, IL-5, TNF-α, histamine and the downstream signaling pathways leading to the production of interleukins involved in mucus hypersecretion. Note: The cytokines written in red are elevated in COVID-19 patients.
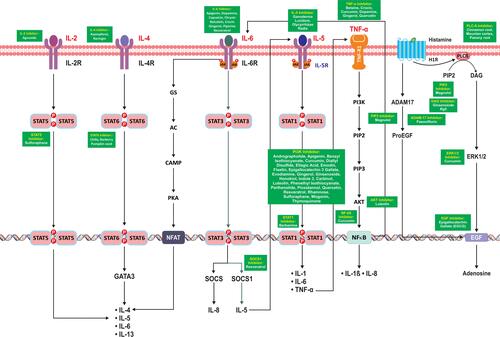
The crosstalk of these cytokines and their downstream signaling upregulates several other inflammatory cytokines. IL-2, IL-4, and IL-6 upregulate the levels of IL-4, IL-5, IL-6, and IL-13 via STAT5, STAT6, and NFAT, respectively. IL-5 also upregulates levels of IL-6, IL-1, and TNFα via STAT1. TNFα through NF-κB activation leads to upregulation of IL-1beta and IL-8. Apart from cytokines, histamine released from mast cell degranulation during inflammatory response results in EGF and adenosine synthesis via ERk1/2 upregulation, as shown in . The inflammation caused by these cytokines can result in mucus hypersecretion which corresponds to the complication arising in COVID-19 patients. The subsequent section describes these mechanisms of inflammatory cytokines in mucus hypersecretion.
Overexpression of Mucin Through STAT Mediated Signaling
The JAK-STAT signaling pathway is a series of interactions between proteins in the cytoplasm. It is involved in various processes leading to STAT dimerization and activation of transcription genes in the DNA. IL-4 via its receptor activates STAT6, leading to self-upregulation and activation of the MUC5AC gene complex consisting of MUC1/2/4.Citation50 Meanwhile IL-8 via its receptor leads to SOCS1 protein (Suppressor of cytokine signaling) upregulation leading to IL-4 mRNA synthesis via STAT1. IL-4 mRNA also induces Ca(2+)-activated Cl(-) channel (CLCA1). Binding of CLCA1 to its receptor (CLCA1-R), leads to the inhibition of FOXA2 (Forehead Box A2) which has a down regulatory effect on MUC5AC gene, through MAPK via SAM Pointed Domain Containing ETS Transcription Factor (SPDEF) protein.Citation51 The same signaling pathway is used by interleukin 13 and IL-18 that are modulated by IL-6,Citation52–Citation56 as shown in
IL-9 can induce pleiotropic function in various immune cells as well as normal cells.Citation57 IL-9 signal transduction requires the receptors which have a common γ chain.Citation58 IL-9R receptor activates JAK1 and JAK3, which through downstream signal transduction leads to the activation of STAT1, STAT3, and STAT5. Dimerization of these STATs enhances the mucus hypersecretion.Citation59 IL-2 via its receptor IL-2R causes the activation of Janus kinases family proteins, followed by recruitment to phosphorylated STAT5, which dimerize and attach to the nucleus and initiate IL-5 mRNA transcription. Therefore IL-2 causes the upregulation of IL-5 via the JAK/STAT pathway.Citation60,Citation61
Overexpression of Mucin Through MAPK Mediated Signaling
The MAPK mediated signaling (known as Ras-Raf-MEK-ERK pathway) consists of a chain protein molecule that transduces signal from a receptor induced by pro-inflammatory cytokines from the cell surface to the DNA present in the nucleus. Elevated levels of IL-6 found in COVID-19 would contribute to pathogenesis by promoting mucus hypersecretion. Studies have demonstrated that IL-6 is an important cytokine for the development of mucus metaplasia in the airways in response to inhaled allergens.Citation62 As shown in , IL-6 binds to its receptor IL-6R and activates Growth factor receptor-bound protein 2 and Son of Sevenless complex (GRB2/SOS) which further leads to the activation of Ras and Raf signaling cascades. These cascades activate JNK via the p38/MAPK pathway. Activated JNK upregulates MUC5AC gene in the airway epithelium which enhances the mucus hypersecretion followed by exocytosis of mucin via MARCKS & SNARE.Citation63,Citation64
The expression of IL-5 is upregulated by IL-2, an interleukin which is elevated in severely affected COVID-19 patients. Mucus production due to IL-5 was initially observed in a pulmonary transgenic mouse model. The mechanism consists of IL-5 binding to IL-5R and activating GRB2/SOS which leads to activation of mucus genes (MUC5AC, MUC5B, and MUC1/2/4) in the airway epithelium, which enhances mucin synthesis and hypersecretion via MARCKS and SNARE.Citation65,Citation66
IL-17, a proinflammatory cytokine secreted by T cells, is elevated by IL-6. IL-17 has been found to be upregulated in the mice model of asthma which be inhibited by anti-IL-17 antibody which cause reduction in granulocyte influx.Citation67,Citation68 Mucin expression is induced by IL-17 in cell cultures of airway epithelial cells via upregulation of the MUC5AC gene through IL-5 and IL-6 mediated signaling.
IL-1β acts as an early response pleiotropic cytokine that is produced by different cells in the pulmonary inflammatory cascade, which is elevated in severely affected COVID-19 patients. Upon binding to its receptor IL1R it causes the activation of MyD88 which leads to the activation of MAPK via MAP3K and MAP2K.Citation69,Citation70 IL-1β has been recently shown to increase the expression of MUC5AC gene and mucin secretion in bronchial epithelial cell line.Citation71
Overexpression of Mucin Through NF-κB Mediated Signaling
NF-κB (Nuclear factor kappa-light-chain-enhancer of activated B cells) comprises a group of protein complexes that controls the transcription of DNA, cytokine production namely Il-6, TNFα, IL-8, and IL-1 Beta and cell survival. TNFα is one of the most extensively studied pleiotropic cytokines of the TNF family which is also induced by IL-5.Citation72 TNFα has an important role in the innate immune response against invading pathogens before triggering the adaptive immune system.Citation73 It acts on the ubiquitously expressed TNFR1.Citation74 This receptor ligand interaction causes downstream signaling, leading to phosphorylation of IκB kinase (IKK) composed of subunits IKKα, IKKβ, and thus nuclear factor kappa beta (NF-kB) activation. NF-kB forms a heterodimer composed of p50 and p65 proteinsCitation73,Citation75 This heterodimer interacts with the DNA to increase transcription of pro-inflammatory cytokine genes, such as IL-1B, IL-6, IL-8, and TNFα itself, as shown in . All of these cause pulmonary inflammation and are known to cause mucus hypersecretion. It also induces MUC5AC overexpression through p38-mitogen activated protein kinases/ERK (MAPK/ERK) and Sp1 in human airway epithelial cells.Citation75
TNF-α increases the expression of EGFR in the airways. EGF binds to its receptor (EGFR) and increases the expression of the MUC5AC gene via ERK signaling. EGFR also cause activation of Ras, Raf, and MUC2 via Erk1/2 which enhances the mucus hypersecretion.Citation76 The role of ATP in MUC5AC release was examined by stimulating cells with polyinosine-polycytidylic acid.Citation77 They found that the concentration of extracellular ATP increased in the NCI-H292 cells due to dsRNA stimulation and viral infection. Binding of adenosine triphosphate (ATP) to P2Y2 receptors causes activation of IP3 and DAG via PIP2 and releases calcium from endoplasmic reticulum which enhances the expression of MUC5AC gene in the airway epithelium. Adenosine via its receptor adenosine A1 receptor (Ad1R) through PLC beta also causes activation of the same signaling cascade leading to mucin production.
Leukotriene-Mediated Mucus Over-Secretion
Leukotrienes are from a class of inflammatory mediators that are produced in leukocytes by the oxidation of arachidonic acid (AA) and other essential fatty acids. Studies using exogenous viruses such as rhinovirus (RV) have found CD4-activated efflux of cytokines like IL-5, IL-4, and IL-2, as shown in , which leads to B cell proliferation and IgE mediated leukotriene synthesis.Citation78
Activation of FcεRI via IgE activates the synthesis and release of arachidonic acid (AA).Citation79 AA metabolises into hydroperoxyeicosatetraenoic acid (HPETE) and forms leukotriene (LT) A4 by enzyme 5-lipoxygenase. LTC4 synthase converts LTA4 into LTC4, which further converts into LTF4 and LTD4 with the help of carboxypeptidase A and gamma glutamyl transpeptidase. Both LTF4 and LTD4 are converted by gamma glutamyl transpeptidase and dipeptidase separately into LTE4.Citation80 LTE4 provokes mucus hypersecretion via binding with its receptor, Cysteinyl Leukotriene Receptor 1 (CysLT1).Citation81 IL-2 is confirmed to be elevated in severe SARS-CoV-2 infection as well. It is also likely that IL-4 and IL-5 would be elevated since they are downstream in the signaling cascade of TNFα. Thus, viral induced leukotriene synthesis and a corresponding increase in mucus secretion is likely to be present in COVID-19.
Protective Role of Cytokines
Considering the severity of inflammation and its importance in COVID-19 patients, it is crucial to identify factors that contribute in the inflammatory response. These include anti-inflammatory cytokines and antibodies, some of which are currently used to treat other inflammatory conditions in the respiratory tract.
Anti-Inflammatory Role of IL-37
Multiple studies have reported significant anti-inflammatory properties of IL-37 and its mode of action in recent years.Citation86,Citation87 These studies demonstrated that IL-37 is capable of inhibiting pro-inflammatory effects that are mediated through activation of receptors belonging to the interleukin-1 receptor/toll-like receptor (TIR) superfamily such as TIRs 2 and 4 and the IL-1 receptor.Citation86
IL-37 has shown to modulate inflammation by downregulating response of Th1, Th2, and Th17 cells.Citation88 Studies have demonstrated that locally administering IL-37 can reduce eosinophil levels in bronchoalveolar (BAL) fluid and respiratory tract tissues. Upon binding to IL-18R, IL-37 suppresses the expression of IL1α, IL6, IL1β, TNFα, GCSF, and GMCSF via JAK/STAT pathway.Citation89,Citation90 IL-37 inhibits NF-κB activation of S100A9 via STAT3 and p62.
Anti-Inflammatory Role of IL-27
IL-27 is a heterodimeric cytokine. IL-27 is primarily secreted by activated macrophages and dendritic cells.Citation91–Citation93 Binding of IL-27 to its receptor Il-27R and gp130 leads to activation of STAT1 and inhibits GATA-3 which further downregulates IL-4 and reduces mucin production via MUC5AC.
Anti-Inflammatory Role of IL-35
Interleukin-35 acts as an anti-inflammatory cytokine which is secreted by T cells and B cells.Citation94 Studied have found that IL-35 induces proliferation of regulatory T cells, inhibiting CD4+ effector cells, and suppressing the development of Th17 cells.Citation95 Upon binding to IL-12Rβ2/gp130, IL-35 activates the JAK/STAT pathway to inhibits GATA3, thereby regulating the expression of MUC5AC.Citation96
Anti-Inflammatory Role of IL-38
IL-38 belongs to the interleukin-1 family.Citation97 IL 38 plays a significant role in inflammation and immune responses, acting against pathogenic microorganisms. IL-38 has a binding affinity to IL-1R and IL-36R and inhibits the MAPK mediated downstream signaling, leading to the decreased activation of cytokines through AP1 thereby modulating inflammation, as shown in .Citation98–Citation102
As shown in various in vitro studies and in animal models for chronic inflammatory diseases, inhibition of specific inflammatory pathways results in diminished production of pro-inflammatory cytokines such as IL-1α, IL-1β, IL-6, IL-17. In addition to neutralization of single pro-inflammatory cytokines, the use of anti-IL6 and anti-IL1 drugs may lead to better control of cytokine storms in COVID-19 patients.
Discussion
Inflammation in the mucosa is the main pathophysiological mechanism leading to congestion in several respiratory tract diseases. It is particularly heightened in COVID-19 due to elevated pro-inflammatory cytokines. The build-up of mucus can also contribute to other complications found in COVID-19 such as venous engorgement, elevation in nasal secretions, and pulmonary edema. Thus, regulation of these inflammatory cascades might be crucial in the treatment of severely ill COVID-19 patients.
Anti-Interleukin Drugs
The use of drugs that inhibit key inflammatory signaling molecules (viz. IL-1β, IL-6, TNFα) may be used. Th2 cytokines, such as IL-5, have been the main therapeutic targets for eosinophilic inflammation-associated pulmonary disease. For the treatment of asthma, the Food and Drug Administration (FDA) approved the use of mepolizumab, an anti IL-5 drug candidate, in 2015.Citation82 Since then, reslizumab and benralizumab are two more anti-IL-5 drugs that have also been given FDA approval for use in asthma.Citation83–Citation85 Sarilumab, siltuximab and tocilizumab are inhibitors of IL-6 that are approved by the FDA for use in diseases such as Castleman disease, rheumatologic disorders and cytokine release syndrome.Citation103 Anakinra is an IL-1 inhibitor that the FDA approved for use in rheumatoid arthritis and cryopyrin-associated periodic syndromes.Citation104 Several clinical trials are underway for the use of these inhibitors in COVID-19. A pilot multicentre study found encouraging results with tocilizumab given to patients with severe COVID-19.Citation105 Current drugs and their trials are shown in .
Table 1 Potential Therapeutic Drugs and Their Targets Currently Under Trial Against COVID-19
Anti-Inflammatory Cytokines
The use of anti-inflammatory cytokines such as IL-27, IL-35, IL-37 and IL-38 can also be explored as a novel treatment modality. At present, there is no data available for the safety and efficacy of such cytokine therapy.Citation105,Citation106
Anti-Inflammatory Active Constituents
Lastly, regular intake of anti-inflammatory food products may be helpful in mitigating the cytokine storm. Many everyday food products have anti-inflammatory active constituents whose properties have been studied extensively. Ginger, turmeric, and green tea have active constituents that inhibit IL-1β, IL-6, TNFα, and NF-κB.Citation107–Citation110
In –, several anti-inflammatory active constituents are mentioned in green boxes at the site of their reported action. These active constituents along with the natural sources are listed in Supplementary Table S1 that contain active constituents which are known to inhibit various inflammatory cascades that arise due to the cytokine storm. The effect of incorporating such anti-inflammatory food substances in the diet of COVID-19 patients can be studied without the risk of any undesired effects. Developing such a modality seems particularly crucial in the absence of specific therapies for targeting cytokine storms in COVID-19.
Conclusion
Since mucus is a fundamental mechanism for defense against allergens and pathogens, its production increases in the respiratory tract in nearly every instance of airway inflammation. The cytokine storm in COVID-19 is particularly potent for the build-up of mucus due to the onset of several inflammatory cascades associated with mucus production. It is therefore important to understand these cascades for identification of new therapeutic targets and drug discovery. In the meantime, dietary supplementation of COVID-19 patients with foods that are known to inhibit key inflammatory molecules may provide some degree of relief in the mucus production and other symptoms of airway inflammation.
Acknowledgments
The authors are grateful to Dr Shadab Raza and Dr Mohd. Faheem Khan for their advice in selection of journal for publication. We are also thankful to Dr. M.M.A. Faridi for the encouragement provided by him for the analytical approach of this review. Lastly, we thank the entire management of Era’s Lucknow Medical College & Hospital for supporting this work.
Disclosure
The authors report no conflicts of interest in this work.
References
- Tian S, Hu W, Niu L, Liu H, Xu H, Xiao SY. Pulmonary pathology of early Phase 2019 novel coronavirus (COVID-19) pneumonia in two patients with lung cancer. J Thorac Oncol. 2020;15:700–704. doi:10.1016/j.jtho.2020.02.010
- Bernheim A, Mei X, Huang M. Chest CT findings in coronavirus disease-19 (COVID-19): relationship to duration of infection. Radiology. 2020;295.
- Khashkhosha HK, Elhadi M. A hypothesis on the role of the human immune system in covid-19 published online ahead of print, [2020 Jul 1]. Med Hypotheses. 2020;143:110066. doi:10.1016/j.mehy.2020.110066
- Earhart AP, Holliday ZM, Hofmann HV, Schrum AG. Consideration of dornase alfa for the treatment of severe COVID-19 acute respiratory distress syndrome. New Microbes New Infect. 2020;35:100689. doi:10.1016/j.nmni.2020.100689
- Zhu N, Zhang D, Wang W, et al. A novel coronavirus from patients with pneumonia in China, 2019. N Engl J Med. 2020;382(8):727–733. doi:10.1056/NEJMoa2001017
- Costela-Ruiz VJ, Illescas-Montes R, Puerta-Puerta JM, Ruiz C, Melguizo-Rodríguez L. SARS-CoV-2 infection: the role of cytokines in COVID-19 disease. Cytokine Growth Factor Rev. 2020;54:62–75. doi:10.1016/j.cytogfr.2020.06.001
- Jiang Y, Xu J, Zhou C, et al. Characterization of cytokine/chemokine profiles of severe acute respiratory syndrome. Am J Respir Crit Care Med. 2005;171(8):850–857. doi:10.1164/rccm.200407-857OC
- Upadhyay J, Tiwari N, Ansari MN. Role of inflammatory markers in corona virus disease (COVID-19) patients: a review. Exp Biol Med. 2020;245(15):1368–1375. doi:10.1177/1535370220939477
- Ye Z, Zhang Y, Wang Y, et al. Chest CT manifestations of new coronavirus disease 2019 (COVID-19): a pictorial review. Eur Radiol. 2020;1–9.
- Li X, Ma X. Acute respiratory failure in COVID-19: is it “typical” ARDS? Crit Care. 2020;24(1):198. doi:10.1186/s13054-020-02911-9
- Holtzman MJ, Battaile JT, Patel AC. Immunogenetic programs for viral induction of mucous cell metaplasia. Am J Respir Cell Mol Biol. 2006;35(1):29–39. doi:10.1165/rcmb.2006-0092SF
- Vetrugno L, Baciarello M, Bignami E, et al. The “pandemic” increase in lung ultrasound use in response to Covid-19: can we complement computed tomography findings? A narrative review. Ultrasound J. 2020;12:39. doi:10.1186/s13089-020-00185-4
- Lai SK, Wang YY, Wirtz D, Hanes J. Micro- and macrorheology of mucus. Adv Drug Deliv Rev. 2009;61(2):86–100. doi:10.1016/j.addr.2008.09.012
- Shukla SD, Mahmood MQ, Weston Set al. The main rhinovirus respiratory tract adhesion site (ICAM-1) is upregulated in smokers and patients with chronic airflow limitation (CAL). Respir Res2017;18(1):6 doi:10.1186/s12931-016-0483-8
- Ohar JA, Donohue JF, Spangenthal S. The role of guaifenesin in the management of chronic mucus hypersecretion associated with stable chronic bronchitis: a comprehensive review. Chronic Obstr Pulm Dis. 2019;6(4):341–349.
- Hodges RR, Dartt DA. Conjunctival goblet cells. Encyclopedia Eye. 2010;369–376.
- Knoop KA, Newberry RD. Goblet cells: multifaceted players in immunity at mucosal surfaces. Mucosal Immunol. 2018;11:1551–1557. doi:10.1038/s41385-018-0039-y
- Zanin M, Baviskar P, Webster R, Webby R. The interaction between respiratory pathogens and mucus. Cell Host Microbe. 2016;19(2):159–168. doi:10.1016/j.chom.2016.01.001
- Adler KB, Tuvim MJ, Dickey BF. Regulated mucin secretion from airway epithelial cells. Front Endocrinol. 2013;4:129. doi:10.3389/fendo.2013.00129
- Chen C, Thai P, Yoneda K, et al. A peptide that inhibits the function of Myristoylated Alanine-Rich C Kinase Substrate (MARCKS) reduces lung cancer metastasis. Oncogene. 2014;33:3696–3706. doi:10.1038/onc.2013.336
- Wickström C, Davies JR, Eriksen GV, et al. MUC5B is a major gel-forming, oligomeric mucin from human salivary gland, respiratory tract and endocervix: identification of glycoforms and C-terminal cleavage. Biochem J. 1998;15(334):685–693. doi:10.1042/bj3340685
- Hovenberg HW, Davies JR. Carlstedt.Different mucins are produced by the surface epithelium and the submucosa in human trachea: identification of MUC5AC as a major mucin from the goblet cells. I Biochem J. 1996;15(318):319–324. doi:10.1042/bj3180319
- Dabbagh K, Takeyama K, Lee HM, et al. IL-4 induces mucin gene expression and goblet cell metaplasia in vitro and in vivo. J Immunol. 1999;162:6233–6237.
- Xiang J, Rir-Sim-Ah J, Tesfaigzi Y. IL-9 and IL-13 induce mucous cell metaplasia that is reduced by IFN-gamma in a Bax-mediated pathway. Am J Respir Cell Mol Biol. 2008;38(3):310–317. doi:10.1165/rcmb.2007-0078OC
- Cerveri I, Brusasco V. Revisited role for mucus hypersecretion in the pathogenesis of COPD. Eur Respir Rev. 2010;19(116):109–112. doi:10.1183/09059180.00002710
- Wen FQ, Shen YC. Expectorant therapy revisited in chronic obstructive pulmonary disease. Zhonghua Jie He He Hu Xi Za Zhi. 2011;34(4):243–245.
- Jartti T, Bønnelykke K, Elenius V, Feleszko W. Role of viruses in asthma. Semin Immunopathol. 2020;42(1):61–74. doi:10.1007/s00281-020-00781-5
- Grunstein MM, Hakonarson H, Maskeri N, et al. Autocrine cytokine signaling mediates effects of rhinovirus on airway responsiveness. Am J Physiol. 2000;6:1146–L1153.
- Baños-Lara Mdel R, Piao B, Guerrero-Plata A. Differential mucin expression by respiratory syncytial virus and human metapneumovirus infection in human epithelial cells. Mediators Inflamm. 2015;2015:347292.
- Persson BD, Jaffe AB, Fearns R, Danahay H. Respiratory syncytial virus can infect basal cells and alter human airway epithelial differentiation. PLoS One. 2014;9(7):e102368. doi:10.1371/journal.pone.0102368
- Stokes KL, Currier MG, Sakamoto K, et al. The respiratory syncytial virus fusion protein and neutrophils mediate the airway mucin response to pathogenic respiratory syncytial virus infection. J Virol. 2013;87(18):10070–10082. doi:10.1128/JVI.01347-13
- Farooqi FI, Morgan RC, Dhawan N, Dinh J, Yatzkan G, Michel G. Airway hygiene in COVID-19 pneumonia: treatment responses of 3 critically Ill cruise ship employees. Am J Case Rep. 2020;21:e926596. doi:10.12659/AJCR.926596
- Sungnak W, Huang N, Bécavin C, et al. SARS-CoV-2 entry factors are highly expressed in nasal epithelial cells together with innate immune genes. Nat Med. 2020;26:681–687. doi:10.1038/s41591-020-0868-6
- Bustamante-Marin XM, Ostrowski LE. Cilia and mucociliary clearance. Cold Spring Harb Perspect Biol. 2017;9(4):a028241. doi:10.1101/cshperspect.a028241
- Arumugham V Immunological mechanisms explaining the role of IgE, mast cells, histamine, elevating ferritin, IL-6, D-dimer, VEGF levels in COVID-19 and dengue, potential treatments such as mast cell stabilizers, antihistamines, Vitamin C, hydroxychloroquine, ivermectin and azithromycin. 2020.
- Prompetchara E, Ketloy C, Palaga T. Immune responses in COVID-19 and potential vaccines: lessons learned from SARS and MERS epidemic. Asian Pac J Allergy Immunol.
- Cohn L, Homer RJ, Marinov A, Rankin J, Bottomly K. Induction of airway mucus production By T helper 2 (Th2) cells: a critical role for interleukin 4 in cell recruitment but not mucus production. J Exp Med. 1997;186(10):1737–1747. doi:10.1084/jem.186.10.1737
- Thai P, Chen Y, Dolganov G, Wu R. Differential regulation of MUC5AC/Muc5ac and hCLCA-1/mGob-5 expression in airway epithelium. Am J Respir Cell Mol Biol. 2005;33(6):523–530. doi:10.1165/rcmb.2004-0220RC
- Kreda SM, Davis CW, Rose MC. CFTR, mucins, and mucus obstruction in cystic fibrosis. Cold Spring Harb Perspect Med. 2012;2(9):a009589. doi:10.1101/cshperspect.a009589
- Saint-Criq V, Gray MA. Role of CFTR in epithelial physiology. Cell Mol Life Sci. 2017;74(1):93–115.
- Almughem FA, Aldossary AM, Tawfik EA, et al. Cystic fibrosis: overview of the current development trends and innovative therapeutic strategies. Pharmaceutics. 2020;12(7):616.
- Jarosz-Griffiths HH, Scambler T, Wong CH, et al. Different CFTR modulator combinations downregulate inflammation differently in cystic fibrosis. Elife. 2020;9:e54556. doi:10.7554/eLife.54556
- Griffin DO, Jensen A, Khan M, et al. Pulmonary embolism and increased levels of d-dimer in patients with coronavirus disease. Emerg Infect Dis. 2020;26(8):1941–1943. doi:10.3201/eid2608.201477
- Huang C, Wang Y, Li X, et al. Clinical features of patients infected with 2019 novel coronavirus in Wuhan, China [published correction appears in Lancet. 2020 Jan 30]. Lancet. 2020;395(10223):497–506. doi:10.1016/S0140-6736(20)30183-5
- Liu K, Fang YY, Deng Y, et al. Clinical characteristics of novel coronavirus cases in tertiary hospitals in Hubei Province. Chin Med J (Engl). 2020;133(9):1025–1031. doi:10.1097/CM9.0000000000000744
- Wang W, Liu X, Wu S, et al. The definition and risks of cytokine release syndrome in 11 COVID-19-affected critically ill patients with pneumonia: analysis of disease characteristics. J Infect Dis. 2020;jiaa387.
- Allinson JP, Hardy R, Donaldson GC, et al. The presence of chronic mucus hypersecretion across adult life in relation to chronic obstructive pulmonary disease development. Am J Respir Crit Care Med. 2016;193(6):662–667. doi:10.1164/rccm.201511-2210OC
- Busse PJ, Zhang TF. Kamal, et al. Chronic exposure to TNF-alpha increases airway mucus gene expression in vivo. J Allergy Clin Immunol. 2005; 116(6):1256–63.
- Girija ASS, Shankar EM, Larsson M. Could SARS-CoV-2-induced hyperinflammation magnify the severity of Coronavirus Disease (CoViD-19) leading to acute respiratory distress syndrome? Front Immunol. 2020;11:1206. doi:10.3389/fimmu.2020.01206
- Xia Y, Cai P, Yu F, et al. IL-4-induced caveolin-1-containing lipid rafts aggregation contributes to MUC5AC synthesis in bronchial epithelial cells. Respir Res. 2017;18:174. doi:10.1186/s12931-017-0657-z
- Gharavi NM, Alva JA, Kevin P, et al. Role of the JAK/STAT pathway in the regulation of IL-8 transcription by oxidized phospholipids in vitro and in atherosclerosis in vivo. J Biol Chem. 2007;282(43):31460–31468. doi:10.1074/jbc.M704267200
- Yu H, Li Q, Kolosov VP, et al. Interleukin-13 induces mucin 5AC production involving STAT6/SPDEF in human airway epithelial cells. Cell Commun Adhes. 2010;17(4–6):83–92. doi:10.3109/15419061.2010.551682
- Grunig G, Warnock M, Wakil AE, et al. Requirement for IL-13 independently of IL-4 in experimental asthma. Science. 1998;282:2261–2264. doi:10.1126/science.282.5397.2261
- Kelly-Welch AE, Hanson EM, Boothby MR, et al. Interleukin-4 and interleukin-13 signalling connection maps. Science. 2003;300:1527–1528. doi:10.1126/science.1085458
- Seibold MA. Interleukin-13 stimulation reveals the cellular and functional plasticity of the airway epithelium. Ann Am Thorac Soc. 2018;15(2):S98–S102.
- Nakanishi K. Unique action of Interleukin-18 on T cells and other immune cells. Front Immunol. 2018. 9:763. doi:10.3389/fimmu.2018.00763
- Goswami R, Kaplan MH. A brief history of IL-9. J Immunol. 2011;186(6):3283–3288. doi:10.4049/jimmunol.1003049
- Demoulin JB, Uyttenhove C, Van Roost E, et al. A single tyrosine of the interleukin-9 (IL-9) receptor is required for STAT activation, antiapoptotic activity, and growth regulation by IL-9. Mol Cell Biol. 1996;16(9):4710–4716.
- Fung M, Chu Y, Fink J, et al. IL-2- and STAT5-regulated cytokine gene expression in cells expressing the Tax protein of HTLV-1. Oncogene. 2005;24:4624–4633. doi:10.1038/sj.onc.1208507
- Wilson MS, Pesce JT, Ramalingam TR, et al. Suppression of murine allergic airway disease by IL-2: anti-IL-2monoclonal antibody-induced regulatory T cells. J Immunol. 2008;181(10):6942–6954. doi:10.4049/jimmunol.181.10.6942
- Bao L, Zhang H, Chan LS. The involvement of the JAK-STAT signaling pathway in chronic inflammatory skin disease atopic dermatitis. JAKSTAT. 2013;2(3):e24137.
- Li L, Zhao GD, Shi Z, Qi LL, Zhou LY, Fu ZX. The Ras/Raf/MEK/ERK signaling pathway and its role in the occurrence and development of HCC. Oncol Lett. 2016;12(5):3045–3050. doi:10.3892/ol.2016.5110
- Paunovic V, Harnett MM. Mitogen-activated protein kinases as therapeutic targets for rheumatoid arthritis. Drugs. 2013;73(2):101–115.
- Lillehoj EP, Kato K, Lu W, Kim KC. Cellular and molecular biology of airway mucins. Int Rev Cell Mol Biol. 2013;303:139–202.
- Burgos-Blasco B, Güemes-Villahoz N, Santiago JL, et al. Hypercytokinemia in COVID-19: tear cytokine profile in hospitalized COVID-19 patients. Exp Eye Res. 2020;200:108253.
- Lee JJ, McGarry MP, Farmer SC, et al. Interleukin-5 expression in the lung epithelium of transgenic mice leads to pulmonary changes pathognomonic of asthma. J Exp Med. 1997;185(12):2143–2156. doi:10.1084/jem.185.12.2143
- Tesmer LA, Lundy SK, Sarkar S, Fox DA. Th17 cells in human disease. Immunol Rev. 2008;223:87–113.
- Hynes GM, Hinks TSC. The role of interleukin-17 in asthma: a protective response? ERJ Open Res. 2020;6(2):00364–2019. doi:10.1183/23120541.00364-2019
- Coperchini F, Chiovato L, Croce L, Magri F, Rotondi M. The cytokine storm in COVID-19: an overview of the involvement of the chemokine/chemokine-receptor system. Cytokine Growth Factor Rev. 2020;53:25–32. doi:10.1016/j.cytogfr.2020.05.003
- Zhang D, Facchinetti V, Wang X, Huang Q, Qin J, Su B. Identification of MEKK2/3 serine phosphorylation site targeted by the Toll-like receptor and stress pathways. EMBO J. 2006;25(1):97–107.
- Chen Y, Garvin LM, Nickola TJ, Watson AM, Colberg-Poley AM, Rose MC. IL-1β induction of MUC5AC gene expression is mediated by CREB and NF-κB and repressed by dexamethasone. Am J Physiol Lung Cell Mol Physiol. 2014;306(8):L797–807. doi:10.1152/ajplung.00347.2013
- Price MM, Oskeritzian CA, Falanga YT, et al. A specific sphingosine kinase 1 inhibitor attenuates airway hyperresponsiveness and inflammation in a mast cell-dependent murine model of allergic asthma. J Allergy Clin Immunol. 2013;131(2):501–11.e1. doi:10.1016/j.jaci.2012.07.014
- Kankaanranta H, Ilmarinen P, Zhang X, et al. Tumour necrosis factor-α regulates human eosinophil apoptosis via ligation of TNF-receptor 1 and balance between NF-κB and AP-1. PLoS One. 2014;9(2):e90298. doi:10.1371/journal.pone.0090298
- Wang X, Lin Y. Tumor necrosis factor and cancer, buddies or foes? Acta Pharmacol Sin. 2008;29(11):1275–1288. doi:10.1111/j.1745-7254.2008.00889.x
- Wang IJ, Wu CY, Hu FR. Effect of proinflammatory cytokines on the human MUC5AC promoter activity in vitro and in vivo. Clin Ophthalmol. 2007;1(1):71–77.
- Thai P, Loukoianov A, Wachi S, Wu R. Regulation of airway mucin gene expression. Annu Rev Physiol. 2008;70:405–429. doi:10.1146/annurev.physiol.70.113006.100441
- Shishikura Y, Koarai A, Aizawa H, et al. Extracellular ATP is involved in dsRNA-induced MUC5AC production via P2Y2R in human airway epithelium. Respir Res. 2016;17(1):121. doi:10.1186/s12931-016-0438-0
- Xue L, Barrow A, Fleming VM, et al. Leukotriene E4 activates human Th2 cells for exaggerated proinflammatory cytokine production in response to prostaglandin D2. J Immunol. 2012;188(2):694–702.
- Huber M. Activation/Inhibition of mast cells by supra-optimal antigen concentrations. Cell Commun Signal. 2013;11(1):7. doi:10.1186/1478-811X-11-7
- Rådmark O, Samuelsson B. 5-Lipoxygenase: mechanisms of regulation. J Lipid Res. 2009;50 Suppl(Suppl):S40–5.
- Hedi H, Norbert G. 5-lipoxygenase pathway, dendritic cells, and adaptive immunity. J Biomed Biotechnol. 2004;2004(2):99–105. doi:10.1155/S1110724304310041
- Leckie MJ, Ten Brinke A, Khan J, et al. Effects of an interleukin-5 blocking monoclonal antibody on eosinophils, airway hyper-responsiveness, and the late asthmatic response. Lancet. 2000;35(6):2144–2148. doi:10.1016/S0140-6736(00)03496-6
- Deeks ED, Brusselle G. Reslizumab in eosinophilic asthma: a review. Drugs. 2017;77:777–784.
- Brightling CE, Bleecker ER, Panettieri RA Jr, et al. Benralizumab for chronic obstructive pulmonary disease and sputum eosinophilia: a randomised, double-blind, placebo-controlled, phase 2 study. Lancet Respir Med. 2014;2:891–901. doi:10.1016/S2213-2600(14)70187-0
- Arora S, Ahmad S, Irshad R, et al. TLRs in pulmonary diseases. Life Sci. 2019;15(233):116671. doi:10.1016/j.lfs.2019.116671
- Wang L, Quan Y, Yue Y, Heng X, Che F. Interleukin-37: a crucial cytokine with multiple roles in disease and potentially clinical therapy. Oncol Lett. 2018;15(4):4711–4719.
- Zhang L, Zhang J, Gao P. The potential of interleukin-37 as an effective therapeutic agent in asthma. Respir Res. 2017;18(1):192. doi:10.1186/s12931-017-0675-x
- Nold MF, Nold-Petry CA. Zepp, et al. IL-37 is a fundamental inhibitor of innate immunity. Nat Immunol. 2010;11:1014–1022. doi:10.1038/ni.1944
- Nold-Petry CA, Lo CY, Rudloff I, et al. IL-37 requires the receptors IL-18Ralpha and IL-1R8 (SIGIRR) to carry out its multifaceted anti-inflammatory program upon innate signal transduction. Nat Immunol. 2015;16:354–365. doi:10.1038/ni.3103
- Abdalla AE, Li Q, Xie L, Xie J. Biology of IL-27 and its role in the host immunity against Mycobacterium tuberculosis. Int J Biol Sci. 2015;11(2):168–175. doi:10.7150/ijbs.10464
- Bosmann M, Ward PA. Modulation of inflammation by interleukin-27. J Leukoc Biol. 2013;94(6):1159–1165. doi:10.1189/jlb.0213107
- de Almeida Nagata DE, Demoor T, Ptaschinski C, et al. IL-27R-mediated regulation of IL-17 controls the development of respiratory syncytial virus-associated pathogenesis. Am J Pathol. 2014;184(6):1807–1818. doi:10.1016/j.ajpath.2014.02.004
- Ito T, Tanaka T, Nakamaru K, et al. Interleukin-35 promotes the differentiation of regulatory T cells and suppresses Th2 response in IgG4-related type 1 autoimmune pancreatitis. J Gastroenterol. 2020;55(8):789–799. doi:10.1007/s00535-020-01689-5
- Huang A, Cheng L, He M, Nie J, Wang J, Jiang K. Interleukin-35 on B cell and T cell induction and regulation. J Inflamm (Lond). 2017;14(16). doi:10.1186/s12950-017-0164-5
- Heinrich PC, Behrmann I, Müller-Newen G, Schaper F, Graeve L. Interleukin-6-type cytokine signalling through the gp130/Jak/STAT pathway. Biochem J. 1998;334(Pt 2):297–314. doi:10.1042/bj3340297
- Seelaender M, Neto JC, Pimentel GD, Goldszmid RS, Lira FS. Inflammation in the disease: mechanism and therapies 2014. Mediators Inflamm. 2015;2015:169852. doi:10.1155/2015/169852
- Van de Veerdonk FL, Stoeckman AK, Wu G, et al. IL-38 binds to the IL-36 receptor and has biological effects on immune cells similar to IL-36 receptor antagonist. Proc Natl Acad Sci U S A. 2012;109(8):3001–3005. doi:10.1073/pnas.1121534109
- Pavord ID, Chanez P, Criner GJ, et al. Mepolizumab for Eosinophilic Chronic Obstructive Pulmonary Disease. N Engl J Med. 2017;377:1613–1629.
- Queen D, Ediriweera C, Liu L. Function and regulation of IL-36 signaling in inflammatory diseases and cancer development. Front Cell Dev Biol. 2019;7:317. doi:10.3389/fcell.2019.00317
- Boutet MA, Nerviani A, Pitzalis C. IL-36, IL-37, and IL-38 cytokines in skin and joint inflammation: a comprehensive review of their therapeutic potential. Int J Mol Sci. 2019;20(6):1257. doi:10.3390/ijms20061257
- Madonna S, Girolomoni G, Dinarello CA, Albanesi C. The significance of IL-36 hyperactivation and IL-36R targeting in psoriasis. Int J Mol Sci. 2019;20(13):3318. doi:10.3390/ijms20133318
- Bassoy EY, Towne JE, Gabay C. Regulation and function of interleukin-36 cytokines. Immunol Rev. 2018;281(1):169–178. doi:10.1111/imr.12610
- Atal S, Fatima Z. IL-6 inhibitors in the treatment of serious COVID-19: a promising therapy? Pharmaceut Med. 2020;34(4):223–231.
- Goldbach-Mansky R. Blocking interleukin-1 in rheumatic diseases. Ann N Y Acad Sci. 2009;1182(1):111–123. doi:10.1111/j.1749-6632.2009.05159.x
- Sciascia S, Aprà F, Baffa A, et al. Pilot prospective open, single-arm multicentre study on off-label use of tocilizumab in patients with severe COVID-19. Clin Exp Rheumatol. 2020;38(3):529–532.
- Russell B, Moss C, George G, et al. Associations between immune-suppressive and stimulating drugs and novel COVID-19-a systematic review of current evidence. Ecancermedicalscience. 2020;14:1022. doi:10.3332/ecancer.2020.1022
- Kim JH, Gupta SC, Park B, Yadav VR, Aggarwal BB. Turmeric (Curcuma longa) inhibits inflammatory nuclear factor (NF)-κB and NF-κB-regulated gene products and induces death receptors leading to suppressed proliferation, induced chemosensitization, and suppressed osteoclastogenesis. Mol Nutr Food Res. 2012;56(3):454–465. doi:10.1002/mnfr.201100270
- Ghandadi M, Sahebkar A. Curcumin: an effective inhibitor of interleukin-6. Curr Pharm Des. 2017;23(6):921–931. doi:10.2174/1381612822666161006151605
- Mao QQ, Xu XY, Cao SY, et al. Bioactive compounds and bioactivities of ginger (Zingiber officinale Roscoe). Foods. 2019;8(6):185. doi:10.3390/foods8060185
- Ohishi T, Goto S, Monira P, Isemura M, Nakamura Y. Anti-inflammatory action of green tea. Antiinflamm Antiallergy Agents Med Chem. 2016;15(2):74–90. doi:10.2174/1871523015666160915154443
- Cabrita I, Benedetto R, Schreiber R, Kunzelmann K. Niclosamide repurposed for the treatment of inflammatory airway disease. JCI Insight. 2019;4(15):e128414. doi:10.1172/jci.insight.128414
- Tufts Medical Center. Niclosamide for mild to moderate COVID-19. NLM identifier: NCT04399356. Available from: https://clinicaltrials.gov/ct2/show/NCT04399356. Accessed September 1, 2020.
- Miklossy G, Hilliard TS, Turkson J. Therapeutic modulators of STAT signalling for human diseases. Nat Rev Drug Discov. 2013;12(8):611–629. doi:10.1038/nrd4088
- Yale University. Tofacitinib for Treatment of Moderate COVID-19 (I-TOMIC). NLM identifier: NCT04415151. Available from: https://clinicaltrials.gov/ct2/show/NCT04415151. Accessed July 29, 2020..
- Gerriets V, Bansal P, Goyal A, et al. Tumor Necrosis Factor (TNF) Inhibitors. [Updated 2020 Jul 4]. In: StatPearls [Internet]. Treasure Island (FL): StatPearls Publishing; 2020 Jan. Available from: https://www.ncbi.nlm.nih.gov/books/NBK482425/. Accessed December 11, 2020.
- Tufts Medical Center. A Phase 2 trial of infliximab in coronavirus disease 2019 (COVID-19). NLM identifier: NCT04425538. Available from: https://clinicaltrials.gov/ct2/show/NCT04425538. Accessed June 1, 2020.
- Choy EH, De Benedetti F, Takeuchi T, Hashizume M, John MR, Kishimoto T. Translating IL-6 biology into effective treatments. Nat Rev Rheumatol. 2020;16(6):335–345. doi:10.1038/s41584-020-0419-z
- Genentech, Inc. A study to evaluate the efficacy and safety of tocilizumab in hospitalized participants with COVID-19 pneumonia. NLM identifier: NCT04372186. Available from: https://clinicaltrials.gov/ct2/show/NCT04372186. Accessed May 14, 2020.
- Wu KK, Dao H Jr. Off-label dermatologic uses of IL-17 inhibitors [published online ahead of print, 2020 Mar 9]. J Dermatolog Treat. 2020;1–7. doi:10.1080/09546634.2020.1737638
- Lomonosov Moscow State University Medical Research and Educational Center. COLchicine versus ruxolitinib and secukinumab in open prospective randomized trial (COLORIT). NLM identifier: NCT04403243. Available from: https://clinicaltrials.gov/ct2/show/NCT04403243. Accessed May 8, 2020.
- Fenini G, Contassot E, French LE. Potential of IL-1, IL-18 and inflammasome inhibition for the treatment of inflammatory skin diseases. Front Pharmacol. 2017;8:278. doi:10.3389/fphar.2017.00278
- University Hospital, Basel, Switzerland. Canakinumab in patients with COVID-19 and Type 2 diabetes (CanCovDia). NLM identifier: NCT04510493. Available from: https://clinicaltrials.gov/ct2/show/NCT04510493. Accessed September 2020.