Abstract
There is a huge unmet need for eye care with more than a hundred million people living without basic eye care services and facilities. There is an exigency to deploy adequate resources in terms of manpower and equipment to address this. The usage of smart devices in optometry and eye care practice has been gaining momentum for last half a decade, due to the COVID-19 pandemic and technological advancements in telemedicine. These smart devices will help facilitate remote monitoring of important visual functions, ocular signs and symptoms, thus providing better eye care services and facilities and promoting outreach services. Smart devices in optometry exist in the form of gadgets that can be worn in the wrist, and spectacle-mounted or head-mounted devices. On the other hand, with the ubiquitous nature of smartphones, a large number of smartphone applications have been developed and tested for advanced optometry and primary eye care practice, which may potentially reduce the burden of inadequate resources and the unmet need for eye care. This article aims to give an overview of the current trends and future perspectives on the application of such smart devices in optometric practice.
Introduction
The burden of visual impairment is expected to increase world-wide with estimated numbers to go as high as 9.8 billion by 2050.Citation1 The burden of vision loss is likely to surge from 0.24 billion in 2020 to 0.35 billion by the year 2050. The load of near visual loss due to presbyopia will be about 1000 million by the year 2050.Citation1
Providing better eye health is an important component of the Sustainable Development Goal (SDG) namely Good health and well-being. Improving the eye health will have a positive impact and will help to improve several other SDGs including quality education, employability and economic growth. Adapting mobile health and artificial intelligence-based technologies play a vital role in improving eye health.Citation2 Use of mobile health is reported to be an effective eye care service delivery model.Citation3 In order to ensure that people receive continuous eye care services, WHO has proposed a new model “Integrated People Centered Eye Care (IPCEC)” that focuses on inclusive patient centric vision care to overcome the pitfalls in eye care delivery. Adopting Artificial Intelligence-based technologies in eye care is suggested as an effective way to make the IPCEC model more vigorous.Citation4
Optometrists, as primary eye care professionals, play a crucial role in providing universal eye health coverage through refractive error management, diagnosis and timely referral. Alongside the delivery of universal eye health, it is essential to stay abreast of the existing smart devices in optometry for having improved efficiency during clinical application.
Several systematic reviews and meta-analysis have been published earlier, which provide a summary of available smartphone-based visual acuity applications and how to integrate them into clinical practice.Citation5–8
This article provides an overview of the features as well as the uses of the available smart devices in optometry including the smartphone-based apps as well as the wearable device technology that can be integrated into primary eye care practice by optometrists for visual acuity screening, diagnosis of ocular conditions and low vision rehabilitation.
Smart Devices for Optometry and Eye Care
Smart devices for primary eye care can be broadly classified into smartphone-based technology and wearables and this review will focus on the same as indicated in the flow chart ().
Smartphone Based Technology
A multitude of applications (apps) are available in both Google Play Store and Appstore for optometric practice including those for testing important visual functions namely visual acuity, colour vision, contrast sensitivity and visual fields as well as for other clinical measures.Citation8 These apps can be categorised in to single featured, multi-featured, eye exercise, eye protection and eye care, dispensing and education apps. Single featured apps are those that incorporate tests for single clinical parameter, for example, visual acuity. Multifeatured apps are those that combine tests for more than one clinical parameter, for instance, visual acuity, color vision and contrast sensitivity.
Vision Screening Apps
The vision screening apps were searched on the online iPhone and Android store. The search terms included “visual acuity”, “vision screening”, “eye screening”, “eye examination”, “eye test”, “vision app”, “vision chart” and “eye test chart”. Articles on validated apps were obtained through literature search in “PubMed”, “Scopus” and “ResearchGate” and the keywords included, “visual acuity” OR “vision screening” OR “refractive error” AND “smartphone apps” OR “mobile apps” OR “validated apps” OR “development of vision apps”. The apps were downloaded in the smartphone and the features were reviewed.
Among the vision screening apps, majority of them have been designed to assess the distance visual acuity with or without near visual acuity test. These apps can be used for home monitoring, community outreach activities such as vision screening and in routine clinical practice. Since few have been validated, the remainder of the apps needs to be validated through clinical studies. The clinically validated distance and near visual acuity apps are summarised in and respectively and those that are not validated in . This review will focus on the clinically validated apps for distance and near vision testing.
Table 1 Clinically Validated Smartphone Apps for Testing Distance Vision
Table 2 Clinically Validated Smartphone Apps for Testing Near Visual Acuity
Table 3 Smartphone Apps for Vision Screening (Not Validated)
Distance Vision Testing Apps
The distance visual acuity measured using some of these apps has been validated against the standard clinical measure from Snellen and/or the ETDRS after converting into logarithm of Minimum Angle of Resolution (logMAR) value.
Eye Chart App developed for iOS, is an iPhone app that measures both distance and near vision. To test distance vision, the app presents Sloan letter at 4 ft (1.2m) distance and gives visual acuity in Snellen metric notation. This app has been validated against Snellen and ETDRS chart in 24 young adults aged 18–27-year-old. After conversion to logMAR value, the distance visual acuity measured using the Eye Chart app (−0.09 ± 0.07 logMAR) differed from the Snellen measure (−0.13 ± 0.08 logMAR, p = 0.0003), but was similar to the ETDRS measure (−0.11 ± 0.08 logMAR, p = 0.076).Citation9
Eye Chart Pro is an extended version of the Eye Chart app that is available as iPad version and includes useful charts like ETDRS, Sloan, Snellen and HOTV. The ETDRS chart available in the app was tested and validated against the ETDRS chart in 25 normal sighted patients (mean age: 71.5 ± 10.7 years) and 26 low vision patients (mean age: 65 ± 19.7 years). The testing was done at 4m for the normally sighted group and 1m for the low vision group. The mean logMAR visual acuity measured using the app (0.53 ± 0.42) and ETDRS chart (0.53 ± 0.42) was the same for all participants (p = 0.98). Although a difference was seen in the mean visual acuity measured from the app and the ETDRS within the normally sighted participants (App: 0.20 ± 0.10; VA ETDRS: 0.12 ± 0.12, p = 0.006) as well as with in the low vision group (VA App: 0.81 ± 0.38 Vs VA ETDRS 0.88 ± 0. 39, p < 0.0001), the absolute difference in visual acuity was not significantly different between the app (0.11 ± 0.07) and ETDRS chart (0.11 ± 0.07). The app overestimated the vision among normally sighted participants, whereas it underestimated the vision among participants with low vision. Hence, results should be interpreted with caution while testing normally sighted individuals and low vision patients using the app in clinical settings.Citation10
AAPOS vision screening App is an iOS-based app developed by the “American Association for Pediatric Ophthalmology and Strabismus (AAPOS)” and available for testing in iPad. This app was developed to screen preschool children aged 3–6 years for reduced visual acuity, in particular for home-based screening. The app consists of Lea symbols chart and a Sloan chart and the testing distance is 3m. The Lea symbol chart of the app has been validated against the LEA symbol chart in 5–6-year-old pre-schoolers (n = 195). Visual acuity was measured by parents, trained ophthalmologists and optometrists and measurements across examiners were compared. The app had a sensitivity of 79% to 86% and a specificity of 72% to 79% for identifying visual impairment when administered by parents. It also provided a highly repeatable measure of visual acuity (Cronbach’s alpha > 0.71, P < 0.0001) across multiple examiners, viz, parents, Ophthalmologists and Optometrist.Citation11
PEEK acuity is an Android-based app that is yet another widely used app for vision screening. It consists of tumbling E that is projected in an Android tablet at a testing distance of 2m. This app has been validated against Snellen and ETDRS charts among 300 adults aged 55 years and above, in the “Nakuru Eye Disease Cohort Study”.Citation12 The visual acuity measured using the app was in agreement with that of Snellen and ETDRS with a mean difference of 0.08 logMAR (95% CI: 0.06–0.10) and 0.07 (95% CI: 0.05–0.09), respectively. The app also showed a minimal test–retest variability of within 0.033 logMAR, with a minimal training and a faster test duration of about a minute.
PEEK acuity app has also been validated in a Randomized Controlled Trial among three to-17-year-old children (n = 111) against the standard ophthalmological examination for visual impairment and referral. The PEEK acuity app showed an overall sensitivity of 83% to 86% for reduced visual acuity and 69% to 83% for ophthalmic referral.Citation13
Vis-Screen app is an Android app that was developed for screening visual impairment and blindness among children by using an algorithm based on specific visual acuity cut-off points viz., 3/60, 6/60, 6/18 and 6/12 to screen for different grades of visual impairment.Citation14,Citation15 The app is available for Android smartphones and tablets and displays Tumbling E optotype. The testing distance is 1.5m for smartphones and 3m for tablets. When pilot tested among children aged 4 years and younger, the app gave a highest sensitivity of about 93% for the 6/12 visual acuity cut-off point, with a specificity higher than 89% for all cut-off points.Citation14 In a further study among children aged 4 years and younger (n = 408), the app yielded a sensitivity of 88.4% for the visual acuity cut-off point of 6/12, which decreased to about 82%, 65%, and 56% respectively for 6/18, 6/60 and 3/60 cut-off points. However, the app yielded a specificity ranging between 95% and 99% for all cut-off points. The AUC (Area Under the Curve) was greater than 0.90 for all visual acuity cut-off points, showing that this app is a valid test for screening visual impairment and blindness.Citation15
Near Vision Testing Apps
For near vision testing, some of the clinically validated apps are the Langone app, Eye Hand-book, Eye Chart near visual acuity app, Smart Vision Check, and e-MOVA app, OdySight and Smart Optometry.
Langone Eye test app was developed by the New York University’s Langone Health Medical Centre and is available in both iOS and Android versions. It tests near visual acuity at a distance of 14 inches using numbers. This app was validated against the standard Rosenbaum near point card among 125 adults aged 18 years and older. Both the iOS and Android versions of the app showed a good agreement with the Rosenbaum near vision with a mean difference of 0.024 ± 0.35 logMAR and 0.016 ± 0.36 logMAR respectively for iOS and Android versions.Citation16
Eye Hand Book app was developed by the “American Academy of Ophthalmology” and is available for both iOS-and Android platforms. It consists of several eye tests including those for distance and near vision, contrast sensitivity and colour vision. The testing distances are 10 ft and 14 inches respectively for distance and near visual acuity testing. The near vision test of this app was validated against Rosenbaum near point card in 100 subjects aged 18–89 years. The mean near visual acuity measured using the app (0.14 ± 0.13 logMAR) and the Rosenbaum near point card (0.23 ± 0.19 logMAR) were significantly different (p < 0.0001). Bland-Altman analysis showed a significant difference between the two tests with a mean difference of 0.11 logMAR (95% CI: −0.08 to 0.27), with the app overestimating the near vision by about one line.Citation17
Eye Chart near visual acuity app (described earlier under distance visual acuity app section) has also been validated against the near visual acuity measured using the Rosenbaum chart at 40 cms among 151 adults older than 18 years (mean age: 64.3 ± 12.5 years). The app showed a good agreement with the Rosenbaum near chart with the visual acuity differing by about 0.04 ± 0.10 logMAR (95% CI: −0.16 to 0.24 logMAR), indicating a two letter mean difference.Citation18
Smart Vision Check is an exclusive, iOS-based app for testing Near Functional Visual Acuity (NFVA) in presbyopes.Citation19 Functional visual acuity represents the mean measure of visual acuities measured across a specific time frame, rather than a single measure. It is a good indicator of visual performance in daily tasks and helpful in measuring vision quality.Citation20–22 It displays a landolt C optotype in iPhone and the patients are required to detect the direction of the landolt ring. This app measures visual acuity at a distance of 40 cms, over 60s timeframe and automatically computes the near functional visual acuity. Near Functional visual acuity measured by this app was compared to the measures from Kowa AS-28, a table top device for NFVA measurement among 115 participants (mean age: 42.5 ± 10.8 years).Citation19 There was a good agreement between the two methods, with an average visual acuity difference of 0.03 logMAR (95% CI − 0.01 to 0.06), with limits of agreement within ± 0.34 logMAR.
eMOVA, the “Electronic Measurement Of Visual Acuity” app developed by researchers at the University of Strasbourg, is an Android-based app that tests the near visual acuity in paediatric group (3–10 years). It uses tumbling E optotypes and the testing distance is 40 cm. This app was validated among 3 to 8-year-old children (n = 96) against the Rossano Weiss test, a paediatric near point card consisting of tumbling E optotypes, numbers and pictures. The average visual acuity difference between the two tests was −0.06 logMAR (95% CI: −0.48 to 0.36) and −0.01 logMAR (95% CI: −0.40 to 0.38) in the right and left eyes,Citation23 indicating a 3-letter difference in right eye and less than a letter difference in the left eye.
OdySight is a smartphone medical application tool that works on both Android and iOS platforms. It consists of distance and near vision tests along with contrast sensitivity test and Amsler grid. It uses tumbling E optotype and has been validated against the standard ETDRS near chart at 40 cm among 78 participants aged 18 years and older. Participants were divided into three cohorts based on the near visual acuity: Cohort 1: LogMAR 0.7 to 1.0; Cohort 2: LogMAR 0.3 to 0.6 and Cohort 1: LogMAR 0.00 to 0.2. The mean difference in visual acuity was about 0.53 letters (95% CI: 10.82 letters to −9.75 letters).Citation24 Although the mean difference agreed well with ETDRS chart, the agreement limits indicate that the app either overestimated or underestimated the visual acuity in some participants in cohorts 1 and 2, with reduced near visual acuity; sub-group analysis revealed a good agreement between the two tests in cohort 3 with normal near visual acuity. Thus, caution should be taken while using this app for patients with near vision impairment.
SMART Optometry App is available in both Android & iOS versions and consists of multiple optometric tests including visual acuity, Amsler, contrast sensitivity and colour vision tests. It tests the near visual acuity in decimal point at 40 cms. The near visual acuity test from this app was validated against standard near vision chart in 5–16-year-old children (n = 100). The mean difference in near vision between the two procedures was 0.018 (95% CI, 0.002–0.034), with the app giving statistically higher near VA (0.13 ± 0.2 logMAR) compared to the near chart (0.12 ± 0.2 logMAR; p = 0.024) VA with the “Smart Optometry” app, underestimating by 2 letters on an average. The overall sensitivity of this app to detect reduced visual acuity was 89.3% with a maximum sensitivity of about 90% and 92% among children aged 5–8-years and 9–12-years, compared to 80% in the older children aged 13–16 years However, the overall specificity to detect reduced visual acuity was only about 69.4%, with the highest specificity of 80% in the older children compared to the 57% in 5 to 8 year old and 75.9% in 9 to 12 year old children.Citation25
Smart Diagnostic Tools for Anterior & Posterior Segment
A comprehensive search of English-language studies up to November 10, 2023, was performed on Google Scholar, Scopus, Web of Science, PubMed, Medline, and Embase. The key terms used were “Smartphone apps”, “Virtual reality”, “Augmented reality” and “Smart devices”.
The field of ophthalmology has witnessed multifold innovations in the form of smart devices that assist in the clinical evaluation of patients and diagnosis at a tertiary eye care center, in screening, imaging and image analysis. Smartphones have been utilized in the identification of keratoconus. The technology involves capturing reflected corneal images of the Placido’s disc and acquiring images of corneal topography as well as tomography for identifying any stage of keratoconus. The abnormal images can then be transferred to the main server for review and advice by physicians at the base hospital. The advantage of this detection method is the convenient portability of the device, particularly useful for screening adolescents and young adults during school screenings.Citation26
Smartphones can also be clipped to slit lamps and optical coherence tomography (OCT) to enable real-time examination of ocular structures, anterior and posterior segment imaging. They are equipped with real-time screen-sharing without having the ophthalmologist attend in person. The only exception is that, in the absence of a slit lamp, there is a need for a 20-D lens to examine the fundus of a patient. The image can then be captured onto the smartphone or the iPad and saved or transferred to the health care physician. In conclusion, smartphones and i-pads have brought phenomenal growth to hand-held imaging, portability, and sharing of data.Citation27
Home Monitoring Devices
Several ophthalmic devices are utilized in various applications, aiding in the detection or monitoring of ocular conditions. The recently developed IOPerfect allows for home monitoring of patient intraocular pressure (IOP) without the need for a local anesthetic. In contrast to various IOP-measuring techniques available, this technique is contactless, takes lesser capturing time, and is not affected by the corneal thickness. Mild air pressure is applied to the patient’s cornea from a headgear, while the difference in the blood vessels to the applied pressure is captured by a micro-video camera. The data are transferred internally to the physician for review.Citation28
Home-based optical coherence tomography (OCT) systems have also emerged that incorporate Artificial Intelligence technology. These provide a quantitative assessment of fluid accumulation in the retina that provides reports to the physicians, who can monitor various stages of the disease. Graphical outputs are provided that present data for comparison at various time points.Citation29
Smart Phone Apps for Low Vision Rehabilitation
In recent years, the development of smartphone-based technology has been advantageous to visually impaired people. These smartphone apps work on human-computer interface and are easily portable, cost-effective and pose less social stigma.Citation30 Smartphone assistive technology promotes social inclusion, and independence of the visually impaired thus enhancing their quality of life.Citation31 Smartphone with accessible interface helps the visually impaired to perform their activities of daily living to a great extent.Citation32
Smartphone devices have inbuilt accessibility features which helps their visual disabilities.Citation30,Citation31 These accessibility features are available both in Android and iOS smartphones.Citation31 Inbuilt accessibility features are talkback which reads what is in the screen by touch screen technology; for example, reads the name of the icon on the screen. Magnification feature zooms the display screen. Invert color is useful for those who cannot read with a white background with black letters. Font size can be increased and it can be made bold. The most commonly used accessibility features are talkback and voiceover.Citation32 The accessibility features are also available in applications.Citation31
The low vision apps were searched on play store and App store. The search terms included, “low vision apps”, “visual impairment”, “blindness app”, “navigation app”, “color identification”, “currency identification app”, “braille app”, “magnifier app”, “reading app”, “object identification app”, “day to day activities” and etc. Information of these apps were also obtained through literature search in the following databases: “PubMed”, “Scopus”, “Cochrane”, “ResearchGate” using the keywords, “low vision” OR “visual impairment” OR “blindness” AND “smartphone apps” OR “vision enhancement app” OR “day to day activities app” OR “validated apps” OR “development of apps”. The apps were downloaded in the smartphone and the features were reviewed.
A variety of smartphone apps are available in play store and app store for the visually impaired ( and ). Using these apps, the visually impaired can identify currency and color, recognize and read text, locate objects, and navigate safely. Some apps are freely available whilst some require a subscription fee. These apps are either single featured, i.e, can be useful only for performing single task or multifeatured, i.e, can be used for performing multiple tasks. Few apps like “Be My Eyes” and “Kibbo” enable the individual to be independent through interaction with sighted peers.Citation32 These Smartphone apps are similar in functionality to a conventional low vision device and enable the visually impaired people to perform their daily living tasks without any limitations.
Table 4 Single Featured Smartphone Apps for Low Vision Rehabilitation
Table 5 Multifeatured Smartphone Apps for Low Vision Rehabilitation
“Multifeature” apps are useful for multiple tasks while performing activities of daily living (e.g. Finding objects, text recognition, face recognition) (). They combine an artificial intelligence-based technology that enables face, color, object and text recognition (OCR – Optical Character Recognition) and generate a voice output of what is seen, thus enabling the visually impaired people to look around the world and explore the surroundings. Some of these apps also help in currency identification and offer reversed contrast polarity along with magnifying the text.
Figure 2 Multifeatured app showing (a) different operating modes (b) colour identification, (c) object identification and (d) currency identification features.
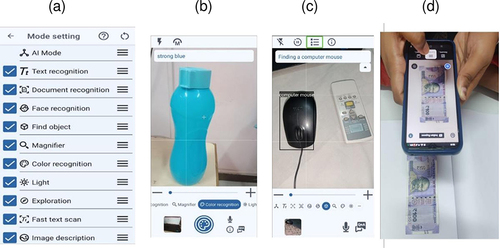
This review describes four multi-featured apps that are more commonly used by the visually impaired patients and have been rated to be most useful.Citation33,Citation34
Seeing AI is an app that harnesses the power of Artificial Intelligence that helps the visually impaired people to see the world through voice narration. It is freely available for both Android and iOS smartphones. It assists them in a variety of daily living activities including face recognition, currency identification, reading printed documents and scanning barcodes.
Be My Eyes app assists the visually impaired people with daily living activities by connecting them to sighted volunteers who guide them in performing the daily tasks. It is freely available for both Android and iOS smartphones. The sighted volunteer receives notification upon request by the visually impaired individual for assistance through the app. The app then connects the visually impaired person with a volunteer, who receives live video feed through the user’s smartphone camera along with audio connection to complete the task together.
Lookout Google Application is a multipurpose app for object identification in the surrounding, text and document reading and currency identification. The app scans the features of the specific object and provides a voice output using the in-built audio technology.
Sullivan + Application is an artificial intelligence-based multipurpose app for text and face recognition, colour identification, magnifier, and exploring the surrounding. The app produces a voice output of what is seen, through its integrated audio assistant.
Wearable Device Technology in Optometry
A major portion of optometry deals with the detection, correction, and treatment of refractive error, with about one-fourth of the research on refractive error conducted to understand the etiology and management of myopia.Citation35 An increasing trend of myopia prevalence is noted across the globe.Citation36–38 With the technology evolving rapidly, several innovations were developed to monitor the risk factors associated with myopia as well as for the management of myopia. This section of the review will focus on wearable devices that have applications in the correction of myopia, monitoring the environmental risk factors associated with myopia, and myopia management.
The literature search in PubMed yielded 63 results using the following search index: ((“myopia*”[Title/Abstract]) OR (“myope*”[Title/Abstract]) OR (“nearsight*”[Title/Abstract]) OR (“near-sight*“[Title/Abstract]) OR (“shortsight*”[Title/Abstract]) OR (“short-sight*”[Title/Abstract]) OR (“refractive error*”[Title/Abstract])) AND ((“wearable*”[Title/Abstract]) OR (“sensors”[Title/Abstract]) OR (“trackers”[Title/Abstract])). The last date of the search was 31st August 2023. We included the studies or review articles that investigated or discussed the non-spectacle wearable devices, that are either used or have the potential to correct, monitor, or manage myopia. Based on the title and abstract, 21 articles seemed relevant to the purpose of our review that reported 3 wearable devices that have the potential to correct refractive error while 10 devices are used to monitor the risk factors and manage myopia. From these articles, the details on the wearable devices pertaining to their features (name of device, developer, size, weight, location, battery life, components of the device, parameters obtained by device, range of measurement, sampling time) were extracted, in addition to information about their validation against the standard device and subsequent application, if available. Apart from the articles/devices obtained through the search, we included the information based on our collective a priori knowledge.
Wearable Devices for Correction of Refractive Error
To combat the barriers of uncorrected refractive error such as the cost of spectacles and a dearth of experienced eyecare practitioners in limited resource settings, self-adjustable spectacles were developed.Citation39,Citation40 Self-adjustable spectacles have variable foci that can be adjusted to measure as well as correct the refractive error. Additionally, to monitor and improve spectacle compliance, especially in amblyopia management, temperature-based spectacle wear time sensors were also developed. The current section describes the current application of these devices in refractive error which have a potential in myopia management as well.
Self-adjustable spectacles are based on two designs: fluid-filled variable focus technology (AdSpecs, Adaptive Eyecare Ltd, UK) and Alvarez’s design (Adlens, Adlens Ltd, UK, and FocusSpecs, Focus On Vision., Netherlands). AdSpecs consists of two thin surfaces that are separated by a fluid and secured in a frame. The fluid is contained in the pumps that are fixed to the spectacle frame and the fluid volume between the surfaces can be controlled by an adjustment wheel connected to the pump. The curvature of the surfaces and the fluid volume determines the best lens power that can correct the spherical refractive error within ±6 D. Adlens and FocusSpecs feature two lenses made of polycarbonate materials that can slide up to 3 mm and 6 mm respectively, using knurled adjustment screw drives to produce optimal refractive correction. Adlens can correct spherical refractive errors ranging between −6.00 and −3.00 D while correction within a range of +0.50 D to +4.50 D and −1.00 to −5.00 D is possible using FocusSpecs. Visual acuity is reported to be better than 20/25 in the majority of the individuals with the self-adjustable spectacles.Citation41–47 Self-refraction performed with these spectacles was found to be within ±1 D of cycloplegic refraction for the majority of the individuals, with an inclination of outcome towards more negative spherical refractive error with self-adjustable glasses.Citation41–47
Spectacle wear time sensors are the temperature-based microsensors, commercially available for recording wear time in various fields such as orthodontics, ophthalmology, orthopedics, and thermo-sensitive logistics. Among these sensors, the application of TheraMon (MC Technology GmbH, Austria) and Smart Button data logger (ACR Systems Inc., Canada) was noted in the field of eye care to track spectacle compliance. Both these sensors are typically affixed to the temple of the eyewear, ensuring direct contact with the skin, and recording the temperature at a desired interval. The spectacle wear and removal time are determined based on the sudden change in temperature in either direction and are found to have good agreement with the self-reported spectacle wear.Citation48,Citation49 SpecsOn monitor is a spectacle-mounted sensor, specifically developed for determining spectacle compliance. It is equipped with two temperature sensors, enclosed in a skin-safe silicone casing. One sensor is for measurement of the skin temperature and is directed towards the wearer’s temple while the other sensor is for recording device temperature as a surrogate for the ambient temperature. Data obtained by the SpecsOn monitor has to be extracted through a connector and a USB port. It is inferred that spectacle is worn if the difference between the temperature recorded by two sensors is greater than a set value (ie >(−0.21 × Ambient Temperature) + 7). A minimal difference was noted in the wear time recorded by the SpecsOn monitor and self-reported wear time in 80% of the participants.Citation50 The monitor was capable of detecting when spectacles were artificially subjected to extreme temperatures when placed in shirt or trouser pockets and held in hand. However, it was reported to produce a false-positive error when spectacles were positioned on the head or the forehead.Citation51
Wearable Devices for Monitoring Risk Factors and Managing Myopia
The International Myopia Institute issued guidelines for practitioners to recommend patients at the risk of developing myopia to spend more time outdoors as well as maintain proper lighting in outdoor and indoor settings,Citation51 as time outdoors is a safe intervention and has other health benefits,Citation52 as indicated in the overviews of literature.Citation53,Citation54 Multiple factors are believed to be responsible for a protective role of outdoor time in myopia development: higher illuminance levels, spectral composition of light, uniform dioptric field, presence of high spatial frequencies, increased Vitamin D levels, greater levels of physical activity, dopamine release, and substitution for near work.Citation55,Citation56 Given that illuminance and spectral power are indicated to vary with different outdoor locations,Citation57,Citation58 the subjective quantification of time outdoors as conducted in previous studies through questionnaires/interviews in terms of hours might not be reliableCitation59–61 Apart from time outdoors, various components of near work including greater duration of near work, lesser number of intermittent breaks during near work, and closer viewing distance were indicated to have a detrimental effect on myopia development.Citation60,Citation62–64 In addition to the recall bias in a subjective method, the quantification of near work was noted to vary with the type of subjective method used.Citation65 Hence, several objective trackers were developed to monitor the risk factors of myopia (). These trackers quantify the time spent outdoors in terms of illuminance measured in lux, time spent outdoors (number of hours spent at >1000 lux, typically), and epoch (frequency of exposure to >1000 lux, typically). The magnitude of near work is measured in terms of viewing distance in centimeters, continuous near work in hours, and dioptric hours. In addition to real-time tracking that improves the objectivity of the measurements, a few of these trackers are additionally equipped to provide feedback to the wearers to modify their myopiogenic environmental behavior which has potential in myopia prevention and control.
Table 6 Existing Wearable Devices used to track risk factors associated with Myopia
Clouclip, developed by the “Glasson Technology Co. Ltd”., Hangzhou, China, is a tracker clipped onto the right temple of the spectacles. It contains an infrared distance sensor and a light intensity sensor for the purpose of measurement of near work in terms of viewing distance and light exposure in regard to illuminance levels in real-time, respectively. It was reported to indicate the viewing distance for a range of 15 to 60 cm every 5 seconds and the illuminance levels for a range of 1 to 655,336 lux at an interval of 2 minutes. It also consists of a tri-axial accelerometer that indicates if the tracker is worn or not. The tracker is considered to be not in use if no change occurred for 40 seconds. The data collected by the tracker is uploaded to a smartphone application via Bluetooth. In the validation study, Clouclip revealed a mean difference of 0.4 cm (95% limits of agreement: 2.5 cm, for printed target) to 0.5 cm (± 1.5 cm, for electronic text) in the measurement of viewing distance compared with the actual distance and a mean difference of 1670.4 lux (± 7959.38 lux) in the measurement of illuminance levels in comparison to lux meter.Citation66 A few studies used the tracker to quantify the change in viewing behaviors and light exposure patterns with change in refractive error status,Citation67–70 region of residence (urban vs rural),Citation71 and COVID-19.Citation72 In addition to quantification of near work, Clouclip was also tested for its utility in the modification of the visual behavior in the children.Citation73 The device was set to vibrate if the viewing distance was <30cm or continuous near-work > 45 minutes, and research findings indicate that there was an improvement in the mean viewing distance and a reduction in near work duration and frequency.
FitSight Fitness tracker consists of a custom-made FitSight watch app installed into a commercialized smartwatch (Sony Smartwatch 3; Sony Corp., Japan). It contains a light sensor to measure the illuminance at an interval of 1 minute. It is also equipped with an accelerometer to record the physical activity levels every 30 seconds. The information about time spent outdoors (> 1000 lux) recorded by the FitSight watch app is displayed on the main home page of the application and synchronized to the application in the parent’s smartphone via Bluetooth. It encourages the wearer to spend more than 3 hours a day outdoors by sending reminders if a set target is not reached at two specific times and a congratulatory message if the target is achieved. FitSight fitness tracker was rated as good by 93% of the parents and compliance (worn on all days) was found to be good for 61% of the children.Citation74
Actiwatch 2 and Actiwatch Spectrum (Philips Respironics, USA) are commercially available wrist-worn trackers (on the non-dominant hand) that have been used in myopia research. Actiwatch 2 is equipped with a silicon photodiode to measure illuminance within a range of 5 to 100,000 Lux for a wavelength that ranges between 400 nm and 900 nm while Actiwatch Spectrum comprises color-sensitive photodiodes that can measure illuminance ranging from 0.1 to 200,000 lux for wavelength ranging from 400 to 700 nm. The time interval between each measurement can be adjusted to either 15, 30, 45, or 60 seconds. Actiwatch 2 and Actiwatch Spectrum contain solid-state Piezoelectric accelerometer that records physical activity if it ranges between 0.35 Hz and 7.5 Hz at a sampling rate of 32 Hz. The illuminance recorded by Actiwatch Spectrum had a very high correlation of 0.99 (P < 0.001) with the lux meter for recording illuminance in multiple indoor and outdoor locations.Citation75 Both these trackers were used for the comparison of sunlight exposure and physical activity between myopic and non-myopic individuals.Citation75–77 The objectivity of the measurements obtained with these devices was also used to understand the association between light exposure, circadian rhythms, and diurnal variation of ocular biometrics in the field of myopia.Citation78–80
MyLyt is a tracker developed by the L V Prasad Eye Institute, India, that can be clipped onto clothing in the upper thoracic region. It consists of an ambient light sensor to record the illuminance from 0 lux and 88,000 lux for the visible light spectrum at an adjustable sampling rate. The data extraction from MyLyt is manual and needs a memory of 6 Giga Byte. The mean difference in light levels between MyLyt and lux meter was found to be within the acceptable error margin for controlled, outdoor, and indoor locations.Citation81 Additionally, there was good concordance noted between the sensor’s ability to distinguish outdoors from indoors (> 1000 lux) and self-reported location in adults (98% sensitivity and 99% specificity).
HOBO (Onset Computer Corp, USA) is a light tracker that can be clipped on either the clothes or armbands. The tracker can measure the light in the range of 0 to 320,000 lux for a peak sensitivity of 900 nm at an adjustable sampling rate. In addition, it also records the temperature within a range of −20 to 70°C. Time spent outdoors (> 1000 lux) logged by HOBO was found to be significantly higher than values noted by the children in their dairy by 2 hoursCitation82 and lower than values reported by adults by 2 to 3 hours.Citation83
Mumu is a smartwatch that consists of two light sensors to measure illuminance as well as ultra-violet levels every 20 seconds and to detect wearing status. It is also equipped with an accelerometer to count the activity level in terms of step count and a GPS “(Global Positioning System)” sensor to record geographic location details. Recorded data are uploaded into a software platform through a mobile terminal. Support vector machine, a machine learning algorithm, was applied to the data obtained from Mumu smart watches for developing models to discriminate indoor and outdoor environments, which were found to have accuracy ranging from 83% to 99%.Citation84
RangeLife is a spectacle-mounted tracker that consists of an infra-red time-of-flight distance sensor (Adafruit, USA). The sensor detects the viewing distance within a range of 0.03 to 1.2 m at a sampling rate of 1 Hertz. The data is transferred to a secure digital card contained in a plastic enclosure along with a real-time clock which is connected to the sensor via a wire. The distance measured by the sensor varied within ±0.002 m, ±0.003 m, and ±0.0012 m compared to the actual distance of 0.10 m, 0.40 m, and 1.0 m respectively. Objective measurements of the near work duration, near viewing distances and time spent outdoors among adults, recorded by RangeLife, were noted to be lesser than subjective reports.Citation85
Vivior Monitor (Zurich, Switzerland) consists of distance, light, inertial, and magnetometer sensors and is attached to the rim of the spectacles to measure viewing distance, illuminance, ultraviolet light intensity, head movement, and position. While the device was previously used to know the visual needs and behavior of presbyopic adults to develop custom intraocular lenses,Citation86 its application in understanding the visual behavior in children with myopia was also investigated.Citation87
Myopia tracker is spectacle mounted tracker that encompasses multiple sensors: an ambient light sensor to measure the illuminance ranging from 0.045 to 188,000 lux, a global positioning system to differentiate indoor and outdoor locations, a time of flight sensor to record viewing distance up to 1 meter, micro spectrograph to record spectral power distribution for wavelength between 450 nm and 950 nm, and temperature sensor which can withstand −40 to +85° C. The illuminance recorded by the Myopia tracker was found to have no statistical difference with the lux meter and measured viewing distance showed a good correlation with actual distance (R2 = 0.99). The spectral power was noted to be greater for the orange and blue channels in indoor (100–160 counts/μW/cm2) and outdoor locations (10,000–19,000 counts/μW/cm2) respectively with the tracker.Citation88
Wearable Devices for Low Vision Rehabilitation
Technological advancements in artificial intelligence have revolutionized the field of low vision rehabilitation. A number of head-mounted/spectacle mounted smart-devices have been developed in the recent past, thus enabling visually impaired and blind people to achieve functional independence. These devices employ a combination of AI-based technology including Optical Character Recognition, object and face recognition, image processing and remapping technology for low vision enhancement along with audio output for the functionally blind.Citation89
Virtual and Augmented Reality based devices
Virtual reality (VR) technology, as the name suggests, creates a virtual environment such as underwater, greenery, mountains, outer space, and beyond without the boundaries of a screen using a wrap-around headgear. However, it has seeped its way into ophthalmology by creating a virtual environment for patients to enable them to improve object detection and their activities. VR gadgets like IrisVision™ are used to assist visually impaired patients. A smartphone housed inside the VR headset captures the patient’s ambient environment and projects it as an enlarged image in peripheral field of vision.
The device provides depth perception by providing slightly different images to each eye, and with the lens between the eyes, they create a three-dimensional image. VR headsets are immersive devices, in that, they provide an increased field of view (width of the picture). Additionally, the headsets make use of “head tracking”- shifting the picture in front when looking up, down, and side to side.
Augmented reality on the other hand is when computer-generated information is improved or enhanced for displaying in real-time and therefore, is widely used in healthcare.Citation90 However, a major limitation for patients is that due to an enclosed and immersive nature of the headsets, peripheral vision is restricted and therefore, it can be unsafe especially while driving.
Orcam is a spectacle mounted assistive device that consists of a small camera based on Optical Character Recognition (OCR) technology that converts scanned images to text or voice output which is communicated to the user through an ear bud. Citation91
The usefulness of OrCam has been studied in visually impaired patients (mean age: 47.6 ± 16.3 years) with advanced inherited retinal dystrophies. National Eye Institute Visual Functioning Questionnaire (NEI-VFQ), the Participation and Activity Inventory (PAI), and the OrCam Function Questionnaire (OFQ), were administered to the patients at the initial visit and after 5.2 weeks. After using Orcam, there was a significant improvement in (i) near vision tasks scores in NEI-VFQ (p < 0.001), (ii) overall vision function score (re-engineered NEI-VFQ), (iii) reading ability in PAI, and (iv) overall quality of life score in OFQ.Citation91
e-Sight is a head borne device that consists of a high-speed camera to capture images of the ambience and converts them into high-definition output using AI algorithms. This is a hand free, autofocus device that provides seamless vision at all range of distances along with maximizing the central as well as peripheral vision.Citation90
SightPlus is an augmented reality-based assistive device, which utilizes the healthy, functional parts of the retina that are not severely damaged, for image focusing. It consists of a AR headgear to mount the smart phone installed with the software and a remote control. The usefulness of this device has been validated in 8–16-year-old childrenCitation92 as well as 18–93-year-old adults.Citation93
Eighteen participants aged 8 to 16 years used SightPlus for a month. Visual functions and functional vision were assessed with and without SightPlus. With SightPlus, distance visual acuity improved on an average by 0.63 logMAR units and near vision improved by about 0.41 logMAR units. The reading acuity also improved by about 0.45 logMAR units, and the ability to read critical print sizes improved by about 0.28 logMAR units.Citation92
Visual functions and reading performance were measured among 60 participants aged 18 to 93 years, in both normal mode and image enhancement mode. The mean improvement in distance visual acuity and contrast sensitivity was 0.63 and 0.22 logMAR units, respectively. Contrast sensitivity increased with further magnification and image enhancement by 0.40 logMAR. There was also an improvement in reading for near visual acuity and critical print size. Nearly half (47%) of participants expressed interest in using the device, in particular for near visual tasks and entertainment.Citation93
NuEyes is an augmented reality-based system that utilizes a smart glass and a software that provides magnification ranging from 1x – 12x, variable contrast and color, text-to-voice output, and media streaming. An advanced version of this device, the NuEyes Pro, comes with added internet browsing features and the complete android tablet functionality.Citation90 NuEyes e3+ is the latest version, powered with visual AI software, that can help visually impaired to watch TV, read a book, cook in the kitchen, or watch live sports at the stadium. The e3+’s automatic pupil adjustment makes it the most advanced low vision wearable to date.Citation90
Aira is a smart glass that provides remote assistive technology to the visually impaired. The device uses the smartphone camera and an app, to seek assistance from a trained agent for visual interpretation of the surroundings. By tapping on the side of the smart glass, the user gets connected to an Aira certified visual interpreter, who has access to the user’s location and profile on Google Maps through the smart glasses and interprets the surrounding, thus assisting the visually impaired person.Citation90
Smart Vision Glass is an innovation that is similar to Orcam, consisting of a camera, remote sensing technology, Bluetooth microphone and a miniscule speaker. Smart Vision Glass is a wearable device for the blind and visually impaired, that transforms patient’s dark world into one that is more dynamic, engaging, and self-sufficient by virtue of artificial intelligence and machine learning. It helps the patients in object identification, face recognition, reading text in multiple languages and safe navigation with alerts to obstacles while walking. The in-built miniature camera takes pictures of the ambient environment, interprets and analyses the visual data, and then provides voice output.Citation94
Argus 2 is an FDA approved retinal prosthesis system also known as the Bionic Eye, that helps to restore some residual vision visual impairment due to conditions like retinitis pigmentosa. This consists of a retinal implant with receiver that captures inputs from the device’s in-built camera, worn by the user. This has been clinically tested among 28 patients with profound visual impairment due to retinitis pigmentosa. These patients were found to perform better on daily living activities when the retinal prosthesis system was switched on compared to when the system was kept off.Citation95
Future Perspectives
The world-wide productivity gain from correcting avoidable blindness, visual impairment, and presbyopia will be about US$ 19 trillion by 2050.Citation1 Keeping this in view, embracing smartphone applications and smart devices for ocular diagnostics represents a significant step forward in advancing the practice of optometry, enabling eye care practitioners to improve patient care and eye care accessibility.
In summary, for distance visual acuity testing, the Eye Chart app, with only about 2-letter difference, can be used by the eye care practitioners for screening as well as in clinical setting. With its highly repeatable visual acuity measures across examiners, AAPOS app is suitable for pediatric age group especially for amblyopia and low vision screening, along with the PEEK acuity app with good agreement and minimal test–retest variability. With four different visual acuity cut-off points and a high validity as a screening tool, the Vis Scr app is recommended for screening visual impairment and blindness among children. Given the low mean difference in visual acuity, good test–retest reliability and agreement and high sensitivity and specificity, eye care practitioners can use these vision screening apps.
Among the apps for near visual acuity testing, the NYU Langone app and the Eye chart app, with a minimal difference of about 2-letter against the gold standard tests, can be used reliably by the eye care practitioners. The SmartVisionCheck app, with its functional near vision component, may add a new dimension to near vision testing while prescribing presbyopic correction as well in low vision management of conditions that cause near visual impairment. Ody Sight app can be used for near vision testing in normal individuals, but to be used cautiously for patients with reduced near vision due to overestimation or underestimation. Smart Optometry app, with its good sensitivity among 5–12-year olds, may be suitable for school vision screening.
With more than 50% of the world’s population predicted to be myopic by the end of fifth decade of the 21st century,Citation96 and association of vision-threatening complications with high myopia,Citation97–100 the scope for the wearable smart devices in myopia prevention or delaying myopia onset and controlling its progression is enormous.
At present, wearable devices meant for myopia correction possess several limitations. Self-adjustable spectacles have a limited range of refractive correction and cannot correct astigmatism. While there was a lesser proportion of individuals in whom inaccuracy of self-adjustment of refractive error was reported with these spectacles, myopic under-correction was linked to a greater rate of progression than full correction and overcorrection of myopia will stimulate accommodation which might increase the risk of asthenopia. Furthermore, the progression of myopia might be unnoticed if the degree of myopia is within the refractive range of the spectacles, as it can be self-adjusted to attain optimal vision. Therefore, one needs to be aware of the limitations of self-adjustable glasses and further research should be carried out to understand its impacts on eye health and refractive error. The spectacle wear time sensors are currently used in the management of amblyopia. However, further research is needed to understand their scope in the field of myopia in terms of the determination of the time of wear of myopia control spectacles.
Wearable light and distance trackers constitute the majority of existing wearable devices in the field of myopia. Most of the currently available trackers can measure a limited number of risk factors associated with myopia. The wrist-worn and cloth-clipped trackers do not provide a measurement of the illuminance reaching the eye and cannot quantify near work-related behaviors. While spectacle-mounted trackers subdue this limitation, the measurement provided by these trackers is not in the line of sight as they are usually attached to the rim of spectacles. Additionally, the individuals with emmetropia are required to wear spectacles with plano lenses for the spectacle-mounted trackers which might limit their uptake in this population and will be demoralizing considering that the intervention of time outdoors was beneficial before the onset of myopia. The futuristic approach should be to develop a tracker that can provide comprehensive quantification of environmental risk factors associated with myopia, keeping in view the multifactorial etiology of myopia. The cost-effectiveness and user-friendliness must be considered during the development of trackers in the future to improve their acceptability and compliance.
In addition to existing trackers, virtual reality headsets have gained popularity and have great potential considering the increasing evidence of their influence on choroidal thickening and axial length modulation in short-term studies.Citation101,Citation102 However, the long-term influence of virtual reality-based techniques in myopia development and progression warrants further investigations. There is a growing evidence which indicate that specific wavelengths of light may play a probable role in controlling myopia.Citation103 Apart from the low level repeated red-light therapy through desktop-based device,Citation104 there are a few wearable devices for myopia control that are developed to emit monochromatic light: violet light from eyeglass frames,Citation102 and blue light simulation of optic nerve head using virtual reality headset,Citation105 which are currently being investigated in the myopia clinical trials. There is a wide scope for the wearable light therapy devices as myopia control intervention, in addition to optical and pharmaceutical interventions. In a comprehensive assessment, existing smart wearable devices have demonstrated a dual impact on both myopia correction and management. Moving forward, the trajectory involves enhancing current devices with the rapidly evolving smart technology and innovating newer ones guided by evidence, thereby advancing research aimed at understanding myopia’s etiology and effectively managing its progression within clinical settings.
From a futuristic view point for vision rehabilitation, IoT (Internet of Things) based devices for smart and safe home may be the next-generation revolution. Smart watches synchronized with ultrasonic sensors fixed in home space may help the visually impaired people to detect obstacles and help in safe navigation.Citation106 Operating electronic appliances may also get simplified through a voice-assisted smart home system that can operate and control all household electronic devices with the use of a single, universal remote control operated via the smartphone.Citation107 Recent advances have expanded assistive technology into providing smart devices for homes such as a voice alarm for stoves, home alarm system, and lighting system configured with Alexa and/or Google Assistant or the tablet/iPad. The TV remote is also voice-enabled through voice-activation technology, which allows one to control even computers and mobile phones through audio commands. Assistance with recipes, playing music, playing games, and checking for weather can be requested by coupling computers and mobile phones with technologies accessible on Amazon, Google, or Apple.Citation107,Citation108 The addition of AR & VR-based smart devices into optometry can offer the practitioners powerful tools for assisting patients with their routine activities. These devices will help those that are visually impaired by creating more independence and autonomy. The choice of such devices should be made based on the visual requirements of patients.
Conclusion
Smart devices are becoming more popular and are readily available and easy to install. With a predicted upsurge in visual impairment globally by the year 2050,Citation1 smartphone based digital tools can revolutionise eye care delivery system. Due to rapid digitalization and technological advancements, these tools will break the barriers in accessing eye care in remote areas and change the landscape of eye care delivery system. These devices will aid the eye care providers to monitor the disease progression from the convenience of their homes and create digital optometry clinics and screening sites in future.
Disclosure
The authors report no conflicts of interest in this manuscript.
Acknowledgment
The authors would like to thank Mr Raisul Azam, PhD Scholar, Department of Optometry, SRM Medical College Hospital and Research Centre, for his assistance in preparing the manuscript.
References
- Bastawrous A, Suni AV. Thirty year projected magnitude (to 2050) of near and distance vision impairment and the economic impact if existing solutions are implemented globally. Ophthalmic Epidemiol. 2020;27(2):115–120. doi:10.1080/09286586.2019.1700532
- Zhang JH, Ramke J, Mwangi N, et al. Global eye health and the sustainable development goals: protocol for a scoping review. BMJ Open. 2020;10(3):e035789. doi:10.1136/bmjopen-2019-035789
- Burn H, Hamm L, Black J, et al. Eye care delivery models to improve access to eye care for Indigenous peoples in high-income countries: a scoping review. BMJ Glob Health. 2021;6(3):e004484. doi:10.1136/bmjgh-2020-004484
- Das T, Keeffe J, Sivaprasad S, Rao GN. Capacity building for universal eye health coverage in South East Asia beyond 2020. Eye. 2020;34(7):1262–1270. doi:10.1038/s41433-020-0801-8
- Hogarty DT, Hogarty JP, Hewitt AW. Smartphone use in ophthalmology: what is their place in clinical practice? Surv Ophthalmol. 2020;65(2):250–262. doi:10.1016/j.survophthal.2019.09.001
- Claessens JLJ, Geuvers JR, Imhof SM, Wisse RPL. Digital tools for the self-assessment of visual acuity: a systematic review [published correction appears in Ophthalmol Ther. 2021 Dec;10(4):731–732]. Ophthalmol Ther. 2021;10(4):715–730. doi:10.1007/s40123-021-00360-3
- Suo L, Ke X, Zhang D, et al. Use of Mobile Apps for Visual Acuity Assessment: systematic Review and Meta-analysis. JMIR mHealth uHealth. 2022;10(2):e26275. doi:10.2196/26275
- Kawamoto K, Stanojcic N, Li JO, Thomas PBM. Visual Acuity Apps for Rapid Integration in Teleconsultation Services in all Resource Settings: a Review. Asia Pac J Ophthalmol. 2021;10(4):350–354. doi:10.1097/APO.0000000000000384
- Ansell K, Maconachie G, Bjerre A. Does the eyechart app for iPhones give comparable measurements to traditional visual acuity charts? Br Ir Orthopt J. 2020;16(1):19–24. doi:10.22599/bioj.146
- Hazari H, Curtis R, Eden K, Hopman WM, Irrcher I, Bona MD. Validation of the visual acuity iPad app eye chart pro compared to the standard early treatment diabetic retinopathy study chart in a low-vision population. J Telemed Telecare. 2022;28(9):680–686. doi:10.1177/1357633X20960640
- Nik Azis NN, Chew FLM, Rosland SF, Ramlee A, Che-Hamzah J. Parents’ performance using the AAPOS vision screening app to test visual acuity in Malaysian preschoolers. J AAPOS. 2019;23(5):268.e1–268.e6. doi:10.1016/j.jaapos.2019.01.019
- Bastawrous A, Rono HK, Livingstone IA, et al. Development and validation of a smartphone-based visual acuity test (peek acuity) for clinical practice and community-based fieldwork [published correction appears in JAMA Ophthalmol. 2015 Sep; 133(9):1096]. JAMA Ophthalmol. 2015;133(8):930–937. doi:10.1001/jamaophthalmol.2015.1468
- Zhao L, Stinnett SS, Prakalapakorn SG. Visual acuity assessment and vision screening using a novel smartphone application. J Pediatr. 2019;213:203–210.e1. doi:10.1016/j.jpeds.2019.06.021
- Siti nor Aishah AR, Mutalib A, Asma H, et al. Development and validation of a new vision screening test algorithm for public use mobile application- A pilot study. Med J Malaysia. 2020;75(6):685–690.
- Abdul Rahman SNA, Naing NN, Othman AM, et al. Validity and reliability of vis-screen application: a smartphone-based distance vision testing for visual impairment and blindness vision screening. Medicina. 2023;59(5):912. doi:10.3390/medicina59050912
- Iskander M, Hu G, Sood S, et al. Validation of the New York University Langone eye test application, a smartphone-based visual acuity test. Ophthalmol Sci. 2022;2(3):100182. doi:10.1016/j.xops.2022.100182
- Tofigh S, Shortridge E, Elkeeb A, Godley BF. Effectiveness of a smartphone application for testing near visual acuity. Eye. 2015;29(11):1464–1468. doi:10.1038/eye.2015.138
- Tiraset N, Poonyathalang A, Padungkiatsagul T, Deeyai M, Vichitkunakorn P, Vanikieti K. Comparison of visual acuity measurement using three methods: standard ETDRS chart, near chart and a smartphone-based eye chart application. Clin Ophthalmol. 2021;15:859–869. doi:10.2147/OPTH.S304272
- Hanyuda A, Kubota M, Kubota S, et al. Validation of a novel iPhone application for evaluating near functional visual acuity. Sci Rep. 2022;12(1):22342. doi:10.1038/s41598-022-27011-2
- Kaido M. Functional Visual Acuity. Invest Ophthalmol Vis Sci. 2018;59(14):DES29–DES35. doi:10.1167/iovs.17-23721
- Kaido M, Dogru M, Ishida R, Tsubota K. Concept of functional visual acuity and its applications. Cornea. 2007;26(9 Suppl 1):S29–S35. doi:10.1097/ICO.0b013e31812f6913
- Katada Y, Negishi K, Watanabe K, et al. Functional Visual Acuity of Early Presbyopia. PLoS One. 2016;11(3):e0151094. doi:10.1371/journal.pone.0151094
- Stoll N, Di Foggia E, Speeg-Schatz C, et al. Development and validation of a new method for visual acuity assessment on tablet in pediatric population: eMOVA test. BMC Ophthalmol. 2022;22(1):180. doi:10.1186/s12886-022-02360-8
- Brucker J, Bhatia V, Sahel JA, Girmens JF, Mohand-Saïd S. Odysight: a mobile medical application designed for remote monitoring-A prospective study comparison with standard clinical eye tests. Ophthalmol Ther. 2019;8(3):461–476. doi:10.1007/s40123-019-0203-9
- Raffa LH, Balbaid NT, Ageel MM. “Smart Optometry” phone-based application as a visual acuity testing tool among pediatric population. Saudi Med J. 2022;43(8):946–953. doi:10.15537/smj.2022.43.8.20220374
- Askarian B, Tabei F, Tipton GA, Chong JW Novel keratoconus detection method using smartphone. 2019 IEEE Healthcare Innovations and Point of Care Technologies, HI-POCT 2019; 2019:60–62. doi:10.1109/HI-POCT45284.2019.8962648.
- Wintergerst MWM, Brinkmann CK, Holz FG, Finger RP. Undilated versus dilated monoscopic smartphone-based fundus photography for optic nerve head evaluation. Sci Rep. 2018;8(1):10228. doi:10.1038/s41598-018-28585-6
- Ophthalmic Sciences unveils world’s first AI contactless device for measuring eye fluid pressure. Available from: https://www.prnewswire.com/il/news-releases/ophthalmic-sciences-unveils-worlds-first-ai-contactless-device-for-measuring-eye-fluid-pressure-301438718.html. Accessed March 30, 2024.
- Liu Y, Holekamp NM, Prospective HJS. Longitudinal study: daily self-imaging with home OCT for neovascular age-related macular degeneration. Ophthalmol Retina. 2022;6(7):575–585. doi:10.1016/j.oret.2022.02.011
- Hakobyan L, Lumsden J, O’Sullivan D, Bartlett H. Mobile assistive technologies for the visually impaired. Surv Ophthalmol. 2013;58(6):513–528. doi:10.1016/j.survophthal.2012.10.004
- Senjam SS, Manna S, Bascaran C. Smartphones-based assistive technology: accessibility features and apps for people with visual impairment, and its usage, challenges, and usability testing [published correction appears in Clin Optom (Auckl). 2021 Dec 07;13:333–334]. Clin Optom. 2021;13:311–322. doi:10.2147/OPTO.S336361
- Senjam SS. The current advances in human-smartphone user interface design: an opportunity for people with vision loss. Indian J Ophthalmol. 2021;69(9):2544–2545. doi:10.4103/ijo.IJO_835_21
- Dockery DM, Krzystolik MG. The use of mobile applications as low-vision aids: a pilot study. R I Med J. 2020;103(8):69–72.
- Tharaniy VE, Dharani R Effectiveness of Smartphone Applications as a tool to Improve Functional Vision and Quality of Life of Visually Impaired People. [ Unpublished Manuscript]; 2023.
- Refractive Error RM. Still the heart of optometry. Ophthalmic Physiol Opt. 2021;41(2):211–212. doi:10.1111/opo.12790
- Chen M, Wu A, Zhang L, et al. The increasing prevalence of myopia and high myopia among high school students in Fenghua city, eastern China: a 15-year population-based survey. BMC Ophthalmol. 2018;18(1):159. doi:10.1186/s12886-018-0829-8
- Priscilla JJ, Verkicharla PK. Time trends on the prevalence of myopia in India - A prediction model for 2050. Ophthalmic Physiol Opt. 2021;41(3):466–474. doi:10.1111/opo.12806
- Kobia-Acquah E, Flitcroft DI, Akowuah PK, Lingham G, Loughman J. Regional variations and temporal trends of childhood myopia prevalence in Africa: a systematic review and meta-analysis. Ophthalmic Physiol Opt. 2022;42(6):1232–1252. doi:10.1111/opo.13035
- Killeen OJ, Cho J, Newman-Casey PA, Kana L, Woodward MA. Barriers and facilitators to obtaining eyeglasses for vulnerable patients in a Michigan free clinic. Optom Vis Sci. 2021;98(3):243–249. doi:10.1097/OPX.0000000000001661
- Marmamula S, Keeffe JE, Raman U, Rao GN. Population-based cross-sectional study of barriers to utilisation of refraction services in South India: rapid Assessment of Refractive Errors (RARE) Study. BMJ Open. 2011;1(1):e000172. doi:10.1136/bmjopen-2011-000172
- Douali MG, Silver JD. Self-optimised vision correction with adaptive spectacle lenses in developing countries. Ophthalmic Physiol Opt. 2004;24(3):234–241. doi:10.1111/j.1475-1313.2004.00198.x
- Smith K, Weissberg E, Travison TG. Alternative methods of refraction: a comparison of three techniques. Optom Vis Sci. 2010;87(3):E176–E182. doi:10.1097/OPX.0b013e3181cf86d6
- Camp AS, Shane TS, Kang J, Thomas B, Pole C, Lee RK. Evaluating self-refraction and ready-made spectacles for treatment of uncorrected refractive error. Ophthalmic Epidemiol. 2018;25(5–6):392–398. doi:10.1080/09286586.2018.1500615
- Zhao L, Wen Q, Nasrazadani D, et al. Refractive accuracy and visual outcome by self-refraction using adjustable-focus spectacles in young children: a randomized clinical trial. JAMA Ophthalmol. 2023;141(9):853–860. doi:10.1001/jamaophthalmol.2023.3508
- Ocansey S, Amuda R, Abraham CH, Abu EK. Refractive error correction among urban and rural school children using two self-adjustable spectacles. BMJ Open Ophthalmol. 2023;8(1):e001202. doi:10.1136/bmjophth-2022-001202
- Ilechie AA, Abokyi S, Owusu-Ansah A, Boadi-Kusi SB, Denkyira AK, Abraham CH. Self-refraction accuracy with adjustable spectacles among children in Ghana. Optom Vis Sci. 2015;92(4):456–463. doi:10.1097/OPX.0000000000000561
- Ilechie AA, Abokyi S, Boadi-Kusi S, Enimah E, Ngozi E. Self-adjustable spectacle wearing compliance and associated factors among rural school children in Ghana. Optom Vis Sci. 2019;96(6):397–406. doi:10.1097/OPX.0000000000001382
- Januschowski K, Bechtold TE, Schott TC, et al. Measuring wearing times of glasses and ocular patches using a thermosensor device from orthodontics. Acta Ophthalmol. 2013;91(8):e635–e640. doi:10.1111/aos.12171
- Lentsch MJ, Marsack JD, Anderson HA. Objective measurement of spectacle wear with a temperature sensor data logger. Ophthalmic Physiol Opt. 2018;38(1):37–47. doi:10.1111/opo.12423
- South J, Roberts P, Gao T, Black J, Collins A. Development of a spectacle wear monitor system: specsOn monitor. Transl Vis Sci Technol. 2021;10(12):11. doi:10.1167/tvst.10.12.11
- Jonas JB, Ang M, Cho P, et al. IMI prevention of myopia and its progression. Invest Ophthalmol Vis Sci. 2021;62(5):6. doi:10.1167/iovs.62.5.6
- Gifford KL, Richdale K, Kang P, et al. IMI - Clinical Management Guidelines Report. Invest Ophthalmol Vis Sci. 2019;60(3):M184–M203. doi:10.1167/iovs.18-25977
- Dhakal R, Shah R, Huntjens B, Verkicharla PK, Lawrenson JG. Time spent outdoors as an intervention for myopia prevention and control in children: an overview of systematic reviews. Ophthalmic Physiol Opt. 2022;42(3):545–558. doi:10.1111/opo.12945
- Karthikeyan SK, Ashwini DL, Priyanka M, Nayak A, Biswas S. Physical activity, time spent outdoors, and near work in relation to myopia prevalence, incidence, and progression: an overview of systematic reviews and meta-analyses. Indian J Ophthalmol. 2022;70(3):728–739. doi:10.4103/ijo.IJO_1564_21
- Lingham G, Mackey DA, Lucas R, Yazar S. How does spending time outdoors protect against myopia? A review. Br J Ophthalmol. 2020;104(5):593–599. doi:10.1136/bjophthalmol-2019-314675
- Ramamurthy D, Lin Chua SY, Saw SM. A review of environmental risk factors for myopia during early life, childhood and adolescence. Clin Exp Optom. 2015;98(6):497–506. doi:10.1111/cxo.12346
- Bhandary SK, Dhakal R, Sanghavi V, Verkicharla PK. Ambient light level varies with different locations and environmental conditions: potential to impact myopia. PLoS One. 2021;16(7):e0254027. doi:10.1371/journal.pone.0254027
- Dhakal R, Huntjens B, Shah R, Lawrenson JG, Verkicharla PK. Influence of location, season and time of day on the spectral composition of ambient light: investigation for application in myopia. Ophthalmic Physiol Opt. 2023;43(2):220–230. doi:10.1111/opo.13085
- Dirani M, Tong L, Gazzard G, et al. Outdoor activity and myopia in Singapore teenage children. Br J Ophthalmol. 2009;93(8):997–1000. doi:10.1136/bjo.2008.150979
- French AN, Morgan IG, Mitchell P, Rose KA. Risk factors for incident myopia in Australian schoolchildren: the Sydney adolescent vascular and eye study. Ophthalmology. 2013;120(10):2100–2108. doi:10.1016/j.ophtha.2013.02.035
- Rose KA, Morgan IG, Ip J, et al. Outdoor activity reduces the prevalence of myopia in children. Ophthalmology. 2008;115(8):1279–1285. doi:10.1016/j.ophtha.2007.12.019
- Saw SM, Zhang MZ, Hong RZ, Fu ZF, Pang MH, Tan DT. Near-work activity, night-lights, and myopia in the Singapore-China study. Arch Ophthalmol. 2002;120(5):620–627. doi:10.1001/archopht.120.5.620
- Ip JM, Saw SM, Rose KA, et al. Role of near work in myopia: findings in a sample of Australian school children. Invest Ophthalmol Vis Sci. 2008;49(7):2903–2910. doi:10.1167/iovs.07-0804
- You QS, Wu LJ, Duan JL, et al. Factors associated with myopia in school children in China: the Beijing childhood eye study. PLoS One. 2012;7(12):e52668. doi:10.1371/journal.pone.0052668
- Saw SM, Nieto FJ, Katz J, Chew SJ. Estimating the magnitude of close-up work in school-age children: a comparison of questionnaire and diary instruments. Ophthalmic Epidemiol. 1999;6(4):291–301. doi:10.1076/opep.6.4.291.4184
- Bhandari KR, Ostrin LA. Validation of the Clouclip and utility in measuring viewing distance in adults. Ophthalmic Physiol Opt. 2020;40(6):801–814. doi:10.1111/opo.12735
- Bhandari KR, Ostrin LA. Objective measures of viewing behaviour in children during near tasks. Clin Exp Optom. 2022;105(7):746–753. doi:10.1080/08164622.2021.1971049
- Li L, Wen L, Lan W, Zhu H, Yang Z. A novel approach to quantify environmental risk factors of myopia: combination of wearable devices and big data science. Transl Vis Sci Technol. 2020;9(13):17. doi:10.1167/tvst.9.13.17
- Wen L, Cao Y, Cheng Q, et al. Objectively measured near work, outdoor exposure and myopia in children. Br J Ophthalmol. 2020;104(11):1542–1547. doi:10.1136/bjophthalmol-2019-315258
- Zhang Y, Su M, Sun Y, et al. Clouclip combined with a questionnaire on the influence factors of myopia in children. Front Pediatr. 2023;11:1228257. doi:10.3389/fped.2023.1228257
- Wen L, Cheng Q, Lan W, et al. An objective comparison of light intensity and near-visual tasks between rural and urban school children in china by a wearable device clouclip. Transl Vis Sci Technol. 2019;8(6):15. doi:10.1167/tvst.8.6.15
- Bhandari KR, Shukla D, Mirhajianmoghadam H, Ostrin LA. Objective measures of near viewing and light exposure in schoolchildren during COVID-19. Optom Vis Sci. 2022;99(3):241–252. doi:10.1097/OPX.0000000000001871
- Cao Y, Lan W, Wen L, et al. An effectiveness study of a wearable device (Clouclip) intervention in unhealthy visual behaviors among school-age children: a pilot study. Medicine. 2020;99(2):e17992. doi:10.1097/MD.0000000000017992
- Verkicharla PK, Ramamurthy D, Nguyen QD, et al. Development of the fitSight fitness tracker to increase time outdoors to prevent myopia. Transl Vis Sci Technol. 2017;6(3):20. doi:10.1167/tvst.6.3.20
- Ostrin LA. Objectively measured light exposure in emmetropic and myopic adults. Optom Vis Sci. 2017;94(2):229–238. doi:10.1097/OPX.0000000000001013
- Read SA, Collins MJ, Vincent SJ. Light exposure and physical activity in myopic and emmetropic children. Optom Vis Sci. 2014;91(3):330–341. doi:10.1097/OPX.0000000000000160
- Mirhajianmoghadam H, Piña A, Ostrin LA. Objective and subjective behavioral measures in myopic and non-myopic children during the COVID-19 Pandemic. Transl Vis Sci Technol. 2021;10(11):4. doi:10.1167/tvst.10.11.4
- Abbott KS, Queener HM, Ostrin LA. The ipRGC-driven pupil response with light exposure, refractive error, and sleep. Optom Vis Sci. 2018;95(4):323–331. doi:10.1097/OPX.0000000000001198
- Ulaganathan S, Read SA, Collins MJ, Vincent SJ. Daily axial length and choroidal thickness variations in young adults: associations with light exposure and longitudinal axial length and choroid changes. Exp Eye Res. 2019;189:107850. doi:10.1016/j.exer.2019.107850
- Flanagan SC, Cobice D, Richardson P, Sittlington JJ, Saunders KJ. Elevated melatonin levels found in young myopic adults are not attributable to a shift in circadian phase. Invest Ophthalmol Vis Sci. 2020;61(8):45. doi:10.1167/iovs.61.8.45
- Dhakal R, Rudrapankte JR, Chittajallu HSNS, et al. Development and validation of a ‘MyLyt’ wearable light tracking device. Ophthalmic Physiol Opt. 2023;43(1):132–140. doi:10.1111/opo.13061
- Dharani R, Lee CF, Theng ZX, et al. Comparison of measurements of time outdoors and light levels as risk factors for myopia in young Singapore children. Eye. 2012;26(7):911–918. doi:10.1038/eye.2012.49
- Alvarez AA, Wildsoet CF. Quantifying light exposure patterns in young adult students. J Mod Opt. 2013;60(14):1200–1208. doi:10.1080/09500340.2013.845700
- Ye B, Liu K, Cao S, et al. Discrimination of indoor versus outdoor environmental state with machine learning algorithms in myopia observational studies. J Transl Med. 2019;17(1):314. doi:10.1186/s12967-019-2057-2
- Williams R, Bakshi S, Ostrin EJ, Ostrin LA. Continuous objective assessment of near work. Sci Rep. 2019;9(1):6901. doi:10.1038/s41598-019-43408-y
- Ribeiro F, Ferreira TB, Silva D, Matos AC, Gaspar S, Piñero DP. Analysis of daily visual habits in a presbyopic population. J Ophthalmol. 2023;2023:6440954. doi:10.1155/2023/6440954
- Mrochen M, Zakharov P, Tabakcι BN, Tanrιverdi C, Kιlιç A, Flitcroft DI. Visual lifestyle of myopic children assessed with AI-powered wearable monitoring. Invest Ophthalmol Vis Sci. 2020;61(7):82.
- Ramamurthy D, Samuel Paulraj AK, Lakshmi T, Rajagopalan T, Lavanya R. Development of smart spectacles to monitor and modify myopia-related health behaviour in children. Ophthalmic Physiol Opt. 2023;43(3):517–524. doi:10.1111/opo.13119
- Deemer AD, Bradley CK, Ross NC, et al. Low vision enhancement with head-mounted video display systems: are we there yet? Optom Vis Sci. 2018;95(9):694–703. doi:10.1097/OPX.0000000000001278
- 6 Vision enhancement devices you need to see. Available from: https://www.nanalyze.com/2017/04/6-vision-enhancement-devices/. Accessed October 28, 2023.
- Nguyen XT, Koopman J, van Genderen MM, Stam HLM, Boon CJF. Artificial vision: the effectiveness of the OrCam in patients with advanced inherited retinal dystrophies. Acta Ophthalmol. 2022;100(4):e986–e993. doi:10.1111/aos.15001
- Cottingham E, Burgum F, Gosling S, Woods L, Tandon A. Assessment of the impact of a head-mounted augmented reality low vision aid on vision and quality of life in children and young people with visual impairment. Br Ir Orthopt J. 2024;20(1):57–68. doi:10.22599/bioj.345
- Crossland MD, Starke SD, Imielski P, Wolffsohn JS, Webster AR. Benefit of an electronic head-mounted low vision aid. Ophthalmic Physiol Opt. 2019;39(6):422–431. doi:10.1111/opo.12646
- SHG Technologies - Home. Available from: https://www.shgtechnologies.com/. Accessed October 28, 2023.
- Dagnelie G, Christopher P, Arditi A, et al. Performance of real-world functional vision tasks by blind subjects improves after implantation with the Argus® II retinal prosthesis system. Clin Exp Ophthalmol. 2017;45(2):152–159. doi:10.1111/ceo.12812
- Holden BA, Fricke TR, Wilson DA, et al. Global Prevalence of myopia and high myopia and temporal trends from 2000 through 2050. Ophthalmology. 2016;123(5):1036–1042. doi:10.1016/j.ophtha.2016.01.006
- Vongphanit J, Mitchell P, Wang JJ. Prevalence and progression of myopic retinopathy in an older population. Ophthalmology. 2002;109(4):704–711. doi:10.1016/s0161-6420(01)01024-7
- Ogawa A, Tanaka M. The relationship between refractive errors and retinal detachment--analysis of 1,166 retinal detachment cases. Jpn J Ophthalmol. 1988;32(3):310–315.
- Chang MA, Congdon NG, Bykhovskaya I, Munoz B, West SK. The association between myopia and various subtypes of lens opacity: SEE (Salisbury Eye Evaluation) project. Ophthalmology. 2005;112(8):1395–1401. doi:10.1016/j.ophtha.2005.02.017
- Marcus MW, de Vries MM, Junoy Montolio FG, Jansonius NM. Myopia as a risk factor for open-angle glaucoma: a systematic review and meta-analysis. Ophthalmology. 2011;118(10):1989–1994.e2. doi:10.1016/j.ophtha.2011.03.012
- Turnbull PRK, Phillips JR. Ocular effects of virtual reality headset wear in young adults. Sci Rep. 2017;7(1):16172. doi:10.1038/s41598-017-16320-6
- Ellrich J, Hoseini-Yazdi H, Read SA, Collins MJ, Bahmani H, Schilling T. Modulation of axial length by repeated blue light stimulation of the optic nerve head in human volunteers. Invest Ophthalmol Vis Sci. 2023;64(8):5091.
- Thakur S, Dhakal R, Verkicharla PK. Short-term exposure to blue light shows an inhibitory effect on axial elongation in human eyes independent of defocus. Invest Ophthalmol Vis Sci. 2021;62(15):22. doi:10.1167/iovs.62.15.22
- Jiang Y, Zhu Z, Tan X, et al. Effect of repeated low-level red-light therapy for myopia control in children: a multicenter randomized controlled trial. Ophthalmology. 2022;129(5):509–519. doi:10.1016/j.ophtha.2021.11.023
- Torii H, Mori K, Okano T, et al. Short-term exposure to violet light emitted from eyeglass frames in myopic children: a randomized pilot clinical trial. J Clin Med. 2022;11(20):6000. doi:10.3390/jcm11206000
- Tayyaba S, Ashraf MW, Alquthami T, Ahmad Z, Manzoor S. Fuzzy-based approach using IOT devices for smart home to assist blind people for navigation. Sensors. 2020;20(13):3674. doi:10.3390/s20133674
- Bajpai S, Radha D, Smart phone as a controlling device for smart home using speech recognition, International Conference on Communication and Signal Processing (ICCSP). Chennai, India; 2019:0701–0705, doi: 10.1109/ICCSP.2019.8697923.
- Smart home technology creates Independence for patients with disabilities. Available from: https://www.optometrytimes.com/view/smart-home-technology-creates-independence-patients-disabilities. Accessed October 30, 2023.