Abstract
Background
Calycosin, an active compound in plants, can promote the apoptosis of various cancer cells; however, the mechanism by which it regulates reactive oxygen species (ROS) in gastric cancer (GC) cells remains unclear.
Purpose
In this study, we investigated the effects of calycosin on apoptosis, the cell cycle, and migration in GC cells under ROS regulation.
Results
The results of the Cell Counting Kit-8 assay suggested that calycosin had significant cytotoxic effects on 12 gastric cancer cells, but no significant cytotoxic effects on normal cells. Hoechst 33342/propidium iodide (PI) double staining and flow cytometry showed that calycosin had clear pro-apoptotic effects on AGS cells. Western blotting revealed that the expression of cytochrome C and pro-apoptotic proteins B-cell lymphoma 2 (Bcl-2)-associated agonist of cell death (Bad), cleaved (cle)-caspase-3, and cle-poly (ADP-ribose) polymerase gradually increased, and the expression of anti-apoptotic protein Bcl-2 gradually decreased. Calycosin also decreased the expression of extracellular signal-regulated kinase, nuclear factor kappa B (NF-κB), and signal transducer and activator of transcription 3 (STAT3), and increased the phosphorylation levels of p38, c-Jun N-terminal kinase, and inhibitor of NF-κB. In addition, calycosin markedly increased ROS accumulation, and pretreatment with active oxygen scavenger n-acetyl-l-cysteine (NAC) clearly inhibited apoptosis. Calycosin downregulated the cell cycle proteins cyclin-dependent kinase 2 (CDK2), CDK4, CDK6, cyclin D1, and cyclin E; upregulated p21 and p27; and arrested cells in the G0/G1 phase. Similarly, calycosin also downregulated Snail family transcriptional repressor 1, E-cadherin, and β-catenin and inhibited cell migration. However, pretreatment with NAC inhibited the calycosin-induced effects of cycle arrest and migration.
Conclusion
In summary, calycosin induces apoptosis via ROS-mediated MAPK/STAT3/NF-κB pathways, thereby exerting its anti-carcinogenic functions in GC cells.
Introduction
With the irregular diet and life of modern people, the incidence of gastric cancer (GC) is gradually increasing. According to the prediction of Joinpoint software, the total number of GC cases will increase with the aging of China’s population, which is a serious threat to human health and life.Citation1,Citation2 Although significant progress has been made in GC treatments over the past few decades, the survival rate of GC patients remains poor.Citation3,Citation4 Several first-line chemotherapy drugs inevitably lead to resistance, strong adverse reactions, and toxicity that damages the normal cell morphology, resulting in low survival rates.Citation5 The above factors have greatly limited advancements in chemotherapy. To overcome this issue with chemotherapy drugs and improve the survival rate, highly efficient Chinese herbs with low toxicity during the treatment process have recently garnered increasing attention.
In the process of aerobic cell metabolism, if oxygen is not completely reduced to water, it generates oxygen free radicals and non-radical oxygen, both of which are known as reactive oxygen species (ROS).Citation6,Citation7 Excessive ROS are generated in mitochondria from tumor cells, which can cause damage to the bacterial biofilm on the cell and simultaneously suppress cancer cell proliferation, and cause apoptosis, cell cycle arrest, and migration by regulating the mitogen-activated protein kinase (MAPK), signal transducer and activator of STAT3, and NF-κB signaling pathways.Citation8–Citation10 These processes are involved in oxidative stress. Cytochrome C and the activation of caspase-3 can cleave the PARP substrates, including the pro-apoptotic protein B-cell lymphoma 2 (Bcl-2)-associated agonist of cell death (Bad) and anti-apoptotic protein B-cell lymphoma 2 (Bcl-2), all of which promote activation of cell apoptotic pathways.Citation11,Citation12
Research on the treatment of cancer by traditional Chinese medicine (TCM) extracts has led to great progress in understanding the mechanism of action and mode of administration of a single Chinese medicine.Citation13–Citation15 Calycosin is a fat-soluble component in the dried root of Astragalus membranaceus, which are organic compounds widely found in nature. Calycosin exerts various anti-tumor activities in vitro and in vivo,Citation16–Citation18 but its mechanism of action in human GC cells is not clear.
In this study, we evaluated the effects of calycosin on apoptosis, the cell cycle, and migration of GC cells under ROS regulation. In addition, we also determined the roles of MAPK/STAT3/NF-κB pathways in these processes.
Materials and Methods
Cell Lines and Cell Culture
Twelve types of GC cells (AGS, KATO-3, MKN-28, MKN-45, NCI-N87, SNU-5, SNU-216, SNU-484, SNU-668, YCC-1, YCC-6, and YCC-16) and normal human lung cells IMR-90 were purchased from American Type Culture Collection (Manassas, VA, USA), and three normal cell lines (GES-1, L-02, and 293T) were purchased from Saiqi Biotech Co., Ltd (Shanghai, China). AGS, KATO-3, MKN-28, MKN-45, SNU-5, SNU-216, SNU-484, SNU-668, IMR-90, and L-02 cells were maintained in RPMI 1640 medium (Gibco, Waltham, MA, USA). NCI-N87, YCC-1, YCC-6, YCC-16, GES-1, and 293T cells were grown in Dulbecco’s Modified Eagle Medium (Gibco) supplemented with 10% fetal bovine serum (Gibco), 100 U/mL penicillin (Gibco), and 100 μg/mL streptomycin (Gibco). All cells were cultured in a humidified atmosphere of 5% CO2 incubator (Sanyo, Osaka, Japan) at 37°C, and the number of cells maintained at 80%.
Cell Counting Kit-8 Assay
The cytotoxic effects of calycosin on 12 GC cells and 4 normal cells were assessed using the Cell Counting Kit-8 (CCK-8) assay (Solabio, Beijing, China). In brief, 16 cells in the logarithmic growth phase were inoculated into a 96-well plate (1×104 cells/well) overnight. Then the cells in each well were treated with calycosin (Chengdu, China) and cisplatin (Princeton, NJ, USA), and 10 μL CCK-8 (Solarbio, Beijing, China) solution, and the cell viability was measured by the enzyme-linked immunosorbent assay (BioTek Instruments, Inc., Winooski, VT, USA).
Cell Apoptosis Analysis
AGS cells were seeded in a 6-well plate and treated with 47 μM calycosin for 24 h. Then the cells were detected using the Hoechst 33342/PI Dual Staining Kit (Solarbio), Annexin V Apoptosis Detection Kit (Solarbio), and Mitochondrial Membrane Potential Assay Kit (Solarbio). According to the manufacturer’s instructions, the cells were stained with Hoechst 33342 and PI for 15 min. The cell morphology was observed using the EVOS FL Auto Cell Imaging System (Thermo Fisher Scientific, Waltham, MA, USA). Furthermore, the AGS cells were cultured in 95 μL Annexin V Binding Buffer, followed by the addition of Annexin V-FITC and PI, after which the apoptotic cells were analyzed by flow cytometry (Beckman Coulter, Inc., Brea, CA, USA). In addition, the AGS cells were incubated with 1 mL JC-1 staining solution at 37°C for 20 min, and changes in mitochondrial membrane potential were analyzed by flow cytometry.
Measurement of Cellular ROS Levels
AGS cells were treated with 47 μM calycosin for different time points (3, 6, 12, and 24 h) in the logarithmic growth phase. The cells were centrifuged for 5 min at 6000×g, followed by washing with phosphate-buffered saline (PBS). The cells were stained with 10 μmol/L DCFH-DA probe (Beyotime Institute Biotechnology, Shanghai, China) in a 37°C constant temperature water bath (Senxin, Jiangsu, China) for 30 min. After the cells were re-suspended with PBS, the level of intracellular ROS was measured using a flow cytometer. AGS cells were pretreated by adding n-acetyl-l-cysteine (NAC) (Beyotime Institute Biotechnology), and apoptotic cells were analyzed by flow cytometry.
Analysis of Cell Cycle Arrest
AGS cells in the logarithmic growth phase were exposed to calycosin for different time points (3, 6, 12, and 24 h), and fixed in 70% EtOH overnight at 4°C. Then AGS cells were stained using the DNA Content Quantitation Assay Kit (Solarbio), followed by incubation in a 37°C constant temperature water bath for 30 min, and the addition of 400 μL PI at 4°C (Sanyo, Osaka, Japan) for 30 min. The DNA content in the AGS cells treated with calycosin was measured by flow cytometry, and the cell cycle arrest was analyzed.
Wound Healing Assay
The AGS cells in logarithmic growth phase were seeded in a 6-well plate and cultured to a monolayer. Then scratches were generated by a 200 µL pipette. The remaining cells were washed with PBS and cultured in serum-free RPMI 1640 medium. After 2 h, 47 μM calycosin was added to the cells, and images were taken at 3, 6, 12, and 24 h.
Preparation of Nuclear Extract
Nuclear proteins were extracted using the NE-PER Nuclear and Cytoplasmic Extraction Reagent Kit (Thermo Fisher Scientific). Cells in a 3.5 cm dish were harvested and washed with pre-cooled PBS, followed by the addition of 60 μL Cytoplasmic Extraction Reagent I for 15 min and incubation with Cytoplasmic Extraction Reagent II for 3 min. The cells were centrifuged (Eppendorf, Hamburg, Germany) for 10 min at 13,000×g. The Nuclear Extraction Reagent for precipitation was shaken once every 10 min, and the nuclear proteins were collected from the supernatant.
Western Blot Analysis
AGS cells were extracted in lysis buffer, and then centrifuged for 30 min at 12,000×g at 4°C. The protein sample was calculated based on the measured OD595 value and the standard curve. The protein sample was separated on 8–12% SDS-PAGE gels and then transferred onto nitrocellulose membranes (Pall Corp., Hauppauge, NY, USA). The desired target bands were cut out and blocked in 5% skim milk (Becton Dickinson, Franklin Lakes, NJ, USA) at room temperature (RT). Then the membrane was incubated overnight with primary antibodies (Santa Cruz Biotechnology, Inc., Dallas, USA) at 4°C. Then the membrane was incubated with 1 μL goat anti-mouse IgG or goat anti-rabbit IgG secondary antibody (ZSGB Bio, Inc., Beijing, China) at RT for 2 h. Following the incubation, Pierce ECL substrate (Thermo Fisher Scientific) was added to the membrane, and proteins were photographed using Amersham Imager 600 (GE, Fairfield, CT, USA).
Statistics
All data were analyzed by SPSS 20.0, and analyses were the average of at least three replicates. The results are presented as x±s. Differences in mean values between two groups were analyzed by the t-test, and *p < 0.05, **p < 0.01, and ***p < 0.001 were considered statistically significant.
Results
Cytotoxic Effects of Calycosin on Human GC Cells
Calycosin had significant cytotoxic effects on the survival of 12 different types of GC cells in concentration- and time-dependent manners, and the toxicity was stronger than that of cisplatin ( and ), but was lower than cisplatin in the 4 normal cell lines ( and ). The IC50 values of cisplatin and calycosin for each cell line are shown in . AGS cells had the lowest IC50 value of 47.18 μM and was most sensitive to calycosin. Therefore, AGS cells were selected for subsequent experiments.
Table 1 IC50 Value of Cisplatin and Calycosin in Twelve GC Cell Lines
Figure 1 Cytotoxic effects of calycosin on GC cells. (A) Twelve human GC cell lines (AGS, KATO-3, MKN-28, MKN-45, NCI-N87, SNU-5, SNU-216, SNU-484, SNU-668, YCC-1, YCC-6, and YCC-16) were treated with different concentrations of calycosin and cisplatin for 24 h, and the cell cytotoxic effects were determined by the CCK-8 assay. (B) Four human normal cell lines (GES-1, IMR-90, L-02, and 293T) were treated with different concentrations of calycosin and cisplatin for 24 h, and the cell cytotoxic effects were determined by the CCK-8 assay. (C) Twelve human GC cell lines were treated with the IC50 of calycosin and cisplatin for 0, 3, 6, 12, 24, and 48 h, and the cell cytotoxic effects were determined by the CCK-8 assay. (D) Four human normal cell lines were treated with the IC50 of calycosin and cisplatin for 0, 3, 6, 12, 24, and 48 h, and the cell cytotoxic effects were determined by the CCK-8 assay. Representative data from at least three independent tests. *p < 0.05, **p < 0.01, and ***p < 0.001.
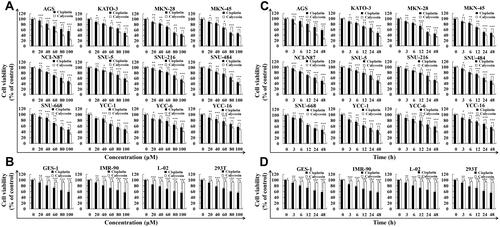
Calycosin Induces Apoptosis in AGS Cells
Hoechst 33342/PI double staining verified that calycosin significantly increased AGS cell apoptosis to 62.11% at 24 h. The rounded edges of apoptotic cells were blurred, and the degree of apoptosis was higher than that in the cisplatin-treated group (). The time of AGS cells with calycosin-treated was positively correlated with the degree of apoptosis, and the number of apoptosis cells reached 64.09%. It is similar with under a microscope at 24 h (). As the treatment time increased, the red-green ratio significantly decreased, and the mitochondrial membrane potential in the AGS cells decreased to 57.66%. The decrease was most significant at 24 h after treatment (). In addition, calycosin significantly increased the protein expression levels of Bad, cle-caspase-3, cle-PARP, and cytochrome C, while decreasing the protein expression levels of Bcl-2 (). Taken together, these results indicate that calycosin may induce AGS cell apoptosis.
Figure 2 Calycosin induces apoptosis on AGS cells. (A) AGS cells were treated with 47 μM calycosin (0, 3, 6, 12, and 24 h) and stained with Hoechst 33342/PI. Fluorescence microscopy of apoptotic AGS cells (original magnification: 400×). (B) AGS cells were stained with Annexin V-FITC/PI and analyzed by flow cytometry. (C) AGS cells were labeled with the JC-1 fluorescent probe and analyzed by flow cytometry. (D) Western blot analysis of the expression levels of Bad, Bcl-2, cle-caspase-3, cle-PARP, and cytochrome C in AGS cells treated with calycosin for different times (0, 3, 6, 12, and 24 h). Representative images from at least three independent tests. *p < 0.05, **p < 0.01, and ***p < 0.001.
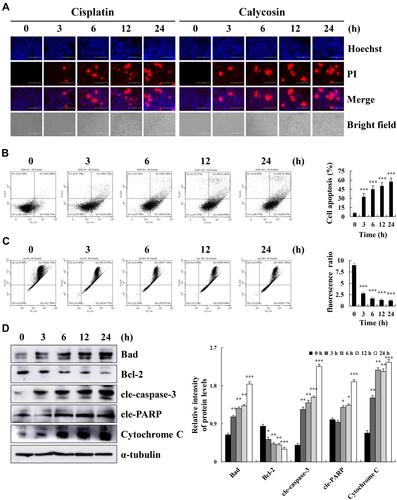
Calycosin Induces Apoptosis via the MAPK/STAT3/NF-κB Signaling Pathways in AGS Cells
Calycosin treatment increased the expression levels of p-p38, p-JNK, and IκB-α and decreased the expression levels of p-ERK, p-STAT3, and NF-κB (p65) (). The decreased expression of nuclear localization signals STAT3, NF-κB, and IκB-α was detected by Western blotting (). In addition, the FR180204 (ERK inhibitor), SB203580 (p38 inhibitor), and SP600125 (JNK inhibitor) were used to further investigate the role of calycosin in inducing apoptosis via MAPK and STAT3 signaling pathways. Inhibitors can increase the protein expression level of p-STAT3 and decrease expression of cle-caspase-3 (–). Taken together, these results demonstrate that the effects of calycosin on apoptosis may be via the MAPK/STAT3/NF-κB signaling pathways, and MAPK may be involved in regulating the STAT3 signaling pathway in AGS cells.
Figure 3 Calycosin affects the expression of MAPK, STAT3, and NF-κB pathway-related proteins in AGS cells. (A) AGS cells were treated with calycosin (0, 3, 6, 12, and 24 h). The expression levels of p-ERK, p-p38, p-JNK, p-STAT3, NF-κB, and IκB-α were detected by Western blotting; α-tubulin was used as the internal control. (B) The expression levels of nuclear STAT3, NF-κB, and IκB-α were detected by Western blotting, and Lamin B1 was used as a nuclear loading control. (C) AGS cells were pretreated with FR180204 (12.1 μmol/L) for 30 min, and the expression levels of p-ERK, ERK, p-STAT3, STAT3 and cle-caspase-3 were detected by Western blotting. (D) AGS cells were pretreated with SB203580 (12.1 μmol/L) for 30 min, and the expression levels of p-p38, p38, p-STAT3, STAT3 and cle-caspase-3 were detected by Western blotting. (E) AGS cells were pretreated with SP600125 (12.1 μmol/L) for 30 min, and the expression levels of p-JNK, JNK, p-STAT3, STAT3 and cle-caspase-3 were detected by Western blotting. Representative data from at least three independent tests. *p < 0.05, **p < 0.01, and ***p < 0.001.
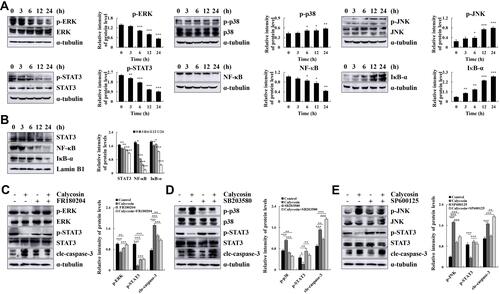
Calycosin Induces AGS Cell Apoptosis Through ROS-Mediated MAPK/STAT3/NF-κB Pathways
Calycosin significantly increased the level of ROS to 81.52% in a time-dependent manner (). Compared with calycosin, apoptosis was significantly reduced to 19.37% after NAC pretreatment (). In addition, NAC markedly increased the expression levels of p-ERK, p-STAT3, and NF-κB (p65), and decreased the expression levels of p-JNK, p-p38, cle-caspase-3, and IκB-α (). Additionally, STAT3, NF-κB, and IκB-α nuclear localization signals proteins were clearly reversed after NAC treatment (). These results indicated that calycosin elevates the level of ROS and promotes AGS cell apoptosis through the MAPK/STAT3/NF-κB pathways.
Figure 4 The effect of calycosin on intracellular ROS levels in AGS cells. (A) AGS cells were treated with calycosin (47 μM), and ROS generation was analyzed by flow cytometry. (B) The apoptosis of AGS cells was analyzed after pretreatment with NAC by flow cytometry. (C) Western blotting was used to analyze the expression of p-ERK, p-p38, p-JNK, p-STAT3, NF-κB, IκB-α, cle-caspase-3, cle-PARP, and cytochrome C protein; α-tubulin was used as the internal control. (D) Nuclear STAT3, NF-κB, and IκB-α expression levels were determined by Western blotting; Lamin B1 was used as a nuclear loading control. Representative data from at least three independent tests. *p < 0.05, **p < 0.01, and ***p < 0.001.
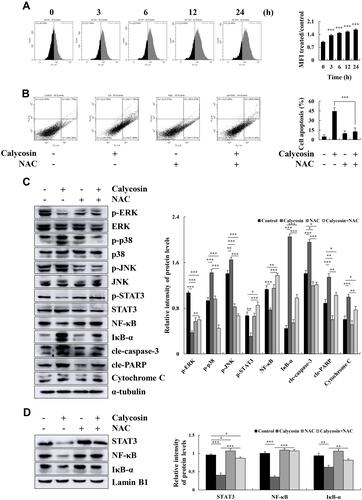
Calycosin Induces G0/G1 Cell Cycle Arrest Through ROS-Mediated Signaling Pathways in AGS Cells
Over time, calycosin increased the number of cells in the G0/G1 phase to 75.24%, and the proportion of cells in the G2/M phase decreased to 9.84% over time (). The expression levels of cell cycle-related proteins were detected by Western blotting. The results showed that the expression levels of p21 and p27 were increased, and those of CDK2, CDK4, CDK6, cyclin D1, and cyclin E were decreased (). The number of calycosin-treated cells in the G0/G1 phase returned to 56.13%, and the G2/M phase returned to 25.68% after pretreatment with NAC (). Relevant cell cycle-related proteins were also significantly reversed ().
Figure 5 Effects of calycosin on the cell cycle and the expression of cell cycle-related proteins in AGS cells. (A) AGS cells were treated with 47 μM calycosin for 0, 3, 6, 12, and 24 h, and cell cycle distribution was analyzed by flow cytometry. (B) The expression levels of G0/G1 cell cycle proteins CDK2, CDK4, CDK6, cyclin D1, cyclin E, p21, and p27 were examined by Western blotting; α-tubulin was used as the internal control. (C) The cell cycle of AGS cells was analyzed after pretreatment with NAC by flow cytometry. (D) The expression level of cell cycle was analyzed by Western blotting after pretreatment with NAC; α-tubulin was used as the internal control. Representative data from at least three independent tests. *p < 0.05, **p < 0.01, and ***p < 0.001.
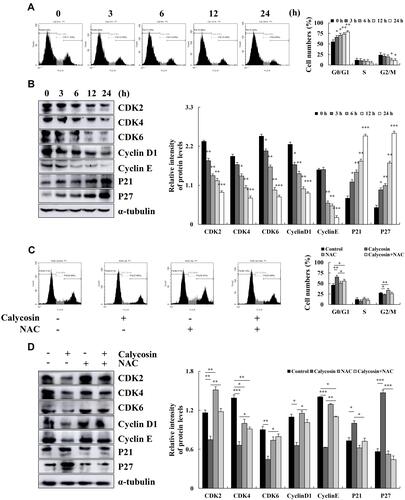
Calycosin Inhibits Cells Migration Through ROS-Mediated Signaling Pathways in AGS Cells
Calycosin inhibited AGS cell migration compared to the control group (). The expression levels of migration-associated proteins were detected by Western blotting, and calycosin significantly reduced the expression levels of SNAI 1, E-cadherin, and β-catenin (). Moreover, the inhibitory effect of calycosin was weakened after NAC was added (), and the levels of migration-associated proteins were increased after the addition of NAC ().
Figure 6 Effect of calycosin on the migration of AGS cells. (A) AGS cells were treated with calycosin (47 μM), and the images were taken under an inverted microscope. (B) Western blotting was used to analyze the expression of SNAI 1, E-cadherin, and β-catenin. (C) AGS cells were pretreated with NAC, followed by the addition of calycosin (47 μM) and imaging under an inverted microscope. (D) The expression of SNAI 1, E-cadherin, and β-catenin was analyzed after pretreatment with NAC; α-tubulin was used as the internal control. Representative data from at least three independent tests. *p < 0.05, **p < 0.01, and ***p < 0.001.
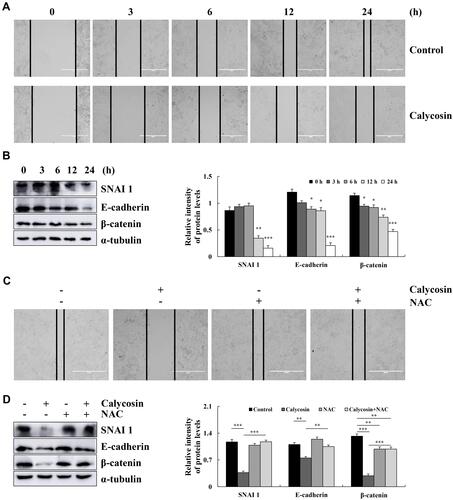
Discussion
TCM extracts inhibit cancer cell growth, proliferation, and differentiation, and thus have garnered great interest by researchers.Citation19 However, TCMs often have a wide variety of characteristics and complex composition. The single components of Astragalus have been studied and showed that calycosin can inhibit the proliferation of osteosarcoma MG-63 cells and liver cancer BEL-7402 cells.Citation20 Our study found that calycosin significantly inhibited the 12 types of GC cells ( and ), with little to no cytotoxicity in the 4 normal cell lines ( and ). To determine the potential mechanism of cytotoxicity, calycosin and AGS cells were used as research objects, studied in depth, and calycosin regulated ROS was found to induce AGS cells apoptosis.
Apoptosis plays an important role in cancer treatment, and the mitochondrial pathway is the main pathway of apoptosis.Citation21–Citation23 A growing amount of evidence suggests that calycosin can potentially downregulate the anti-apoptotic protein Bcl-2; increase the expression levels of pro-apoptotic proteins cle-caspase-3, cle-PARP, and cytochrome C; and induce human osteosarcoma by regulating the PI3K/AKT/mTOR signaling pathway.Citation24,Citation25 In this study, Hoechst 33342 and PI double staining showed that calycosin can promote cell apoptosis (). More importantly, compared with the control groups, calycosin increased early and late apoptosis in AGS cells (), and decreased the mitochondrial membrane potential (). Our results showed that calycosin promoted AGS apoptosis by upregulating the levels of Bad, cle-PARP, cle-caspase-3, and cytochrome C and downregulating Bcl-2 levels (). In addition, studies have shown that suppression of A549 cell proliferation and metastasis by calycosin via inhibition of the PKCα/ERK1/2 pathway.Citation26–Citation30 Our results indicated that the calycosin may regulate the MAPK/STAT3/NF-κB pathway proteins binding to DNA or transcriptional activity, thereby promoting apoptosis in AGS cells ( and ). To further investigate whether MAPK activation can regulate STAT3, MAPK inhibitors (FR180204, SP600125, and SB203580) were used to detect changes in STAT3 protein content. The results indicated that MAPK is located upstream of STAT3 (–).
Oxidative stress and cellular redox imbalance will lead to excessive ROS, which promote apoptosis and necrosis under many physiological or pathological conditions.Citation31,Citation32 Previous studies have shown that ROS is a second messenger that mediates apoptosis by activating the MAPK cascade NF-κB transcription factor.Citation33 The phenolic hydroxyl groups at the C3ʹ and C7ʹ positions of the calycosin molecules are most likely to be active sites for the elimination of oxidative free radicals. In addition, calycosin has relatively small polarity, which not only removes oxidative free radicals but also penetrates the lipid bilayer to counteract lipid peroxidation.Citation34 Our results indicated that the time to treat AGS cells with calycosin was positively correlated with the amount of ROS produced (). In addition, cell apoptosis () and MAPK/STAT3/NF-κB ( and ) were significantly reversed after NAC was added. These results showed that calycosin can upregulate ROS to promote cell apoptosis via MAPK/STAT3/NF-κB signaling pathway.
When cell proliferation is not restrained by a homeostatic mechanism, the tissue or organ can progress to cancer.Citation35–Citation37 Calycosin may induce G0/G1 cell cycle arrest by promoting ROS production and regulating apoptosis-related signaling pathways.Citation38 In this study, calycosin significantly reduced the levels of cyclin D1, cyclin E, CDK2, CDK4, and CDK6, and increased the expression levels of p27 and p21 in the arrested cell cycle at G0/G1 ( and ). Similarly, calycosin inhibited cell migration ( and ). Taken together, cell cycle ( and ) and migration ( and ) were significantly reversed after NAC pretreatment, which indicated that calycosin can upregulate ROS in cells arrested at the G0/G1 phase and inhibit cell migration.
Calycosin has anti-oxidation effects.Citation39 Thus, we tested whether calycosin could specifically induce an increase in ROS in GC cells, but have antioxidant effects in normal cells (Supplementary Figure S1). Although we do not have clear proof that calycosin has a targeting effect on certain cell membrane receptors, understanding the signal transduction relationships among profiles of calycosin can pave the way to progress on novel therapies with greater curative efficacy and fewer side effects. In addition, calycosin, a traditional Chinese medicine compound, the research and clinical application are still in its infancy. How calycosin binds to membrane surface receptors to enter cells, and by which ways it inhibits tumor cells proliferation and regulate immune activity needs to be further identified. Based on this, combined with relevant clinical practice theory, the calycosin is studied in depth from animal tumor model, various tumor cell models, and molecular level, is needed to promote the development of calycosin as a Chinese medicine target molecule for therapy tumors and lay a new cornerstone to improve the survival of life of cancer patients.
Conclusion
The molecular mechanism underlying the induction of apoptosis in AGS cells by calycosin includes increased levels of ROS and regulation of MAPK/STAT3/NF-κB signaling pathway-associated proteins, which leads to G0/G1 cell cycle arrest and inhibition of cell growth and migration (). These results demonstrate that calycosin may be a potential target for the treatment of GC.
Ethics Statement
This article does not contain any studies with human or animal subjects.
Acknowledgment
We thank LetPub (www.letpub.com) for its linguistic assistance during the preparation of this manuscript.
Disclosure
The authors declare they have no conflicts of interest for this work.
Additional information
Funding
References
- Wang F, Shen L, Li J, et al. The Chinese Society of Clinical Oncology (CSCO): clinical guidelines for the diagnosis and treatment of gastric cancer. Cancer Commun. 2019;39(1):10–41. doi:10.1186/s40880-019-0349-9
- McCall MD, Graham PJ, Bathe OF. Quality of life: a critical outcome for all surgical treatments of gastric cancer. World J Gastroenterol. 2016;22(3):1101–1113. doi:10.3748/wjg.v22.i3.1101
- Jian W, Jia Z, Jun L, et al. High tumor-associated macrophages infiltration is associated with poor prognosis and may contribute to the phenomenon of epithelial-mesenchymal transition in gastric cancer. Onco Targets Ther. 2016;9:3975–3983. doi:10.2147/OTT.S103112
- Purohit V, Simeone DM, Lyssiotis CA. Metabolic regulation of redox balance in cancer. Cancers. 2019;11(7):955–979. doi:10.3390/cancers11070955
- Huang M, Zhang L, Ding J, et al. Anticancer drug discovery from Chinese medicinal herbs. Chin Med. 2018;13(1):35–44. doi:10.1186/s13020-018-0192-y
- Yang H, Villani RM, Wang H, et al. The role of cellular reactive oxygen species in cancer chemotherapy. J Exp Clin Cancer Res. 2018;37(1):266–276. doi:10.1186/s13046-018-0909-x
- Jalmi SK, Sinha AK. ROS mediated MAPK signaling in abiotic and biotic stress-striking similarities and differences. Front Plant Sci. 2015;6:769–778. doi:10.3389/fpls.2015.00769
- Keuss MJ, Hjerpe R, Hsia O, et al. Unanchored tri-NEDD8 inhibits PARP-1 to protect from oxidative stress-induced cell death. EMBO J. 2019;38(6):e100024. doi:10.15252/embj.2018100024
- Huang Z, Liu CA, Cai PZ, et al. Omega-3PUFA attenuates MNU-induced colorectal cancer in rats by blocking PI3K/AKT/BCL-2 signaling. Onco Targets Ther. 2020;13:1953–1965. doi:10.2147/OTT.S241298
- Zhang XC. Effects of polydatin on the proliferation, migration, and invasion of ovarian cancer. BIOCELL. 2019;43(4):313–319. doi:10.32604/biocell.2019.07973
- Alhoshani A, Alatawi FO, Al-Anazi FE, et al. BCL-2 inhibitor venetoclax induces autophagy-associated cell death, cell cycle arrest, and apoptosis in human breast cancer cells. Onco Targets Ther. 2020;13:13357–13370. doi:10.2147/OTT.S281519
- Julien O, Wells JA. Caspases and their substrates. Cell Death Differ. 2017;24(8):1380–1389. doi:10.1038/cdd.2017.44
- Liu Y, Xie X, Wang W, et al. A randomized controlled trial for the effect of Modified Shenling Baizhu Powder on delaying the illness progress of COPD stable phase patients (GOLD 1-2 stages): a study protocol. Medicine. 2020;99(43):e22700. doi:10.1097/MD.0000000000022700
- Lu Y, Yang J, Wang X, et al. Research progress in use of traditional Chinese medicine for treatment of spinal cord injury. Biomed Pharmacother. 2020;127:110136. doi:10.1016/j.biopha.2020.110136
- Wu SY, Wang WJ, Dou JH, et al. Research progress on the protective effects of licorice-derived 18β-glycyrrhetinic acid against liver injury. Acta Pharmacol Sin. 2020;42:18–26. doi:10.1038/s41401-020-0383-9
- Jiang Y, Sun W, Li W, et al. Calycosin-7-O-β-D-glucoside promotes oxidative stress-induced cytoskeleton reorganization through integrin-linked kinase signaling pathway in vascular endothelial cells. BMC Complement Altern Med. 2015;15(1):315–326. doi:10.1186/s12906-015-0839-5
- Zhou R, Chen H, Chen J, et al. Extract from Astragalus membranaceus inhibit breast cancer cells proliferation via PI3K/AKT/mTOR signaling pathway. BMC Complement Altern Med. 2018;18(1):83–91. doi:10.1186/s12906-018-2148-2
- Liu M, Li P, Zeng X, et al. Identification and pharmacokinetics of multiple potential bioactive constituents after oral administration of radix astragali on cyclophosphamide-Induced Immunosuppression in Balb/c Mice. Int J Mol Sci. 2015;16(3):5047–5071. doi:10.3390/ijms16035047
- Chan SL, Ang X, Sani LL, et al. Prevalence and characteristics of adverse drug reactions at admission to hospital: a prospective observational study. Br J Clin Pharmacol. 2016;82(6):1636–1646. doi:10.1111/bcp.13081
- Sun H, Yin M, Qian W, et al. Calycosin a PHYTOESTROGEN ISOflAVONE INDUCES APOPTOSIS OF ESTROGEN RECEPTOR-POSITIve MG-63 Osteosarcoma Cells via the Phosphatidylinositol 3-Kinase (PI3K)/AKT/Mammalian Target of Rapamycin (mTOR) Pathway. Medical Science Monitor. 2018;24(5):6178–6186. doi:10.12659/MSM.910201
- Yang M, Liu B, Jin L, et al. Estrogen receptor β exhibited anti-tumor effects on osteosarcoma cells by regulating integrin, IAP, NF-kB/BCL-2 and PI3K/Akt signal pathway. J Bone Oncol. 2017;9:15–20. doi:10.1016/j.jbo.2017.09.005
- Nichani K, Li J, Suzuki M, et al. Evaluation of caspase-3 activity during apoptosis with fluorescence lifetime-based cytometry measurements and phasor analyses. Cytometry Part A. 2020;97(12):1265–1275. doi:10.1002/cyto.a.24207
- Kamil A, Khan MA, Aasim M, et al. Detection of ROS and translocation of ERP-57 in apoptotic induced human neuroblastoma (SH-SY5Y) cells. BIOCELL. 2019;43(3):167–174.
- Chertkova RV, Brazhe NA, Bryantseva TV, et al. New insight into the mechanism of mitochondrial Cytochrome C function. PLoS One. 2017;12(5):e0178280. doi:10.1371/journal.pone.0178280
- Nie XH, Ouyang J, Xing Y, et al. Calycosin inhibits migration and invasion through modulation of transforming growth factor beta-mediated mesenchymal properties in U87 and U251 cells. Drug Des Devel Ther. 2016;10:767–779.
- Wang Y, Xia CH, Lun ZQ, et al. Crosstalk between p38 MAPK and caspase-9 regulates mitochondria-mediated apoptosis induced by tetra-α-(4-carboxyphenoxy) phthalocyanine zinc photodynamic therapy in LoVo cells. Oncol Rep. 2018;39:61–70. doi:10.3892/or.2017.6071
- Qin X, Yuan LQ, Ruan HX, et al. Interaction of IL-22/IL-22R1 promotes cell proliferation and suppresses apoptosis of colorectal cancer via phosphorylation of STAT3. BIOCELL. 2019;43(2):89–98.
- Gilles C, Marie Odile F. The many roles of ubiquitin in NF-κB signaling. Biomedicines. 2018;6(2):43–89. doi:10.3390/biomedicines6020043
- Ren M, Wang X, Du G, et al. Calycosin-7-O-β-d-glucoside attenuates ischemia-reperfusion injury in vivo via activation of the PI3K/Akt pathway. Mol Med Rep. 2016;13(1):633–640. doi:10.3892/mmr.2015.4611
- Cheng XD, Gu JF, Yuan JR, et al. Suppression of A549 cell proliferation and metastasis by Calycosin via inhibition of the PKC-/ERK1/2 pathway: an in vitro investigation. Mol Med Rep. 2015;12(6):7992–8002. doi:10.3892/mmr.2015.4449
- Xia B, Wang J. Effects of adenosine on apoptosis of ovarian cancer A2780 cells via ROS and caspase pathways. Onco Targets Ther. 2019;12:9473–9480. doi:10.2147/OTT.S216620
- Hiroyasu I, Tatsuya A, Mitsuru S. Inhibition of iNOS activity enhances the anti-tumor effects of alpha-galactosylceramide in established murine cancer model. Oncotarget. 2015;6(39):41863–41874. doi:10.18632/oncotarget.6172
- Sergio DM, Tanea TR, Paola V. et al. Role of ROS and RNS Sources in Physiological and Pathological Conditions. Oxid Med Cell Longev;2016. 1245049–1245093. doi:10.1155/2016/1245049
- Quan GH, Wang H, Cao J, et al. Calycosin Suppresses RANKL-mediated osteoclastogenesis through inhibition of MAPKs and NF-κB. Int J Mol Sci. 2015;16(12):29496–29507. doi:10.3390/ijms161226179
- Zerjatke T, Gak IA, Kirova D, et al. Quantitative cell cycle analysis based on an endogenous all-in-one reporter for cell tracking and classification. Cell Rep. 2017;19(9):1953–1966. doi:10.1016/j.celrep.2017.05.022
- Xie Y, Li F, Li Z, et al. miR-135a suppresses migration of gastric cancer cells by targeting TRAF5-mediated NF-κB activation. Onco Targets Ther. 2019;12:975–984. doi:10.2147/OTT.S189976
- Örd M, Venta R, Möll K, et al. Cyclin-specific docking mechanisms reveal the complexity of M-CDK function in the cell cycle. Mol Cell. 2019;75(1):76–89. doi:10.1016/j.molcel.2019.04.026
- Guo C, Ma Y, Ma S, et al. The role of TRPC6 in the neuroprotection of calycosin against cerebral ischemic injury. Sci Rep. 2017;7(1):3039–3052. doi:10.1038/s41598-017-03404-6
- Liu C, Zheng P, Liu X, et al. Calycosin attenuates TNBS-induced colitis through inibiting inflammatory cytokine and oxidative stress. Int J Clin Exp Med. 2017;10(11):15094–15105.