Abstract
Tissue transglutaminase (tTG) is a rather unique GTP-binding/protein crosslinking enzyme that has been shown to play important roles in a number of cellular processes that impact both normal physiology and disease states. This is especially the case in the context of aggressive brain tumors, such as glioblastoma. The diverse roles played by tTG in cancer survival and progression have led to significant interest in recent years in using tTG as a therapeutic target. In this review, we provide a brief overview of the transglutaminase family, and then discuss the primary biochemical activities exhibited by tTG with an emphasis on the role it plays in glioblastoma progression. Finally, we consider current approaches to target tTG which might eventually have clinical impact.
Graphical Abstract
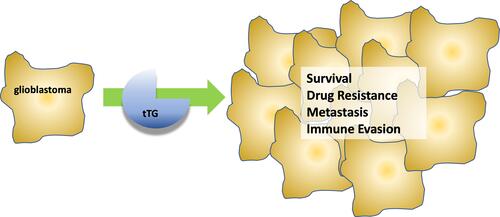
Plain Language Summary
Tissue transglutaminase is an enzyme which is able to crosslink other proteins together. It plays a large number of roles both inside and outside cells, and in the context of cancer aids in cell survival, drug resistance, and metastatic spread. Glioblastoma is a highly deadly malignant brain tumor, and treatment for glioblastoma involves high-risk surgery, radiation treatment, and the use of chemotherapeutic agents. Even with the best available care, survival odds are very low, and there is tremendous unmet need for improved approaches to treating the cancer. High expression of tissue transglutaminase in malignant brain tumors correlates with a poorer prognosis, and inhibition of tissue transglutaminase function in glioblastoma cell lines has frequently resulted in cancer cell death. Because of this, tissue transglutaminase is considered a promising drug target for glioblastoma. Here, we review recent literature describing the biology of tissue transglutaminase, its role in glioblastoma, and various small-molecule inhibitors which have been designed to block its function.
Introduction
Tissue transglutaminase (tTG, TG2, or TGM2, EC 2.3.2.13) is the most-studied member of a family of nine enzymes.Citation1 The enzymes are best known for their ability to crosslink proteins together, by creating a covalent bond between a glutamine and lysine residue. tTG was discovered in 1957, when Heinrich Waelsch detected the calcium-dependent incorporation of radiolabeled amines into proteins in guinea pig liver extracts.Citation2 Since then, the importance of tTG has been demonstrated in several contexts, including Celiac disease, Alzheimer’s disease, and numerous aggressive cancers.Citation3–Citation8 Many of its functions have related to cell survival, heightening interest in the enzyme as a target in various diseases. Because of its prevalence across multiple biological contexts, tTG has been extensively reviewed in recent years.Citation8–Citation12
Among the many cancers tTG plays a role in, one of the most lethal, with a poor patient outlook, is glioblastoma. Glioblastoma is the most aggressive type of malignant brain tumor, and it is particularly difficult to treat due to the high chance of dangerous side effects when applying chemotherapeutics, radiation, or surgery to the brain. Glioblastomas arise from the brain’s glial cells, where tTG is known to play various critical and diverse roles in a cell-specific manner, leading to very complex behaviors by the enzyme in glioblastoma. Here, we therefore maintain a narrow focus describing the roles played by tTG and its clinical outlook in glioblastoma. We briefly examine the basic biology of the transglutaminases, and of glial cells, then investigate the most recent research examining tTG in the context of glioblastoma, where it is an important factor contributing to the aggressiveness of this incurable disease. We then examine the current progress toward making tTG a viable clinical target, and comment on possible future directions in the field.
Biology of Tissue Transglutaminase
The transglutaminases comprise a family of nine proteins, eight of which (TGM1-7 and Factor XIII) have protein crosslinking activity.Citation13 Of these, only tTG is ubiquitously expressed, which helps to explain why it is a recurring factor in several diseases. Each family member is comprised by four domains (a beta-sandwich domain, the catalytic core, and then two beta-barrels, );Citation14,Citation15 however, they show distinct tissue distributions. Moreover, tTG adopts two substantially different conformations: an open-state, in which the domains have a semi-linear arrangement, and a closed-state, in which the two β-barrels fold over onto the catalytic domain ( and ).Citation9 The open-state ( and ) is dominant in the presence of calcium, while the closed-state is favored in the absence of calcium and when in the presence of micromolar concentrations of guanine nucleotides.Citation16–Citation18
Figure 1 Structure of tissue transglutaminase. (A) Crystal structures of tTG in the closed-state conformation (left, PDB ID 1KV3), and the open-state conformation (right, PDB ID 2Q3Z). Boxes show the binding site for nucleotide or crosslinking substrate, respectively. Addition of calcium drives tTG to the open state, while addition of nucleotide stabilizes the closed state. (B) Zoomed in image of the nucleotide binding pocket of closed-state tTG. Shown in black and colored by heteroatom is the nucleotide GTP. The GTP bound state (PDB 4PYG) is colored in blue and the GDP bound state (1KV3) is colored in grey. GDP is omitted from the nucleotide binding site for clarity, since the position of the nucleotide does not change between states. Amino acid side chains that form hydrogen bonds with the nucleotide are labeled and drawn as sticks. (C) Zoomed in image of the peptide binding site of open-state tTG (PDB 2Q3Z). Shown in green is an irreversible gluten peptide mimetic, Ac-P(DON)LPF-NH2. The highly conserved catalytic triad (C277, H335, D358) is labeled and shown as sticks, with the addition of W241 which is also conserved among transglutaminases.
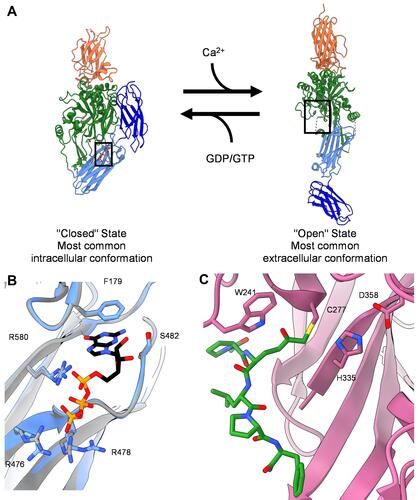
tTG exhibits two functional capabilities – a protein crosslinking activity, and GTP/ATP-binding and hydrolytic activity.Citation8,Citation9 Protein crosslinking occurs when tTG adopts the open-state, which exposes the substrate binding site (). The protein crosslinking active sites for each member of the transglutaminase family are highly conserved. Catalysis is mediated by a triad of residues, namely Cysteine 277, Histidine 335, and Aspartic acid 358 in tTG, with Tryptophan 241 also being highly conserved and helping to stabilize the transition state for crosslinking activity.Citation1 The protein crosslinking-defective family member, Protein Band 4.2, has a Cysteine-to-Alanine mutation rendering it catalytically incompetent.Citation13,Citation19 Protein crosslinking occurs via transamidation, in which the γ-carboxamide group of a glutamine residue is reacted with the ε-amino group of a lysine residue, forming a new amide bond and releasing ammonia. However, tTG can utilize other substrates, including amines such as cadaverine, and water, to catalyze several different reactions.
No strong consensus sequence has been identified for the glutamine-bearing proteins crosslinked by tTG (). However, its catalytic activity does not appear to be entirely indiscriminate,Citation20,Citation21 suggesting consensus/recognition potential for crosslinking substrates at some other region on the protein. Since excessive protein crosslinking could be cytotoxic,Citation9 this activity is probably tightly regulated in normal biological contexts.
Figure 2 Consensus sequence for tTG crosslinking substrates. Alignment of all glutamine-donor crosslinking substrates described in the Transdab database demonstrates that other than glutamine (Q at position 0), there is little to no favoritism for different residues at up to five positions before or after the glutamine residue to be crosslinked. Figure generated with Seq2Logo 2.0.
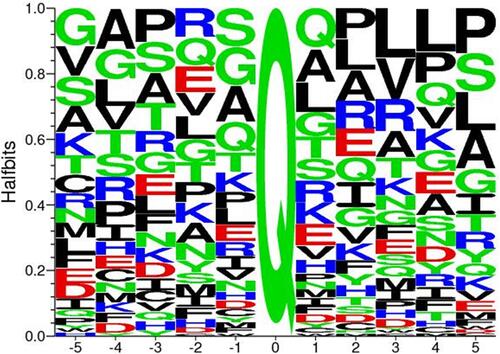
In the closed-state, the protein crosslinking active site of tTG is not accessible,Citation14 but the guanosine nucleotide binding pocket of tTG is revealed (). Guanine nucleotides compete with calcium to promote the closed-state conformation.Citation9,Citation21 Since tTG has GTP hydrolytic activity, some early reports suggested that tTG might function as a molecular switch similar to G-proteins acting in signal transduction pathways, although no such signaling functions have as yet been established.Citation22–Citation24 Furthermore, structural studies have failed to reveal differences between the GTP- and GDP-bound forms of tTG, so it is not clear how such signaling function might be regulated.Citation14,Citation25
Tissue Transglutaminase in Glial Cell Biology
The brain is comprised primarily of two types of cells: neurons and glial cells. Neurons, the nerve cells of the brain, generate electrical impulses to transmit information between one another and are responsible for higher order brain function. Glial cells (from which glioblastomas arise) do not produce electrical impulses, although they do communicate via other means, and support neuronal function in a variety of ways.Citation26 Glial cells are genetically diverse, and various types of glial cells exist.Citation27 These include:
Astrocytes, star-shaped cells that perform several functions.Citation28 They contribute to the blood-brain barrier, which regulates chemical access to the brain.Citation29 They help to regulate the microenvironment surrounding neurons, by adjusting ion concentration, recycling neurotransmitters, and releasing signaling molecules. Although largely nonreactive, upon injury to the central nervous system, astrocytes respond with significant changes to their morphology and function to effect repair.Citation30
Ependymal cells, thin epithelial cells that produce and help circulate cerebrospinal fluid. They also activate following brain injury, and upon activation differentiate into astrocytes, thus bearing some characteristics of stem cells.Citation31
Oligodendrocytes, large cells that act as scaffolding for neurons.Citation28 Via the process of myelination, oligodendrocyte projections wrap around the axon of neurons and insulate them from the local environment, while also securing them in place. While coating neurons, oligodendrocytes also provide various growth factors and metabolites,Citation32 thus supporting neuronal survival and growth.
Microglia, smaller, specialized immune cells that constantly move throughout the brain and communicate with other glial cells while searching for potential defects in brain function.Citation33 They use specialized potassium channels to work with astrocytes to regulate potassium levels in the extracellular environment. Microglia actively scavenge for debris throughout the brain and act in effect as a “cleanup crew”. To compensate for the inability of antibodies to pass the blood-brain barrier, microglia can act as antigen-presenting cells, priming T-cells in the brain for immunological function.
The myriad roles of tTG in each of these cell types are beyond the scope of this review, but have been outlined in detail in a recent review from Rudlong et al.Citation34 Here we will thus highlight just a few to provide examples of the diverse functions of tTG in glial cells.
It is widely accepted that tTG plays important roles in cell survival,Citation8 and this is case for many glial cells.Citation35 However, in astrocytes following ischemic injury, tTG instead promotes cell death, whereas the depletion of tTG results in greater cell survival.Citation36,Citation37 Extracellular tTG released by microglia bind to the oligodendrocyte G-protein-coupled receptor ADGRG1 (also known as GPR56) to drive myelination, as demonstrated in a mouse model of demyelination.Citation35 However, in astrocytes, tTG contributes to fibronectin deposition during the process of demyelination.Citation37 Further, in various glial cells tTG contributes to differentiation (in oligodendrocytes)Citation35,Citation38 and migration (in astrocytes).Citation39 The diverse roles played by tTG help explain how it can be co-opted to support key malignant phenotypes in glioblastomas.
Roles of Tissue Transglutaminase in Glioblastoma
Gliomas are the most common form of primary brain tumors. The World Health Organization has recently updated classification of gliomas into four distinct categories that reflect the age of the patient and the aggressiveness of the tumor.Citation40,Citation41 This includes adult-type diffuse gliomas (including glioblastoma), pediatric-type diffuse low-grade gliomas, pediatric-type diffuse high-grade gliomas, and circumscribed astrocytic gliomas. Here, we will focus on the behavior of tTG in glioblastoma. Prognosis for glioblastoma is poor, with the median survival following treatment being under two years. Despite the investments in the study of glioblastoma, standard-of-care treatment remains aggressive and toxic. It typically involves maximal safe surgical removal of the tumor, followed by treatment with radiation and temozolomide (an alkylating chemotherapeutic).Citation42 Newer therapies, such as immune checkpoint inhibitors (ICI) have shown some limited promise in preliminary studies, however the identification of subgroups of patients responding to ICIs and predictive factors for ICI success are necessary to achieve superior outcomes.Citation43 Thus, glioblastoma remains largely incurable, and significant unmet need remains among patients. tTG has attracted attention as a protein that contributes to numerous phenotypes supporting glioblastoma aggressiveness, and thus a potential therapeutic target.
The roles played by tTG in tumorigenesis in most cancers will depend upon its ability to adopt the conformational states described above (). Intracellular tTG is exposed to low levels of calcium, but high levels of nucleotide, so it assumes a GTP-bound “closed” conformation that, in some contexts, protects epidermal growth factor (EGF) receptors from c-Cbl catalyzed receptor ubiquitylation and degradation, and helps assemble a signaling complex between PI-3K and c-Src, resulting in their activation.Citation44–Citation46 When secreted from cells, tTG is exposed to extracellular calcium, and adopts the open-state conformation, which can associate with microvesicles (MVs), a class of extracellular vesicles that form on the surfaces of aggressive cancer cells and support tumor progression.Citation47–Citation49 These two distinct conformations, and the dynamics between intracellular and extracellular tTG, likely account for the majority of roles played by tTG in cancer progression. Now, we consider specific roles tTG plays in glioblastoma.
Survival
tTG frequently promotes the survival of healthy cells, and it serves a similar role in glioblastoma, where inhibition or deletion of tTG causes cell death in U87MG and LN229 glioblastoma cells and reduces their ability to survive stresses.Citation44 However, tTG expression is relatively low in healthy brain tissue as well as in most low-grade gliomas, despite being overexpressed in high-grade tumors.Citation44 The gene for tTG, TGM2, is hypermethylated at the 5’ flanking region in lower-grade gliomas, resulting in reduced tTG expression.Citation50 Treatment of U87MG, U118MG, T98G, or A172 glioblastoma cells featuring TGM2 hypermethylation with the demethylating agent 5-aza-2’-deoxycytidine restored tTG expression, but had no discernible impact upon cell viability. This suggests that higher grade gliomas may be under stresses that require tTG for survival, while lower grade gliomas reduce its expression to support other phenotypes.
The function of tTG to promote cancer cell survival can be broadly split into two categories: evasion of cell death and promotion of cell growth. Upregulation of tTG expression and activity by retinoic acid offers strong protection against artificially induced apoptosis.Citation51 Similarly, Yuan and coworkers demonstrated that inhibition of tTG with peptidomimetic inhibitors promoted proapoptotic changes in glioblastoma cells. These included decreased phosphorylation of Akt and the associated reduction in the expression of survivin, phospho-GSK-3β, and phospho-Bad, and increased expression of the proapoptotic protein Bim. Thus, tTG plays an important role in the ability of glioma cancer cells to evade apoptosis.Citation52 Relatedly, Gundemir and coworkers found that inhibition of tTG activity did little to block growth factor signaling, but had a strong impact on pathways downstream of NfκB, which plays a key role regulating proliferation in various contexts.Citation53
Drug and Radiation Resistance
Many aggressive brain tumors display the ability to resist therapeutic intervention, including chemotherapy, and tTG has been strongly implicated in the conferral of chemoresistance in cancer.Citation5 However, tTG does not render cells resistant to all chemotherapeutic agents. In the U118MG glioblastoma cell line, expression of tTG induces resistance to the DNA-damaging drugs doxorubicin and CCNU, but not to vincristine, temozolomide, cisplatin, or cyclophosphamide.Citation50 Similarly, treatment of murine DBT glioblastoma xenografts with tTG inhibitors alongside the chemotherapeutic carmustine is much more effective than treatment with carmustine alone, suggesting that carmustine sensitivity is at least partially modulated by tTG.Citation52 tTG-mediated chemoresistance extends to glioma stem cells (GSCs) as well. Cancer stem cells are thought to be one of the most important factors causing the cancer to evade treatment. In gliomas, mesenchymal GSCs tend to be highly chemoresistant, and to be associated with the most aggressive glioblastomas. Sullivan et al observed that tTG was preferentially overexpressed in this GSC subtype through a process mediated by aldehyde dehydrogenase 1A3 (ALDH1A3) and its product retinoic acid.Citation54 Inhibition of tTG with the peptidomimetic Z-Don induced sensitivity to temozolomide, strongly suggesting that tTG provides different types of chemoprotection in different cell types.
In addition to conferring resistance to chemotherapeutic treatment, tTG can also bestow glioblastomas with radiation resistance. Daniel and coworkers demonstrated that tTG enhances radio-resistance in glioblastoma both in vitro and in vivo by repressing the expression of the DNA damage repair protein RAD51.Citation55 They and others have also noted that radiation treatment of glioblastomas frequently resulted in upregulation of tTG.Citation55,Citation56 Interestingly, Berg demonstrated that irradiation of healthy astrocytes caused tTG to be secreted into the extracellular matrix, which in turn generated a supportive microenvironment for implanted glioma cells, leading to more rapid tumor growth in mouse models. This modified extracellular matrix supported stemness and radio-resistance. This supported earlier work by Sullivan, which demonstrated that tTG inhibition restored radiation sensitivity to mesenchymal GSCs.Citation54 It similarly corroborated work by Yin showing that tTG was overexpressed in the perinecrotic environment surrounding glioblastomas,Citation57 where it assists in trans-differentiation of GSCs.Citation58 Typically, GSCs differentiate to the radiation-resistant mesenchymal subtype following radiation treatment. Knockdown or inhibition of tTG in a glioblastoma mouse model suppressed trans-differentiation of GSCs to the aggressive mesenchymal state. In total, these studies demonstrate that tTG confers radio-resistance both directly through expression in cells, and indirectly via its actions in the tumor microenvironment. These effects are likely driven by tTG crosslinking activity, which is driven by tTG adopting the open-state conformation in response to relatively high levels of calcium ions in the extracellular space.
Tumor Progression and Metastatic Spread
Another phenotype supported by tTG is tumor cell migration and invasion. When examining the differences between highly motile U87MG and largely immobile U343MG-A cells, tTG was one of just five proteins found to be significantly more highly expressed in U87MG cells.Citation59 One possible explanation for this involves the interplay between tTG and the EGF receptor. Overexpression and hyperactivation of the EGF receptor has been demonstrated to be a hallmark of glioblastoma, and to correlate with tumor aggressiveness and invasive potential.Citation60,Citation61 In U87MG and LN229 glioblastoma cells, tTG sequesters the E3 ubiquitin ligase c-Cbl, thus blocking the ubiquitination of the EGF receptor and preventing its degradation ().Citation44 Depleting these glioblastoma cell lines of tTG using siRNA results in a decrease in the expression of the EGF receptor. Moreover, ectopic expression of tTG in T98G glioblastoma cells, which express very low endogenous levels of tTG, resulted in a marked increase in EGF receptor stability. Interestingly, mutations in tTG that blocked nucleotide binding (ie R580K) blocked tTG binding to c-Cbl. Based on previous SAXS analysis, nucleotide binding defective mutants of tTG adopt the open-state conformation and ectopic expression of these mutants results in potent cell death, suggesting that the open-state conformation of tTG is cytotoxic to glioblastoma cell lines.
Figure 3 Mechanisms by which tissue transglutaminase impacts glioblastoma aggressiveness. (A) Without tTG, in many glioblastoma cells c-Cbl ubiquitinates the EGFR, priming it for degradation. When tTG is present, it sequesters the c-Cbl, preventing ubiquitination and effectively stabilizing the growth receptor, functionally increasing its expression. (B) Extracellular tTG is able to crosslink fibronectin displayed on the outside of microvesicles (MVs) to integrins expressed on cell surfaces. This allows the MVs to dock to the cells, and release their contents.
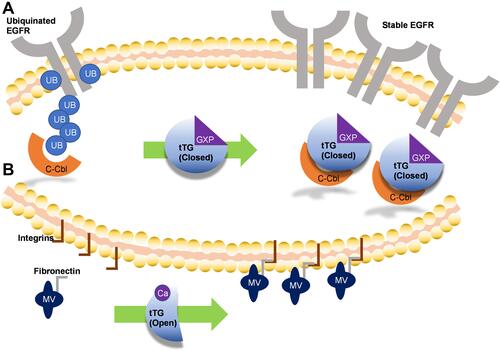
tTG also assists in the spread of cancer cells through its presence in the tumor microenvironment. The involvement of extracellular tTG in cell attachment and motility has been widely appreciated.Citation8,Citation12 This occurs through the ability of tTG to crosslink extracellular matrix components including collagen and fibronectin. These changes have been shown to change the stiffness of the extracellular matrix and promote tumor cell growth and migration. Notably, however, tTG also plays important roles in the actions of microvesicles, which are shed from various cancer cells and assist in stimulating tumor angiogenesis, metastasis, and other malignant phenotypes.Citation62 For example, treatment of noncancerous NIH-3T3 fibroblasts with MVs derived from U87MG glioblastoma cells confers cancer-like effects on the recipient cells.Citation62 This effect was driven by tTG-catalyzed crosslinking of fibronectin along the surfaces of MVs to cell-based integrins (). These events take place in the extracellular space, where tTG adopts primarily the open-state conformation. In the same study, MVs shed by aggressive breast cancer cells were also shown to confer transformed phenotypes to fibroblasts, and to support tumor formation in mice.Citation62
Drugs Targeting tTG
There has been substantial research focusing on the development of tTG inhibitors,Citation63,Citation64 and well over a dozen different scaffolds have been described at this point. Here, we discuss representative highlights from various inhibitor classes. Transglutaminase inhibitors predominantly target the protein crosslinking active site and include alternative substrates, peptidic compounds, and non-peptidic compounds (). The earliest inhibitors fell into the first class. Competitive amine inhibitors such as monodansyl cadaverineCitation65 and cystamineCitation66 reduce access to the crosslinking active site by amine-donor substrates. They in turn are crosslinked to glutamine-donor substrates, thus reducing the rate of crosslinking activity directed toward the normal cellular substrates. These compounds have shown promise in protection against insoluble aggregate formation and oxidative stress.Citation67–Citation69 However, these inhibitors are nonspecific, limiting their usefulness as therapeutic reagents targeting tTG.
Figure 4 Inhibitors of tissue transglutaminase. Tissue transglutaminase inhibitors can be broadly classified as (A) alternate substrates, an older class of inhibitor which takes the place of a natural substrate of tTG, thus blocking biologically relevant crosslinking reactions. (B) Irreversible substrate competitive inhibitors, which bind to the crosslinking substrate site and covalently attach to tTG, blocking crosslinking. These tend to be derived from peptide structures. (C) Reversible substrate competitive inhibitors. These molecules bind to the substrate crosslinking site of tTG, but do not modify the enzyme. (D) Inhibitors which bind to the nucleotide binding pocket. Relatively few inhibitors utilize this strategy, but they stabilize the closed-state of tTG, thus blocking crosslinking activity.
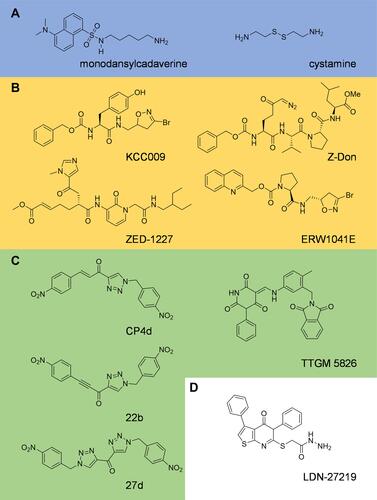
Peptidic inhibitors offer significantly improved selectivity and potency, but generally at the expense of cell permeability. One such drug, KCC009,Citation70,Citation71 inhibits tTG-mediated fibronectin remodeling, and when combined with the chemotherapy drug carmustine, an increased inhibitory effect was achieved in DBT glioblastoma xenografts in vivo.Citation71 Another commonly used inhibitor, Z-Don, is moderately cell permeable. Z-don has been used to block glioblastoma cell migration in wound-healing assays,Citation72 and to help demonstrate that when neurons are exposed to organophosphates, they covalently bind to and activate tTG, causing neurotoxic effects.Citation73 Arguably the most important inhibitor in this class is ZED-1227, the only tTG inhibitor to have been entered into clinical trials.Citation74 ZED-1227 is cell-impermeable however, having been designed to target extracellular tTG in Celiac disease, so it would be unable to inhibit intracellular tTG functions in glioblastoma.
In recent years, several studies have reported non-peptidic inhibitors of tTG. Some, such as ERW1041E, are still semi-peptidic. ERW1041E contains a proline residue in its core and has been demonstrated to inhibit tTG with a minimum inhibitory concentration of 6–12 µM in vitro, and to be well tolerated in mouse models of Celiac disease at concentrations as high as 50 mg/kg.Citation75 Another commonly used compound is CP4d,Citation76 which is a substrate competitive inhibitor with a potency of ~1 µM; however, it is also highly susceptible to nucleophilic attack by glutathione at the electrophilic alkene. Apperley and colleagues attempted to rectify this problem, generating two new molecules – 22b and 27d. Unfortunately, potency and metabolic stability did not go hand in hand. While 22b improved upon the potency of CP4d by ~2 fold, only 27d significantly reduced the rate of attack from glutathione, losing an order of magnitude in potency at the same time.Citation77
One particularly interesting compound is LDN-27219. While most tTG inhibitors are designed to bind to the protein crosslinking active site, LDN-27219 instead binds to the nucleotide binding pocket of the enzyme while it is in its closed-state conformation, and stabilizes that conformation to prevent crosslinking activity.Citation78 Schneider et al utilized this compound when demonstrating that tTG mediates an increase in PD-L1 expression by crosslinking serotonin taken up from the microenvironment to various small G-proteins at their glutamine residues essential for GTP hydrolysis, thus activating their signaling pathways.Citation79 PD-L1 expression is a key mechanism by which tumor cells evade the immune response. Similarly, Pinilla and coworkers demonstrated that stabilizing the closed-state conformation of tTG with LDN-27219 was more effective than peptidomimetic inhibition of catalytic activity with Z-Don in reducing blood pressure in Wistar Hannover rats.Citation80 Taking an opposite approach, TTGM-5826 was explicitly designed to stabilize the open-state conformation of tTG and to take advantage of the findings that maintaining the open-state conformation of tTG, which can be significant in aggressive cancer cells that highly express tTG, confers cytotoxicity.Citation9,Citation81,Citation82 TTGM 5826 was shown to stabilize the tTG open-state conformation through various biochemical assays, and to inhibit the growth and migration of U87 and LN229 glioblastoma cells in vitro.Citation72 It also exhibited a degree of selectivity between transformed and healthy cells, further raising the hope that tTG inhibitors might eventually become part of a low toxicity anti-cancer treatment regimen.
Clinical Outlook
Given the demonstrations of tTG as an important factor in glioblastoma oncogenesis and disease progression, and the diverse chemical scaffolds that have been reported to inhibit tTG activity, it is perhaps surprising that so little has been done to bring a tTG inhibitor to the clinic. Clinicaltrials.gov is the world’s largest database of current and past clinical trials. Currently, clinicaltrials.gov lists 68 studies featuring the keyword “transglutaminase”. Of these, only two pertain to cancer, and both use tTG exclusively as a biomarker to assess the effectiveness of the treatments being examined.Citation83,Citation84 Over 40 of the entries, by comparison, are trials looking at Celiac disease, where tTG is also a major causative factor.Citation85 Most of these trials are examining the use of tTG antibodies as therapeutic agents, or exploring tTG as a diagnostic marker, or other applications not especially relevant to treatment of glioblastoma.
However, there is at least one diamond in the rough, so to speak. A trial of the peptidomimetic tTG inhibitor ZED-1227, in which three different dose points (10, 25, or 100 mg of compound daily for 6 weeks) were tested, showed the compound to have no acute toxic effects, and to have a statistically significant impact in preventing gluten-induced mucosal damage due to Celiac disease.Citation74 While peptidomimetic compounds are typically at best only minimally cell permeable, limiting the applicability of this specific inhibitor to glioblastomas, it does raise hopes for small-molecule tTG therapeutics coming to the clinic.
Opinions on the Future of Tissue Transglutaminase in Glioblastoma
Translating tTG inhibitors from the bench to the bedside requires, in our opinion, three steps to occur. These are 1) a more complete understanding of the role of tTG in glioblastoma specifically, 2) the development of enhanced potency and improved pharmacological characteristics for clinically relevant tTG inhibitors, and 3) identification of an ideal treatment approach. The first issue represents perhaps the most difficult challenge to address. tTG is a comparative rarity among enzymes in that it that exhibits a wide range of functional effects. In addition, the specific processes it contributes to vary by context, in both disease and in healthy tissue, thus making it very difficult to predict a priori how tTG will function in a given cell system and/or under conditions of different types of cellular stress, until it has been directly examined in those situations. For example, tTG has been shown to play important roles in models of pancreatic cancer, ovarian cancer, cervical cancer, and breast cancer.Citation6,Citation45,Citation86–Citation88 Many of the effects observed in these cancer models have not been conclusively demonstrated in glioblastomas; given the discrepancies between tTG function between even glioblastoma and lower-grade gliomas, it is unlikely that all effects will be reproduced in glioblastoma. However, a fuller understanding of which effects are conserved between model systems could potentially help to direct tTG-based treatment approaches. It will thus be important to reproduce findings that have been reported for a variety of cancers in malignant brain tumors before trusting that tTG will perform any given function in glioblastoma.
The second challenge is that of inhibitor design. Current tTG inhibitors frequently have comparatively low potency, and/or relatively poor cell permeability. The most potent drugs are generally the least cell permeable, which is acceptable for treating extracellular tTG in eg Celiac disease, but greatly limits applicability to glioblastoma. Drug optimization is substantially easier when a structure of the drug bound to its target exists. However, tTG is notoriously difficult to crystallize, and only nine different structures exist, only half of which have a bound drug, despite the wide variety of drug scaffolds that have been reported. We suspect that this will be partially resolved via newer methods. One potential option is cryogenic electron microscopy (cryoEM). The explosion of cryoEM as a method for high resolution structural analysis has resulted in numerous protein-protein complex structures that were previously too challenging to interrogate with X-ray crystallography. CryoEM does not require crystallization of the protein, so could possibly overcome the current challenges associated with crystallizing tTG. This also opens the exciting possibility to investigate tTG complexes that could play important roles in cancer progression. For example, formation of the tTG-Src complex suggests that it plays a key role in the activation of the Src tyrosine kinase and subsequent cancer cell growth.Citation89 Additionally, the interaction of tTG with the extracellular N-terminus of the G-protein coupled receptor ADGRG1 has been suggested to prevent metastasis in melanomas.Citation90 ADGRG1 is often overexpressed in aggressive brain tumors and has been inversely correlated with patient survival.Citation91 Structural characterization of these complexes would yield important mechanistic details into the role tTG plays in cancer progression and open the door to the development of drugs targeting complex formation.
Finally, any treatment involving a tTG inhibitor will likely be part of a combination treatment. While tTG is strongly implicated in drug resistance in various tumor models, and thus there are many candidate drugs with which it might be dosed, in our opinion the ICIs offer a particularly attractive partner. ICIs have shown substantial promise in some indications, but are not entirely effective as single-agent treatments in glioblastoma.Citation92 Meanwhile, tTG has been implicated in immune evasion, both directlyCitation80 and via its effects in MVs,Citation62 which are a key component of the tumor microenvironment that assist tumors in evading immune responses.Citation93,Citation94 Thus, inhibiting tTG could help to restore a normal immune response, even while checkpoint blockade inhibitors such as pembrolizumabCitation95 act to hyper-activate T cells.
Conclusions
Tissue transglutaminase is a multi-functional GTP-binding/protein crosslinking enzyme which has been shown to contribute to a number of different stages of cancer progression. Thus, given what has been observed in a variety of types of cancer, it is perhaps unsurprising that tTG influences the survival, growth, and metastatic spread of malignant brain tumors. These effects are observed through aberrant signaling in numerous pathways including Akt and NfκB to promote glioblastoma cell survival, acquired resistance to treatment with chemotherapeutics and radiation, and disruption of the machinery necessary for EGFR degradation resulting in aggressive, uncontrolled growth. tTG expression is correlated with a poor prognosis for patients with glioblastoma, and ongoing research has developed over a dozen different drug scaffolds which inhibit the enzyme in vitro and in vivo. However, no tTG inhibitor has yet entered clinical trials for any cancer indication; thus, more research is needed for tTG inhibitors to be used in such applications.
Abbreviations
tTG, tissue transglutaminase; EGF, epidermal growth factor; VEGF, vascular endothelial growth factor.
Author Contributions
All authors made a significant contribution to the work reported, whether that is in the conception, study design, execution, acquisition of data, analysis and interpretation, or in all these areas; took part in drafting, revising or critically reviewing the article; gave final approval of the version to be published; have agreed on the journal to which the article has been submitted; and agree to be accountable for all aspects of the work.
Disclosure
The authors declare no competing interests which would influence this work.
Additional information
Funding
References
- Iismaa SE, Holman S, Wouters MA, Lorand L, Graham RM, Husain A. Evolutionary specialization of a tryptophan indole group for transition-state stabilization by eukaryotic transglutaminases. Proc Natl Acad Sci U S A. 2003;100(22):12636–12641. doi:10.1073/pnas.1635052100
- Sarkar NK, Clarke DD, Waelsch H. An enzymically catalyzed incorporation of amines into proteins. Biochim Biophys Acta. 1957;25:451–452. doi:10.1016/0006-3002(57)90512-7
- Anderson RP, Degano P, Godkin AJ, Jewell DP, Hill AVS. In vivo antigen challenge in celiac disease identifies a single transglutaminase-modified peptide as the dominant A-gliadin T-cell epitope. Nat Med. 2000;6(3):337–342. doi:10.1038/73200
- Martin A, de Vivo G, Gentile V. Possible role of the transglutaminases in the pathogenesis of Alzheimer’s disease and other neurodegenerative diseases. J Alzheimer’s Dis. 2011;2011:865432.
- Budillon A, Carbone C, Di Gennaro E. Tissue transglutaminase: a new target to reverse cancer drug resistance. Amino Acids. 2013;44(1):63–72. doi:10.1007/s00726-011-1167-9
- Lee J, Condello S, Yakubov B, et al. Tissue transglutaminase mediated tumor–stroma interaction promotes pancreatic cancer progression. Clin Cancer Res. 2015;21(19):4482–4493. doi:10.1158/1078-0432.CCR-15-0226
- Li B, Cerione RA, Antonyak M. Tissue transglutaminase and its role in human cancer progression. Adv Enzymol Relat Areas Mol Biol. 2011;78:247–293.
- Katt WP, Antonyak MA, Cerione RA. The diamond anniversary of tissue transglutaminase: a protein of many talents. Drug Discov. 2018;23(3):575–591.
- Katt WP, Antonyak MA, Cerione RA. Opening up about Tissue Transglutaminase: when Conformation Matters More than Enzymatic Activity. Med One. 2018;3(6):e180011. doi:10.20900/mo.20180011
- Keillor JW, Apperley KYP. Transglutaminase inhibitors: a patent review. Expert Opin Ther Pat. 2016;26(1):49–63. doi:10.1517/13543776.2016.1115836
- Nurminskaya MV, Belkin AM. Cellular functions of tissue transglutaminase. Int Rev Cell Mol Biol. 2012;294:1–97. doi:10.1016/B978-0-12-394305-7.00001-X
- Gundemir S, Colak G, Tucholski J, Johnson GV. Transglutaminase 2: a molecular Swiss army knife. Biochim Biophys Acta. 2011;1823(2):406–419. doi:10.1016/j.bbamcr.2011.09.012
- Grenard P, Bates MK, Aeschlimann D. Evolution of transglutaminase genes: identification of a transglutaminase gene cluster on human chromosome 15q15: structure of the gene encoding transglutaminase X and a novel gene family member, transglutaminase Z*. J Biol Chem. 2001;276(35):33066–33078. doi:10.1074/jbc.M102553200
- Liu S, Cerione RA, Clardy J. Structural basis for the guanine nucleotide-binding activity of tissue transglutaminase and its regulation of transamidation activity. Proc Natl Acad Sci U S A. 2002;99(5):2743–2747. doi:10.1073/pnas.042454899
- Pinkas DM, Strop P, Brunger AT, Khosla C. Transglutaminase 2 undergoes a large conformational change upon activation. PLoS Biol. 2007;5(12):e327. doi:10.1371/journal.pbio.0050327
- Achyuthan KE, Greenberg CS. Identification of a guanosine triphosphate-binding site on Guinea pig liver transglutaminase. Role of GTP and calcium ions in modulating activity. J Biol Chem. 1987;262(4):1901–1906. doi:10.1016/S0021-9258(19)75724-X
- Casadio R, Polverini E, Mariani P, et al. The structural basis for the regulation of tissue transglutaminase by calcium ions. Eur. J. Biochem. 1999;259:262. doi:10.1046/j.1432-1327.1999.00031.x
- Zhang JW, Lesort M, Guttmann RP, Johnson GVW. Modulation of the in situ activity of tissue transglutaminase by calcium and GTP. J Biol Chem. 1998;273(4):2288–2295. doi:10.1074/jbc.273.4.2288
- Eckert RL, Kaartinen MT, Nurminskaya M, et al. Transglutaminase regulation of cell function. Physiol Rev. 2014;94(2):383–417. doi:10.1152/physrev.00019.2013
- Csősz É, Meskó B, Fésüs L. Transdab wiki: the interactive transglutaminase substrate database on web 2.0 surface. Amino Acids. 2009;36(4):615–617. doi:10.1007/s00726-008-0121-y
- Kiraly R, Demeny M, Fesus L. Protein transamidation by transglutaminase 2 in cells: a disputed Ca2 + −dependent action of a multifunctional protein. FEBS J. 2011;1:278.
- Nakaoka H, Perez DM, Baek KJ, et al. Gh: a GTP-binding protein with transglutaminase activity and receptor signaling function. Science. 1994;264(5165):1593–1596. doi:10.1126/science.7911253
- Murthy SNP, Lomasney JW, Mak EC, Lorand L. Interactions of Gh/transglutaminase with phospholipase Cδ1 and with GTP. Proc Natl Acad Sci U S A. 1999;96(21):11815. doi:10.1073/pnas.96.21.11815
- Mhaouty-Kodja S. Ghα/tissue transglutaminase 2: an emerging G protein in signal transduction. Biol Cell. 2004;96(5):363–367. doi:10.1111/j.1768-322X.2004.tb01427.x
- Jang TH, Lee DS, Choi K, et al. Crystal structure of transglutaminase 2 with GTP complex and amino acid sequence evidence of evolution of GTP binding site. PLoS One. 2014;9(9):e107005. doi:10.1371/journal.pone.0107005
- Fields RD, Araque A, Johansen-Berg H, et al. Glial biology in learning and cognition. Neuroscientist. 2013;20(5):426–431. doi:10.1177/1073858413504465
- von Bartheld CS. Myths and truths about the cellular composition of the human brain: a review of influential concepts. J Chem Neuroanat. 2018;93:2–15. doi:10.1016/j.jchemneu.2017.08.004
- Freeman MR, Rowitch DH. Evolving concepts of gliogenesis: a look way back and ahead to the next 25 years. Neuron. 2013;80(3):613–623. doi:10.1016/j.neuron.2013.10.034
- Rubin LL, Staddon JM. The cell biology of the blood-brain barrier. Annu Rev Neurosci. 1999;22(1):11–28. doi:10.1146/annurev.neuro.22.1.11
- Hauwel M, Furon E, Canova C, Griffiths M, Neal J, Gasque P. Innate (inherent) control of brain infection, brain inflammation and brain repair: the role of microglia, astrocytes, “protective” glial stem cells and stromal ependymal cells. Brain Res Rev. 2005;48(2):220–233. doi:10.1016/j.brainresrev.2004.12.012
- Johansson CB, Momma S, Clarke DL, Risling M, Lendahl U, Frisén J. Identification of a neural stem cell in the adult mammalian central nervous system. Cell. 1999;96(1):25–34. doi:10.1016/S0092-8674(00)80956-3
- Philips T, Rothstein JD. Oligodendroglia: metabolic supporters of neurons. J Clin Investig. 2017;127(9):3271–3280. doi:10.1172/JCI90610
- Guillemin GJ, Brew BJ. Microglia, macrophages, perivascular macrophages, and pericytes: a review of function and identification. J Leukoc Biol. 2004;75(3):388–397. doi:10.1189/jlb.0303114
- Rudlong J, Cheng A, Johnson GVW. The role of transglutaminase 2 in mediating glial cell function and pathophysiology in the central nervous system. Anal Biochem. 2020;591:113556. doi:10.1016/j.ab.2019.113556
- Giera S, Luo R, Ying Y, et al. Microglial transglutaminase-2 drives myelination and myelin repair via GPR56/ADGRG1 in oligodendrocyte precursor cells. eLife. 2018;7:e33385. doi:10.7554/eLife.33385
- Monteagudo A, Feola J, Natola H, Ji C, Pröschel C, Johnson GVW. Depletion of astrocytic transglutaminase 2 improves injury outcomes. Mol Cell Neurosci. 2018;92:128–136. doi:10.1016/j.mcn.2018.06.007
- Elahi A, Emerson J, Rudlong J, et al. Deletion or inhibition of astrocytic transglutaminase 2 promotes functional recovery after spinal cord injury. bioRxiv. 2021;15:460455.
- Espitia Pinzon N, van Mierlo H, de Jonge JC, et al. Tissue transglutaminase promotes early differentiation of oligodendrocyte progenitor cells. Front Cell Neurosci. 2019;13:281. doi:10.3389/fncel.2019.00281
- Monteagudo A, Ji C, Akbar A, Keillor JW, Johnson GVW. Inhibition or ablation of transglutaminase 2 impairs astrocyte migration. Biochem Biophys Res Commun. 2017;482(4):942–947. doi:10.1016/j.bbrc.2016.11.137
- Omuro A, DeAngelis LM. Glioblastoma and other malignant gliomas: a clinical review. JAMA. 2013;310(17):1842–1850. doi:10.1001/jama.2013.280319
- Louis DN, Perry A, Wesseling P, et al. The 2021 WHO classification of tumors of the central nervous system: a summary. Neuro-Oncology. 2021;23(8):1231–1251. doi:10.1093/neuonc/noab106
- Tan AC, Ashley DM, López GY, Malinzak M, Friedman HS, Khasraw M. Management of glioblastoma: state of the art and future directions. CA Cancer J Clin. 2020;70(4):299–312. doi:10.3322/caac.21613
- Medikonda R, Dunn G, Rahman M, Fecci P, Lim M. A review of glioblastoma immunotherapy. J Neurooncol. 2021;151(1):41–53. doi:10.1007/s11060-020-03448-1
- Zhang J, Antonyak MA, Singh G, Cerione RA. A mechanism for the upregulation of EGF receptor levels in glioblastomas. Cell Rep. 2013;3(6):2008–2020. doi:10.1016/j.celrep.2013.05.021
- Boroughs LK, Antonyak MA, Cerione RA. A novel mechanism by which tissue transglutaminase activates signaling events that promote cell survival. J Biol Chem. 2014;289(14):10115–10125. doi:10.1074/jbc.M113.464693
- Singh G, Zhang J, Ma Y, Cerione RA, Antonyak MA. The different conformational states of tissue transglutaminase have opposing effects on cell viability. J Biol Chem. 2016;291(17):9119–9132. doi:10.1074/jbc.M115.699108
- Raposo G, Stoorvogel W. Extracellular vesicles: exosomes, microvesicles, and friends. J Cell Biol. 2013;200(4):373–383. doi:10.1083/jcb.201211138
- Antonyak MA, Wilson KF, Cerione RA. R(h)oads to microvesicles. Small GTPases. 2013;3(4):219–224. doi:10.4161/sgtp.20755
- Muralidharan-Chari V, Clancy JW, Sedgwick A, D’Souza-Schorey C. Microvesicles: mediators of extracellular communication during cancer progression. J Cell Sci. 2010;123(10):1603–1611. doi:10.1242/jcs.064386
- Dyer LM, Schooler KP, Ai L, et al. The transglutaminase 2 gene is aberrantly hypermethylated in glioma. J Neurooncol. 2011;101(3):429–440. doi:10.1007/s11060-010-0277-7
- Antonyak MA, Singh US, Lee DA, et al. Effects of tissue transglutaminase on retinoic acid-induced cellular differentiation and protection against apoptosis. J Biol Chem. 2001;276(36):33582–33587. doi:10.1074/jbc.M105318200
- Yuan L, Choi K, Khosla C, et al. Tissue transglutaminase 2 inhibition promotes cell death and chemosensitivity in glioblastomas. Mol Cancer Ther. 2005;4(9):1293–1302. doi:10.1158/1535-7163.MCT-04-0328
- Gundemir S, Monteagudo A, Akbar A, Keillor JW, Johnson GVW. The complex role of transglutaminase 2 in glioblastoma proliferation. Neuro-Oncology. 2017;19(2):208–218. doi:10.1093/neuonc/now157
- Sullivan KE, Rojas K, Cerione RA, Nakano I, Wilson KF. The cancer stem cell marker ALDH1A3 is the transcriptional regulator of the pharmaceutical target, tissue transglutaminase in mesenchymal glioma stem cells. Oncotarget. 2016;8:22325–22343. doi:10.18632/oncotarget.16479
- Daniel P, Meehan B, Rak J, et al. TGM2 novel biomarker of radiation response after neo-adjuvant TMZ in glioblastoma. Int J Radiat Oncol Biol Phys. 2020;108(3):e711–e712. doi:10.1016/j.ijrobp.2020.07.081
- Berg TJ, Marques C, Pantazopoulou V, et al. The irradiated brain microenvironment supports glioma stemness and survival via astrocyte-derived transglutaminase 2. Cancer Res. 2021;81(8):2101. doi:10.1158/0008-5472.CAN-20-1785
- Schiffer D, Annovazzi L, Casalone C, Corona C, Mellai M. Glioblastoma: microenvironment and niche concept. Cancers. 2019;11:1.
- Yin J, Oh YT, Kim JY, et al. Transglutaminase 2 inhibition reverses mesenchymal transdifferentiation of glioma stem cells by regulating C/EBPβ signaling. Cancer Res. 2017;77(18):4973. doi:10.1158/0008-5472.CAN-17-0388
- Pei J, Moon KS, Pan S, et al. Proteomic analysis between U87MG and U343MG-A cell lines: searching for candidate proteins for glioma invasion. Brain Tumor Res Treat. 2014;2(1):22–28. doi:10.14791/btrt.2014.2.1.22
- Frederick L, Wang XY, Eley G, James CD. Diversity and frequency of epidermal growth factor receptor mutations in human glioblastomas. Cancer Res. 2000;60(5):1383.
- Oprita A, Baloi SC, Staicu GA, et al. Updated insights on EGFR signaling pathways in glioma. Int J Mol Sci. 2021;22:2. doi:10.3390/ijms22020587
- Antonyak MA, Li B, Boroughs LK, et al. Cancer cell-derived microvesicles induce transformation by transferring tissue transglutaminase and fibronectin to recipient cells. Proc Natl Acad Sci U S A. 2011;108(12):4852–4857. doi:10.1073/pnas.1017667108
- Keillor JW, Apperley KYP, Akbar A. Inhibitors of tissue transglutaminase. Trends Pharmacol Sci. 2015;36(1):32–40. doi:10.1016/j.tips.2014.10.014
- Zhuang R, Khosla C. Substrates, inhibitors, and probes of mammalian transglutaminase 2. Anal Biochem. 2020;591:113560. doi:10.1016/j.ab.2019.113560
- Dickson RB, Willingham MC, Pastan I. Binding and internalization of 125I-alpha 2-macroglobulin by cultured fibroblasts. J Biol Chem. 1981;256(7):3454–3459. doi:10.1016/S0021-9258(19)69630-4
- Lorand L, Parameswaran KN, Stenberg P, et al. Specificity of Guinea-pig liver transglutaminase for amine substrates. Biochemistry. 1979;18(9):1756–1765. doi:10.1021/bi00576a019
- Igarashi S, Koide R, Shimohata T, et al. Suppression of aggregate formation and apoptosis by transglutaminase inhibitors in cells expressing truncated DRPLA protein with an expanded polyglutamine stretch. Nat Genet. 1998;18(2):111–117. doi:10.1038/ng0298-111
- Basso M, Berlin J, Xia L, et al. Transglutaminase inhibition protects against oxidative stress-induced neuronal death downstream of pathological ERK activation. J Neurosci. 2012;32(19):6561. doi:10.1523/JNEUROSCI.3353-11.2012
- Jeitner TM, Pinto JT, Cooper AJL. Cystamine and cysteamine as inhibitors of transglutaminase activity in vivo. Biosci. 2018;38:5.
- Choi K, Siegel M, Piper JL, et al. Chemistry and biology of dihydroisoxazole derivatives: selective inhibitors of human transglutaminase 2. Chem Biol. 2005;12(4):469–475. doi:10.1016/j.chembiol.2005.02.007
- Yuan L, Siegel M, Choi K, et al. Transglutaminase 2 inhibitor, KCC009, disrupts fibronectin assembly in the extracellular matrix and sensitizes orthotopic glioblastomas to chemotherapy. Oncogene. 2007;26(18):2563–2573. doi:10.1038/sj.onc.1210048
- Katt WP, Blobel NJ, Komarova S, Antonyak MA, Nakano I, Cerione RA. A small molecule regulator of tissue transglutaminase conformation inhibits the malignant phenotype of cancer cells. Oncotarget. 2018;9:34379–34397. doi:10.18632/oncotarget.26193
- Almami IS, Aldubayan MA, Felemban SG, et al. Neurite outgrowth inhibitory levels of organophosphates induce tissue transglutaminase activity in differentiating N2a cells: evidence for covalent adduct formation. Arch Toxicol. 2020;94(11):3861–3875. doi:10.1007/s00204-020-02852-w
- Schuppan D, Mäki M, Lundin KEA, et al. A randomized trial of a transglutaminase 2 inhibitor for celiac disease. N Engl J Med. 2021;385(1):35–45. doi:10.1056/NEJMoa2032441
- Dafik L, Albertelli M, Stamnaes J, Sollid LM, Khosla C. Activation and Inhibition of Transglutaminase 2 in Mice. PLoS One. 2012;7(2):e30642. doi:10.1371/journal.pone.0030642
- Pardin C, Roy I, Lubell WD, Keillor JW. Reversible and competitive cinnamoyl triazole inhibitors of tissue transglutaminase. Chem Biol Drug Des. 2008;72(3):189–196. doi:10.1111/j.1747-0285.2008.00696.x
- Apperley KY, Roy I, Saucier V, et al. Development of new scaffolds as reversible tissue transglutaminase inhibitors, with improved potency or resistance to glutathione addition. MedChemComm. 2017;8(2):338–345. doi:10.1039/C6MD00565A
- Case A, Stein RL. Kinetic analysis of the interaction of tissue transglutaminase with a nonpeptidic slow-binding inhibitor. Biochemistry. 2007;46(4):1106–1115. doi:10.1021/bi061787u
- Schneider MA, Heeb L, Beffinger MM, et al. Attenuation of peripheral serotonin inhibits tumor growth and enhances immune checkpoint blockade therapy in murine tumor models. Sci Transl Med. 2021;13(611):eabc8188. doi:10.1126/scitranslmed.abc8188
- Pinilla E, Comerma-Steffensen S, Prat-Duran J, et al. Transglutaminase 2 inhibitor LDN 27219 age-dependently lowers blood pressure and improves endothelium-dependent vasodilation in resistance arteries. Hypertension. 2021;77(1):216–227. doi:10.1161/HYPERTENSIONAHA.120.15352
- Datta S, Antonyak MA, Cerione RA. GTP-binding-defective forms of tissue transglutaminase trigger cell death. Biochemistry. 2007;46(51):14819–14829. doi:10.1021/bi701422h
- Colak G, Keillor JW, Johnson GVW. Cytosolic guanine nucledotide binding deficient form of transglutaminase 2 (R580a) potentiates cell death in oxygen glucose deprivation. PLoS One. 2011;6(1):e16665. doi:10.1371/journal.pone.0016665
- National Cancer Institute. Pioglitazone hydrochloride in preventing head and neck cancer in patients with oral leukoplakia. ClinicalTrials.gov; 2003. Available from: https://clinicaltrials.gov/ct2/show/NCT00099021. Accessed October 3, 2021.
- National Cancer Institute. Rosiglitazone maleate in treating patients with oral leukoplakia. Clinicaltrials.gov; 2006. Available from: https://clinicaltrials.gov/ct2/show/study/NCT00369174. Accessed October 3, 2021.
- Sulic AM, Kurppa K, Rauhavirta T, Kaukinen K, Lindfors K. Transglutaminase as a therapeutic target for celiac disease. Expert Opin Ther Targets. 2015;19:1–14. doi:10.1517/14728222.2014.985207
- Yakubov B, Chelladurai B, Schmitt J, Emerson R, Turchi JJ, Matei D. Extracellular tissue transglutaminase activates noncanonical NF-κB signaling and promotes metastasis in ovarian cancer. Neoplasia. 2013;15(6):609–IN8. doi:10.1593/neo.121878
- Antonyak MA, Li B, Regan AD, Feng QY, Dusaban SS, Cerione RA. Tissue transglutaminase is an essential participant in the epidermal growth factor-stimulated signaling pathway leading to cancer cell migration and invasion. J Biol Chem. 2009;284(27):17914–17925. doi:10.1074/jbc.M109.013037
- Bordeleau F, Wang W, Simmons A, Antonyak MA, Cerione RA, Reinhart-King CA. Tissue transglutaminase 2 regulates tumor cell tensional homeostasis by increasing contractility. J Cell Sci. 2020;133(1):765.
- Li B, Antonyak MA, Druso JE, Cheng L, Nikitin AY, Cerione RA. EGF potentiated oncogenesis requires a tissue transglutaminase-dependent signaling pathway leading to Src activation. Proc Natl Acad Sci U S A. 2010;107(4):1408–1413. doi:10.1073/pnas.0907907107
- Xu L, Begum S, Hearn JD, Hynes RO. GPR56, an atypical G protein-coupled receptor, binds tissue transglutaminase, TG2, and inhibits melanoma tumor growth and metastasis. Proc Natl Acad Sci U S A. 2006;103(24):9023–9028. doi:10.1073/pnas.0602681103
- Shashidhar S, Lorente G, Nagavarapu U, et al. GPR56 is a GPCR that is overexpressed in gliomas and functions in tumor cell adhesion. Oncogene. 2005;24(10):1673–1682. doi:10.1038/sj.onc.1208395
- Patel SA, Minn AJ. Combination cancer therapy with immune checkpoint blockade: mechanisms and strategies. Immunity. 2018;48(3):417–433. doi:10.1016/j.immuni.2018.03.007
- Huber V, Fais S, Iero M, et al. Human colorectal cancer cells induce T-cell death through release of proapoptotic microvesicles: role in immune escape. Gastroenterology. 2005;128(7):1796–1804. doi:10.1053/j.gastro.2005.03.045
- Yin Y, Cai X, Chen X, et al. Tumor-secreted miR-214 induces regulatory T cells: a major link between immune evasion and tumor growth. Cell Res. 2014;24(10):1164–1180. doi:10.1038/cr.2014.121
- Mahoney KM, Freeman GJ, McDermott DF. The next immune-checkpoint inhibitors: PD-1/PD-L1 blockade in melanoma. Clin Ther. 2015;37(4):764–782. doi:10.1016/j.clinthera.2015.02.018