Abstract
The present study reports on the production of low molecular weight heparin (LMWH) loaded polymeric microspheres for delivery via the oral route. The microspheres were prepared by the spray-drying technique using Eudragit® as the polymer. The objective of this study was to examine extensively the influence of formulation and process variables on the characteristics of the microspheres prepared. The effects of various experimental parameters such as polymer concentration, inlet temperature, and liquid feed flow rate on particle morphology, particle dimensions, and production yields were evaluated by means of experimental factorial designs. Electron microscopy, moisture content analysis, and fractal dimensional analysis were employed to characterize the microspheres. The inlet temperature and polymer concentration had the greatest effects on the production yield. Results showed that the polymer concentration affected the dimensions of the microspheres. Drug-loaded microspheres were spherical in shape and had a smooth surface with sizes ranging between 19–60 μm. Production yields were above 50% under most of the operating parameters studied. The selection of appropriate parameters yielded spray-dried microparticles characterized by smooth morphology and narrow dimensional distribution.
Keywords:
Introduction
Spray drying is a well-established drying process traditionally used for thermolabile materials (CitationMasters 1990). It has been used successfully in the pharmaceutical industry to produce products of defined physical and chemical properties. In this study we have investigated spray drying as a potential method for the production of micron-sized particles of a LMWH (ardeparin). Spray drying is widely used for the microencapsulation of drugs due to reliability, reproducibility and possible control of particle size and drug release. In addition, it has the advantage of being a continuous process which is easy to scale-up (Bodmeier and Chen 1998). Another important advantage is the fact that microparticles obtained by spray-drying are usually free of organic solvents, whereas other methods often result in particles contaminated with organic solvents which may be toxic (CitationBroadhead 1992). The spray drying technique consists of spraying an emulsion of polymer and drug through the nozzle of a spray dryer apparatus. The solvent evaporates very quickly, leaving behind solid microparticles (CitationConti et al 1992). The spray drying process involves the following four sequential stages: atomization of the product into a spray nozzle, spray-air contact, drying of the sprayed droplets, and collection of the solid product (spray-dried microparticles) obtained (CitationConti et al 1992). A stable powder is rapidly obtained without lyophilization. In a single operation a drug can be entrapped into a polymer either as a solution, dispersion, or an emulsion (CitationBittner et al 1998). Hydrophobic as well as hydrophilic drugs can be incorporated into a polymer by spray drying. In this study, the spray dryer was a mini-Büchi 190 (Büchi Co., Flawil, Switzerland) which is a laboratory scale spray dryer. The main components of the equipment include the air-heater, the nozzle/atomizer, the desiccation chamber (spray-drying cylinder), the fan, the cyclone collector (for separation between the product and the air-flow) and a final collector vessel in which the spray-dried product is collected.
The quest for an ideal anticoagulant has resulted in a number of oral factor Xa inhibitors and direct thrombin inhibitors being introduced in the market. Low molecular weight heparin (LMWH) is the agent of choice in the anti-coagulant therapy and prophylaxis of thrombosis and coronary syndromes (Gallus 1992; CitationWeltermann et al 2003). However, a major disadvantage of LMWHs is that they can only be administered parenterally, due to a lack of absorption when administered orally (CitationGrau et al 2001; CitationBreddin et al 2001; CitationMoney and York 2001). The development of oral formulations for LMWH would have tremendous clinical importance as it would result in avoidance of the pain and discomfort associated with injections, thereby offering better patient compliance. The intestinal absorption of LMWHs is limited owing to their poor membrane permeability characteristics such as hydrophilicity, anionic surface charges and high molecular weight (CitationRoss and Toth 2005). Moreover, these therapeutic macromolecules are unstable in the acidic conditions of the stomach. It has been shown that desulfation of heparin may occur in the acidic pH of the stomach (CitationLarsen et al 1989). Selective deacetylation and desulfation of heparin have been shown to affect both its anticoagulant activity and in vivo disposition characteristics (CitationBjornsson et al 1988). Since LMWHs are not absorbed in the gastrointestinal tract, recent studies have focused on combining LMWH with carriers (CitationSalartash et al 1999; CitationJiao et al 2002).
Since several polymeric microparticles are proven to protect and target drugs, we made use of this formulation approach in our study (Esposito et al 2001). A polymer belonging to the class of Eudgragit® polymers was selected for this study. These polymers are widely accepted in the pharmaceutical industry (Esposito et al 2001). The carrier chosen was Eudragit S100 which is a methacrylate polymer composed of methacrylic acid-methacrylic acid methyl ester copolymers. This was chosen for its gastroresistance as it is a pH-dependant enterosoluble polymer which is soluble above pH 7.0. S100 is a non-biodegradable polycationic polymer which is generally used for enteric coating (Esposito et al 2001). This polymer was chosen for the production of microparticles by spray-drying in the current study because of its inertness and solubility characteristics (Esposito et al 2001). Since spray drying depends on various interacting variables and operating conditions, the effects of process and formulation variables were investigated. The effects of these variables on particle size and yield were of primary importance to this study. Other response parameters of interest were particle morphology, moisture content, encapsulation efficiency, in vitro release and fractal dimension of the resulting microparticles obtained using the spray-drying process.
Materials and methods
LMWH was purchased from Celcus Laboratories Inc. (Boston, MA). Eudragit S100 was kindly provided by Rohm Pharma (Darmstadt, Germany). The commercial kit used for the evaluation of anti-factor Xa activity was purchased from Dia-pharma group (Westchester, OH). Other reagents used were purchased from Sigma Chemical Co. (St. Louis, MO).
Design of experiments
The design of experiments (DOE) technique was used to provide an efficient means to optimize the spray drying process. DOE is an approach for effectively and efficiently exploring the cause and effect relationship between numerous process variables and the output. A sequence of experiments were performed that would yield the most information about the factors and their interactions in as few experiments as possible. A 3-factor 3-level factorial Box-Behnken experimental design technique was employed to investigate the variables using the statistical software package Statvision (Statgraphics, Manugistics Inc., Rockville, MD). This technique was applied to quantify the influence of operating parameters on the production of microparticles during the spray drying operation. The fractional factorial design created constituted 15 of the experiments in this study. The dependant variables were polymer concentration, inlet temperature and feed flow rate. Preliminary experiments were performed to confirm the operational phase range that would successfully yield spray-dried microparticles and to verify that the runs could be conducted at the operational units dictated by the factorial design. The software was also used to construct mathematical models for making response predictions for further experiments. Graphs showing the magnitude of effects for each variable and interactions were generated for analysis. The experimental design matrix is shown in . The goal of the experimental design was to find out, with the minimum number of experimental runs, which process variables have the biggest impact on the final product.
Table 1 Factors and levels in the Box-Behnken design
Spray-drying procedure
The mini spray dryer employed was a Büchi 290 (Büchi Co., Flawil, Switzerland). Aqueous solutions of LMWH ardeparin and Eudragit S100® (methacrylic acid copolymer type B MW 135,000) were prepared at their given concentrations in purified water. The various spray-drying process conditions are listed in . Briefly, given amounts of S100 were dissolved in 100 mL of purified water and 500 mg of LMWH, ardeparin was added to this solution under stirring. The resultant mixture was fed into the spray-dryer by a peristaltic pump and sprayed with a 0.7 mm nozzle, by means of a flow of compressed air, in the drying chamber of the apparatus. A flow of heated air aspirated by a pump induced the quick evaporation of the solvent from the drops, leading to the formation of solid microparticles. The particles were separated from the exhausted air in a cyclone and settled into a bottom collector using a Model 290 bench top spray dryer (Büchi Co, Flawil, Switzerland) using the following settings: pressure 85 mbar, solvent flow rate 15%–25%, and temperature 80–100 °C. The drug loading was kept constant for all runs at 0.5%. At the end of the spray-drying process, the particles were collected and subsequently characterized.
Particle morphology and geometric size
Surface characteristics of the various spray dried microparticles were examined using scanning electron microscopy (SEM). Dried microparticles were analyzed without sputter coating at 15–20 kV. SEM images were obtained for all the runs to examine particle morphology. Samples were analyzed using a Hitachi S-3400 II Scanning Electron Microscope (Hitachi High Technologies America, Inc. Schaumburg, IL). Microsphere size was determined by examining the diameter on digital photomicrographs. The analysis was performed by a computerized size analysis system built into the above SEM system.
Fractal analysis
Fractal dimension was applied to study the surface roughness of microparticles. The fractal dimension was measured using BenoitTM 1.3 (St. Petersburg, FL). The fractal dimension was calculated using the Box-dimension method. The fractal dimension Df, is a measure of the particle surface. The SEM images were used for the computation of dimensions after conversion to a bitmap image file.
Determination of residual moisture content
The residual water content for the microparticle samples was determined by Karl Fisher titration using a Brinkmann 756 KF Coulometer (Hamburg, Germany). Typically 10 mg of sample was dissolved in 1 mL of reagent Hydranal®-Coulomat AG-H and added to the Karl Fisher titration vessel following equilibration of the instrument for moisture determination. The moisture content of the anhydrous reagent was determined as the baseline and subtracted from data as baseline correction (CitationTattini et al 2005).
Production yield/recovery
Microparticle recovery efficiencies were calculated as percentage of weight of microparticles obtained compared to the total amount of polymer and drug initially used in the formulation process.
Results and discussion
Fractal dimension
The fractal concept of Mandelbrot (CitationMandelbrot 1982) offers the theoretical basis for the understanding of the changes in boundary lengths due to differences in resolution. The surface fractal dimension (Df) is the measure of the space-filling ability of a curve (CitationClark 1986). The more irregular and unsteady an object is, the higher the value of Df. This has implications in understanding the physical behavior of pharmaceutical solids. An advantage of fractal analysis is that it partitions roughness characteristics of a surface into a scale-free component (fractal dimension). It is an attractive method for summarizing properties of roughness (CitationDavies 1999). The earliest work in fractal analysis has shown that the degree of surface roughness or irregularities can be expressed by the parameter Df (CitationAvnir and Pfeifer 1983; CitationFarin and Avnir 1992; CitationPfeifer 1983). A perfectly smooth surface has a low Df value while a highly rough, disordered surface has a high Df. The fractal dimension can be derived from the slope of Richardson plots which are linear. The importance of this technique is that the roughness of solid surfaces can now be reasonably quantified. The fractal dimension of solid surfaces can be determined using a variety of techniques including scanning electron microscopy (CitationFriel and Pande 1998).
The fractal dimension of the particle contour provides information on the appearance of the surface that is difficult to be evidenced even in qualitative form. indicates that formulation no. 3 had the smoothest surface with fewer irregularities while formulation no. 12 had a more irregular surface (). The lowest value for formulation no. 3 not only confirms the SEM observation, but also suggests a lower degree of irregularity than that appreciated by direct vision. Fractal geometry has been used to determine effects of surface morphology on drug dissolution (CitationFarin and Avnir 1992). In this regard, the Noyes-Whitney equation and Hixon-Crowell cube root laws have been modified to include surface roughness effects on the dissolution rate of drugs. Surface irregularity and shape allow better contact with the dissolution medium and improvement of the dissolution rate and therefore, a more efficient pharmaceutical form. Thus, the particles in our study possessing greater irregularity would be able to release drug more efficiently.
Table 2 Box-Behnken experimental design grid and responses pertaining to microparticle production
Table 3 Fractal dimension and residual moisture content for microparticles prepared by spray drying
Influence of operating conditions on microsphere characteristics
The input parameters and the resulting responses for the 15 experimental runs are listed in . The Box-Behnken design is suitable for understanding the quadratic response surfaces. The design consists of replicated center points and the set of points lying at the mid-point of each surface of the cube defining the region of interest. Interaction effects between the factors resolved by the design allow a mid-level value for a combination of factors (CitationBox 1960).
Qualitative estimates of the influence of the individual variables could be made by inspection of the data in . However, it would be difficult visually to make predictions as to whether the interactions actually existed between the variables, or which single variable had the most dominant effect. To achieve this, fractional factorial statistical design methods were applied to all 15 runs. These designs are good alternatives to a full factorial design, especially in the initial screening stage. In this study a 3-factorial design was used where main factor effects are confounded with two factor and higher order interactions. The main effects and interaction effects calculated for the responses of percent yield and mean diameter are shown in .The variables A, B, and C represent polymer concentration, inlet temperature, and feed flow rate, respectively. Interactions between two and among three variables are shown as AB, BC, AC, and ABC. The standardized Pareto Chart shown contains a bar for each effect, sorted from most significant to least significant. The length of each bar is proportional to the standardized effect, which is equal to the magnitude of the t-statistic that would be used to test the statistical significance of that effect. A vertical line is drawn at the location of the 0.05 critical values for Student’s t. Any bars that extend to the right of that line indicate effects that are statistically significant at the 5% significance level. From the magnitudes of all the effects, inlet temperature and polymer concentration appeared to be the main factors affecting the percent yield. There was no main factor for the effect on particle size. The interactions between the variables did not seem very prominent for the percent yield or particle size. From the analysis, it is concluded that inlet temperature and polymer concentration were the important factors affecting yield or recovery of particles. The results obtained for all experiments performed revealed that the particle size distribution were practically insensitive to the spray drying operating parameters. indicates that the production yield was highest for formulation no. 3 (59.26%) while it was lowest for both formulation no.’s 2 and 7 (0%). This difference may be attributed to either higher temperature or higher polymer concentration. In terms of mean particle size, formulation no. 4 has the smallest size (19.62 μm) while formulation no. 3 had the largest size (42.92). The operation variable responsible for this cannot be isolated from these results.
Figure 1 Scanning electron micrographs of spray-dried microparticles and native heparin. (Magnification 200X. scale corresponds to 200 μm) Note: no particles obtained for run no.’s 2 and 7.
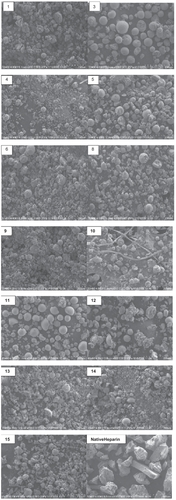
The yields ranged from 0 to 60%. Most of the runs resulted in low yields because of the difficulties in particle collection. Condensation inside the chamber leads to a low yield as the dry particles tend to stick to the walls of the chamber and therefore cannot be collected. The yield was expected to be greater at higher temperature due to the increase throughput of the polymer slurry and rapid evaporation of the solvent. Dramatic yield losses in formulation no’s 2 and 7 were caused by lack of drying at lower temperature and adherence of some of the polymer onto the sides of the atomization chamber. Also, the mean diameters at higher temperature were increased. Interdroplet agglomeration probably contributed to larger mean particle sizes with formulation. Inlet temperature of air-drying is an important parameter for both particle size and yield. The temperature employed in spray drying operation has to be compatible with the material to be dried and the solvent used (CitationTorrado et al 1989). High pumping rates during the spray drying process result in large volumes of nebulized solution to be dried. Owing to this, heated air may not instantaneously transform the liquid droplets into solid microparticles, leading to the formation of larger/irregular particles that are not completely dried after leaving the desiccating chamber (CitationWan et al 1991). At higher feed rates, the atomizing air may not be able to penetrate the stream of liquid. As a result, incomplete atomization may lead to wider droplet size distribution (CitationMasters, 1990). The reduction in yield may be attributed to the incomplete atomization and drying, resulting in the deposition of a large amount of microparticles on the walls of the desiccating chamber and the cyclone separator. The spray drying process resulted in a range of particle sizes. Five different runs produce the smallest size and there were no common input conditions for these runs. These results are expected as low polymer concentration decreases the amount of solid in each droplet exiting the nozzle. Therefore, when the water in the droplet evaporates, a smaller particle remains. Also, when the feed flow rate is high, the drop exiting the nozzle tends to be smaller and hence the resulting particle will be smaller. The polynomial equation (regression) relating dependant (response) and independent variables obtained is: Percent Yield = −119.86 + 2.42438*Polymer Concentration + 1.85462*Inlet Temperature - 1.35525*Feed Flow Rate To test the statistical significance of the effects, ANOVA was carried out. The ANOVA table partitions the variability in percent yield into separate pieces for each of the effects. It then tests the statistical significance of each effect by comparing the mean square against an estimate of the experimental error. In this case, 2 effects have P-values less than 0.05 indicating that they are significantly different from zero. The R2 statistic indicated that the model as fitted explains 73.25% of the variability in percent yield. The standard error of the estimate shows the standard deviation of the residuals to be 11.33. In the case of particle size, 1 effect has P-values less than 0.05, indicating that they are significantly different from zero. The R2 statistic indicated that the model as fitted explains 37.17% of the variability in particle size. The adjusted R2 statistic, which is more suitable for comparing models with different numbers of independent variables, is 20.03%. The standard error of the estimate shows the standard deviation of the residuals to be 10.98. To fully understand the fitted model, a wire frame surface plot was created (). In this plot, the height of the surface represents the predicted value of yield over the space of temperature and polymer concentration; with the third factor ie, feed flow rate held constant at its middle values. A similar plot was made for the particle size response ().
Prediction of theoretical response values (Response Optimization)
After establishing the relationship between the dependant and independent variables, the process was optimized. A computerized optimization procedure was used to obtain the levels of polymer concentration, inlet temperature and feed flow rate at which a maximized percent yield and particle size could be obtained. The combination of factor levels leading to attainment of maximum response was 9%, 100 °C, and 15% for polymer concentration, inlet temperature and feed flow rate, respectively. The predicted optimum values found were 67.03 and 40.87 for percent yield and particle size, respectively.
Scanning electron microscopy
The particle morphologies observed were smooth spheres (no’s 3, 5, 10, 11, and 12) and collapsed particles (1, 4, 6, 8, and 9). Examples of these can be seen in the images shown in . The figure indicates that formulation no’s 3 and 11 possess uniform spherical morphology characteristics. The common factor in these two cases was the polymer concentration at 9%. SEM studies of the remaining formulation numbers indicated the formation of collapsed microparticles. Microparticles made at low polymer concentration of 1% were poorly formed. The viscosity of the polymer solution was too low to maintain the stability of the droplets during spray-drying. Higher concentration and higher viscosity of the polymer solution resulted in good droplet stability. The SEM micrograph of the native drug illustrates large crystalline particles while the SEM micrographs of the spray dried particles indicate smooth surfaces which are much smaller in size when compared with the drug. The particle size is an important parameter in drug design and delivery. For example, decreasing the particle size increases the surface area, which enhances the dissolution rate (CitationKondo et al 1993).
Production yields
The yields ranged from 0% to 60%. Most of the runs resulted in low yields because of the difficulties in particle collection. Condensation inside the chamber leads to a low yield as the dry particles tend to stick to the walls of the chamber and therefore cannot be collected.
Moisture content
The moisture content of a powder influences flow and dispersion from the perspective of capillary forces and liquid bridging between the particles. The strength of the cohesive force depends on the surface tension, wetting angle, space between the particles and particle diameter. The same particle characteristics that result in moisture uptake will influence dissolution properties. This parameter is also important for the prediction of the flow behavior of the product in the tabletting machines or in the encapsulation machines during the preparation of pharmaceutical dosage forms. shows the results obtained. It can be observed that the temperature is the most important parameter for the product moisture content. In general, higher temperatures lead to a product with lower moisture content. In terms of residual moisture content, formulation no. 8 was found to be the best due to lowest water content (2.80%). This was in compliance with USP requirements for product storage (CitationUSP 1994).
Conclusions
Microparticles were successfully prepared by spray-drying technique. SEM micrographs showed that the particles sizes of the various formulations ranged from 19 to 60 μm and that the surfaces were smooth in appearance. The factorial design proved to be a valuable technique for optimizing the production of spray-dried microparticles. Since spray-drying involved various parameters for study, the number of experiments was minimized while a detailed evaluation of the dominant variable effects and interactions was accomplished. The conditions for obtaining microparticles with optimal yield (67.03%) and mean diameter (40.87 μm) under the operating conditions used were determined. Highest yields (56%) were obtained with highest temperature (100 °C) and at a polymer concentration of 9%. Smallest particle size (19 μm) was obtained with the temperature at 80 °C and a polymer concentration of 5%. Results indicate that the pH-sensitive polymer may protect LMWH against acidic pH after microencapsulation using the spray drying technique. This approach may contribute to enhanced oral bioavailability of LMWHs. This work confirms the feasibility of the spray drying for the preparation of microparticulate systems with incorporated controlled-release mechanism to modify LMWH release. Results indicate that the drug release rate from microparticles can be controlled by a proper choice of polymer type, polymer amount and, spray drying operating conditions such as the inlet temperature and feed rate of the microencapsulating composition. One limitation of this study is that the stability of the drug within the microparticles has not been determined. This data would be useful to determine whether the polymer interacts in a significant manner with the LMWH contained within the particles. Further research is needed before this study can provide important clinical relevance with regards to anti-coagulant therapy. Future studies describing the in vivo absorption and anti-coagulant activity of the formulation in an appropriate animal model will aid in determining the potential of these microparticles for successfully overcoming the challenges faced in the delivery of LMWH via the oral route.
Acknowledgments
The administrators at the department of pharmaceutical sciences are gratefully acknowledged for their support. The experiments in this study were carried out at Texas Tech University. There are no conflicts of interest to report.
References
- AvnirDPfeiferP1983Chemistry in noninteger dimensions between two and three. II. Fractal surfaces of adsorbentsJ Chem Phys79356673
- BittnerBRonnebergerBZangeR1998Bovine serum albumin loaded poly(lactide-co-glycolide) microspheres: the influence of polymer purity on particle characteristicsJ Microencapsul154955149651871
- BjornssonTDSchneiderDEHechtAR1988Effects of N-deacetylation and N-desulfation of heparin on its anticoagulant activity and in vivo dispositionJ Pharmacol Exp Ther24580483164387
- BodmeierRChenHG1988Preparation of biodegradable poly(+/−)lactide microparticles using a spray-drying techniqueJ Pharm Pharmacol4075472907552
- BoxGBD1960Some new three level designs for the study of quantitative variablesTechnometrics245575
- BreddinHKHach-WunderleVNakovRETAL2001Effects of a low-molecular-weight heparin on thrombus regression and recurrent thromboembolism in patients with deep-vein thrombosisN Engl J Med3446263111228276
- BroadheadER1992The spray drying of pharmaceuticalsDrug Dev and Ind Pharm181169206
- ClarkN1986Powder Technology464552
- ContiBPavanettoFGentaI1992Use of polylactic acid for the preparation of microparticulate drug delivery systemsJ Microencapsul9153661593398
- DaviesSH1999Statistical methodologyJournal of the Royal Statistical Society (series B)613
- EspositoERoncaratiRCortesiR2000Production of Eudragit microparticles by spray-drying technique: influence of experimental parameters on morphological and dimensional characteristicsPharm Dev Technol52677810810756
- FarinDAvnirD1992Use of fractal geometry to determine effects of surface morphology on drug dissolutionJ Pharm Sci815471619570
- FrielJJPandeCS1998A direct determination of fractal dimension of fracture surfaces using scanning electron mocroscopy and stereoscopyJ Mater Res81006
- GallusAS1990Anticoagulants in the prevention of venous thromboembolismBaillieres Clin Haematol3651842148697
- GrauETeniasJMRealE2001Home treatment of deep venous thrombosis with low molecular weight heparin: Long-term incidence of recurrent venous thromboembolismAm J Hematol67101411279651
- JiaoYYUbrichNHoffartV2002Preparation and characterization of heparin-loaded polymeric microparticlesDrug Dev Ind Pharm2810334112378958
- KondoNIwaoTMasudaH1993Improved oral absorption of a poorly water-soluble drug, HO-221, by wet-bead milling producing particles in submicron regionChem Pharm Bull (Tokyo)41737408508476
- LarsenAKRiceKGLinhardtRJ1989Resistance of heparinase-derived heparin fragments to biotransformationJ Biol Chem264157072912974
- MandelbrotB1982The fractal geometry of natureSan FranciscoFreeman
- MastersK1990Spray Drying HandbookNew YorkLongmans
- MoneySRYorkJW2001Development of oral heparin therapy for prophylaxis and treatment of deep venous thrombosisCardiovasc Surg92111811336843
- PfeiferPAvnirD1983Chemistry in noninteger dimensions between two and three. I. Fractal theory of heterogenous surfacesJ Chem Phys79355865
- RossBPTothI2005Gastrointestinal absorption of heparin by lipidization or coadministration with penetration enhancersCurrent Drug Delivery22778716305430
- SalartashKGonzeMDLeone-BayA1999Oral low-molecular weight heparin and delivery agent prevents jugular venous thrombosis in the ratJ Vasc Surg305263110477646
- TattiniVParraDFPolakiewiczB2005Effect of lyophilization on the structure and phase changes of PEGylated-bovine serum albuminInt J Pharm3041243416188407
- TorradoJIllumLDavisS1989Particle size and size distribution of albumin microspheres produced by heat and chemical stabilizationInt J Pharm518593
- U.S. Pharmacopoeia National Formulary, USP XXIII NF XVIII. 1994. pg. 2300.
- WanLHengPChiaC1991Preparation of coated particles using a spray drying process with an aqueous systemInt J Pharm7718391
- WeltermannAKyrlePAEichingerS2003Novel anticoagulants for the prevention and treatment of venous thromboembolismWien Med Wochenschr1534263314648923