Abstract
Bacteriophages, as abundant and specific agents, hold significant promise as a solution to combat the growing threat of antimicrobial resistance. Their unique ability to selectively lyse bacterial cells without harming humans makes them a compelling alternative to traditional antibiotics and point-of-care diagnostics. The article reviews the current landscape of diagnostic technologies, identify gaps and highlight emerging possibilities demonstrates a comprehensive approach to advancing clinical diagnosis of microbial pathogens and covers an overview of existing phage-based bioassays. Overall, the provided data in this review effectively communicates the potential of bacteriophages in transforming therapeutic and diagnostic paradigms, offering a holistic perspective on the benefits and opportunities they present in combating microbial infections and enhancing public health.
Plain language summary
Bacteriophages, a type of virus that infects bacteria, have a lot of potential to help us understand and detect bacteria that can cause disease. This review talks about how bacteriophages could be used in medicine and for testing for diseases, and for identifying disease-causing bacteria in easy-to-use diagnostic tools. This is important for not only population health, but also for animals and the protection of food supplies by stopping the spread of harmful bacteria in farming.
The topic of phage therapeutics has garnered widespread attention, and a deep dive into phage biology, including its life cycle and interactions with host bacteria, underscores its promising role as a versatile tool for addressing persistent challenges posed by infections – be they foodborne, airborne, waterborne or clinical.
Phage biology & their diagnostic potential
The diagnostic potential of phages arises from the inherent characteristics of phages, which exhibit self-replication and host-specificity. Of particular interest in the realm of phage-based diagnostics is the remarkable degree of specificity and sensitivity that can be achieved, coupled with the advantage of minimizing costs and time.
Diagnostic applications of phages
This diagnostic approach employs various strategies, such as phage capture elements, phage-labeling, reporter phages and phage amplification, all of which hold immense promise for rapid and sensitive detection of bacterial infections.
Future perspective
Phage display, with its ability for the rapid isolation of specific ligands, holds numerous advantages across various applications. It is instrumental in selecting inhibitors for both the active and allosteric sites of enzymes, as well as identifying receptor agonists, antagonists and modulatory peptides for G-protein binding.
Conclusion
Leveraging phages as diagnostic tools capitalizes on their distinct interactions with bacterial hosts, enabling rapid and precise detection of pathogenic microorganisms.
Bacterial infections have a pervasive presence in nature, impacting the health of humans, plants and animals alike due to their wide ecological range. While certain bacterial pathogens co-exist harmlessly with specific biological hosts, they can cause infections in other hosts. For example, Borrelia burgdorferi, the bacterium responsible for Lyme disease, co-exists in ticks, mice and deer without causing harm, but becomes pathogenic in dogs and humans. This phenomenon highlights the diverse range of hosts that serve as new platforms for bacterial pathogens, leading to varying infections due to bacterial adaptations [Citation1,Citation2]. The global increase in the use of drugs across agriculture, livestock, and healthcare has contributed to a rise in antimicrobial resistance (AMR), presenting a significant public health challenge. AMR-related diagnostic issues are becoming more pressing and require comprehensive solutions [Citation3]. A major challenge in addressing AMR is accurately measuring its prevalence in various environments such as soil, water, and food for effective surveillance. To counteract AMR, there is a need for innovative point-of-care diagnostic methods that can promptly identify drug-resistant bacteria. Such timely identification enables tailored treatment strategies based on individual patient needs [Citation4]. Timely and precise diagnosis is a crucial step in the battle against infections, as it helps curtail unnecessary antibiotic use. The unregulated use of antibiotics in agriculture and livestock further contributes to this issue. Globally, approximately 73% of antimicrobials are used to enhance meat production due to the high demand for high-protein diets [Citation4,Citation5]. The emergence of periodic outbreaks caused by multidrug-resistant bacteria of global concern, including Pseudomonas, Acinetobacter, E. coli, Klebsiella, Serratia and Proteus, poses significant threats to human health and requires effective control strategies [Citation6]. As such, addressing bacterial infections and combating AMR demand a multifaceted approach that includes accurate diagnostics, judicious antibiotic use, and innovative solutions for various sectors, from healthcare to agriculture. The transmission dynamics of these pathogens underscore their spread within the environment through various routes, including water, food, air and clinical settings. Phage therapeutics has emerged as a highly debated and explored topic, with the fundamental understanding of phage biology, its life cycle, and interactions with host bacteria shedding light on its potential as a bio-probe or assay [Citation7,Citation8]. This potential holds promise in addressing persistent challenges associated with infections, whether they are foodborne, airborne, waterborne or clinical in nature. The inherent qualities of phages, such as their self-replicating ability and host-specific nature, position them as a valuable tool in combating these infectious threats [Citation9,Citation10]. Phage-based diagnostics offer a captivating aspect of achieving unparalleled levels of specificity and sensitivity while minimizing both cost and time requirements. The ingenious utilization of the phage display technique further enhances the diagnostic potential [Citation11]. This technique involves the incorporation of a protein of interest into the phage coat protein, resulting in the external display of the protein. This connection between genotype and phenotype showcases the fusion of genetic information with observable characteristics. By exploiting this technique, phage-based diagnostics can leverage the unique properties of phages to precisely target and identify specific pathogens or molecules of interest [Citation11,Citation12]. This approach promises to revolutionize the field of diagnostics by providing a powerful tool that combines the advantages of phage biology with the precision of molecular recognition, ultimately enhancing our ability to detect and diagnose a wide range of infections and diseases [Citation13]. Phage display platforms offer a versatile approach for screening phages against a variety of DNA sequences, peptides and proteins [Citation10,Citation14]. This technique involves the presentation of diverse peptides or proteins on the surface of phages, which can then be subjected to interactions with specific target entities. By measuring the interactions between these displayed proteins and the desired targets, researchers can gain valuable insights into molecular binding, recognition, and potential applications. This methodology enables the identification of peptides or proteins that have a high affinity for the intended targets. It can be used for purposes such as identifying potential drug candidates, understanding protein–protein interactions, studying immune responses and developing diagnostic assays. By harnessing the power of phage display, researchers can expedite the process of discovering molecules with desired properties, leading to advancements in various fields including medicine, biotechnology and molecular research [Citation15,Citation16]. Indeed, a growing body of evidence highlights the successful application of phage-based assays for the detection of bacteria in diverse settings, including clinical specimens, food samples, and environmental samples. These innovative assays leverage the specific interactions between phages and their target bacteria, offering rapid and accurate detection methods. In clinical contexts, phage-based assays have been utilized to detect various bacterial pathogens in patient samples, enabling early and precise diagnosis. Similarly, in the realm of food safety, these assays have been employed to identify harmful bacteria in food products, contributing to enhanced quality control and public health protection. Furthermore, the application of phage-based assays in environmental monitoring has allowed for the detection of bacteria in water, soil, and other ecological samples, aiding in the surveillance of potential contaminants. These successful demonstrations underscore the potential of phage-based assays as versatile tools with broad-ranging applications, offering valuable solutions for various sectors concerned with bacterial detection and identification [Citation17–19]. provides an overview of the various phage-based biosensors that have been developed for bacterial detection using different analytical approaches. These biosensors leverage whole phage particles or their encoded proteins to effectively screen for the presence of bacteria. Phage-based diagnostics encompass a range of techniques, including phage capture elements, phage labeling, reporter phages and phage amplification. These approaches offer the potential for rapid and highly sensitive bacterial detection. In contrast to current diagnostic procedures, which often require extensive technician training, sophisticated laboratory infrastructure, and carry a high risk of producing false results, phage-based biosensors offer a promising alternative. Additionally, these biosensors have the potential to address the need for diagnostic methods capable of identifying drug-resistant bacteria and conducting antibiotic susceptibility testing. This represents a significant advancement in the field of bacterial detection and has the potential to enhance patient care and public health outcomes [Citation20]. Molecular diagnostic methods are commonly employed in both clinical and agricultural contexts. Polymerase chain reaction (PCR) stands out as the most prevalent molecular technique, widely used for detecting specific genetic sequences. However, PCR has its limitations – it is costly, susceptible to inhibition, and requires skilled personnel to perform and interpret the assays [Citation21]. Additionally, immunological assays such as enzyme-linked immunosorbent assay (ELISA) are commonly utilized in the diagnostic industry for detecting bacteria and food toxins. Despite their widespread use, immune-based assays often exhibit lower sensitivity and specificity compared with other methods. An alternative approach is whole-genome sequencing, which offers comprehensive genetic information about an organism. However, the high costs associated with whole-genome sequencing limit its broader adoption, especially in industries like agriculture and food production, where demand is high. This review article delves into the current landscape of phage-based detection methods for microbial infections across various sectors including healthcare, food production, farming and livestock management. Phages, which are viruses that infect bacteria, can serve as valuable tools in detecting and identifying specific bacterial strains. Phage-based techniques include phage typing, where different phage strains are used to differentiate and classify bacterial strains based on their susceptibility to specific phages. These innovative approaches have the potential to offer enhanced sensitivity, specificity, and cost–effectiveness compared with traditional methods. In summary, this review sheds light on the evolving field of phage-based detection, which holds promise for revolutionizing microbial infection diagnosis across diverse industries, ranging from healthcare to agriculture and beyond. Phage typing strategy exists for the variety of clinical pathogens such as Clostridium [Citation22], Brucella [Citation23], Enterococci [Citation24], Salmonella [Citation25,Citation26], Shigella [Citation27], toxigenic Escherichia coli [Citation28], Listeria [Citation29], Bacillus cereus [Citation30], Campylobacter [Citation31], Vibrio cholerae [Citation32], Mycobacterium tuberculosis [Citation33], Yersinia [Citation34], Proteus [Citation35] and Staphylococci [Citation32] but it depends on maintenance of huge stocks of phages and strains. Indeed, while a wealth of information exists regarding various phage typing schemes, these approaches are not commonly utilized for the identification of bacterial pathogens in clinical settings. Despite the availability of numerous studies and reviews that have explored phage diagnosis for specific pathogens, your review serves as a groundbreaking contribution by summarizing the collective research conducted on phage-based diagnostics targeting a wide range of pathogens. By comprehensively covering phage-based diagnostic methods across different sectors and discussing their potential applications for a broad spectrum of bacterial infections, your review offers a unique and comprehensive perspective. This pioneering work highlights the value of phage-based diagnostics in addressing the challenges associated with identifying and managing bacterial pathogens, encompassing a diverse array of industries and environments. Through this review, we have provided an invaluable resource for researchers, clinicians and professionals across various fields, enabling them to understand the potential and implications of phage-based diagnostics in the context of microbial infections that pose significant global health concerns. This review article provides baseline data to summarize significant steps taken in advancing the field of diagnostic methodologies and has the potential to drive further research, innovation, and practical implementation of phage-based approaches () [Citation36].
Figure 1. Traditional and recent approaches of bacteriophages in diagnostics.
Bacteriophage-based methods are employed for bacteria detection, utilizing the unique properties of phages. Phages can infect target bacteria, and in cases of cell lysis, the detection can involve progeny phages or released cell content. Certain phages have the capability to introduce exogenous genes into bacteria, thereby labeling the host with a fluorescent, colorimetric, or optical marker for identification. Additionally, phages can be utilized to construct sensing layers in biosensors. By attaching bacteriophages to micro- or nano-particles, they can serve as capture elements in various assays, contributing to effective and specific bacteria detection methods.
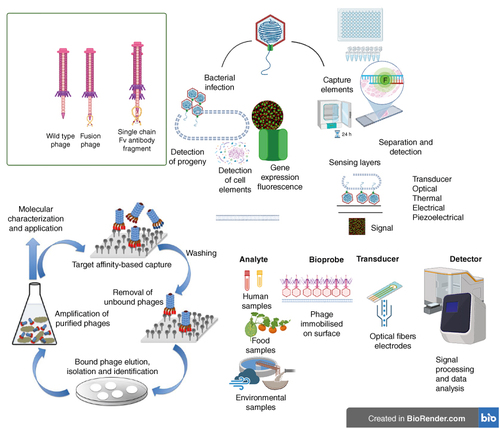
Mycobacterium tuberculosis
Mycobacterium tuberculosis, the causative agent of tuberculosis (TB), poses a significant global health challenge due to delayed diagnosis, misdiagnosis and the emergence of multidrug resistance. Rapid and accurate diagnosis of TB is crucial for effective treatment and the prevention of its spread. Recent advancements in phage-based bioassays have expedited the screening and profiling of antibiotic susceptibility for M. tuberculosis [Citation37].
There are two primary phage-based diagnostic approaches for detecting M. tuberculosis:
▪ | Phage Amplification and Plaque Count: This method involves the amplification of phages that infect M. tuberculosis, followed by quantification of plaques formed by the infected bacteria. This approach has demonstrated high specificity and has been commercialized as PhageTekMB and FASTPlaqueTB [Citation38,Citation39]; | ||||
▪ | Light Detection of Fluorescent Reporter Phages: Another method utilizes fluorescent reporter phages or luciferase reporter phages that emit light in response to TB infection. These assays have also shown high specificity and have been commercialized as diagnostic tools, including the Actiphage™ kit, which allows for TB detection within 6–8 h [Citation40]. Phage RNA can also serve as a biomarker for TB detection [Citation41] Some studies in the literature have reported the use of qPCR-based detection of phage's natural RNA or surrogate marker loci in engineered phages specifically isolated against M. tuberculosis [Citation42,Citation43]. PCR-based phage detection methods offer higher sensitivity and are less prone to false-negative or false-positive results compared with phage amplification-based methods [Citation44]. PCR-based phage detection methods offer higher sensitivity and are less prone to false-negative or false-positive results compared with phage amplification-based methods [Citation45,Citation46]. Fluorescently labeled mycobacteriophages have been utilized to bind with clinical isolates of M. tuberculosis bacteria and drugs of interest, enabling the detection of drug-resistant M. tuberculosis based on fluorescence measurements obtained from flow cytometry and microscopy [Citation47]. The reported sensitivity limit for the flow cytometric approach is 1 colony-forming unit (CFU) per ml, while the microscopic platform achieves a sensitivity limit of 20 CFU/ml [Citation9,Citation48,Citation49]. These innovative approaches hold promise for enhancing the accuracy and speed of TB diagnosis, thereby contributing to improved patient outcomes and public health. Reporter mycobacteriophages utilized in current diagnostic assays have been derived from various phage strains, including L5, D29, TM4 and Che12 [Citation39,Citation50–54]. A recent addition to this list is the derivative Φ2GFP10 of TM4, which has demonstrated the ability to screen low-frequency subpopulations of drug-resistant variants of M. tuberculosis. This approach was successfully applied in both in vitro studies and sputum samples from TB patients in South Africa [Citation45]. In another development, a fluoromycobacteriophage carrying an optimized mCherrybomb gene expression was designed to enable rapid detection of M. tuberculosis and rifampicin resistance. This innovation was tested on sputum samples from TB patients in Brazil, highlighting its potential clinical utility [Citation46,Citation47]. Furthermore, researchers such as Serena et al. have identified new peptides of M. tuberculosis and harnessed phage display peptide libraries to screen IgG antibodies in TB-infected patients. One specific peptide, designated as P.Mt.PD.7, demonstrated significant promise and was adapted for use in enzyme-linked immunosorbent assays (ELISA), exhibiting 100% sensitivity and specificity [Citation55]. A variety of reporter genes have also been incorporated into mycobacteriophages to enhance diagnostic capabilities. These genes include green and yellow fluorescent proteins as well as the protein encoded by the firefly luciferase gene. These genetic modifications result in an output in the form of light emission upon infection, which can be quantified using a luminometer [Citation39,Citation56]. These advancements in reporter mycobacteriophages underscore their potential to revolutionize the field of TB diagnostics by providing rapid and specific detection methods. |
Staphylococcus aureus
The application of fluorescence-labeled phage sensors extends to the detection of bacterial toxins. For example, the phage display technique was harnessed to detect staphylococcal enterotoxin B (SEB), a causative agent of food poisoning. Through this method, a 12-mer peptide was employed to detect SEB, achieving a remarkable sensitivity limit of 1.4 ng of SEB per well [Citation57,Citation58]. This approach was also successfully adapted to detect toxins in the MS2 bacteriophage and Bacillus globigii [Citation58]. In a related study, the efficacy of a cocktail composed of luciferase-labeled bacteriophages was evaluated in human serum. This investigation shed light on the importance of establishing a clinical matrix between bacteria and phage. The presence of IgG antibodies in the serum posed a challenge, hindering the detection of S. aureus strains and dampening the signal produced by phage-encoded luciferase. However, this obstacle was overcome by incorporating recombinant protein A into the phage cocktail, ultimately enabling sensitive detection of S. aureus in serum [Citation59]. These applications underscore the versatility of fluorescence-labeled phage sensors not only in bacterial detection but also in the identification of bacterial toxins. Such innovative techniques hold potential for various diagnostic and surveillance applications, particularly in scenarios involving food safety and clinical settings.
Escherichia coli
The threat posed by foodborne diseases, with E. coli being a significant pathogen, has garnered substantial attention due to their substantial impact on global human health, resulting in approximately 420,000 deaths annually [Citation60]. In the realm of E. coli detection, several studies have explored innovative methods to achieve high sensitivity [Citation61]. Certain investigations have successfully detected pathogenic E. coli at concentrations as low as 102 CFU/ml in contaminated milk and 104 CFU/ml in nutrient broth. These achievements were accomplished through a combination of fluorescent techniques and immunomagnetic separation [Citation62]. Furthermore, a separate study underscored the effectiveness of using fluorescent quantum dots (QDs) to enhance the sensitivity of labeled phages [Citation48]. The core principle of phage-based screening revolves around the inherent consequences of the phage infection cycle, which culminates in the release of progeny phages. This increase in phage concentration within the environment serves as a signal for quantitative bacterial analysis. For example, a phage-amplification assay applied to clinical specimens yielded a specificity of 0.60–0.88 in smear-positive samples [Citation63]. This principle has also been harnessed in quantitative real-time PCR assays, which demonstrated the detection of increased phage progeny in response to E. coli and Brucella infections [Citation23,Citation64]. Intriguingly, some studies have delved into the use of mass spectrometry and liquid chromatography-mass spectrometry to identify proteins and peptides generated as a result of trypsin-based digestion of phages [Citation65]. These innovative approaches collectively highlight the dynamic and versatile nature of phage-based detection methods, which hold great potential for achieving sensitive and accurate bacterial analysis, particularly in the context of foodborne pathogens like E. coli. The utilization of matrix-assisted laser desorption/ionization mass spectrometry (MALDI-MS) was not only employed for bacterial detection, but also extended to determine the susceptibility of Staphylococcus aureus bacteria to antibiotics. However, the detection limits of the aforementioned studies were not explicitly provided [Citation66,Citation67]. In an innovative approach, Tilton et al. employed bacteriophage T7 for the detection of E. coli in water. This method relied on the quantitation of β-galactosidase without the need for bacterial culturing or DNA extraction. Additionally, nanophotonics were incorporated to amplify the fluorescent signal, achieving a sensitivity limit of 10 CFU/ml within 8 h of infection [Citation68]. Building upon this concept, other studies, such as those by Burhman et al. and Chen et al., utilized bacteriophage T4 and magnetic beads coated with T7 phage, respectively, to enable the detection of E. coli through the quantification of β-galactosidase [Citation69,Citation70]. In a contrasting approach, Yang et al. employed a unique methodology involving direct image-based quantitation of released compounds. This approach relied on measuring morphological changes induced by the T7 phage infection cycle [Citation71]. In a recent advancement, a study developed a phage-based assay incorporating a NanoLuc luciferase reporter for the quantification of E. coli in drinking water within the context of Nairobi, Kenya. This assay was integrated with a microfluidic chip platform, providing a comprehensive automated solution for the detection and quantification of E. coli. Impressively, this system achieved a sensitivity limit of ten most probable number (MPN) per 100 ml within 5.5 h, eliminating the need for trained personnel to operate the assay [Citation63]. These studies collectively highlight the diverse and innovative applications of phage-based assays for the detection and quantification of bacterial pathogens, with potential implications for various fields, including water quality monitoring and healthcare. In a recent study conducted in Nairobi, Kenya, a phage-based assay labeled with the NanoLuc luciferase reporter was developed for the quantification of E. coli in drinking water. This innovative approach was integrated with a microfluidic chip platform, resulting in a fully automated solution for the detection and quantification of E. coli. Remarkably, this system achieved a sensitivity limit of ten most probable number (MPN) per 100 ml within 5.5 h, and it operated without the need for trained personnel [Citation72]. Reporter phages have proven valuable for the detection of E. coli O157:H7 due to their host-specific nature and their ability to differentiate viable from nonviable cells. The underlying principle relies on the expression of the NanoLuc luciferase (Nluc) reporter gene from the engineered ΦV10 phage genome following infection. This expression leads to robust bioluminescence, enabling fast, simple, and sensitive detection of E. coli O157:H7 [Citation73]. Another significant development comes from Willford et al., who designed a rapid, sensitive, and specific enzyme-labeled phage-based luminescent assay (Phazyme) targeting five Shiga toxin-producing E. coli serogroups (O26, O127, O123, O111, O145) in water and food samples. The accuracy of this assay was impressive, with sensitivity limits ranging from 105 to 106 CFU/ml in pure culture, and 0.1–1 CFU/g and 102 CFU/100 ml in food and water samples, respectively. Importantly, the results were easily interpretable without the need for complex instrumentation [Citation74]. Furthermore, a phage labeled PP01 with tetracysteine has been utilized for the specific and sensitive detection of E. coli O157:H7. This tagged phage produced a fluorescence signal in viable E. coli O157:H7 cells, which was quantified through microscopy. The sensitivity limit of this approach was as low as 1 CFU/ml in 100 contaminated apple juice [Citation49]. In a different approach, Wang et al. employed a phage-based electrochemical strategy, involving the conjugation of phages with magnetic beads serving as affinity reporter probes for detecting E. coli in drinking water. The signal expression was achieved through a gold binding peptide fused with alkaline phosphatase (GBPs-ALP), enabling the detection of target E. coli through enzymatic activity and binding capacity. The bacterial load was quantified via linear sweep voltammetry-based electrochemical measurement of enzymatic activity. This method exhibited a sensitivity limit of 105 CFU/ml within 4 h or 1 CFU/100 ml of water following a 9-h preincubation [Citation75]. These diverse approaches showcase the versatility of phage-based assays for the detection and quantification of E. coli in various sample types.
Acinetobacter baumannii
Multidrug-resistant A. baumannii presents a significant threat as a leading cause of blood and wound-related infections. Prompt diagnosis of A. baumannii infections is crucial, given that septic shock contributes to 40–80% of ICU patient fatalities. Conventional diagnostic techniques for blood infections, such as blood culturing and PCR, are susceptible to false-negative or false-positive outcomes and are time consuming. There exists a clear imperative to explore alternative diagnostic strategies to address the challenges of accuracy and delay associated with current diagnostic methods. In the current literature, only two studies have reported on the sensitivity and accuracy of phage-based real-time PCR assays for A. baumannii detection. A notable study by Luo et al. harnessed the potential of phage p53, isolated from A. baumannii, in a quantitative PCR (qPCR) approach. This innovative method aimed to detect viable A. baumannii loads by quantifying p53 replication and titer in serum samples. The assay demonstrated remarkable sensitivity, capable of detecting bacterial loads as low as 10 CFU/100 μl serum, with a phage titer of 103 plaque-forming units (PFU) per ml, all achieved within a mere 4-h timeframe. Notably, the assay bypassed the need for DNA isolation, streamlining the diagnostic process [Citation76]. Continuing their innovative research, the same group conducted another study that integrated qPCR with enrichment culture to effectively screen for viable A. baumannii in sputum samples. This approach involved a short-term plate incubation method for bacterial enrichment. Notably, this strategy helped mitigate the interference caused by DNA polymerase present in sputum samples. The study demonstrated the capability to detect A. baumannii at concentrations as low as 1 CFU within a remarkably brief span of 6 h, all without the need for DNA isolation. The significance of this technique was further underscored by its successful application in a clinical scenario. Specifically, the study illustrated the rapid, sensitive, and specific detection of viable A. baumannii in an 80-year-old male patient afflicted with lung infection. This approach offers a promising avenue for the timely and accurate diagnosis of A. baumannii infections, particularly in the context of complex clinical samples such as sputum. The combination of qPCR and enrichment culture addresses both sensitivity and specificity concerns, providing a robust and efficient means of detecting this clinically significant pathogen [Citation77].
Salmonella
Salmonella, a significant foodborne pathogen, is responsible for a staggering 153 million cases of gastroenteritis and approximately 57,000 deaths each year on a global scale [Citation78]. Given the gravity of its impact, accurate and sensitive detection methods for Salmonella are paramount. The established standard requires that Salmonella should not be detectable in 10–25 g of a food sample [Citation79]. Therefore, detection assays for this pathogen are expected to exhibit heightened accuracy and sensitivity, capable of identifying even a single CFU [Citation80]. Time is another critical factor in Salmonella detection, considering that conventional methods often demand nearly 10 days or more to identify certain Salmonella serovars. While molecular methods have expedited the process, enabling Salmonella identification within 24 to 30 h, the results often necessitate confirmation through conventional techniques [Citation81,Citation82]. This indicates a need for improved and more efficient detection methods that can offer both rapid results and a high degree of accuracy. In summary, due to the significant health risks posed by Salmonella and the stringent detection requirements, the development of sensitive, rapid, and accurate detection assays for this pathogen is of utmost importance. Such advancements in detection technology can contribute significantly to preventing foodborne illnesses and enhancing public health. Over the past decade, the utilization of bacteriophages in various detection assays has been a subject of extensive discussion in the literature. Notably, Vinay et al. conducted a compelling study where genetically modified phages were labeled with a fluorescent reporter protein, enabling them to produce a signal upon binding with their bacterial hosts. This innovative approach facilitated the use of a portable flow cytometer for on-site detection, capable of identifying a single bacterium within 1 hour of infection. The study focused on the HK620 prototype of the phage sensor and demonstrated its effectiveness in rapidly and sensitively detecting Salmonella and E. coli TD2158 (the original host) [Citation83]. Additionally, a recombinant bacteriophage assay named phageDx has been shown to be a sensitive and rapid tool for Salmonella detection in lettuce plants. This study encompassed six different Salmonella serovars and revealed that while the detection limit varied by serovar, most strains could be detected within 2 h at a concentration of 100 CFU/ml. Even at a low concentration of 10 CFU/25 g, Salmonella could still be detected within 9 h. Interestingly, the presence of natural microbiota on lettuce plants did not impact the detection of Salmonella [Citation84]. Subsequently, a retrospective validation of the phageDx assay reported consistent detection of Salmonella in powdered infant formula and raw turkey samples, demonstrating its robustness across different sample types. The phageDx assay exhibited high species specificity, detecting 108 Salmonella strains while not infecting 30 non-Salmonella strains. Addressing the challenge of extended enrichment times for sensitive detection, researchers developed luciferase-tagged phages expressing NanoLuc. This strategy aimed to overcome the reported loss of sensitivity in phage-based tests when attempts were made to reduce detection time, an especially critical concern for food samples. The NanoLuc-expressing phages successfully detected 10–100 CFU/ml without the need for enrichment and exhibited a broad host spectrum, identifying 99.3% of 269 tested Salmonella strains. Notably, detection limits were further enhanced to 1 CFU/25 g of ground turkey and 1 CFU/100 g within 7 and 16 h, respectively, with the use of a phage cocktail [Citation85].
Pseudomonas aeruginosa
P. aeruginosa, a pathogen responsible for a range of infections including metabolic disorders, urinary tract infections (UTIs), bloodstream infections, and wound infections, necessitates the development of reliable, rapid and cost-effective detection methods [Citation86,Citation87]. Despite the crucial need, phage-based diagnostics for P. aeruginosa have not received extensive attention in the literature. However, there are a few instances where researchers have explored the potential of phages for diagnosing P. aeruginosa infections. For instance, Harada et al. devised an immobilization strategy involving three lytic phages enclosed within bioreactive porous biopolymeric alginate matrices. This innovative bioreactive hydrogel generated signals, manifesting as color or light, to indicate the presence of viable P. aeruginosa cells in biological fluids or contact fluids, achieving results within a remarkably short span of 3 h [Citation88]. Furthermore, a plaque assay that emerged from molecular and phylogenetic analysis has been reported as an effective means of detecting P. aeruginosa in water by Ezzat and Azam [Citation89]. This assay underscores the significance of molecular techniques in enhancing diagnostic capabilities. In another approach, researchers selected phage clones from M13 phage display libraries based on their binding affinity to P. aeruginosa, E. coli and S. aureus. These phage clones were subsequently covalently attached to magnetic beads, forming phage-bead complexes. This method facilitated the capture and concentration of bacteria from blood samples. The resulting phage-bead complexes enabled sensitive, specific and cost-effective detection of bacteria within 6 h, with an impressive detection limit of 7 CFU/ml. Collectively, while phage-based diagnostics for P. aeruginosa may not have been extensively explored, the studies highlighted showcase innovative strategies that offer promising avenues for the development of effective and efficient diagnostic methods for this pathogen.
Bacillus cereus & Bacillus anthracis
The gamma-phage assay has been extensively explored in the literature as a means of detecting Bacillus anthracis, Bacillus cereus and Bacillus thuringiensis, in addition to other conventional detection methods. These conventional methods often rely on the analysis of colony morphology, capsule production and real-time PCR-based detection of virulence plasmids [Citation90]. The gamma-phage assay stands out due to its simplicity, cost–effectiveness and reliable performance. This method capitalizes on the binding capacity, lytic activity and notably high specificity (97%) of gamma-phages against B. anthracis [Citation83]. This specificity ensures that the gamma-phage assay can accurately distinguish the target pathogen from other organisms, enhancing its utility as a diagnostic tool. By virtue of these attributes, the gamma-phage assay offers a practical and efficient approach for the detection of these Bacillus species. Its ease of use, affordability, and high specificity position it as a valuable alternative to traditional detection methods, providing a promising solution for rapid and accurate identification of these pathogens [Citation91].
Listeria monocytogenes & Listeria innocua
L. monocytogenes is a Gram-positive, rod-shaped, facultative anaerobic bacterium commonly found in food processing facilities, and it is responsible for causing listeriosis [Citation92]. To combat food contamination, listeriophages like Listex™ P100 and ListShield™ have been utilized as biocontrol agents [Citation93,Citation94]. A specific study explored the diagnostic potential of A511:luxAB, a recombinant derivative of a Listeria-specific bacteriophage from the A511 strain. This approach enabled the rapid and convenient detection of viable Listeria cells in environmental and food samples. Detection was facilitated by the bioluminescence produced by infected Listeria cells. The assay exhibited impressive sensitivity, capable of identifying very low contamination levels in foods tainted with L. monocytogenes Scott A cells. The Lux phage-based assay enabled the detection of <1 cell/g in cabbage, chocolate, and cheese samples. Comparative analysis of light emission from phage-treated and untreated control food samples facilitated this detection. In the case of food samples rich in microflora, such as meat and soft cheese, the assay achieved a detection limit of approximately 10 cells/g. Notably, the study demonstrated the superiority of the lux-phage assay, which provided results within 24 h, in contrast to the 4 days required by the standard plating procedure [Citation95]. Regarding the diagnosis of L. innocua, the current literature lacks evidence of available phage-based assays for its detection. However, Schmelcer et al. proposed the utilization of phage endolysins as a promising diagnostic tool for Listeria species [Citation88]. This suggests a potential avenue for further exploration in the realm of L. innocua detection using phage-based approaches [Citation96].
Streptococcus pneumoniae
Costa et al., has reported the use of a phage-based assay for the detection of serotypes of S. pneumoniae [Citation97]. This approach leverages the unique interactions between bacteriophages and different serotypes of the bacterium to facilitate serotype identification. However, the study also highlighted a significant challenge associated with the use of this phage-based assay: the high cost involved in the testing process. This cost factor has posed a limitation on the widespread adoption of the assay, particularly for large-scale batch testing scenarios. While the potential of phage-based assays for serotype detection is evident, the cost issue underscores a practical barrier that needs to be addressed in order to make such assays more accessible and feasible for routine use, especially in scenarios where batch testing is required. As the field of phage-based diagnostics advances, efforts to optimize and streamline the testing process, reduce costs and enhance the scalability of these assays could play a crucial role in expanding their utility for various applications, including serotype detection [Citation98–100].
Haemophilus influenzae & Shigella
While the current literature does not provide evidence of phage utilization for the detection of Haemophilus influenzae and Shigella, it is notable that there has been extensive research into phage-based biocontrol strategies targeting these pathogens. These efforts have primarily focused on using phages as biocontrol agents to combat and reduce the populations of Haemophilus influenzae and Shigella bacteria. Phage-based biocontrol involves employing bacteriophages to specifically target and lyse pathogenic bacteria, thereby mitigating their impact. This approach has garnered significant attention as a potential solution for addressing infections caused by H. influenzae and Shigella, especially in contexts where traditional antibiotics may be less effective due to resistance or other factors. Although the use of phages for detecting these specific pathogens may not have been extensively explored in the literature, it is possible that ongoing research could explore the diagnostic potential of phages against H. influenzae and Shigella in the future. The field of phage-based diagnostics is continuously evolving, and novel applications may emerge as researchers delve deeper into the interactions between phages and bacterial pathogens [Citation101,Citation102].
Challenges, future directions & recommendations
The emerging field of phage-based diagnosis has predominantly focused on Mycobacterium detection through extensive discussions and assays. However, there exists a notable gap in research pertaining to the diagnostic potential of phages against other bacterial species. The comprehensive findings underline a significant challenge: the risk of both false-positive and false-negative results. This challenge has been particularly evident in PhaB and FASTPlaqueTB assays used for Mycobacterium detection in respiratory samples. Moreover, receptor phage-based assays have demonstrated a limited host range, often linked to phage resistance. Additional complexity arises from the impact of food matrices, which can affect assay accuracy. To address these challenges, the utilization of amplified reporter proteins has proven effective. This approach offers a viable solution to circumvent the earlier mentioned issues. In the therapeutic context, the challenges of host range and resistance can be mitigated through the use of phage cocktails and combinations with antibiotics. Encouragingly, recent reports on engineered and/or modified phages instil optimism, suggesting the potential for cost-effective, sensitive, and specific diagnostic options. The integration of phage-based diagnostics with multidisciplinary fields like nanotechnology and biotechnology presents an opportunity to address these challenges. Notably, the deployment of phage nanoprobe M13 has enabled sensitive virus detection. Recognizing the gaps in existing literature, there is a clear imperative for further research. Timely detection of bacterial pathogens in areas such as food production, healthcare, and agricultural supply chains holds the promise of preventing infection transmission and curbing substantial economic losses. While these phage-based diagnostic technologies have yet to achieve commercial and routine clinical implementation, the trajectory suggests promise. Additional efforts are required to validate the potential of phages in diagnostics. As this technology matures, it has the potential to revolutionize disease detection, ultimately contributing to improved public health and economic stability.
Conclusion
Bacteriophages, commonly referred to as phages, exist ubiquitously across various environments, displaying a remarkable presence in nature. Their abundance is a testament to their widespread distribution. Beyond their sheer prevalence, phages function as both predators and catalysts for bacterial evolution. Their inherent ability to initiate infection cycles within bacteria underscores their substantial potential as both biocontrol agents and invaluable tools for diagnostics. Phages' role as natural predators of bacteria contributes to the regulation of bacterial populations in diverse ecosystems. Moreover, their capacity to drive evolutionary changes within bacterial populations has far-reaching implications for shaping microbial communities and their adaptations over time. Importantly, the innate capability of phages to kickstart infection processes within bacteria positions them as promising candidates for biocontrol strategies. This potential is rooted in their capacity to selectively target and eliminate specific bacterial strains, offering a means to combat harmful pathogens while minimizing the disruption of beneficial microbial populations. Furthermore, the dynamic interplay between phages and bacteria serves as a foundation for their application in diagnostics. Leveraging phages as diagnostic tools capitalizes on their distinct interactions with bacterial hosts, enabling rapid and precise detection of pathogenic microorganisms. This capacity holds great promise for revolutionizing disease detection and surveillance, with implications spanning clinical, environmental and agricultural domains. In summation, the intricate relationship between bacteriophages and bacteria underscores their multifaceted potential, extending from biocontrol interventions to transformative diagnostic applications.
Future perspective
Due to the method's target specificity and the flexibility of genome editing and chemical modification, phage-based diagnostics offer distinct advantages over other quantitative technologies like ELISA and PCR. This versatility positions them for application in various multidisciplinary contexts. The promising integration of synthetic biology and phage genome engineering offers exciting prospects for reprogramming phage host ranges and functions, as well as isolating functional proteins for synthetic manufacturing and precise control of phage development in detection/diagnostic applications. The field could benefit from automation to minimize human sample handling errors and facilitate point-of-use applications. Combining synthetic biology with automated processes for sample processing, detection and reporting represents a significant advancement in biosensor development. A biosensor with integrated phage detection allows for phage-based strategies to be applied outside laboratory settings, reducing reporting times and enabling real-time tracking of pathogen transmission. This real-time identification can prevent costly procedures like product recall and extensive contamination investigations, potentially saving millions of lives in future. Phage display, with its ability for the rapid isolation of specific ligands, holds numerous advantages across various applications. It is instrumental in selecting inhibitors for both the active and allosteric sites of enzymes, as well as identifying receptor agonists, antagonists, and modulatory peptides for G-protein binding. Additionally, phage display finds utility in epitope mapping and the analysis of protein–protein interactions. Therefore, the ligands isolated from phage libraries will be playing crucial roles in diagnostics and therapeutic target validation, drug design, and vaccine development. Furthermore, phage display will be effectively combined with other methods. With past innovations and ongoing advancements, the future of this field looks very promising.
Author contributions
B Wahid – analysis of literature data, drafted the manuscript. MS Tiwana – significant contribution to reanalysis of literature survey, data, proof-reading and preparation of draft. Drafted the work, revised it critically for important intellectual content.
Financial disclosure
The authors have no financial involvement with any organization or entity with a financial interest in or financial conflict with the subject matter or materials discussed in the manuscript. This includes employment, consultancies, honoraria, stock ownership or options, expert testimony, grants or patents received or pending, or royalties.
Competing interests disclosure
The authors have no competing interests or relevant affiliations with any organization or entity with the subject matter or materials discussed in the manuscript. This includes employment, consultancies, honoraria, stock ownership or options, expert testimony, grants or patents received or pending, or royalties.
Writing disclosure
No writing assistance was utilized in the production of this manuscript.
References
- McNally L, Brown SP. Building the microbiome in health and disease: niche construction and social conflict in bacteria. Philos. Trans. R. Soc. B 2015;370(1675):20140298.
- Falkow S. Who speaks for the microbes? Emerg. Infect. Dis. 1998;4(3):495.
- Graham DW, Bergeron G, Bourassa MW et al. Complexities in understanding antimicrobial resistance across domesticated animal, human, and environmental systems. Ann. NY Acad. Sci. 2019;1441(1):17–30.
- Van Boeckel TP, Pires J, Silvester R et al. Global trends in antimicrobial resistance in animals in low-and middle-income countries. Science 2019;365(6459):eaaw1944.
- Van Boeckel TP, Brower C, Gilbert M et al. Global trends in antimicrobial use in food animals. Proc. Natl Acad. Sci. USA 2015;112(18):5649–5654.
- Murray CJ, Ikuta KS, Sharara F et al. Global burden of bacterial antimicrobial resistance in 2019: a systematic analysis. Lancet 2022;399(10325):629–655.
- Schirrmann T, Meyer T, Schütte M et al. Phage display for the generation of antibodies for proteome research, diagnostics and therapy. Molecules 2011;16(1):412–426.
- Daubie V, Chalhoub H, Blasdel B et al. Determination of phage susceptibility as a clinical diagnostic tool: a routine perspective. Front. Cell. Infect. Microbiol. 2022;12:1000721.
- Farooq U, Wajid Ullah M, Yang Q, Wang S. Applications of phage-based biosensors in the diagnosis of infectious diseases, food safety, and environmental monitoring. Biosens. Environ. Monit. 2019;12:1–18.
- Bahara NHH, Tye GJ, Choong YS et al. Phage display antibodies for diagnostic applications. Biologicals 2013;41(4):209–216.
- Wang L-F, Yu M. Epitope identification and discovery using phage display libraries: applications in vaccine development and diagnostics. Curr. Drug Targ. 2004;5(1):1–15.
- Roth KDR, Wenzel EV, Ruschig M et al. Developing recombinant antibodies by phage display against infectious diseases and toxins for diagnostics and therapy. Front. Cell. Infect. Microbiol. 2021;11:697876.
- Azzazy HM, Highsmith WE Jr.. Phage display technology: clinical applications and recent innovations. Clin. Biochem. 2002;35(6):425–445.
- Deutscher SL. Phage display in molecular imaging and diagnosis of cancer. Chem. Rev. 2010;110(5):3196–3211.
- Smith GP. Filamentous fusion phage: novel expression vectors that display cloned antigens on the virion surface. Science 1985;228(4705):1315–1317.
- Jamal M, Bukhari SM, Andleeb S et al. Bacteriophages: an overview of the control strategies against multiple bacterial infections in different fields. J. Basic Microbiol. 2019;59(2):123–133.
- Yue H, He Y, Fan E et al. Label-free electrochemiluminescent biosensor for rapid and sensitive detection of pseudomonas aeruginosa using phage as highly specific recognition agent. Biosens. Bioelectron. 2017;94:429–432.
- Park M-K, Li S, Chin BA. Detection of Salmonella typhimurium grown directly on tomato surface using phage-based magnetoelastic biosensors. Food Bioproc. Technol. 2013;6(3):682–689.
- Edgar R, McKinstry M, Hwang J et al. High-sensitivity bacterial detection using biotin-tagged phage and quantum-dot nanocomplexes. Proc. Natl Acad. Sci. USA 2006;103(13):4841–4845.
- Kawakamii S, Ono Y, Miyazawa Y. Consider the advantages and disadvantages of microbial examinations in a hospital, and ideal microbial laboratory. Rinsho Byori Japan. J. Clin. Pathol. 2011;59(10):940–943.
- Garibyan L, Avashia N. Research techniques made simple: polymerase chain reaction (PCR). J. Investig. Dermatol. 2013;133(3):e6.
- Sell TL, Schaberg DR, Fekety FR. Bacteriophage and bacteriocin typing scheme for Clostridium difficile. J. Clin. Microbiol. 1983;17(6):1148–1152.
- Sergueev KV, Filippov AA, Nikolich MP. Highly sensitive bacteriophage-based detection of Brucella abortus in mixed culture and spiked blood. Viruses 2017;9(6):144.
- Caprioli T, Zaccour F, Kasatiya S. Phage typing scheme for group D streptococci isolated from human urogenital tract. J. Clin. Microbiol. 1975;2(4):311–317.
- Hickman-Brenner F, Stubbs A, Farmer J 3rd. Phage typing of Salmonella enteritidis in the United States. J. Clin. Microbiol. 1991;29(12):2817–2823.
- Castro D, Morińigo M, Martinez-Manzanares E et al. Development and application of a new scheme of phages for typing and differentiating Salmonella strains from different sources. J. Clin. Microbiol. 1992;30(6):1418–1423.
- Slopek S, Przondo-Hessek A, Mulczyk M. New scheme of phage typing of Shigella flexneri. Arch. Roumain. de Patholog. Experiment. et de Microbiolog. 1969;28(4):974–975.
- Khakhria R, Duck D, Lior H. Extended phage-typing scheme for Escherichia coli 0157: h7. Epidemiol. Infect. 1990;105(3):511–520.
- Loessner MJ, Busse M. Bacteriophage typing of Listeria species. Appl. Environ. Microbiol. 1990;56(6):1912–1918.
- Ahmed R, Sankar-Mistry P, Jackson S et al. Bacillus cereus phage typing as an epidemiological tool in outbreaks of food poisoning. J. Clin. Microbiol. 1995;33(3):636–640.
- Khakhria R, Lior H. Extended phage-typing scheme for Campylobacter jejuni and Campylobacter coli. Epidemiol. Infect. 1992;108(3):403–414.
- Chakrabarti A, Ghosh A, Nair GB et al. Development and evaluation of a phage typing scheme for Vibrio cholerae O139. J. Clin. Microbiol. 2000;38(1):44–49.
- McNerney R. TB: the return of the phage. A review of fifty years of mycobacteriophage research. Inter. J. Tubercul. Lung Dis. 1999;3(3):179–184.
- Baker P, Farmer J 3rd. New bacteriophage typing system for Yersinia enterocolitica, Yersinia kristensenii, Yersinia frederiksenii, and Yersinia intermedia: correlation with serotyping, biotyping, and antibiotic susceptibility. J. Clin. Microbiol. 1982;15(3):491–502.
- Sekaninová G, Rychlík I, Kolářová M et al. A new bacteriophage typing scheme for Proteus mirabilis and Proteus vulgaris strains: 3. Analys. Lytic Propert. Folia Microbiol. 1998;43:136–140.
- Singh A, Poshtiban S, Evoy S. Recent advances in bacteriophage based biosensors for food-borne pathogen detection. Sensors 2013;13(2):1763–1786.
- Keeler E, Perkins MD, Small P et al. Reducing the global burden of tuberculosis: the contribution of improved diagnostics. Nature 2006;444(Suppl. 1):49–57.
- McNerney R, Kambashi BS, Kinkese J et al. Development of a bacteriophage phage replication assay for diagnosis of pulmonary tuberculosis. J. Clin. Microbiol. 2004;42(5):2115–2120.
- Hazbón MH, Guarín N, Ferro BE et al. Photographic and luminometric detection of luciferase reporter phages for drug susceptibility testing of clinical Mycobacterium tuberculosis isolates. J. Clin. Microbiol. 2003;41(10):4865–4869.
- Beinhauerova M, Slana I. Phage amplification assay for detection of mycobacterial infection: a review. Microorganisms. 2021;9(2):237.
- Malagon F, Estrella LA, Stockelman MG et al. Phage-mediated molecular detection (PMMD): a novel rapid method for phage-specific bacterial detection. Viruses 2020;12(4):435.
- Mulvey MC, Lemmon M, Rotter S et al. Optimization of a nucleic acid-based reporter system to detect Mycobacterium tuberculosis antibiotic sensitivity. Antimicrob. Agents Chemother. 2015;59(1):407–413.
- Mulvey MC, Sacksteder KA, Einck L, Nacy CA. Generation of a novel nucleic acid-based reporter system to detect phenotypic susceptibility to antibiotics in Mycobacterium tuberculosis. MBio. 2012;3(2):e00312–11.
- Wang X, Li X, Liu S et al. Ultrasensitive detection of bacteria by targeting abundant transcripts. Scient. Reports 2016;6(1):20393.
- O'Donnell MR, Larsen MH, Brown TS et al. Early detection of emergent extensively drug-resistant tuberculosis by flow cytometry-based phenotyping and whole-genome sequencing. Antimicrob. Agents Chemother. 2019;63(4):e01834–18.
- Urdániz E, Rondón L, Martí MA et al. Rapid whole-cell assay of antitubercular drugs using second-generation Fluoromycobacteriophages. Antimicrob. Agents Chemother. 2016;60(5):3253–3256.
- Rondón L, Urdániz E, Latini C et al. Fluoromycobacteriophages can detect viable Mycobacterium tuberculosis and determine phenotypic rifampicin resistance in 3–5 days from sputum collection. Front. Microbiol. 2018;9:1471.
- Yim PB, Clarke ML, McKinstry M et al. Quantitative characterization of quantum dot-labeled lambda phage for Escherichia coli detection. Biotechnol. Bioeng. 2009;104(6):1059–1067.
- Wu L, Song Y, Luan T et al. Specific detection of live Escherichia coli O157: h7 using tetracysteine-tagged PP01 bacteriophage. Biosens. Bioelectron. 2016;86:102–108.
- Ford M, Stenstrom C, Hendrix R, Hatfull G. Mycobacteriophage TM4: genome structure and gene expression. Tubercl. Lung Dis. 1998;79(2):63–73.
- Jacobs WR Jr, Barletta RG, Udani R et al. Rapid assessment of drug susceptibilities of Mycobacterium tuberculosis by means of luciferase reporter phages. Science 1993;260(5109):819–822.
- Kumar V, Loganathan P, Sivaramakrishnan G et al. Characterization of temperate phage Che12 and construction of a new tool for diagnosis of tuberculosis. Tuberculosis 2008;88(6):616–623.
- Pearson RE, Jurgensen S, Sarkis GJ et al. Construction of D29 shuttle phasmids and luciferase reporter phages for detection of mycobacteria. Gene 1996;183(1–2):129–136.
- Sarkis GJ, Jacobs WR Jr, Hatfulll GF. L5 luciferase reporter mycobacteriophages: a sensitive tool for the detection and assay of live mycobacteria. Mol. Microbiol. 1995;15(6):1055–1067.
- Serena NN, Boschero RA, Hospinal-Santiani M et al. High-performance immune diagnosis of tuberculosis: use of phage display and synthetic peptide in an optimized experimental design. J. Immunol. Methods 2022;503:113242.
- Riska PF, Su Y, Bardarov S et al. Rapid film-based determination of antibiotic susceptibilities of Mycobacterium tuberculosis strains by using a luciferase reporter phage and the Bronx Box. J. Clin. Microbiol. 1999;37(4):1144–1149.
- Goldman ER, Pazirandeh MP, Mauro JM et al. Phage-displayed peptides as biosensor reagents. J. Mol. Recogn. 2000;13(6):382–387.
- Rowe CA, Tender LM, Feldstein MJ et al. Array biosensor for simultaneous identification of bacterial, viral, and protein analytes. Anal. Chem. 1999;71(17):3846–3852.
- Brown M, Hall A, Zahn H et al. Bacteriophage-based detection of Staphylococcus aureus in human serum. Viruses 2022;14(8):1748.
- Organization WH. WHO estimates of the global burden of foodborne diseases: foodborne disease burden epidemiology reference group 2007–2015 2015.
- Goodridge L, Chen J, Griffiths M. The use of a fluorescent bacteriophage assay for detection of Escherichia coli O157: h7 in inoculated ground beef and raw milk. Inter. J. Food Microbiol. 1999;47(1–2):43–50.
- Goodridge L, Chen J, Griffiths M. Development and characterization of a fluorescent-bacteriophage assay for detection of Escherichia coli O157: h7. Appl. Environ. Microbiol. 1999;65(4):1397–1404.
- Kalantri S, Pai M, Pascopella L et al. Bacteriophage-based tests for the detection of Mycobacterium tuberculosis in clinical specimens: a systematic review and meta-analysis. BMC Infect. Dis. 2005;5:1–13.
- Anany H, Brovko L, El Dougdoug NK et al. Print to detect: a rapid and ultrasensitive phage-based dipstick assay for foodborne pathogens. Analyt. Bioanalyt. Chem. 2018;410:1217–1230.
- Rees JC, Pierce CL, Schieltz DM, Barr JR. Simultaneous identification and susceptibility determination to multiple antibiotics of Staphylococcus aureus by bacteriophage amplification detection combined with mass spectrometry. Anal. Chem. 2015;87(13):6769–6777.
- Rees JC, Barr JR. Detection of methicillin-resistant Staphylococcus aureus using phage amplification combined with matrix-assisted laser desorption/ionization mass spectrometry. Analyt. Bioanalyt. Chem. 2017;409:1379–1386.
- Richter Ł, Janczuk-Richter M, Niedziółka-Jönsson J et al. Recent advances in bacteriophage-based methods for bacteria detection. Drug Discov. Today 2018;23(2):448–455.
- Tilton L, Das G, Yang X et al. Nanophotonic device in combination with bacteriophages for enhancing detection sensitivity of Escherichia coli in simulated wash water. Analyt. Lett. 2019;52(14):2203–2213.
- Burnham S, Hu J, Anany H et al. Towards rapid on-site phage-mediated detection of generic Escherichia coli in water using luminescent and visual readout. Analyt. Bioanalyt. Chem. 2014;406:5685–5693.
- Chen J, Alcaine SD, Jiang Z et al. Detection of Escherichia coli in drinking water using T7 bacteriophage-conjugated magnetic probe. Anal. Chem. 2015;87(17):8977–8984.
- Yang X, Wisuthiphaet N, Young GM, Nitin N. Rapid detection of Escherichia coli using bacteriophage-induced lysis and image analysis. PLOS ONE 2020;15(6):e0233853.
- Alonzo LF, Jain P, Hinkley T et al. Rapid, sensitive, and low-cost detection of Escherichia coli bacteria in contaminated water samples using a phage-based assay. Scient. Rep. 2022;12(1):7741.
- Zhang D, Coronel-Aguilera CP, Romero PL et al. The use of a novel NanoLuc-based reporter phage for the detection of Escherichia coli O157: h7. Scient. Rep. 2016;6(1):33235.
- Willford JD, Bisha B, Bolenbaugh KE, Goodridge LD. Luminescence based enzyme-labeled phage (Phazyme) assays for rapid detection of Shiga toxin producing Escherichia coli serogroups. Bacteriophage 2011;1(2):101–110.
- Wang D, Hinkley T, Chen J et al. Phage based electrochemical detection of Escherichia coli in drinking water using affinity reporter probes. Analyst 2019;144(4):1345–1352.
- Luo J, Jiang M, Xiong J et al. Exploring a phage-based real-time PCR assay for diagnosing Acinetobacter baumannii bloodstream infections with high sensitivity. Analyt. Chim. Acta 2018;1044:147–153.
- Luo J, Jiang M, Xiong J et al. Rapid ultrasensitive diagnosis of pneumonia caused by Acinetobacter baumannii using a combination of enrichment and phage-based qPCR assay. 2020;8:147–153.
- de Aquino NSM, de Oliveira Elias S, Gomes LVA, Tondo EC. Phage-based assay for the detection of Salmonella in Brazilian poultry products. J. Food Sci. Nutr. Res. 2021;4(3):249–258.
- Kuipers EJ. Encyclopedia of Gastroenterology. Elsevier; 2019.
- Polese P, Del Torre M, Venir E, Stecchini ML. A simplified modelling approach established to determine the Listeria monocytogenes behaviour during processing and storage of a traditional (Italian) ready-to-eat food in accordance with the European Commission Regulation N° 2073/2005. Food Control. 2014;36(1):166–173.
- Foods NACoMCf. Response to questions posed by the food safety and inspection service regarding Salmonella control strategies in poultry. J. Food Protect. 2019;82(4):645–668.
- Heymans R, Vila A, van Heerwaarden CA et al. Rapid detection and differentiation of Salmonella species, Salmonella Typhimurium and Salmonella Enteritidis by multiplex quantitative PCR. PLOS ONE 2018;13(10):e0206316.
- Vinay M, Franche N, Grégori G et al. Phage-based fluorescent biosensor prototypes to specifically detect enteric bacteria such as E. coli and Salmonella enterica Typhimurium. PLOS ONE 2015;10(7):e0131466.
- de Aquino NSM, Elias SdO, Tondo EC. Evaluation of PhageDX Salmonella Assay for Salmonella detection in hydroponic curly lettuce. Foods 2021;10(8):1795.
- Nguyen MM, Gil J, Brown M et al. Accurate and sensitive detection of Salmonella in foods by engineered bacteriophages. Scient. Rep. 2020;10(1):17463.
- Krithiga N, Viswanath KB, Vasantha V, Jayachitra A. Specific and selective electrochemical immunoassay for Pseudomonas aeruginosa based on pectin–gold nano composite. Biosens. Bioelectron. 2016;79:121–129.
- Pastells C, Pascual N, Sanchez-Baeza F, Marco M-P. Immunochemical determination of pyocyanin and 1-hydroxyphenazine as potential biomarkers of Pseudomonas aeruginosa infections. Anal. Chem. 2016;88(3):1631–1638.
- Harada LK, Júnior WB, Silva EC et al. Bacteriophage-based biosensing of Pseudomonas aeruginosa: an integrated approach for the putative real-time detection of multi-drug-resistant strains. Biosensors 2021;11(4):124.
- Ezzat SM, Azzam MI. An approach using a novel phage mix for detecting Pseudomonas aeruginosa in water. Water Environm. J. 2020;34(2):189–202.
- Hoffmaster AR, Meyer RF, Bowen MP et al. Evaluation and validation of a real-time polymerase chain reaction assay for rapid identification of Bacillus anthracis. 2002.
- Kolton CB, Podnecky NL, Shadomy SV et al. Bacillus anthracis gamma phage lysis among soil bacteria: an update on test specificity. BMC Res. Notes 2017;10(1):1–6.
- Jeffs E, Williman J, Brunton C et al. The epidemiology of listeriosis in pregnant women and children in New Zealand from 1997 to 2016: an observational study. BMC Public Health 2020;20(1):1–8.
- Kawacka I, Olejnik-Schmidt A, Schmidt M, Sip A. Effectiveness of phage-based inhibition of Listeria monocytogenes in food products and food processing environments. Microorganisms 2020;8(11):1764.
- Gutiérrez D, Rodríguez-Rubio L, Fernández L et al. Applicability of commercial phage-based products against Listeria monocytogenes for improvement of food safety in Spanish dry-cured ham and food contact surfaces. Food Control. 2017;73:1474–1482.
- Loessner MJ, Rudolf M, Scherer S. Evaluation of luciferase reporter bacteriophage A511:: luxAB for detection of Listeria monocytogenes in contaminated foods. Appl. Environ. Microbiol. 1997;63(8):2961–2965.
- Schmelcher M, Shabarova T, Eugster MR et al. Rapid multiplex detection and differentiation of Listeria cells by use of fluorescent phage endolysin cell wall binding domains. Appl. Environ. Microbiol. 2010;76(17):5745–5756.
- Costa SP, Dias NM, Melo LD et al. A novel flow cytometry assay based on bacteriophage-derived proteins for Staphylococcus detection in blood. Scient. Rep. 2020;10(1):6260.
- Zhou J, Zhou D, Chen W et al. Study on the clinical application of Streptococcus pneumoniae serotype detection based on MALDI-TOF MS technology. Food Sci. Technol. 2022;23:42.
- Llull D, López R, García E. Characteristic signatures of the lytA gene provide a basis for rapid and reliable diagnosis of Streptococcus pneumoniae infections. J. Clin. Microbiol. 2006;44(4):1250–1256.
- Cardaci A, Papasergi S, Midiri A et al. Protective activity of Streptococcus pneumoniae Spr1875 protein fragments identified using a phage displayed genomic library. PLOS ONE 2012;7(5):e36588.
- Shahin K, Bouzari M. Bacteriophage application for biocontrolling Shigella flexneri in contaminated foods. J. Food Sci. Technol. 2018;55:550–559.
- Mallick B, Mondal P, Dutta M. Morphological, biological, and genomic characterization of a newly isolated lytic phage Sfk20 infecting Shigella flexneri, Shigella sonnei, and Shigella dysenteriae1. Scient. Rep. 2021;11(1):19313.