Abstract
Molecular characterization of tumors has shifted cancer treatment strategies away from nonspecific cytotoxic treatment of histology-specific tumors toward targeting of actionable mutations that can be found across multiple cancer types. The development of high-throughput technologies such as next-generation sequencing, combined with decision support applications and availability of patient databases, has provided tools that optimize disease management. Precision oncology has proven success in improving outcomes and quality of life, as well as identifying and overcoming mechanisms of drug resistance and relapse. Addressing challenges that impede its use will improve matching of therapies to patients. Here we review the current status of precision oncology medicine, emphasizing its impact on patients – what they understand about precision oncology medicine and their hopes for the future.
Lay abstract
Precision oncology offers individualized treatment of cancer on a per-patient basis, based on the unique DNA fingerprint of a patient’s cancer. New, advanced technologies for DNA sequencing have led to rapid advancement in developing novel therapies. Decision-making tools have followed pace, leading to improved matching of therapy to patient, ultimately improving outcomes (including quality of life), building trust between patient and physician, and increasing hope for the future.
Overview of precision oncology medicines
New diagnostic technologies and bioinformatics tools have contributed to the knowledge base of cancer biology, heralding a new era of precision oncology medicine. Growing interest in identifying and characterizing genomic biomarkers has led to a paradigm shift away from nonspecific cytotoxic treatment strategies toward personalized treatment strategies based on the genomic profile of a tumor [Citation1]. The value of these therapies may be optimized through correct matching of targets and improving access for patients who are likely to benefit from precision oncology approaches. The discovery of ‘actionable’ mutations, in combination with new technologies that promote rapid and high-volume testing, has contributed to the growing enthusiasm from patients and physicians alike. Molecular testing enables the classification and diagnosis of cancers with greater precision, potentially improving prognosis, therapeutic decision-making and outcomes, while sparing patients from treatments that may confer less clinical benefit. Longitudinal monitoring can further predict treatment resistance and potential for relapse.
provides a summary of select genomic markers of interest for which targeted therapies have been developed and approved by the US FDA and/or EMA for the treatment of solid tumors [Citation2,Citation3]. While some are approved for both tumor-specific and tumor-agnostic indications (i.e., genomic alterations that span multiple tumor types), new therapies continue to be developed, many having reached clinical stages of testing. For tumor-specific mutations, genomically/molecularly targeted agents have received regulatory approval or marketing authorisation in the US and EU for the following indications: EGFR-mutated non-small-cell lung cancer (NSCLC); ALK fusion-positive NSCLC and anaplastic large cell lymphoma; ROS1-positive NSCLC; BRAF V600 mutation-positive melanoma, thyroid cancer and NSCLC; KRAS G12C mutation in NSCLC, FGFR2-rearranged or fusion-positive cholangiocarcinoma or FGFR2/3-altered urothelial cancer; metastatic RET alteration-positive NSCLC and thyroid cancers; KIT/PDGFRA-positive gastrointestinal stromal tumors; HER2-overexpressing breast cancers; and homogolous recombination repair gene-mutated metastatic castration-resistant prostate cancers () [Citation2,Citation3]. While most approvals are for advanced or metastatic disease, a shift is anticipated to earlier lines of therapy, where precision oncology medicines have also demonstrated activity.
Table 1. Select precision oncology targets or biomarkers with matched agents that are approved or in clinical development for solid tumors.
Recent approvals of tumor-agnostic therapies represent another milestone in cancer research, upending the widely held belief that pharmacological approaches to cancer management must be tumor-specific. The finding that the high response rates to genomically targeted therapies could be achieved across multiple tumor types has been practice-changing. Three agents have been approved in the USA and two in the EU in tumor-agnostic indications () [Citation2,Citation3]: larotrectinib for solid tumors harboring an NTRK gene fusion (USA/EU), pembrolizumab for patients with microsatellite instability-high (MSI-H) / mismatch repair-deficient (dMMR) and tumor mutational burden-high solid tumors (USA) and entrectenib for solid tumors harboring an NTRK gene fusion (USA/EU).
Addressing challenges that currently impede the use of precision oncology will improve effective matching of therapies to patient, with the hope of improving treatment outcomes as well as quality of life (QoL). This review provides a summary of the current clinical perspective of precision oncology medicine, with an emphasis on its patient impact beyond efficacy and safety outcomes alone. A patient advocate’s perspective offers insight into patients’ understanding and perception about precision oncology medicine and their expectations and hopes for the future.
Next-generation sequencing
Next-generation sequencing (NGS) is a high-throughput technology that offers several advantages over other diagnostic tests. While PCR, RT-PCR and FISH have proven to be robust and comparably cheap tools for detecting genomic alterations, they are limited by requirements for specific primers or probes for a limited number of targets. In contrast, NGS allows analysis of multiple targets, using advanced sequencing techniques in an efficient manner [Citation21–23]. By providing a more comprehensive tumor profile per individual patient, NGS allows clinicians to select therapies based on detailed diagnoses and tumor characteristics. Identification of specific molecular signatures via NGS is expected to aid in effective matching of therapy to patient, thus reducing and/or avoiding unnecessary delays to treatment [Citation24–26]. Another advantage of NGS-based technology is that it is sensitive enough to be used on liquid biopsies, allowing minimally invasive testing for cancer genomic status based on circulating tumor DNA. Liquid biopsy has been used in many clinical applications, including selection of molecularly targeted drugs, prediction of recurrence by detection of residual cancer, and early cancer diagnosis of asymptomatic patients [Citation27].
NGS options include small to large gene panels, whole-exome sequencing (WES), whole-genome sequencing and focused or whole-transcriptome sequencing. In pediatric oncology, this has been developed largely for patients with incurable malignancies through research programs, such as MAPPYACTS and INFORM in Europe [Citation28]. Currently, targeted gene panels are the most commonly used method in the adult oncology setting due to lower costs, faster turnaround times and high coverage of genomic regions of interest, as well as their sensitivity for detecting lower-level molecular alterations and ability to focus on clinically actionable alterations [Citation29]. At the other end of the NGS spectrum is WES/whole-genome sequencing – currently the gold standard for assessing tumor mutational burden [Citation30]. However, these comprehensive sequencing approaches are more costly, resource-heavier and slower, making them currently unsuitable for broad routine clinical application.
Cost–effectiveness analyses for NGS are limited and have shown mixed findings [Citation31], but are anticipated to shift the balance in its favor, as the unveiling of more biomarkers will justify the cost of a single test that detects multiple targets, vs multiple tests that detect few targets. Therefore, although approaches for NGS testing and reimbursement currently vary by tumor type and by country, NGS is likely to become standardized in the near future and will have broad applicability across tumors.
Using precision oncology to match therapies
Precision oncology has enabled identification of patients who are likely to respond to EGFR-targeted therapy, including those who have developed resistance to other drugs. By understanding the biological basis for such resistance, new approaches to targeted therapy have been uncovered, such as through combination therapies that are capable of dual targeting. For example, mutations in BRAF, whose encoded protein is part of a signaling cascade triggered by activation of the EGFR receptor, have negative predictive value for treatment with EGFR-targeted therapies (). Approximately 50% of melanomas harbor activating BRAF mutations, over 90% of which are V600E substitutions [Citation32]. These observations prompted the investigation of BRAF inhibitors (e.g., vemurafenib, dabrafenib) in patients with melanoma. Acquired resistance to BRAF inhibitors, in turn, was linked to mutations in MEK1/2 and other MAPK pathway genes [Citation33]. Combination of BRAF inhibitors with MEK inhibition was subsequently evaluated and found to have superior efficacy over BRAF inhibitor monotherapy in several BRAF V600 mutation-positive cancers [Citation34–36]. The combination of the BRAF inhibitor dabrafenib with the MEK inhibitor trametinib has since received FDA and EMA approval in BRAF V600 mutation-positive melanoma, NSCLC and thyroid cancer [Citation2,Citation3]. Novel combinations of BRAF inhibitors with PI3K inhibitors are being further explored, with promising preliminary results, both preclinically and in early-stage clinical trials [Citation37–39].
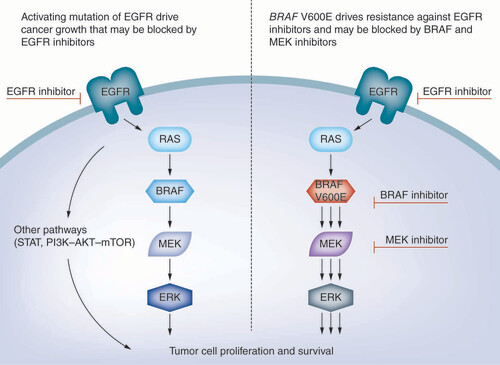
In another example, ALK-TKI resistance in ALK-positive NSCLC has been attributed to mutations in the ALK gene itself or activation of compensatory alternative oncogenic drivers (e.g., MET, EGFR, KRAS, KIT), giving rise to new strategies to overcome resistance mechanisms [Citation12,Citation40]. These have been identified through repeat biopsies of patients who developed resistance, underscoring the importance of longitudinal tracking of tumors – for both identifying targetable resistance mechanisms and monitoring for relapse [Citation41,Citation42]. Precision oncology therefore enables detection of acquired or existing mutations that contribute to resistance, providing valuable information that informs when certain therapies will fail, while pinpointing others that have greater potential for clinical benefit.
In contrast to adult cancers, very few targeted agents have been approved for treatment of pediatric solid tumors. Patients with actionable targets are encouraged to participate in early-phase trials, including academic platform trials, such as pediatric NCI-MATCH in the USA and ESMART in Europe [Citation43]. Clinical trials for the three FDA- and EMA-approved tumor-agnostic medicines with indications in children (pembrolizumab, larotrectinib) and patients aged >12 years (entrectinib, pembrolizumab) included early pediatric evaluations during drug development, assuring relevant pediatric data and drug formulations at the time of first approval.
Matching of cancer therapies based on a tumor’s genomic profile can tailor treatments to best available therapy, while minimizing exposure to or time spent on unnecessary or ineffective therapies [Citation1]. Studies evaluating the benefit of prospective matching of therapies have, however, yielded mixed findings. A 2015 study by Beltran et al. showed that WES provided clinically or biologically informative results in 94% (91/97) of patients with advanced cancers who provided tumor–normal pairs of biopsied tissues. WES led to the identification of alterations for which either matched therapies were approved or in clinical or preclinical development, or which were considered drivers and potentially actionable [Citation44]. At the time of the analysis, however, access to precision oncology medicines was still rather limited due to the scarcity of approved precision oncology therapies, and only 5% of patients had their treatment guided by WES. In a real-world study by Kato et al., 62% (265/429) of therapy-evaluable patients were matched to at least one recommended drug [Citation45]. Patients who received regimens recommended by a molecular tumor board (MTB), compared with ‘physician choice’ regimens with low matching, had significantly longer progression-free survival (PFS) and overall survival. An analysis of high vs low matching showed significant correlation between matching score and PFS, as well as stable disease and remission rates. The authors concluded that MTB-recommended therapies were better matched to genomic alterations and that the degree of matching was an independent predictor of outcomes including survival.
Alternatively, some studies have also shown limited success in using molecular profiling to match therapies. The MOSCATO 01 trial showed that of 843 patients who had a molecular portrait, high-throughput genomics led to matched targeted therapies for 199, but altogether only 7% (63/843) of successfully screened patients demonstrated clinical benefit based on predefined PFS thresholds [Citation46]. In another study, potentially actionable targets were identified in 70% (352/460) of patients, and 20% (101/460) were matched to treatments, yet overall response rate was only 15% (all partial responses), and median PFS was 12 weeks (95% CI: 9.9–14.4) [Citation47]. Finally, in an institution-wide, large-scale, prospective sequencing initiative using a hybridization capture-based NGS panel at Memorial Sloan Kettering Cancer Center (MSK-IMPACT), matched tumor and normal sequence data were obtained from >10,000 patients with >300 detailed tumor types, among which 37% of patients harbored a targetable alteration, yet only 11% of patients proceeded to enroll in genomically matched clinical trials [Citation48]. The low enrollment was attributed to availability and geographical accessibility of relevant trials, patient preference and eligibility for trial enrollment. Failure to demonstrate clear clinical benefit has been attributed to end point selection (e.g., PFS ratio, whose validity has been questioned) [Citation46,Citation47]. Therefore, despite the promise of precision oncology, the main limitation may not be identification of drug targets, but translation into actual therapy. Access to drugs varies widely across different countries and remains the most important hurdle between tumor molecular profiling and patient benefit.
The same issues that have plagued cancer therapy in general are evident with precision oncology therapies, such as inevitable evolution of tumors, intratumor heterogeneity, toxicity of targeting multiple pathways and the high costs of both testing and treatment [Citation49,Citation50]. A lack of matched therapies for many oncogenic drivers also limits the practical application of the abundance of data [Citation49]. Strategies are still needed to address access to medicine by patients who harbor actionable mutations in their tumors. Despite these hurdles, however, there is a steady influx of new targeted therapies in clinical development that are anticipated to enable better matching of therapy to target in the near and distant future.
Approaches & frameworks available for decision support
Although precision oncology can offer a plethora of genomic information about a patient’s cancer, practical limitations to its utility include insufficient evidence to guide biomarker implementation, limited understanding of functional effects of variant alleles, and lack of consensus on levels of evidence [Citation1]. Several models have been proposed for defining the ‘actionability’ of biomarkers, in which categories are based on strength of evidence and range between not currently actionable/variants of unknown significance, validated association between biomarker and response, and drug approval based on demonstrate effectiveness [Citation1].
To guide decision-making for clinicians, several decision support applications have been created. These include data-sharing technologies – such as AACR Project GENIE (Genomics Evidence Neoplasia Information Exchange) [Citation51,Citation52], Oncology Precision Network [Citation53] and Northwestern University’s OncoSET (Sequence, Evaluate, Treat) [Citation54] – that may be used to aggregate and link cancer genomics data with clinical outcomes. MTBs, such as those affiliated with OncoSET [Citation54] and University of California San Diego Moores Cancer Center [Citation55], are research initiatives designed to provide patients with personalized cancer treatment options through a combined oncology and genomics approach by bringing together a multidisciplinary team for weekly discussions of cancer cases and to propose recommendations for patient treatment plans [Citation56]. Most importantly, both the American Society of Clinical Oncology and the European Society for Medical Oncology have provided guidelines for reporting and ranking genomic alterations as targets for precision medicine [Citation57,Citation58].
Another patient management support model is the ‘decision support system’ (e.g., the Sheikh Khalifa Bin Zayed Al Nahyan Institute for Personalized Cancer Therapy at MD Anderson Cancer Center [Citation59]), where a decision support team provides clinicians with relevant information for assessing the actionability of variant alleles and identifying available matched therapies and clinical trials. The goals of that platform are to support genomic testing in their molecular diagnostic laboratory and to create infrastructure for integrating genomic analysis and patient data, to support development and recruitment of clinical trials for matched therapies, to promote research in mechanisms of response and resistance to targeted therapies, to provide decision support for personalized treatment plans, to demonstrate the value of those approaches and to educate, both within and outside the institution [Citation60].
Finally, virtual decision support tools such as Watson for Genomics from Quest Diagnostics have demonstrated proof-of-concept for the speed and utility of cognitive computing in identifying actionable mutations [Citation61]. In a study by Patel et al., Watson for Genomics was applied to 1018 patient cases that previously underwent targeted exon sequencing via NGS at the University of North Carolina (UNC) and the data compared with the university’s MTB recommendations. Watson demonstrated 100% concordance with clinician recommendations from MTBs, along with an additional 32% more strategies not identified by MTBs [Citation62]. Watson has multiple capabilities, including reading of radiological scans and molecular diagnostics to identify abnormalities and potentially actionable genomic mutations. The analysis conducted took only ∼3 min per case and showed that Watson was able to identify all genomic events found by the MTB using standard practices [Citation62]. The availability of such new bioinformatics tools and databases will aid clinicians in better predicting the functional significance of variants of unknown significance while providing new, faster and more thorough approaches for data analysis.
Impact of precision oncology on patients & their QoL
Treatment outcomes in oncology are frequently focused on improving survival and/or slowing progression of disease. As many precision oncology medicines have successfully achieved these end points, many patients are living longer and, in some cases, may require ongoing or sustained treatment. Thus when assessing outcomes related to a treatment, it is important to consider the potential for added toxicities given that they could contribute to diminished QoL. Patient-reported outcomes have been included in many clinical studies for precision oncology medicines, but publications on QoL impact from those trials are limited. Given the high rate of toxicities that accompany cytotoxic chemotherapy [Citation63–65], it is not unexpected that QoL findings from tumor-agnostic therapies have shown an overall positive impact ().
Table 2. Summary of quality of life findings for agents approved in tumor-agnostic indications.
When examining QoL, it is essential to look at patient health outcomes and global health status. Developing and implementing this strategy relies on collaboration between patients, patient organizations, healthcare professionals and industry. Patient health outcomes should be measured using the results that matter most to patients: recovery, functional improvement, physical functioning, emotional well-being and the ability to perform activities of daily living.
In patients with MSI-H/dMMR metastatic colorectal cancer receiving pembrolizumab, clinically meaningful improvements were seen in both of these measures, compared with standard of care [Citation66]. In patients with solid tumors harboring NTRK or ROS1 mutations treated with entrectinib, the overall QoL scores showed no change or a trend toward improvement from baseline [Citation77]. In patients receiving larotrectinib for TRK fusion cancer, sustained overall QoL improvements occurred by 2 months of treatment for 68% of adults and 71% of children; the median duration of sustained improvement was 12 months for adults (not evaluable for children) [Citation71,Citation72]. In patients with MSI-H/dMMR metastatic colorectal cancer, the time to deterioration in their overall QoL was longer in those receiving pembrolizumab compared with standard of care [Citation66].
Children with TRK fusion cancer treated with larotrectinib reported clinically meaningful improvements in physical, emotional, social and school areas of their lives, while adults with TRK fusion cancer treated with larotrectinib reported improvements in physical, role functioning, cognitive, emotional and social aspects [Citation71,Citation72]. Improvements were rapid (usually by cycle 3 or 5), lasted at least two cycles and were seen across most tumor types [Citation80]. In patients with solid tumors harboring NTRK or ROS1 mutations treated with entrectinib in the STARTRK-2 study, clinically meaningful improvements were seen in role functioning and pain [Citation77].
Future perspective: patient organization commentary
Patient understanding of precision oncology and personalized medicine has increased over time. In 2012, <50% of patients surveyed were familiar with the term ‘precision oncology’, and many expressed significant concerns regarding somatic genomic testing of their tumors [Citation81]. Over time, both patient and physician perspectives have shifted, with a growing proportion showing optimism for matching therapies that enable longer-term survivorship. In a multinational survey published in 2016, 78% of patients and 73% of physicians reported that patients understood a tumor could be tested to identify the most effective treatment and that patients would be willing to participate in a personalized treatment plan. While the vast majority of patients (85%) felt that they understood their treatment as explained to them, only 23% of physicians felt that their patients were fully informed [Citation82]. Likewise, in a separate survey of patients at a cancer network in Canada, patients’ understanding regarding their own cancer’s blood-based biomarkers was low (23%), yet the majority of patients considered the development of such biomarkers as important [Citation83]. Finally, in order to achieve successful transformative decision-making guided by tumor molecular profiling, it is imperative to build the trust between patients and their physicians; physicians are at the interface between research and real-world medicine, and physician trustworthiness is instrumental in influencing and promoting such programs [Citation84].
Historically, advanced cancer typically has a very poor prognosis, and still today for many people, there is a limited choice of treatments. Most current treatments are also very invasive, have significant side effects and often have quite serious side effects that significantly reduce patients’ QoL.
It is a constant battle to delay the further growth and spread of the cancer. The condition is physically and emotionally tough. Treatment can involve surgery, radiotherapy and/or chemotherapy and often comes with a known low survival rate and the understanding that the battle is to prolong life rather than to find a cure.
People with advanced cancer report that it has a substantial impact on their ability to enjoy life, work, ability to travel, ability to exercise and ability to carry out daily activities [Citation85,Citation86]:
Everything I do is tinged with a sadness & a sorrow of ‘will this be the last time I do this?’
For caregivers and other family members, the pressure is on them, from day 1, to help support and care for their loved ones. People caring for someone with advanced cancer report that it has a substantial impact on their ability to work, ability to travel and ability to spend time with their family and friends [Citation86]:
Caring for her means constant worry & constant vigilance.
Precision oncology medicine offers hope not only of an extended life, but also that those years are filled with life of good quality.
When comparing the adverse effects from immunotherapies and oncogene-targeted therapies, patients report less fatigue and weakness compared with chemotherapy [Citation63].
Before my treatment, I was worried that I would experience the same sort of abject fatigue that I felt after chemotherapy. However after a few cycles with minimal side effects, I felt much more relaxed with this new type of treatment.
As people are now living longer with cancer, these benefits can also translate into milestones they never thought they would get to experience, such as telling their story, creating art, contributing to science, seeing loved ones graduate or simply watching the sun rise one more time.
Offering tumor molecular profiling to all patients with advanced cancer increases the chances that they can be matched to the most appropriate and effective treatment. However, not all patients who would benefit from precision oncology medicine are being identified because of existing inequity in access to both tumor molecular profiling and affordable precision oncology medicines.
In order to resolve this inequity, we need to ensure that patients can understand and request tumor molecular profiling from their clinician, that the clinician is able to send the tumor for molecular profiling and that patients are able to access any precision oncology medicine that is suitable for them. Work is needed at all levels of the healthcare system to integrate molecular profiling into the advanced cancer patient journey and to make certain that cost-effective treatments based on molecular profiling are made accessible to all patients who would benefit.
Conclusion
Precision oncology holds promise in optimizing treatment strategies, improving outcomes and increasing QoL for many patients. The therapeutic potential of these medicines has been accompanied by growing enthusiasm from patients and physicians alike, prompting the creation of support networks and decision-making tools and teams. As the field continues to grow and expand, so will the support and enthusiasm by those who will benefit the most: the patients.
Overview of precision oncology medicines
Growing interest in identifying and characterizing genomic biomarkers has led to a paradigm shift away from nonspecific cytotoxic treatment strategies, toward personalized treatment strategies based on the genomic profile of a tumor.
Molecular testing enables the classification and diagnosis of cancers with greater precision, potentially improving prognosis, therapeutic decision-making and outcomes, while sparing patients from treatments that may confer less clinical benefit.
Recent approvals of tumor-agnostic therapies (larotrectinib, entrectinib and pembrolizumab) represent a milestone in cancer research, upending the widely held belief that pharmacological approaches to cancer management must be tumor specific.
Next-generation sequencing
Tools such as next-generation sequencing provide a comprehensive tumor profile per patient, enabling clinicians to select therapies based on detailed diagnoses and tumor characteristics.
Identification of specific molecular signatures via NGS is expected to aid in effective matching of therapy to patient, thus reducing and/or avoiding unnecessary delays to treatment.
Precision oncology for matching therapies
Challenges with precision oncology medicines have included inevitable evolution of tumors, intratumor heterogeneity, toxicity of targeting multiple pathways, and high costs; practical limitations include insufficient evidence to guide biomarker implementation, limited understanding of functional effects of variant alleles and lack of consensus on levels of evidence.
To address these challenges, decision support applications have been created to guide decision-making for clinicians, including data-sharing technologies, molecular tumor boards, bioinformatics and artificial intelligence.
Impact of precision oncology on patients & their quality of life
With the proven success of precision oncology in improving outcomes and quality of life, as well as identifying and overcoming mechanisms of drug resistance and relapse, patient understanding of precision oncology has increased over time, with more patients showing optimism for matching therapies that enable longer-term survivorship; from a patient perspective, precision oncology has enabled longer survivorship, with benefits translating into milestones they never thought they would get to experience.
Work is needed at all levels of the healthcare system to integrate molecular profiling into the advanced cancer patient journey, and to make certain that cost-effective treatments based on molecular profiling are made accessible to all who would benefit.
Author contributions
Conception/design: U Lassen, L Makaroff, A Stenzinger, A Italiano, G Vassal, J Garcia-Foncillas, B Avouac. Manuscript writing: U Lassen, L Makaroff, A Stenzinger, A Italiano, G Vassal, J Garcia-Foncillas, B Avouac. Final approval of manuscript: U Lassen, L Makaroff, A Stenzinger, A Italiano, G Vassal, J Garcia-Foncillas, B Avouac.
Financial & competing interests disclosure
U Lassen has received research grants from BMS, GSK, Pfizer and Roche, and participated on advisory boards for Bayer, Novartis and Pfizer. L Makaroff has received grants from Astellas, AstraZeneca, Bayer, BMS, Ferring, Ipsen, Janssen, Merck, MSD, Pfizer, Roche and Seagen, and participated on advisory boards/speaker’s bureau for Bayer. A Stenzinger received research grants from BMS, Bayer and Chugai, and participated in advisory boards/speaker’s bureaus for AstraZeneca, Bayer, BMS, Eli Lilly, Illumina, Janssen, MSD, Novartis, Pfizer, Roche, Seattle Genetics and Thermo Fisher. A Italiano has held an advisory role for Bayer Healthcare. G Vassal provided advice to Bayer, BMS, Roche/Genentech, Celgene, Debiopharm, Incyte, Ipsen, Lilly, Pfizer, Servier and Takeda without personal remuneration. J Garcia-Foncillas received research grants from Merck and Roche and participated in advisory boards/speaker’s bureau for AstraZeneca, Bayer, BMS, Eli Lilly, Janssen, Merck MSD, Novartis, Pfizer and Roche. B Avouac participated in advisory boards for AstraZeneca, Bayer, Novartis, Roche and BMS, and provided advice to Pfizer, Incyte, Ipsen and Takeda. The authors have no other relevant affiliations or financial involvement with any organization or entity with a financial interest in or financial conflict with the subject matter or materials discussed in the manuscript apart from those disclosed.
Medical writing assistance was provided by I Koo and funded by Bayer.
Additional information
Funding
References
- Chae YK , PanAP, DavisAAet al. Path toward precision oncology: review of targeted therapy studies and tools to aid in defining ‘actionability’ of a molecular lesion and patient management support. Mol. Cancer Ther.16(12), 2645–2655 (2017).
- US Food & Drug Administration . Drug approvals and databases. www.fda.gov/drugs/development-approval-process-drugs/drug-approvals-and-databases
- European Medicines Agency . Medicines. www.ema.europa.eu/en/medicines
- Sabir SR , YeohS, JacksonG, BaylissR. EML4-ALK variants: biological and molecular properties, and the implications for patients. Cancers9(9), 118 (2017).
- Riely GJ , YuHA. EGFR: the paradigm of an oncogene-driven lung cancer. Clin. Cancer Res.21(10), 2221–2226 (2015).
- Chae YK , RanganathK, HammermanPSet al. Inhibition of the fibroblast growth factor receptor (FGFR) pathway: the current landscape and barriers to clinical application. Oncotarget8(9), 16052–16074 (2017).
- Zhou Y , WuC, LuG, HuZ, ChenQ, DuX. FGF/FGFR signaling pathway involved resistance in various cancer types. J. Cancer.11(8), 2000–2007 (2020).
- Li AY , McCuskerMG, RussoAet al. RET fusions in solid tumors. Cancer Treat. Rev.81, 101911 (2019).
- Subbiah V , CoteGJ. Advances in targeting RET-dependent cancers. Cancer Discov.10(4), 498–505 (2020).
- Giustini NP , BazhenovaL. ROS1-rearranged non-small cell lung cancer. Thorac. Surg. Clin.30(2), 147–156 (2020).
- Patil T , SimonsE, MushtaqR, PachecoJM, DoebeleRC, BowlesDW. Targeted therapies for ROS1-rearranged non-small cell lung cancer. Drugs Today55(10), 641–652 (2019).
- Yang J , NieJ, MaX, WeiY, PengY, WeiX. Targeting PI3K in cancer: mechanisms and advances in clinical trials. Mol. Cancer18(1), 26 (2019).
- Croce L , CoperchiniF, MagriF, ChiovatoL, RotondiM. The multifaceted anti-cancer effects of BRAF-inhibitors. Oncotarget10(61), 6623–6640 (2019).
- Kelly CM , GutierrezSainz L, ChiP. The management of metastatic GIST: current standard and investigational therapeutics. J. Hematol. Oncol.14(1), 2 (2021).
- File D , CuriglianoG, CareyLA. Escalating and de-escalating therapy for early-stage HER2-positive breast cancer. Am. Soc. Clin. Oncol. Educ. Book40, 1–11 (2020).
- Jang A , SartorO, BarataPC, PallerCJ. Therapeutic potential of PARP inhibitors in the treatment of metastatic castration-resistant prostate cancer. Cancers12(11), 3467 (2020).
- Li K , LuoH, HuangL, LuoH, ZhuX. Microsatellite instability: a review of what the oncologist should know. Cancer Cell Int.20, 16 (2020).
- Amatu A , Sartore-BianchiA, BencardinoK, PizzutiloEG, TosiF, SienaS. Tropomyosin receptor kinase (TRK) biology and the role of NTRK gene fusions in cancer. Ann. Oncol.30(Suppl. 8), VIII5–VIII15 (2019).
- Marino FZ , PagliucaF, RonchiAet al. NTRK fusions, from the diagnostic algorithm to innovative treatment in the era of precision medicine. Int. J. Mol. Sci.21(10), 1–15 (2020).
- Shao C , LiG, HuangLet al. Prevalence of high tumor mutational burden and association with survival in patients with less common solid tumors. JAMA Netw. Open.3(10), e2025109 (2020).
- Xie J , LuX, WuXet al. Capture-based next-generation sequencing reveals multiple actionable mutations in cancer patients failed in traditional testing. Mol. Genet. Genom. Med.4(3), 262–272 (2016).
- Hillman RT , WardK, SaenzC, McHaleM, PlaxeS. Barriers prevent patient access to personalized therapies identified by molecular tumor profiling of gynecologic malignancies. J. Pers. Med.5(2), 165–173 (2015).
- Arsenic R , TreueD, LehmannAet al. Comparison of targeted next-generation sequencing and Sanger sequencing for the detection of PIK3CA mutations in breast cancer. BMC Clin. Pathol.15, 20 (2015).
- Hux A , LewisA, SachwitzD, GregoryT. Clinical utility of next-generation sequencing in precision oncology. J. Am. Acad. Phys. Assist.32(1), 35–39 (2019).
- Meldi K , QinT, BuchiFet al. Specific molecular signatures predict decitabine response in chronic myelomonocytic leukemia. J. Clin. Invest.125(5), 1857–1872 (2015).
- Walker BA , BoyleEM, WardellCPet al. Mutational spectrum, copy number changes, and outcome: results of a sequencing study of patients with newly diagnosed myeloma. J. Clin. Oncol.33(33), 3911–3920 (2015).
- Sato Y , MatobaR, KatoK. Recent advances in liquid biopsy in precision oncology research. Biol. Pharm. Bull.42(3), 337–342 (2019).
- Pfaff E , de BeaumaisT, MarchaisAet al. NTRK alterations in pediatric relapsed/refractory/very high risk malignancies identified through European clinical sequencing programs constitute promising drug targets. J. Clin. Oncol. Prec. Oncol.5, 450–454 (2021).
- Brown NA , Elenitoba-JohnsonKSJ. Enabling precision oncology through precision diagnostics. Annu. Rev. Pathol.15, 97–121 (2020).
- Galuppini F , DalPozzo CA, DeckertJ, LoupakisF, FassanM, BaffaR. Tumor mutation burden: from comprehensive mutational screening to the clinic. Cancer Cell Intern.19, 209 (2019).
- Tan O , ShresthaR, CunichM, SchofieldDJ. Application of next-generation sequencing to improve cancer management: a review of the clinical effectiveness and cost-effectiveness. Clin. Genet.93(3), 533–544 (2018).
- Ascierto PA , KirkwoodJM, GrobJ-Jet al. The role of BRAF V600 mutation in melanoma. J. Transl. Med.10, 85 (2012).
- Planchard D , SmitEF, GroenHJMet al. Dabrafenib plus trametinib in patients with previously untreated BRAF(V600E)-mutant metastatic non-small-cell lung cancer: an open-label, Phase 2 trial. Lancet Oncol.18(10), 1307–1316 (2017).
- Long GV , StroyakovskiyD, GogasHet al. Dabrafenib and trametinib versus dabrafenib and placebo for Val600 BRAF-mutant melanoma: a multicentre, double-blind, Phase 3 randomised controlled trial. Lancet386(9992), 444–451 (2015).
- Subbiah V , KreitmanRJ, WainbergZAet al. Dabrafenib and trametinib treatment in patients with locally advanced or metastatic BRAF V600-mutant anaplastic thyroid cancer. J. Clin. Oncol.36(1), 7–13 (2018).
- Subbiah V , LassenU, ÉlezEet al. Dabrafenib plus trametinib in patients with BRAF(V600E)-mutated biliary tract cancer (ROAR): a Phase 2, open-label, single-arm, multicentre basket trial. Lancet Oncol.21(9), 1234–1243 (2020).
- Yang H , HigginsB, KolinskyKet al. Antitumor activity of BRAF inhibitor vemurafenib in preclinical models of BRAF-mutant colorectal cancer. Cancer Res.72(3), 779–789 (2012).
- van Geel RMJM , TaberneroJ, ElezEet al. A Phase Ib dose-escalation study of encorafenib and cetuximab with or without alpelisib in metastatic BRAF-mutant colorectal cancer. Cancer Discov.7(6), 610–619 (2017).
- Mao M , TianF, MariadasonJMet al. Resistance to BRAF inhibition in BRAF-mutant colon cancer can be overcome with PI3K inhibition or demethylating agents. Clin. Cancer Res.19(3), 657–667 (2013).
- Alshareef A . Novel molecular challenges in targeting anaplastic lymphoma kinase in ALK-expressing human cancers. Cancers9(11), 1–13 (2017).
- Brown NA , Elenitoba-JohnsonKSJ. Enabling precision oncology through precision diagnostics. Annu. Rev. Pathol. Mech. Dis.15(1), 97–121 (2020).
- Kurnit K , DumbravaE, LitzenburgerBet al. Precision oncology decision support: current approaches and strategies for the future. Clin. Cancer Res.24(12), 2719–2731 (2018).
- Khan T , StewartM, BlackmanSet al. Accelerating pediatric cancer drug development: challenges and opportunities for pediatric master protocols. Ther. Innov. Regul. Sci.53(2), 270–278 (2019).
- Beltran H , EngK, MosqueraJMet al. Whole-exome sequencing of metastatic cancer and biomarkers of treatment response. JAMA Oncol.1(4), 466–474 (2015).
- Kato S , KimKH, LimHJet al. Real-world data from a molecular tumor board demonstrates improved outcomes with a precision N-of-one strategy. Nat. Commun.11(1), 4965 (2020).
- Massard C , MichielsS, FertéCet al. High-throughput genomics and clinical outcome in hard-to-treat advanced cancers: results of the MOSCATO 01 trial. Cancer Discov.7(6), 586–595 (2017).
- Tuxen IV , RohrbergKS, OestrupOet al. Copenhagen Prospective Personalized Oncology (CoPPO) – clinical utility of using molecular profiling to select patients to Phase I trials. Clin. Cancer Res.25(4), 1239–1247 (2019).
- Zehir A , BenayedR, ShahRHet al. Mutational landscape of metastatic cancer revealed from prospective clinical sequencing of 10.000 patients. Nat. Med.23(6), 703–713 (2017).
- Brock A , HuangS. Precision oncology: between vaguely right and precisely wrong. Cancer Res.77(23), 6473–6479 (2017).
- Sánchez NS , MillsGB, ShawKRM. Precision oncology: neither a silver bullet nor a dream. Pharmacogenomics18(16), 1525–1539 (2017).
- American Association for Cancer Research . AACR Project GENIE. http://aacr.org/genie
- AACR Project GENIE Consortium . AACR Project GENIE: powering precision medicine through an international consortium. Cancer Disc.7(8), 818–831 (2017).
- Syapse Inc . Syapse Learning Health Network (2021). www.syapse.com/offerings/syapse-learning-health-network
- Northwestern University . OncoSET Precision Medicine (2021). http://cancer.northwestern.edu/oncoset
- Schwaederle M , ParkerBA, SchwabRBet al. Molecular tumor board: the University of California-San Diego Moores Cancer Center experience. Oncologist19(6), 631–636 (2014).
- Patel M , KatoSM, KurzrockR. Molecular tumor boards: realizing precision oncology therapy. Clin. Pharmacol. Ther.103(2), 206–209 (2018).
- Li MM , DattoM, DuncavageEJet al. Standards and guidelines for the interpretation and reporting of sequence variants in cancer: a joint consensus recommendation of the Association for Molecular Pathology, American Society of Clinical Oncology, and College of American Pathologists. J. Mol. Diagn.19(1), 4–23 (2017).
- Mateo J , ChakravartyD, DienstmannRet al. A framework to rank genomic alterations as targets for cancer precision medicine: the ESMO Scale for Clinical Actionability of molecular Targets (ESCAT). Ann. Oncol.29(9), 1895–1902 (2018).
- Meric-Bernstam F , JohnsonA, HollaVet al. A decision support framework for genomically informed investigational cancer therapy. J. Natl Cancer Inst.107(7), djv098 (2015).
- University of Texas MD Anderson Cancer Center . Sheikh Khalifa Bin Zayed Al Nahyan Institute for Personalized Cancer Therapy (2021). www.mdanderson.org/research/departments-labs-institutes/institutes/zayed-institute-for-personalized-cancer-therapy.html
- IBM Watson Health . IB Watson for Genomics. www.ibm.com/watson/health/oncology/genomics/
- Patel NM , MicheliniVV, SnellJMet al. Enhancing next-generation sequencing-guided cancer care through cognitive computing. Oncologist23(2), 179–185 (2018).
- Barnes TA , AmirE, TempletonAJet al. Efficacy, safety, tolerability and price of newly approved drugs in solid tumors. Cancer Treat. Rev.56, 1–7 (2017).
- Schirrmacher V . From chemotherapy to biological therapy: a review of novel concepts to reduce the side effects of systemic cancer treatment. Int. J. Oncol.54(2), 407–419 (2019).
- Haanen JBAG , CarbonnelF, RobertCet al. Management of toxicities from immunotherapy: ESMO Clinical Practice Guidelines for diagnosis, treatment and follow-up. Ann. Oncol.28(Suppl. 4), iv119–iv142 (2017).
- André T , AmonkarM, NorquistJet al. Health-related quality of life (HRQoL) in patients (pts) treated with pembrolizumab (pembro) vs chemotherapy as first-line treatment in microsatellite instability-high (MSI-H) and/or deficient mismatch repair (dMMR) metastatic colorectal cancer (mCRC): ph. Ann. Oncol.31(Suppl. 4), S409–S461 (2020).
- KEYTRUDA® [package insert]. Merck & Co., Inc, NJ, USA (2020). www.merck.com/product/usa/pi_circulars/k/keytruda/keytruda_pi.pdf
- Marabelle A , LeDT, AsciertoPAet al. Efficacy of pembrolizumab in patients with noncolorectal high microsatellite instability/mismatch repair-deficient cancer: results from the Phase II KEYNOTE-158 study. J. Clin. Oncol.38(1), 1–10 (2020).
- André T , ShiuK-K, KimTWet al. Pembrolizumab in microsatellite-instability-high advanced colorectal cancer. N. Engl. J. Med.383(23), 2207–2218 (2020).
- Marabelle A , FakihM, LopezJet al. Association of tumour mutational burden with outcomes in patients with advanced solid tumours treated with pembrolizumab: prospective biomarker analysis of the multicohort, open-label, Phase 2 KEYNOTE-158 study. Lancet Oncol.21(10), 1353–1365 (2020).
- Kummar S , Van TilburgCM, AlbertCMet al. Quality of life of adults and children with TRK fusion cancer treated with larotrectinib compared to the general population. J. Clin. Oncol.38(Suppl.), Abstract 3614 (2020).
- Kummar S , BerlinJ, MascarenhasLet al. Quality of life in adult and pediatric patients with tropomyosin receptor kinase fusion cancer receiving larotrectinib. Curr. Probl. Cancer.doi:10.1016/j.currproblcancer.2021.100734 (2021) ( Epub ahead of print).
- VITRAKVI Summary of Product Characteristics (SPC). www.ema.europa.eu/en/documents/product-information/vitrakvi-epar-product-information_en.pdf
- VITRAKVI® [package insert]. Bayer Healthcare Pharmaceuticals Inc, NJ, USA (2019).
- Drilon A , LaetschTW, KummarSet al. Efficacy of larotrectinib in TRK fusion-positive cancers in adults and children. N. Engl. J. Med.378(8), 731–739 (2018).
- Hong DS , DuBoisSG, KummarSet al. Larotrectinib in patients with TRK fusion-positive solid tumours: a pooled analysis of three Phase 1/2 clinical trials. Lancet Oncol.21(4), 531–540 (2020).
- Conley AP , DemetriGD, DoebeleRCet al. Patient-reported outcomes (PROs) from patients (Pts) with NTRK fusion-positive (NTRK-fp) solid tumours receiving entrectinib in the global Phase II STARTRK-2 study. Ann. Oncol.31(Suppl. 4), S462–S504 (2020).
- ROZLYTREK® [package insert]. Genentech USA, Inc, CA, USA (2019). www.gene.com/download/pdf/rozlytrek_prescribing.pdf
- Doebele RC , DrilonA, Paz-AresLet al. Entrectinib in patients with advanced or metastatic NTRK fusion-positive solid tumours: integrated analysis of three Phase 1–2 trials. Lancet Oncol.21(2), 271–282 (2020).
- Kummar S , MascarenhasL, GeoergerBet al. Patient-reported outcomes from two global multicenter clinical trials of children and adults with tropomyosin receptor kinase (TRK) fusion cancer receiving larotrectinib. J. Clin. Oncol.37(Suppl. 15), 6602 (2019).
- Gray SW , KimB, ShollLet al. Medical oncologists’ experiences in using genomic testing for lung and colorectal cancer care. J. Oncol. Pract.13(3), e185–e196 (2017).
- Ciardiello F , AdamsR, TaberneroJet al. Awareness, understanding, and adoption of precision medicine to deliver personalized treatment for patients with cancer: a multinational survey comparison of physicians and patients. Oncologist21(3), 292–300 (2016).
- Lee MJ , HuenikenK, KuehneNet al. Cancer patient-reported preferences and knowledge for liquid biopsies and blood biomarkers at a comprehensive cancer center. Cancer Manag. Res.12, 1163–1173 (2020).
- Persaud A , BonhamVL. The role of the health care provider in building trust between patients and precision medicine research programs. Am. J. Bioeth.18(4), 26–28 (2018).
- Albrecht TA , TaylorAG. Physical activity in patients with advanced-stage cancer: a systematic review of the literature. Clin. J. Oncol. Nurs.16(3), 293–300 (2012).
- Shilling V , StarkingsR, JenkinsV, FallowfieldL. The pervasive nature of uncertainty – a qualitative study of patients with advanced cancer and their informal caregivers. J. Cancer Surviv.11(5), 590–603 (2017).