Abstract
Aim: To find a practical biomonitoring method for researchers exposed to nanoparticles causing oxidative stress. Methods: In a continuation of a study in 2016–2018, biological samples (plasma, urine and exhaled breath condensate [EBC]) were collected in 2019–2020 from 43 researchers (13.8 ± 3.0 years of exposure) and 45 controls. Antioxidant status was assessed using glutathione (GSH) and ferric-reducing antioxidant power, while oxidative stress was measured as thiobarbituric acid reactive substances, all using spectrophotometric methods. Researchers’ personal nanoparticle exposure was monitored. Results: Plasma GSH was elevated in researchers both before and after exposure (p < 0.01); postexposure plasma GSH correlated with nanoparticle exposure, and GSH in EBC increased. Conclusion: The results suggest adaptation to chronic exposure to nanoparticles, as monitored by plasma and EBC GSH.
Plain language summary
What is this study about?
Identifying markers of oxidative stress and/or adaptation to oxidation stress could offer tools for monitoring exposure to nanoparticles in exposed researchers. In this study, we question whether these markers correlate with their personal exposure during the shift.
What were the results?
We found that exposure to nanoparticles correlated with the antioxidant marker glutathione, which is higher in workers who are already pre-exposed.
What do the results mean?
This study suggests that the researchers have adapted to nanoparticle exposure and are ready to combat oxidative stress. However, the similarity with increased markers of oxidative stress from asbestos and silica exposure, including nucleic acid oxidation, previously found in these researchers highlights the need for further research in this area to better understand and prevent potential future effects.
Nanotechnology, based on the application of ultrafine particles having dimensions in the range of 1–100 nm, represents one of the fastest-growing areas of technology and research, including medicine. The high surface energy of nanoparticles, reflected in their high reactivity and specific properties, including catalytic behavior, improved strength and increased thermal or electrical conductivity, gives them unique properties. However, aside from their undoubted benefits, nanoparticles raise concerns about their potential toxicity and harmful effects on human health. In particular, data on the toxicity of nanoparticles in researchers are very limited, and quantitative risk assessment and biological monitoring of nanoparticle exposure are lacking [Citation1–5].
Oxidative stress is a disturbance in the balance between the production of reactive oxygen species (ROS) and reactive nitrogen species and their scavenging by the defense system of low- and high-molecular-weight antioxidants in the organism [Citation6,Citation7]. Importantly, nanoparticles can lead to the overproduction of highly reactive ROS, such as singlet oxygen, hydrogen peroxide, superoxide radical or hydroxyl radical [Citation8–11], and reactive nitrogen species, including nitric oxide, peroxynitrite or nitrogen dioxide [Citation7]. Nanoparticles can modify cell protein structures, disrupt cell membrane integrity, disrupt mitochondrial structure and cause apoptosis [Citation12,Citation13]. Reactive species can participate in tumorigenesis in multiple ways [Citation14,Citation15]. However, no generally accepted guidelines have been prepared for the biological monitoring of researchers exposed to nanoparticles. Therefore, biomarkers of exposure and effects are urgently needed to monitor and prevent their potentially harmful health effects [Citation16,Citation17].
After inhalation, the systemic health effects of nanoparticles are dependent on their ability to enter the blood circulation [Citation12]. Plasma, urine and exhaled breath condensate (EBC) have been successfully used for the analysis of oxidative stress markers in researchers [Citation18,Citation19]. EBC is the liquid phase of the exhaled air, collected noninvasively during tidal breathing and by cooling the exhaled air, which reflects the composition of the airways’ epithelial lining fluid, which is the source of several biomarkers of respiratory and systemic diseases. EBC consists of 99.9% water and volatile (e.g., oxygen, carbon dioxide, nitric oxide) and non-volatile macromolecular compounds, including oxidation products of nucleic acids, lipids and proteins [Citation20,Citation21]. EBC collection has been used in the diagnosis and monitoring of lung diseases [Citation20,Citation22–27]. Also in our studies, pre-shift samples of EBC, plasma and urine were used to measure oxidative stress and inflammation markers to study the subacute/chronic effects on researchers resulting from previous exposures; a comparison of the pre-shift and post-shift samples confirmed the acute effect of exposure during the shift [Citation25,Citation26].
In this follow-up on the group of researchers previously examined in September 2016–2018 [Citation26], and then again in 2019 and 2020, we also compared both chronic and acute effects. This time, we focused on their antioxidant status, considered an indicator of the resistance of their organisms to oxidative stress.
As the first antioxidant marker, GSH – the main non-enzymatic regulation factor of intracellular redox homeostasis – was chosen, due to its high importance in the lungs in protecting alveolar membrane integrity from the toxic effects of environmental oxidants such as O3, SO2 and occupational dusts [Citation28].
Secondly, the antioxidant capacity was evaluated using the ferric-reducing antioxidant power (FRAP) assay. The basic principle of this assay is the reduction of colorless ferric tripyridyltriazine to blue ferrous tripyridyltriazine by the action of electron-donating antioxidants at low pH [Citation29].
Thirdly, oxidative stress was measured by the thiobarbituric acid reactive substances (TBARS) assay, one of the most widely used methods for the evaluation of lipid peroxidation in biological fluids. The assay is based on the reaction of lipid peroxidation products, primarily malondialdehyde (MDA), with thiobarbituric acid, leading to the formation of MDA–thiobarbituric acid 2 adducts named TBARS [Citation30]. The TBARS assay was used here because MDA had been elevated in the earlier studies of these researchers [Citation26].
These three markers were analyzed in plasma, urine and EBC using simple, easily available spectrophotometric methods. The second objective of this study was to verify the usefulness of this method in proving the oxidation of lipids found earlier by HPLC–MS/MS [Citation26]. Although GSH’s antioxidant properties are well known and GSH has also been studied in nanomedicine as a drug and and in drug-delivery systems [Citation31], these three markers have not yet been examined in nanomaterial-exposed researchers. To the best of our knowledge, it is the first study of adaptation to oxidative stress in the occupationally exposed population.
Materials & methods
Workshops aerosol sampling & personal exposure measurement
Static air monitoring was carried out using offline and online instruments. Furthermore, personal exposure measurements were used for the evaluation of particle concentrations in the researchers’ breathing zones.
The particle mass size distributions and PM2.5 concentrations (mass concentrations of all particulate matter under 2.5 μm in aerodynamic diameter) were measured at a fixed point with a Berner Low-Pressure Impactor (BLPI 25/0.018/2; Hauke GmbH, Gműnden, Austria) and a PM2.5 low volume sampler (Sven Leckel Ingenieurbüro GmbH, Berlin, Germany), respectively. Two standard online aerosol spectrometers, a scanning mobility particle sizer (SMPS) and an aerodynamic particle sizer (APS) (both TSI, Inc., MN, USA), were used during the monitored individual working exposures. APS and SMPS determine aerosol particle number, size distribution and concentration (covering particle diameters ranging from 10 nm up to 20 μm). In addition, a condensation particle counter (TSI, Inc.) was used to monitor the total number concentration with high time resolution.
Personal exposure was measured in all researchers using a personal nanoparticle sampler (PENS; Pluto Technology Co., Ltd, Taiwan), allowing for the collection of both respirable particles with an aerodynamic diameter under 4 μm (PM4) and nanoparticles under 0.1 μm (PM0.1) simultaneously [Citation32]. Both PM4 and PM0.1 particles were collected during all working activities. The impaction substrate was a 25-mm-diameter aluminum foil with a double-sprayed layer of Dekati DS-515 collection substrate (Dekati, Kangasala, Finland). Nanoparticles were collected using a filter cassette containing a 37-mm Teflon filter (polytetrafluoroethylene with polymethylpentene support ring, 37 mm, 2 μm; SKC 225-1709; SKC, Inc., PA, USA). Each PENS was connected to its own AirChek XR5000 personal sampling pump (SKC, Inc.). The flow rate calibration was performed with a Gilibrator-2 (Gilian, Sensidyne, Schauenburg International GmbH, Mülheim, Germany) before each measurement. Finally, aluminum foils and Teflon filters were weighed using an M5P balance (Sartorius GmbH, Göttingen, Germany; 1 μg resolution).
Subjects
Researchers
Occupationally exposed researchers (21 researchers in September 2019 and 22 researchers in September 2020) were interviewed by occupational physicians using a detailed questionnaire. Occupational history included the type of exposure in the workshops (machining or welding), length of exposure to nanoparticles (years), length of usual daily exposure (hours), latency time since the last shift (hours) and the use of personal protective equipment (yes/no). The personal history section contained questions about diseases, symptoms, medication, diet and lifestyle habits such as smoking (yes/no), alcohol intake (yes/no) and minimum weekly physical activity (defined as walking for more than 30 min five times a week). Then the subjects underwent a physical examination, including BMI, spirometry and fractional exhaled nitric oxide (FeNO) measurement. The samples of plasma, urine and EBC were collected both before and after exposure.
In both years, the researchers were occupationally exposed to nanoparticles released during their usual work – that is, machining (11 in 2019 and 11 in 2020; 22 researchers in total) or welding (10 in 2019 and 11 in 2020; 21 researchers in total) – in two separate workshops for approximately 3 h.
In workshop 1, the machining of a nanocomposite containing an epoxy composite filled with synthetically produced SiO2 primary particles of approximately 10 nm in size that aggregate into clusters of hundreds of nanometers was ground and milled. The grinders used pink corundum discs containing Al2O3, Cr2O3 and Fe2O3. Finally, nanocomposites containing SiO2 were milled, as described in our earlier studies [Citation26,Citation33].
In workshop 2, welding on metal surfaces (metal active gas technology) was conducted, and the processes did not differ from that in the years 2016–2018, including the chemical composition of the materials used [Citation26,Citation33].
Controls
The control subjects (21 subjects in 2019 and 24 in 2020), living in the same location and not exposed to dust or aerosols at their workplaces, were examined at approximately the same time as the researchers. These participants had comparable characteristics to the researchers, as can be seen in .
Table 1. General characteristics of the groups.
All subjects gave their written consent to inclusion before participating in the study. The study was performed in accordance with the Declaration of Helsinki.
Biological samples
Pre- and post-exposure plasma, urine and EBC samples were collected in groups of researchers and controls.
The pre-exposure samples of the researchers were chosen to study potential chronic effects when compared with the morning samples of the controls. Postexposure samples were used to evaluate the effect of the 3-h shift in the workshops when compared with afternoon samples of the controls.
EBC samples were collected using an Ecoscreen Turbo Disposable Exhaled Condensate Collection System device (Erich Jaeger GmbH, Hochberg, Germany). After rinsing the mouth with water several times, all participants breathed tidally for 15 min through a mouthpiece connected to the condenser while wearing a nose clip. All samples were frozen and stored for up to 15 months at -80°C until analysis.
Analysis of markers of antioxidant status (GSH, FRAP) & oxidative stress (TBARS)
Reduced GSH was measured spectrophotometrically using 5,5′-dithiobis (2-nitrobenzoic acid). Specimens (200 μl of a sample or saline solution as a blank) were treated with 600 μl of 2.5% trichloroacetic acid to remove proteins. The suspension was incubated for 10 min and centrifuged at 1000 × g for 10 min at 4°C. Next, 500 μl of supernatant was neutralized by 125 μl of 0.5 mol/l sodium hydroxide. The neutralized solution (400 μl) was poured into 400 μl of 5,5′-dithiobis (2-nitrobenzoic acid), and absorbance at 412 nm was measured using the extinction coefficient for conjugate GSH-5-thio-bis-2-nitrobenzoic acid equal to 14,150 M-1cm-1.
FRAP assays were used to determine total levels of low-molecular-weight antioxidants in samples using 2,4,6-tris(2-pyridyl)-s-triazine and ferric chloride. In the first step, the reagent for the assay was prepared: 2.5 ml of 10 mmol/l 2,4,6-tris (2-pyridyl)-s-triazine in 40 mmol/l hydrochloric acid was mixed with 2.5 ml of 20 mmol/l ferric chloride. Then the mixture was added to 25 ml of 0.1 mmol/l acetate buffer (pH 3.6) and heated to 37°C for 10 min. Next, after cooling down, 200 μl of the reagent was mixed with a sample (or saline solution for blank purposes) in a volume of 30 μl. The addition of 770 μl of demineralized water followed, and the mixture was left to incubate for 10 min. Finally, the sample was centrifuged at 10,000 × g for 10 min. The absorbance of the supernatant was measured against a blank at 593 nm, and the extinction coefficient ϵ = 26,000 M-1cm-1 served for the FRAP value calculation.
Using the TBARS method, 67 mg of thiobarbituric acid was dissolved in 1 ml of dimethyl sulfoxide, then 9 ml of demineralized water was added. In a separate step, samples were treated with trichloroacetic acid (200 μl of the sample was poured into 400 μl of 10% trichloroacetic acid) and incubated for 15 min. The suspension was centrifuged at 3000 × g for 15 min. Finally, 400 μl of the purified sample was mixed with 400 μl of the thiobarbituric acid solution, heated at 100°C for 10 min, then left to cool. Absorbance was measured at 532 nm, and the extinction coefficient ϵ = 156,000 M-1cm-1 was used for the MDA concentration calculation.
The limits of detection for GSH, FRAP and TBARS were 0.85, 1.3 and 0.039 μmol/l, respectively; the limits of quantitation (LOQs) for GSH, FRAP and TBARS were 2.8, 4.2 and 0.13 μmol/l, respectively.
Environmental air pollution
To evaluate the potentially interfering effects of environmental air pollution, values of air levels of environmental pollutants measured every 30 min were sourced from the National Hydrometeorological Monitoring System (www.chmi.cz/?l=en). The data from the stationary monitoring station nearest to the research institute with the ambulatory room for the examination of the subjects (a distance of less than 3 km) included five parameters: air levels of SO2, NO2, O3, and PM2.5 and PM10.
Statistics
Basic descriptive characteristics were computed for all variables (mean, median, standard deviation and CI). A statistical F-test was used to assess the equality of variances. The paired t-test was used to compare the concentrations of markers of antioxidant status and oxidative stress in the researchers, measured pre- and post-shift, as well as in controls. Unpaired t-tests for different variances and t-tests for the same variance were also performed. Spearman’s rank correlation coefficient was used to quantify relationships between continuous variables. p-values less than 0.05 were considered significant.
Results
Workshops aerosol measurement
The total number concentrations of two size fractions and their contributions to the total number concentrations of particles measured in both workshops during the shift and during the night before the shift (background) are shown in . The background total number concentrations were comparable for both fractions in both years, with only a slight increase observed in 2020 for the 100 nm–1 μm size fraction. Machining of materials containing nanoparticles produced some mechanically emitted particles primarily larger than 1 μm, whereas particles from evaporation and subsequent condensation were mainly below 100 nm [Citation26]. Metal active gas welding of metal surfaces mainly produced particles in the size range of 100 nm–1 μm due to the fast coagulation of smaller primary particles forming larger agglomerates.
Table 2. Number concentrations of selected particulate matter fractions and their proportions measured by scanning mobility particle sizer and aerodynamic particle sizer in 2019 and 2020.
Personal exposure measurement
By comparing the ratios of nanoparticles/respirable particles for the PENS and BLPI, an overestimation of PENS-PM0.1 concentration due to a relatively large bounce of respirable particles onto the nanoparticle filter was found. Therefore, to correct the PENS-PM0.1 concentration, a concentration ratio calculated from the concentrations measured by the SMPS/APS systems was used. In 2019, the dose of PM0.1 for the exposure period collected by PENS ranged from 0.5 to 16.5 μg in machining researchers and from 0.3 to 8.2 μg in welders. In 2020, the total dose of PM0.1 ranged from 0.5 to 2.6 μg in machining researchers and from 1.3 to 6.7 μg in welders. As can be seen in , personal exposure expressed as dose and concentration appeared higher in the year 2020. However, the difference was not significant.
Table 3. The dose and concentration of particulate matter measured by personal nanoparticle sampler in both years.
Characteristics of subjects
No statistically significant differences were found in age, BMI, smoking, physical activity or alcohol consumption between the researchers and controls in 2019 and 2020 ().
The exposure characteristics of a total of 43 researchers exposed to engineered nanoparticles (21 in machining and 22 in welding) did not differ in 2019 and 2020, as shown in .
Table 4. Exposure characteristics of the researchers.
Lung functions & FeNO
No significant differences were found in either year in either pre- or post-exposure spirometry in the researchers as compared with controls. In addition, FeNO did not differ in the researchers after exposure as compared with the pre-exposure measurement.
Environmental air pollution
All measured levels of environmental pollutants (SO2, NO2, O3, PM2.5 and PM10) were classified as low or mild for both years. These concentrations did not significantly differ among the four mean levels of measurements, as shown in .
Table 5. Mean actual concentrations of local environmental air pollutants in 2019 and 2020.
Markers of antioxidant status & oxidative stress
The values of markers measured in groups of subjects are shown in . No differences were found between markers studied in the researchers performing machining and welding in both 2019 and 2020; the results from both years were merged together.
Means and standard deviations of the levels of GSH in the researchers and in the controls in plasma, urine and EBC in merged 2019 and 2020.
*p < 0.05; **p < 0.01; ***p < 0.001).
creat.: Creatinine; EBC: Exhaled breath condensate; GSH: Glutathione.
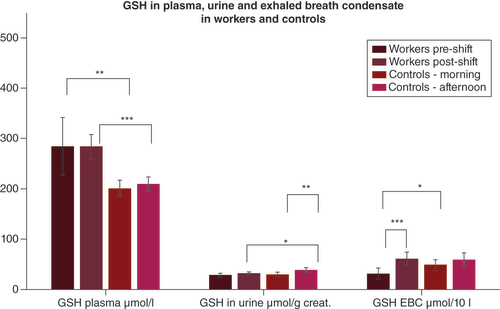
Means and standard deviations of the levels of FRAP in the researchers and in the controls in plasma and urine in merged 2019 and 2020.
*p < 0.05.
creat.: Creatinine; FRAP: Ferric-reducing antioxidant power.
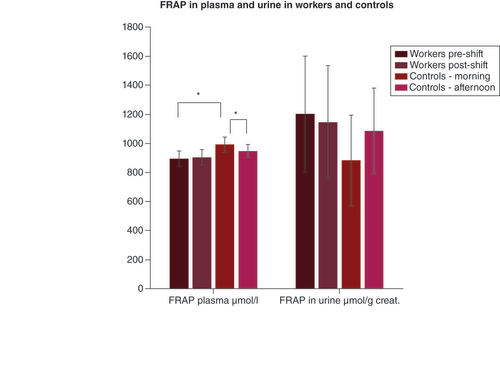
Means and standard deviations of the levels of TBARS in the researchers and in the controls in plasma, urine and EBC in merged 2019 and 2020.
*p < 0.05; ***p < 0.001.
creat.: Creatinine; EBC: Exhaled breath condensate; TBARS: Thiobarbituric acid reactive substance.
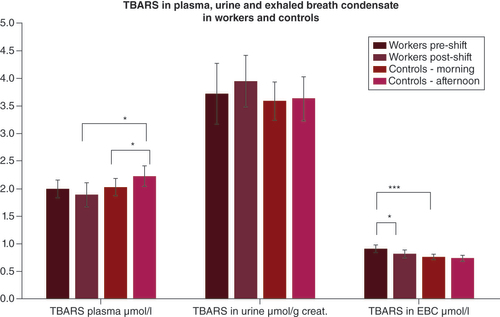
A total of 32 EBC and five plasma samples were excluded due to sample turbidity.
Glutathione
Chronic effect
The pre-exposure samples, potentially reflecting chronic exposure, showed that the concentration of GSH, a ROS scavenger, was higher in the plasma of the researchers than in the morning plasma samples of the controls. No difference was found in urine samples.
Acute effect
No postexposure change was seen in the plasma GSH of the researchers; however, postexposure plasma GSH was higher as compared with controls.
No postexposure change in urine GSH was seen in the researchers. In addition, it increased in the controls as compared with the morning samples. In EBC, postexposure GSH in the researchers increased, as shown in .
Ferric-reducing antioxidant power
As the majority of EBC samples (75.0%) in both groups did not reach the LOQ of the method, EBC values could not be calculated; therefore only plasma and urine markers are shown in . In addition, FRAP in EBC was also excluded from further calculations, including correlations.
Chronic effect
The pre-exposure plasma antioxidant marker FRAP of the researchers was significantly lower as compared with the morning samples of the controls.
Acute effect
The acute effect of exposure was not seen in plasma or urine. FRAP in plasma did not change in the researchers postexposure; however, it decreased in the afternoon samples of the controls.
Thiobarbituric acid reactive substances
Chronic effect
Among the pre-shift samples, the oxidative stress marker TBARS was only higher in researchers’ EBC samples, as shown in .
Acute effect
No elevation of postexposure TBARS as compared with pre-shift TBARS was found in the researchers in any fluid examined. Surprisingly, the only significant change was a decrease in EBC TBARS; a similar trend was seen in the plasma but not in the urine.
Correlations
Correlations of the pre-exposure & postexposure markers
Pre-exposure GSH correlated with postexposure levels in all biological fluids. Similarly, FRAP and TBARS plasma and urine levels pre-exposure correlated with postexposure levels; such a correlation, however, was missing in the EBC.
In all subjects studied, no correlation was found for GSH concentration with age, smoking or alcohol consumption.
Correlations of the markers with personal exposure measured by PENS
In both years, a significant positive association was observed between several parameters of exposure in the workshops and postexposure plasma GSH of the researchers. In 2019, the association was in the air concentration of particles PM0.1–PM4 (r = 0.542; p < 0.05); in 2020, in air concentration of particles PM4 (r = 0.464; p < 0.05), concentration PM0.1 (r = 0.440; p < 0.05) and PM0.1 dose for the monitored exposure time (r = 0.446; p < 0.05). No correlation was found between exposure parameters and the markers TBARS and FRAP.
Correlations of markers with environmental air pollution
Several correlations were found, many of which were contradictory, both in the researchers and in the controls, and the same air pollution parameter showed opposite correlations; that is, first a positive and then a negative one.
The plasma antioxidant parameter GSH was positively correlated with the environmental parameters SO2, O3, PM2.5 and PM10. However, it was negatively correlated with two of them (SO2 and PM2.5) in the afternoon.
Interestingly, no positive correlation between plasma and EBC oxidative stress marker TBARS was found in the researchers, while opposite correlations were seen in the controls, both in plasma and EBC TBARS with PM2.5.
In the controls, there were three significant changes in the afternoon compared with the morning samples. The first was the elevation of GSH in the urine; the second, the decrease of FRAP in plasma; and the third, the increase of TBARS in plasma. Likewise, none of them corresponded to a logical change in the environmental markers.
Discussion
The testing of antioxidant status using GSH, FRAP and the oxidative stress marker TBARS in researchers exposed to nanoparticles for almost 14 years was carried out in an identical working environment as in our preceding studies in the years 2016–2018 [Citation26].
The most important finding is that GSH was elevated, as compared with the controls. This marker of antioxidant status was higher both pre-exposure (which relates to previous, i.e., chronic, exposures in these researchers) and postexposure as compared with the control group. Importantly, plasma GSH levels were significantly correlated with several parameters of individual exposure measured by PENS in both years, such as the sum of sample mass on the filters, the concentration of nanoparticles corrected by SMPS/APS, and the total nanoparticle dose (PM0.1) for the approximately 3-h monitored period of exposure, as shown in . The postexposure elevation of GSH in EBC was significant; urine, on the other hand, did not show an increase in postexposure GSH in the researchers.
The elevations of this marker of antioxidant capacity in plasma and EBC may potentially reflect the subjects’ adaptation to suppress oxidative stress. The pathological stimulus by nanoparticles was likely relatively mild and within the limits of the organism’s ability to defend itself against it. The human body has the potential to produce low-molecular-weight antioxidants by increasing the level of GSH in the plasma and EBC, thereby mitigating the pathological effects of the nanoparticles [Citation34]. The rapid response to the high exposure in the EBC agrees with the high potential of the response of the respiratory system due to its high level of GSH, especially in the lining fluid of the lungs [Citation26].
In plasma, GSH may be increased by the induction of GSH reductase production in some organs, especially in muscles, as was observed in laboratory animals exposed to sulfur mustard; however, in urine, the level of GSH is not maintained due to the limited activity of GSH reductase [Citation35]. A similar finding was observed in sulfur mustard-exposed laboratory animals used in military research, when the amount of the toxicant administered was well below the median lethal dose [Citation36]. Another factor may be the delayed appearance of some markers in the urine and the collection of urine samples 4–5 h after the start of exposure to nanoparticles.
Plasma GSH in the researchers was not affected by personal characteristics such as lifestyle, age, BMI, smoking or occasional alcohol intake. Despite a small number of correlations with parameters of environmental air pollution, albeit still within normal limits, the results were not affected. The correlations were frequently contradictory for the same environmental pollution marker, as was also seen in the controls. Of importance, the sampling of both groups of subjects within a very short timespan should eliminate both environmental influences and the diurnal variability of GSH [Citation37]. Furthermore, in our previous studies using HPLC–MS/MS, no interfering effects of environmental air pollution were found with markers of oxidative stress [Citation38].
These new data concerning GSH, although not completely uniform, support the hypothesis of an adaptation of the function of the genome to chronic occupational exposure in humans, as detected by DNA methylation studies in these researchers exposed to nanoparticles in previous years [Citation39–41]. A significant difference in modified CpG dinucleotides was found in genes related to the immune system, signaling pathways, or cancer development [Citation39]. The study, which focused on the dynamics of DNA methylation during the years 2016–2018 in both exposed and control subjects, showed an association with the fixation of relevant epigenetic changes related to chronic exposure [Citation41]. These results suggest a possible adaptation of the organism against the harmful effect of nanoparticles after repeated low occupational burden.
To the best of our knowledge, GSH has not yet been studied in researchers exposed to nanoparticles. An increase in GSH was described in miners after exposure to mercury vapors [Citation42]. On the contrary, a study attributed the depletion of GSH in bronchoalveolar lavage or plasma to a massive and rapid attack of chemical agents (e.g., sulfur mustard) [Citation43].
The second antioxidant status marker, measured as FRAP, showed lower pre-exposure levels in the researchers. However, no decrease after exposure was observed, which corresponds to the FRAP content in the organs of animals exposed to sulfur mustard [Citation35]. The FRAP concentration in most EBC samples did not reach the LOQ of the method. Furthermore, the high intra- and inter-individual variability of FRAP in humans remains a complication. In earlier studies, FRAP reflected several external factors, such as alcohol consumption, diet, obesity, sedentary behavior, ascorbic acid intake and other constituents found, which makes using FRAP very complicated [Citation44,Citation45]. Therefore, the researchers and controls in our study had to have comparable characteristics in age, BMI, regularity of physical activities and lifestyle, which makes the monitoring process rather demanding.
TBARS, a marker of lipid oxidation, did not confirm data from our previous studies, where MDA and other markers of oxidation of lipids, measured by HPLC–MS/MS, reflected very well both the acute and chronic effects of engineered nanoparticle exposure in the identical research workplace in 2016–2018 [Citation26]. As MDA is the main component of TBARS and the working conditions did not substantially change, we suppose that the spectrophotometric method is not sensitive enough for the analysis of oxidative stress in researchers.
Several studies have already reported an increase in oxidative stress markers in researchers exposed to nanoparticles using sensitive analytical methods. An increase in oxidative damage markers was detected in researchers exposed to welding fumes (92% nanoparticles) [Citation46]. Another study confirmed the effect on leukocyte DNA methylation, oxidative DNA damage and lipid peroxidation in a group exposed to nano-metal oxides (TiO2, SiO2, indium oxide and tin oxide) [Citation47].
Nanomaterial-handling researchers exposed to the nano TiO2 pigment in our studies had increased levels of lipid oxidation markers (MDA, 4-hydroxy-trans-hexenal, 4-hydroxy-trans-nonenal, 8-isoprostaglandin F2α and aldehydes C6–C12), nucleic acid oxidation markers (8-hydroxy-2-deoxyguanosine, 8-hydroxyguanosine, 5-hydroxymethyluracil) and biomarkers of protein oxidative damage (o-tyrosine, 3-chlorotyrosine and 3-nitrotyrosine) in the EBC [Citation38,Citation48]. This finding was warranted by a recent systematic review and meta-analysis study [Citation49]. Another study confirmed the relationship between exposure to copier-emitted nanoparticles and urinary levels of markers of oxidative damage (8-hydroxyguanosine, 8-hydroxy-2-deoxyguanosine, 8-isoprostaglandin F2α, 4-hydroxynonenal) which were elevated as a result of both acute exposure (in the samples collected after 6, 24 or more hours since the beginning of exposure) and chronic exposure [Citation50].
EBC proved to be an excellent matrix for evaluating the effect of nanoparticles, as particles of 10–50 μm in size were preferentially concentrated in the EBC of researchers. In addition, their proportion reflected the length and character of their exposure, regardless of the type of metal [Citation51].
Recently, EBC and urine have been selected for the harmonized protocol of the international multicenter prospective study of nanotechnology researchers called the NanoExplore Cohort [Citation52].
Plasma GSH, although slightly more invasive, might be considered as an additional marker to evaluate potential adaptations of the subjects.
There are several limitations to the present study, the main one being the relatively low number of exposed subjects. One of the reasons is that plants handling nanocomposites typically employ a small number of researchers, as was the case with the research plant studied. Then, as discussed above, there are several potential factors influencing oxidative stress markers. Therefore, in this study, a great effort was made to examine comparable groups of subjects with respect to age, demographic data, lifestyle factors, location and its environmental pollution, and time of day.
Finally, the most important limitation is the type of method used for the analysis of oxidative stress – spectrophotometry – in light of the lower sensitivity and high individual variability of the results, especially in the EBC. This lower sensitivity in EBC, especially given the number of subjects, may be the explanation of the fact that the pre-exposure GSH was not elevated, as would be expected in chronic exposure. In addition, further experiments are needed to confirm the lack of a relationship between the nanoparticles exposure and FRAP or TBARS levels.
Conclusion
Antioxidant status evaluated using GSH was increased in researchers who had long-term exposure to inhaled engineered nanoparticles, both before and after exposure. The postexposure plasma GSH correlated with individual researchers’ nanoparticle exposure as measured by PENS. In addition, postexposure GSH in EBC increased. These results suggest the adaptation to chronic exposure to nanoparticles and delayed consequences associated with exposure to asbestos [Citation53] and silica [Citation54] do not seem probable. GSH measurement by spectrophotometry should be further tested for the assessment of antioxidant capacity and adaptation to oxidative stress. On the other hand, due to its low sensitivity, spectrophotometric measurement using TBARS cannot replace HPLC–MS/MS methods for the measurement of biomarkers of oxidative stress.
Researchers exposed in the long term (~14 years) to inhaled engineered nanoparticles developed increased antioxidant capacity, as measured by glutathione (GSH).
The results suggest that the pre-exposure elevation of antioxidation status in the researchers is associated with chronic exposure and may reflect the adaptation of their organism to oxidative stress.
Postexposure GSH in plasma correlated with short (3 h) exposure to nanoparticles, as measured individually by personal nanoparticle sampler.
GSH in exhaled breath condensate postexposure increased, as supposedly did its concentration in the lining fluid. GSH may react quickly, and the airway is the place where nanoparticles are most retained.
The measurements of GSH in plasma proved that the effect of inhalation of nanoparticles is not limited to the respiratory tract, but a systemic effect is probable.
Spectrophotometric measurements of thiobarbituric acid reactive substances, due to their low sensitivity, cannot replace HPLC–MS/MS methods for the measurement of oxidative stress in periodic examinations. This is especially important for exhaled breath condensate analysis.
Analysis of thiobarbituric acid reactive substances is not an adequate substitute for specific markers of oxidative stress, such as malondialdehyde or 8-hydroxy-2′-deoxyguanosine using HPLC, for use in practice in smaller groups of subjects.
This study brings new perspectives related to antioxidant capacity of exposed subjects. GSH measured by spectrophotometry can be recommended for the assessment of antioxidant capacity and adaptation to oxidative stress.
Further investigations are necessary to draw firm conclusions regarding adaptation, and precautionary principles should be maintained. Nevertheless, the results of our study show a more optimistic possibility of avoiding delayed consequences associated with exposure to asbestos and silica.
Author contributions
D Pelclova, V Zdimal, S Dvorackova and A Rossnerova conceived and designed the study; D Pelclova, L Lischkova, V Zdimal, L Ondrackova, J Schwarz, J Ondracek, S Vlckova, P Klusackova, V Kolesnikova, M Kostejn and Z Fenclova performed the field study; L Lischkova drafted the manuscript; M Pohanka analyzed the samples; T Navratil, L Ondrackova, V Zdimal and P Bradna performed data analysis; D Pelclova, V Zdimal and P Bradna performed data acquisition; T Navratil and D Pelclova designed the figures; D Pelclova and P Klusackova adapted the manuscript. All authors revised the manuscript critically and approved the final version.
Writing disclosure
No writing assistance was utilized in the production of this manuscript.
Ethical conduct of research
The authors state that they have obtained appropriate institutional review board approval or have followed the principles outlined in the Declaration of Helsinki for all human experimental investigations. In addition, for investigations involving human subjects, informed consent has been obtained from the participants involved.
Acknowledgments
The authors thank L Windell for editing the English language of the manuscript.
Financial disclosure
This study was supported by Cooperatio 207041-3 of the Charles University and Czech Science Foundation (GA ČR no. 22-08358S). The authors have no other relevant affiliations or financial involvement with any organization or entity with a financial interest in or financial conflict with the subject matter or materials discussed in the manuscript apart from those disclosed. This includes employment, consultancies, honoraria, stock ownership or options, expert testimony, grants or patents received or pending, or royalties.
Competing interests disclosure
The authors have no competing interests or relevant affiliations with any organization or entity with the subject matter or materials discussed in the manuscript. This includes employment, consultancies, honoraria, stock ownership or options, expert testimony, grants or patents received or pending, or royalties.
Additional information
Funding
References
- Fischman M , Murashov V , Borak J , Seward J ; ACOEM Task Force on Nanotechnology and Health . Nanotechnology and health. J. Occup. Environ. Med. 61(3), E95–E98 (2019).
- Park SG , Kim TJ , Kim HM et al. Novel preparation method of carbon nano fibers/DAAQ electrode for supercapacitor system. Presented at: 2006 IEEE 8th International Conference on Properties & Applications of Dielectric Materials. Bali, Indonesia, 103–106 (2006). https://ieeexplore.ieee.org/document/4062617
- Vollath D , Fischer FD , Holec D . Surface energy of nanoparticles – influence of particle size and structure. Beilstein J. Nanotechnol. 9, 2265–2276 (2018).
- Chen LJ , Liu J , Zhang YL et al. The toxicity of silica nanoparticles to the immune system. Nanomedicine 13(15), 1939–1962 (2018).
- Bayda S , Adeel M , Tuccinardi T , Cordani M , Rizzolio F . The history of nanoscience and nanotechnology: from chemical-physical applications to nanomedicine. Molecules 25(1), (2020). doi.10.3390/molecules25010112
- Jakubczyk K , Druzga A , Katarzyna J , Skonieczna-Zydecka K . Antioxidant potential of curcumin – a meta-analysis of randomized clinical trials. Antioxidants 9(11), (2020). doi.10.3390/antiox9111092
- Pohanka M , Martinkova P , Brtnicky M , Kynicky J . Changes in the oxidative stress/anti-oxidant system after exposure to sulfur mustard and antioxidant strategies in the therapy, a review. Toxicol. Mech. Methods 27(6), 408–416 (2017).
- Dayem AA , Hossain MK , Lee SB et al. The role of reactive oxygen species (ROS) in the biological activities of metallic nanoparticles. Int. J. Mol. Med. Sci. 18(1), (2017). doi:10.3390/ijms18010120
- Khanna P , Ong C , Bay BH , Baeg GH . Nanotoxicity: an interplay of oxidative stress, inflammation and cell death. Nanomaterials 5(3), 1163–1180 (2015).
- Giustarini D , Colombo G , Garavaglia ML et al. Assessment of glutathione/glutathione disulphide ratio and S-glutathionylated proteins in human blood, solid tissues, and cultured cells. Free Radic. Biol. Med. 112, 360–375 (2017).
- Ushio-Fukai M , Ash D , Nagarkoti S , De Chantemele EJB , Fulton DJR , Fukai T . Interplay between reactive oxygen/reactive nitrogen species and metabolism in vascular biology and disease. Antioxid. Redox Signal. 34(16), 1319–1354 (2021).
- Najahi-Missaoui W , Arnold RD , Cummings BS . Safe nanoparticles: are we there yet? Int. J. Mol. Med. Sci. 22(1), (2021). doi:10.3390/ijms22010385
- Huang YW , Cambre M , Lee HJ . The toxicity of nanoparticles depends on multiple molecular and physicochemical mechanisms. Int. J. Mol. Med. Sci. 18(12), (2017). doi:10.3390/ijms18122702
- Aggarwal V , Tuli HS , Varol A et al. Role of reactive oxygen species in cancer progression: molecular mechanisms and recent advancements. Biomolecules 9(11), (2019). doi:10.3390/biom9110735
- Monteiro HP , Rodrigues EG , Reis AKCA et al. Nitric oxide and interactions with reactive oxygen species in the development of melanoma, breast, and colon cancer: a redox signaling perspective. Nitric Oxide Biol. Chem. 89, 1–13 (2019).
- Mendoza RP , Brown JM . Engineered nanomaterials and oxidative stress: current understanding and future challenges. Curr. Opin. Toxicol. 13, 74–80 (2019).
- Schulte P , Leso V , Niang M , Iavicoli I . Biological monitoring of workers exposed to engineered nanomaterials. Toxicol. Lett. 298, 112–124 (2018).
- Ghafari J , Moghadasi N , Shekaftik SO . Oxidative stress induced by occupational exposure to nanomaterials: a systematic review. Ind. Health 58(6), 492–502 (2020).
- Bello D , Chanetsa L , Christophi CA et al. Biomarkers of oxidative stress in urine and plasma of operators at six Singapore printing centers and their association with several metrics of printer-emitted nanoparticle exposures. Nanotoxicology 16(9–10), 913–934 (2022).
- Aldakheel FM , Thomas PS , Bourke JE , Matheson MC , Dharmage SC , Lowe AJ . Relationships between adult asthma and oxidative stress markers and pH in exhaled breath condensate: a systematic review. Allergy 71(6), 741–757 (2016).
- Horvath I , Barnes PJ , Loukides S et al. A European Respiratory Society technical standard: exhaled biomarkers in lung disease. Eur. Resp. J. 49(4), (2017). doi:10.1183/13993003.00965-2016
- Caffarelli C , Calcinai E , Rinaldi L , Dascola CP , Terracciano L , Corradi M . Hydrogen peroxide in exhaled breath condensate in asthmatic children during acute exacerbation and after treatment. Respiration 84(4), 291–298 (2012).
- Fesen K , Silveyra P , Fuentes N et al. The role of microRNAs in chronic pseudomonas lung infection in cystic fibrosis. Respir. Med. 151, 133–138 (2019).
- Muccilli V , Saletti R , Cunsolo V et al. Protein profile of exhaled breath condensate determined by high resolution mass spectrometry. J. Pharm. Biomed. Anal. 105, 134–149 (2015).
- Pelclova D , Zdimal V , Kacer P et al. Leukotrienes in exhaled breath condensate and fractional exhaled nitric oxide in workers exposed to TiO2 nanoparticles. J. Breath Res. 10(3), (2016). doi:10.1088/1752-7155/10/3/036004
- Pelclova D , Zdimal V , Komarc M et al. Three-year study of markers of oxidative stress in exhaled breath condensate in workers producing nanocomposites, extended by plasma and urine analysis in last two years. Nanomaterials 10(12), (2020). doi:10.3390/nano10122440
- Syslova K , Kacer P , Kuzma M et al. LC–ESI–MS/MS method for oxidative stress multimarker screening in the exhaled breath condensate of asbestosis/silicosis patients. J. Breath Res. 4(1), 017104 (2010).
- Sotgia S , Fois AG , Paliogiannis P , Carru C , Mangoni AA , Zinellu A . Methodological fallacies in the determination of serum/plasma glutathione limit its translational potential in chronic obstructive pulmonary disease. Molecules 26(6), (2021). doi:10.3390/molecules26061572
- Benzie IFF , Strain JJ . The ferric reducing ability of plasma (FRAP) as a measure of ‘antioxidant power’: the FRAP assay. Anal. Biochem. 239(1), 70–76 (1996).
- Aguilar Diaz de Leon J , Borges CR . Evaluation of oxidative stress in biological samples using the thiobarbituric acid reactive substances assay. J. Vis. Exp. (159), 10.3791/61122 (2020).
- Gaucher C , Boudier A , Bonetti J , Clarot I , Leroy P , Parent M . Glutathione: antioxidant properties dedicated to nanotechnologies. Antioxidants 7(5), 62 (2018).
- Young LH , Lin YH , Lin TH et al. Field application of a newly developed personal nanoparticle sampler to selected metal working operations. Aerosol Air Qual. Res. 13(3), 849–861 (2013).
- Pelclova D , Zdimal V , Schwarz J et al. Markers of oxidative stress in the exhaled breath condensate of workers handling nanocomposites. Nanomaterials 8(8), (2018). doi:10.3390/molecules26061572
- Gould NS , Min E , Gauthier S , Martin RJ , Day BJ . Lung glutathione adaptive responses to cigarette smoke exposure. Respir. Res. 12 (2011). doi:10.1186/1465-9921-12-133
- Pohanka M , Stetina R , Svobodova H et al. Sulfur mustard causes oxidative stress and depletion of antioxidants in muscles, livers, and kidneys of Wistar rats. Drug Chem. Toxicol. 36(3), 270–276 (2013).
- Pohanka M , Sobotka J , Jilkova M , Stetina R . Oxidative stress after sulfur mustard intoxication and its reduction by melatonin: efficacy of antioxidant therapy during serious intoxication. Drug Chem. Toxicol. 34(1), 85–91 (2011).
- Blanco RA , Ziegler TR , Carlson BA et al. Diurnal variation in glutathione and cysteine redox states in human plasmas. Am. J. Clin. Nutr. 86(4), 1016–1023 (2007).
- Pelclova D , Zdimal V , Kacer P et al. Markers of lipid oxidative damage in the exhaled breath condensate of nano TiO2 production workers. Nanotoxicology 11(1), 52–63 (2017).
- Rossnerova A , Honkova K , Pelclova D et al. DNA methylation profiles in a group of workers occupationally exposed to nanoparticles. Int. J. Mol. Med. Sci. 21(7), (2020). doi:10.3390/ijms21072420
- Rossnerova A , Izzotti A , Pulliero A , Bast A , Rattan SIS , Rossner P . The molecular mechanisms of adaptive response related to environmental stress. Int. J. Mol. Med. Sci. 21(19), (2020). doi:10.3390/ijms21197053
- Rossnerova A , Honkova K , Chvojkova I et al. Individual DNA methylation pattern shifts in nanoparticles-exposed workers analyzed in four consecutive years. Int. J. Mol. Med. Sci. 22(15), (2021). doi:10.3390/ijms21197053
- Kobal AB , Prezelj M , Horvat M , Krsnik M , Gibicar D , Osredkar J . Glutathione level after long-term occupational elemental mercury exposure. Environ. Res. 107(1), 115–123 (2008).
- Tahmasbpour E , Emami SR , Ghanei M , Panahi Y . Role of oxidative stress in sulfur mustard-induced pulmonary injury and antioxidant protection. Inhal. Toxicol. 27(13), 659–672 (2015).
- Staruchova M , Collins AR , Volkovova K et al. Occupational exposure to mineral fibres. Biomarkers of oxidative damage and antioxidant defence and associations with DNA damage and repair. Mutagenesis 23(4), 249–260 (2008).
- Cruz Dos Santos RR , Rosa ECC , Gris EF et al. Association between lifestyle and oxidative stress markers in young active men. J. Exercise Physiol. 22(3), 75–86 (2019).
- Liou SH , Wu WT , Liao HY et al. Global DNA methylation and oxidative stress biomarkers in workers exposed to metal oxide nanoparticles. J. Hazard. Mater. 331, 329–335 (2017).
- Graczyk H , Lewinski N , Zhao JY et al. Increase in oxidative stress levels following welding fume inhalation: a controlled human exposure study. Part. Fibre Toxicol. 13(1), 31 (2016).
- Pelclova D , Zdimal V , Fenclova Z et al. Markers of oxidative damage of nucleic acids and proteins among workers exposed to TiO2 (nano) particles. Occup. Environ. Med. 73(2), 110–118 (2016).
- Luo X , Xie D , Hu J , Su J , Xue Z . Oxidative stress and inflammatory biomarkers for populations with occupational exposure to nanomaterials: a systematic review and meta-analysis. Antioxidants 11(11), 2182 (2022).
- Zhang YP , Bello A , Ryan DK , Demokritou P , Bello D . Elevated urinary biomarkers of oxidative damage in photocopier operators following acute and chronic exposures. Nanomaterials 12(4), (2022). doi:10.3390/nano12040715
- Fireman E , Edelheit R , Stark M , Bar Shai A . Differential pattern of deposition of nanoparticles in the airways of exposed workers. J. Nanopart. Res. 19(2), (2017). doi:10.1007/s11051-016-3711-8
- Canu IG , Plys E , Creze CV et al. A harmonized protocol for an international multicenter prospective study of nanotechnology workers: the NanoExplore cohort. Nanotoxicology 17(1), 1–19 (2023).
- Pelclova D , Fenclova Z , Kacer P , Kuzma M , Navratil T , Lebedova J . Increased 8-isoprostane, a marker of stress in exhaled breath condensate in subjects with asbestos exposure. Ind. Health 46(5), 484–489 (2008).
- Pelclova D , Fenclova Z , Kacer P et al. 8-isoprostane and leukotrienes in exhaled breath condensate in Czech subjects with silicosis. Ind. Health 45(6), 766–774 (2007).