Abstract
The aim of this work was to investigate the impact of intercellular contact during radiation exposure on cell survival in regions of reduced dose. Methods. The PC3 human prostate adenocarcinoma cell line was irradiated using a 6 MV x-ray beam to assess clonogenic cell deaths with the specific aim to investigate cell survival in a dose cold spot. Radiation-induced cell survival in a 20% lower dose region, compared to that of cells receiving 100% of the prescribed dose (2 Gy), was assessed for experimental set-ups when under-irradiated cells were either in direct contact with cells receiving 2 Gy or irradiated separately. In addition, the results were compared against non-irradiated controls. Results. A significant (p < 0.001) decrease in cell survival was found when cells, collocated in the same flask, received either 100% or 80% of the prescribed dose (the dose distribution contained a cold spot of 20% lower dose) compared to non-irradiated cells. However, in the experiment in which the entire flask was exposed to only 80% of the prescribed dose, the mean difference in cell survival compared to non-irradiated control was not significant (p > 0.05). This was contrary to a significant decrease (p < 0.001) in survival of cells receiving 100% of the prescribed dose versus the control. Additionally, significant reduction (p < 0.05) in cell survival was observed for cells which were under-irradiated by 20% but collocated in the same flask with cells receiving 100% dose compared to cells where the entire flask was irradiated with 80% of the prescribed dose. Conclusion. For the given cell line, under existing growing and treatment conditions, the cell survival in the dose cold spot region was significantly lower when under-irradiated cells were in contact with the cells receiving 100% of the prescribed dose compared to survival of cells under-irradiated by the same amount of radiation but treated separately to cells receiving 100% dose.
Prostate cancer is one of the most common cancers and the second highest cause of cancer deaths in men. Radiation therapy (RT) combined with hormone therapy has been successful in curing this disease [Citation1]. RT treatment modalities currently used worldwide include permanent trans-perineal low dose rate brachytherapy, external-beam radiotherapy combined with low or high dose rate brachytherapy boost, intensity-modulated radiotherapy (IMRT) and three-dimensional (3D) conformal radiotherapy. Treatment success of either of these modalities is usually assessed on whether local tumor control has been maximized while keeping normal tissue complications at a minimum. This is, however, not always achievable due to conflicting demands of a desired radiation dose to tumor volume and dose constraints to healthy structures which may result in dose cold spots within a treatment volume – a well-known problem for radiotherapy.
Theoretically, dose cold spots in a small part of the treated volume should not have a significant effect on the objective of the physical dose plan, especially when taking into account that only 1% or less of clonogenic cells are capable of unlimited proliferation within a tumor [Citation2]. However, it has been shown that tumor control probability (TCP) can be greatly diminished as a result of dose cold spots. Tome et al. evaluated TCP loss due to dose cold spots within a tumor as a function of dose reduction and the under-dosed volume. The study demonstrated that dose cold spots can be deleterious to TCP if the cold dose is lower by more than 10% of the prescribed dose for fractions of cold volumes as small as 1% [Citation3]. This indicates that cold spots cannot be ignored and require time-consuming adjustments to a treatment plan.
In the last decade, the long-held DNA centric radiation-damage model has been questioned due to experimental evidence of biological responses in cells not directly hit by ionizing radiation that cannot be explained by the direct DNA damage cell killing mechanism [Citation4,Citation5]. These radiation-induced phenomena in non-targeted cells include increased radio resistance, adaptive responses, long-lasting alterations in gene expression, genomic instability, sister chromatid exchanges, low dose hyper-radio sensitivity, mutagenesis, reproductive cell death, micronucleus formation, increased apoptosis and others [Citation6]. The multifaceted nature of this phenomenon in normal and cancerous cells results in controversy in terms of being either potentially detrimental or potentially beneficial to radiotherapy treatment outcome.
The treatment prescription in clinical radiotherapy is based on the assumption that radiobiological response in the target volume is proportional to the dose received. However, recent studies [Citation7,Citation8] reported a significant decrease in cell survival following in vitro cell exposure to a 6 MV modulated x-ray field (compared to a non-modulated x-ray field) that cannot be predicted using the classical dose response relationship. These in vitro studies have suggested that intercellular communication may play a role in cell survival response after modulated exposures.
Correspondingly, the main objective of the current work was to investigate differences in cell survival in under-dosed versus fully-dosed regions for cells which were in direct contact with each other during and shortly after radiation treatment versus cells which were separated. It was hypothesized that dose cold spots in treatment plans may not lead to effects as significant as predicted by Tome [Citation3], especially for very small volumes. This is because a small amount of clonogenic cells left in an under-dosed region could be inactivated as a result of inter-cellular signaling. To test this hypothesis, cellular responses to irradiation, using a 6 MV x-ray field modulated by partial shielding, were investigated and assessed by clonogenic cell death.
Material and methods
Cell culture
The PC3 human prostate epithelial adenocarcinoma cells were cultured as a monolayer in RPMI 1640 (Sigma, USA) cell growth medium, supplemented with 10% fetal calf serum (FCS) (Gibco, Australia), 1% L-Glutamine, 1% sodium pyruvate, 1% ascorbate, 1% penicillin/streptomycin at 37oC in a humidified atmosphere containing 5% /CO2. Preliminary experiments were conducted to establish the colony forming efficiency and doubling time of the PC3 cell line. The numbers of sham irradiated (i.e. control) cells were plated to yield the survival rate established in the preliminary experiment which evaluated plating efficiency.
Clonogenic assay
The clonogenic assay technique of Pluck and Marcus was used [Citation9]. Approximately 1.3 × 106 cells per flask were plated in T75 (Corning, USA) flasks 24 h prior irradiation at 37oC /5% CO2. Ten minutes prior to irradiation, growth media was replaced with phosphate buffered saline (PBS). Following irradiation, the PBS was removed by aspiration and cells were washed with Hanks balanced salt solution (HBSS) and harvested from flasks – released by incubation with trypsin for 5 min in 37oC/5% CO2. Cells were resuspended in HBSS, containing 5% FCS and centrifuged (4000 rpm, 4oC). Cell pellets were then resuspended in RPMI 1640 growth media. Cell numbers were determined using trypan blue exclusion. Cells were plated into six well dishes (Corning, USA). Depending on the radiation dose received, the density of plated cells ranged from 200 cells/well for control flasks to no more than 3300 cells/well for flasks receiving 8 Gy dose. Cells were cultured for two weeks according to the estimated doubling time in order to mature into colonies containing more than 50 cells per colony and to exclude cells which had limited growth. Growth media was very carefully replaced every 4–5 days to avoid disturbance of colonies through media changing. Colonies were then fixed with methanol, stained with crystal violet and the number of clonogen colonies was counted using a stereomicroscope.
The colony forming efficiency (CFE) was evaluated from the cell survival of untreated control.
Cell survival itself for the PC3 cell line was determined from two independent experiments using six-fold sampling for the first measurement and three-fold sampling for the second measurement (nine samples in total). Cell survival data was plotted on a logarithmic scale as a function of dose received plotted on a linear scale. The dose which killed half of clonogenic population (D50) as well as the fraction of cells that survived the standard clinical daily dose of 2 Gy (SF2) as used in current curative radiation protocols were estimated from this graph.
Dose cold spot experiment
The modulated irradiation experiment, designed in this work to generate a dose cold spot in communicating cells, involved four different irradiation set ups, all using a 6 MV x-ray beam (see ):
Figure 1. Stages of dose cold spot experiment performed applying five different types of radiation treatments: non-irradiated control, ‘shielded’ cells received 80% of the prescribed dose, ‘open’ cells received 100% of the prescribed dose separately from shielded cells, ‘slide’ and ‘flask’ cells received 80% and 100% of the prescribed dose in the same flask.
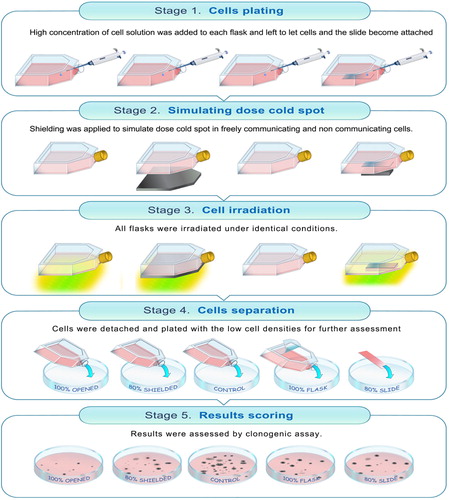
The first group/flask of cells was not irradiated and used for control purposes (called ‘control’ in the following paragraphs) [Citation2]. Another flask was to receive 100% of the prescribed 2 Gy dose. This standard clinical daily 2 Gy dose was selected after confirmation of D50 and SF2 for the PC3 cell line. For this flask and its cells the nomenclature ‘open’ is used in the following text [Citation3]. The next cell flask was to receive 80% of the prescribed 2 Gy dose. This flask is called ‘shielded’ as lead shielding/attenuator of appropriate thickness was used to achieve the required dose reduction. As the cells in the open and shielded flasks were irradiated, processed and plated separately, they represent irradiation measurements with non-communicating cells exposed either to either 100% or 80% of 2 Gy dose [Citation4]. The last flask was a combination of the previous two irradiation set-ups, with some cells aimed to receive 100% and some cells to receive 80% of the prescribed 2 Gy dose. In this case, the cell groups exposed to two different doses were in direct contact with each other during irradiation and also 2 h after radiation exposure. The nomenclatures ‘flask’ (100% dose) and ‘slide’ (80% dose) are used in the paper as these names reflect the geometrical set up of the experiment, where cells receiving 80% of the dose were plated on a slide within the flask that (apart from the slide region) was exposed to 100% of the prescribed dose.
For ‘control’, ‘open’ and ‘shielded’ irradiations, cells were plated in high densities (7 × 104 cell/cm2) into corresponding Peel-Off Tissue Culture Flasks (TPP, Techno Plastic Products AG, Switzerland) and incubated for 48 h. For ‘flask’ and ‘slide’ radiation treatment a single peel-off flask was used. This had required additional preparation before the same amount of cells (7 × 104 cell/cm2) was plated. First, a very thin glass slide (5 cm × 2.1 cm and thickness of less than 0.1 cm), sterilized in pure ethanol, was inserted into peel-off flask using long tweezers and positioned in the middle of the flask. Afterwards, the complete RPMI-1640 cell growth media was carefully added into flask to avoid slide movements. This was followed by cell injection and flask placement into an incubator. Preliminary investigation regarding the plating efficiency of PC3 cell line grown on different types of plastic ware (T75 and 115 peel-off flasks) and glassware had been performed. The plating efficiency was found to be largely the same with differences of around 1%. This flask was also incubated for 48 h in order to allow cell adhesion and for the slide to adhere to the flask's surface as a result of tension forces and uniform cell growth on the top of the slide.
Ten minutes prior to irradiation the media in each flask was replaced with PBS. PBS was used instead of media to avoid secondary toxicity due to culture medium modifications as it has been demonstrated that the magnitude of the intercellular- mediated effects was dependent on the serotonin levels present in FCS [Citation10]. Following this, each group of cells, except the ‘control’ group, was treated with either 100% or 80% of the prescribed dose, applying appropriate lead shielding as described next. The dose cold spot in freely communicating cells was achieved by placing 5 cm × 2.1 cm × 0. 6 cm lead sheet to fully cover the cells growing on the slide only. The thickness (0.6 cm) of the lead attenuator used reduced the prescribed dose by 20%. This reduced dose was delivered to approximately 9% of the total cell population treated in this flask. The ‘open’ and ‘shielded’ flasks were irradiated as well. Radiation dose of 2 Gy was delivered to the ‘open’ flask. The ‘shielded’ flask was fully covered during irradiation with 0.6 cm thick lead sheet receiving as a result 80% of the prescribed dose (i.e. the same dose as cells in the ‘slide’ group).
Following the irradiations, cells were incubated for 2 h to allow for intercellular communication and then collected as described above in the clonogenic assay section. In order to harvest the cells growing on the slide, the slide was carefully removed from the flask and the ‘slide’ cells were separated from cells growing and treated within the rest of the flask. Cells were washed, resuspended, replated for the clonogenic assay and incubated for two weeks as described previously. The post-irradiation incubation time of 2 h was chosen based on published literature demonstrating significant increase in responsiveness of bystander cells when co-cultured with irradiated cells immediately or 1–3 h after irradiation [Citation11].
This experiment was repeated twice with triple samplings.
Results were scored 14 days after cell irradiation. Survival of cells from flasks irradiated separately with opened and shielded radiation fields was compared with survival of cells exposed to spatially non-uniform radiation beam (80% and 100% of the prescribed dose) within the same flask. Data was analyzed in Graph Pad Prism 5 software (GraphPad Software, Inc., CA, USA) with one-way Anova analysis of variances followed by Tukey’s Multiple Comparison Test.
Cell irradiation set-up and validation of irradiation procedure
Cell irradiations for clonogenic assay and dose cold spot experiments were performed at the Radiation Oncology Department, Royal Adelaide Hospital, using a 6 MV x-ray beam produced by a Varian 600CD linear accelerator (Varian, Palo Alto, CA, USA). Clinically applied nominal dose rate of 3 Gy/min was used. The flasks were placed on top of 1.5 cm thick solid water build up sheets (RW3, ρ = 1.0459g/cm3, PTW, Germany) to ensure that the cell line was positioned at the depth of maximum dose. Cells were treated posteriorly with gantry positioned at 180o (as cells were adhered to the bottom flask surface). In each case, radiation treatment was performed with the build-up surface at 100 cm from the beam focal spot and with 20 × 20 cm2 radiation field size. To minimize the effect of an air inhomogeneity on dose distribution and possible changes in scatter radiation due to an air gap above the cell monolayer surface, flasks were fully filled with PBS. Flasks were inserted in custom made wax phantoms (for different flask sizes) and 5 cm thick solid water slab was put on top of the flasks to ensure full scatter conditions. Accelerator radiation output, calibrated using IAEA TRS 398 protocol [Citation12] was routinely checked with Daily QA 3™ device (Sun Nuclear, USA) prior to all radiation treatments. The beam- on-time parameters, so-called monitor units (MU), were determined using chip LiF100 thermoluminecent dosimeters (TLDs). TLDs were irradiated using the same experimental setup, (i.e. they were positioned inside flasks at the location of the cell monolayer) to determine the MUs required to deliver 2 Gy absorbed dose to the cells. In the case of dose cold spot experiments, the thickness of lead shielding, which results in 20% dose reduction, was determined with farmer type NE 2577 0.2 cm3 ionization chamber (Nuclear Enterprises, England). Once the required lead thickness was found, it was again confirmed using LiF100 thermoluminescent chips; i.e. water-proofed TLD chips were placed on the beam central axis between flask's bottom surface and lead shielding. Flask was filled with water and irradiation was performed posteriorly using same 1.5 cm thick solid water build up sheets. The results confirmed dose reduction by 20%. In addition, gafchromic RTQA2 film (International Specialty Products, Wayne, New Jersey, USA) was positioned underneath the flask. The dose map obtained confirmed the position and dimensions of the cold spot as well as the sharpness of the penumbra between 100% and 80% dose regions.
Results
Clonogenic survival of PC3 cell line
The population doubling time of the PC3 cell line for the cell growing conditions used in the current work was found to be 48 h and the average colony forming efficiency (CFE) was around 0.60 (± 0.027). In the current work the mean survival fractions () were fitted with a linear-quadratic curve ().
Table I. Mean cell survival fractions and associated standard error values from two independent experiments using triplicate and six-fold samplings.
The dose required to kill half of the clonogenic population (D50) was determined to be 2.037 Gy, using the measured cell survival curve. The fraction of cells that survived the standard clinical daily dose of 2 Gy (SF2) was found to be 0.586 (± 0.0279). The SF2 values reported in the literature for the same PC3 cell line are shown in together with data determined in this work.
Dose cold spot experiment
Statistically significant (p < 0.001) decrease of up to 42.2% in cell survival was found between non-irradiated ‘control’ group and cells from the ‘open’ flask receiving 100% of the prescribed 2 Gy dose. This was in agreement with the above mentioned SF2 value of 0.586 (± 0.0279) determined by clonogenic assay. Furthermore, cell survival of cells from ‘open’ and ‘shielded’ flasks that received doses of 2 Gy and 80% of 2 Gy, respectively, was also found to be significantly different (p < 0.001). This indicates that cells which received 100% of the prescribed dose and cells under-dosed by 20% responded differently to doses delivered, when irradiated separately.
However, survival comparison between cells from ‘flask’ and ‘slide’ positions within the flask exposed to the modulated radiation field (with both groups of cells being in direct contact during and shortly after irradiation) did not indicate any statistically significant (p > 0.05) difference even though the delivered radiation dose also differed by 20% (see and ).
Figure 3. PC3 cell survival analysis after radiation-induced damage in the underdosed region with and without intercellular cellular communication. ‘Shielded’ and ‘open’ columns represent cell survival after receiving 80% and 100%of the prescribed dose separately. ‘Slide’ and ‘flask’ columns demonstrate survival data in cells which were in direct contact with each other and treated with 80% and 100% of the prescribed dose. In Graph Pad Prism 5 software the level of statistical significance can be chosen between 0.05, 0.01, or 0.001, which graphically presented as *, ** and *** symbols, respectively.
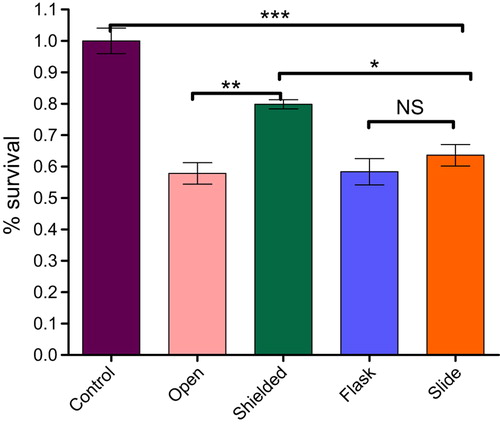
Table II. One-way ANOVA analysis of variances with Post Tukey’s Multiple Comparison Test of the PC3 cell survival in dose cold spot experiment.
However, there was a significant (p < 0.001) decrease in cell survival observed for ‘control’ versus ‘flask’ cells (41.6%) and ‘control’ versus ‘slide’ cells (36.4%). This indicated that communicating cells treated with 100% and 80% of the prescribed 2 Gy dose responded similarly (only 5.2% mean difference). These results are in contradiction with cell survival data from ‘open’ and ‘shielded’ flask irradiation groups (i.e. the same radiation doses applied to non-communicating cells) where the mean survival difference was 22.0%.
As mentioned above, survival decrease between cells from non-irradiated ‘control’ and ‘flask’ (100% of the prescribed 2 Gy dose) groups was 41.6%. This correlated well with the mean survival difference of 42.2% between ‘control’ and ‘open’ cell groups, treated with the same doses.
The mean difference of 16.2%, however, in survival between cells from ‘shielded’ and ‘slide’ groups (both exposed to 80% of the prescribed dose) was found to be statistically significant (p < 0.05); i.e. higher cell kill was observed in under-dosed cells when they were directly communicating with cells exposed to 100% of the prescribed dose.
In addition, using the measured cell survival curve (see ), the survival fraction corresponding to 1.6 Gy (i.e. 80% of 2 Gy) radiation dose was estimated to be 0.68. The survival fraction of cells from the ‘shielded’ flask irradiated with 1.6 Gy was found to be 0.79. These values agree within ± three standard deviations and therefore are not considered to be significantly different.
Discussion
It was hypothesized in the current work that radiation induced bystander killing caused by intercellular signaling may have an impact on cell survival of the PC3 prostate cancer cells in dose cold spots. To test this assumption, cellular capacity to produce sizeable colonies of descendants after radiation exposure was assessed by clonogenic assays. Clonogenic assay method was also used as the measured endpoint to quantify the extent of radiation damage as it includes all radiation-induced cell death modes. In addition, as it was noted that radiosensitivity of PC3 cells differed widely between laboratories, it was crucial to evaluate D50 independently rather than to rely on published data. Experimental uncertainties related to cell irradiation, including irradiation set-ups, and cell handling, such as growthing media contents, possibility of human error during cell plating or possibility of colonies disturbance through media changing may have a potential to contribute to the results reported in this study.
It has been demonstrated in the current work that survival of PC3 prostate cancer cells (for given growing and treatment conditions) was significantly different for under-dosed cells that were either in physical contact with cells receiving 100% of the prescribed 2 Gy dose or irradiated separately.
It has been concluded that reduced survival in under-dosed cells, when cells were in contact with cells irradiated with full 2 Gy dose, was most likely a result of intercellular communication and the ability to share the same stress chemical messengers (that mediate this communication, such as hormones, cytokines, growth regulators, etc.) secreted by irradiated cells. This conclusion has been based on the fact that cells growing on the ‘slide’ were treated with the same amount of radiation dose (80% of 2 Gy radiation dose), plated at the same densities and maintained in the exact same conditions as cells also irradiated with 80% dose but irradiated separately from the cells that received 100% dose. It could therefore be reasoned that intercellular communication might have contributed to the decrease in cell survival of cells collected from the ‘slide’.
Communication between cells is important for maintaining homeostasis, i.e. the physiological regulatory processes that keep the internal environment of a system in a constant state. It allows cells to sense changes in their microenvironment, to integrate internal or external signals, to respond to them by changes in transcriptional activity, metabolism, or other regulatory measures. Signaling pathways generally consist of widespread building blocks, such as receptors, extracellular signal-regulated kinase (ERK) or mitogen-activated protein kinase (MAPK) cascades, G proteins and small G proteins such as Ras, Rho, Rab, Ran, or Arf, etc [Citation13]. Signaling can be seen as a linear connection between input elements (the receptors) and output elements (such as regulators of gene expression). However, there is evidence that signaling pathways interact with each other, forming a network. In spite of their variety in function and design, many signaling pathways usually consist of the same essential components, which are often highly conserved through evolution and between species.
Investigations of specific signaling pathways and possible underlying mechanisms responsible for the observed variation in radio-responsiveness in dose cold spot regions were out of the scope of this study. However, it is likely that the variation was caused by increased amount of cytotoxins secreted into the culture medium by cells receiving higher radiation dose or by substances passing through gap junctions from the directly affected cells to neighboring cells causing additional damage in under-dosed cells [Citation14]. There is experimental evidence that cytokines, i.e. cell-signaling protein molecules, play a major role in the cellular response to radiation and can greatly affect intrinsic cellular radiosensitivity as well as the incidence and type of radiation tissue complications [Citation15–17].
Extensive work investigating non-targeted effects of ionizing radiation implied that there were several signaling mechanisms responsible for this phenomenon. Burr et al. suggested a few signaling pathways involving FasL, TNF-alpha, nitric oxide (NO), reactive oxygen species (i.e. superoxide and hydrogen peroxide) and macrophages as a source of DNA damaging signals [Citation18]. Hei et al. investigated the inhibition of the ERK and confirmed the involvement of the MAPK signaling cascade in the bystander process [Citation19,Citation20]. MAPK pathways were associated with growth factor-mediated regulation of cellular proliferation, senescence, differentiation and apoptosis. Furthermore, Hei et al. provided evidence that NO and calcium signaling are part of the signaling cascade. Work of Zhou et al. demonstrated that the cyclooxygenase-2 (COX-2)-related pathway (which mediates cellular inflammatory response) was the crucial signaling link in the bystander phenomenon [Citation21].
It is plausible that some of the literature-reported signaling pathways might be involved in the decreased cell survival observed in this work.
The main emphasis of the current work was to investigate whether there is any impact of non-targeted additional cell killing induced by intercellular communication in dose cold spot. We were able to quantify, for the current experimental set-ups, the magnitude of survival reduction in under-dosed cells that were in direct contact and shared same environmental media with cells receiving higher radiation dose. Our results agreed with previously reported 10–30% survival reduction [Citation22,Citation23] and indicated involvement of factors that were previously associated with the radiation-induced bystander effects after exposure to modulated radiation fields or to irradiated cell conditioned medium.
To date, there have been several studies reported in the literature investigating cell survival in modulated radiation fields as intensity modulated radiation therapy (IMRT) is at present routinely used in many health institutions. For example, Bromley et al. [Citation24] investigated spatial distribution of cell growth after irradiation with modulated x-ray intensity pattern. The work aimed to develop a method which could be used for in vitro investigation of changes in cellular radiation response associated with IMRT techniques. Later, the same research group presented two methods of predicting the number of cells that would survive modulated x-ray irradiation [Citation25]. They reported differences between the measured and predicted surviving fraction of A549 cells following exposure to modulated x-ray fields: when the x-ray fluence map produced a steep dose gradient across the sample, fewer cells survived in the non-irradiated region than expected; when the x-ray fluence map produced a less steep dose gradient across the sample, more cells survived in the non-irradiated region than expected.
More recent studies of Butterworth et al. [Citation26] and Trainor et al. [Citation27] used modulated field irradiation and cell separation techniques similar to those of Suchowerska et al. [Citation28] and Bromley et al. [Citation25]. Both studies investigated responses to steep dose gradients across populations of cells and reported significant decreases in cell survival for cells outside the primary radiation fields. Butterworth concluded that out-of-field effects were important determinants of cellular response following exposure to modulated irradiation fields and that survival could be affected by cellular communication between differentially irradiated cells. These experiments were performed using sparsely seeded cell cultures. Therefore, the RIBE could have only occurred through release of soluble factors from irradiated cells.
In the current work non-uniform irradiations were performed directly within the primary field of the 6 MV x-ray beam, quality of which (in terms of low energy component of the fluence spectra) is different from the penumbra regions [Citation26] used in studies of Butterworth et al., Trainor et al. and Bromley et al. In the present study we investigated the worst possible scenario (from the clinical point of view) by simulating a dose cold spot on the central axis of the primary beam. Additionally, in this work novel cell separation technique was used. Unlike other experimental works investigating cell survival in modulated radiation fields [Citation25,Citation27], in this study cells were initially seeded at high densities to achieve relatively high cellular confluence during radiation treatment, and later cells were replated at low densities for colonies to grow. The advantage of treating densely populated cells is the close match to physiological in vivo conditions where cells are tightly packed. Additionally, this approach allows investigation of all types of radiation-induced cellular damages including direct hit and inactivation induced by intercellular signaling.
Our results, obtained from two independent sets of experiments with six-fold samplings for the first set of experiments and three-fold samplings for the repeated set of experiments, clearly indicate that for the PC3 cell line used and for given growing and irradiation conditions, there was statistically significant decrease in cell survival in dose cold spots, presumably due to intercellular communication. However, our findings do not imply that one can clinically ignore a dose cold spot in treatment planning and rely on intercellular signaling, which may or may not inactivate an unknown amount of clonogens. The mechanisms behind radiation-induced additional cell killing are still not clear. Little is also known about the types of DNA damage affecting bystander cells and whether irradiated cells have persistent ability to generate bystander signals.
From clinical point of view, the impact of non-targeted effects of ionizing radiation in novel radiotherapy treatment techniques, such as IMRT and tomotherapy, requires further investigation as these techniques deliver highly conformal but modulated doses to tumors and also cover bigger tissue volumes with lower integral doses where bystander responses are more pronounced [Citation29]. It is also possible to contemplate that if RIBE in vivo can be controlled and modulated, it may contribute to development of novel therapeutic approaches to cancer treatment and to improve the existing ones. However, its clinical relevance in radiotherapy remains yet to be elucidated.
Acknowledgments
The authors would like to thank Prof Wayne Tilley (the Dame Roma Mitchell Cancer Research Laboratories, University of Adelaide and the Hanson Institute) who kindly donated the PC3 cell line.
Declaration of interest: The authors report no conflicts of interest. The authors alone are responsible for the content and writing of the paper.
References
- Wilt TJ, MacDonald R, Rutks I, Shamliyan TA, Taylor BC, Kane RL. Systematic review: Comparative effectiveness and harms of treatments for clinically localized prostate cancer. Ann Intern Med 2008;148:435–48.
- Bromfield GP, Meng A, Warde P, Bristow RG. Cell death in irradiated prostate epithelial cells: Role of apoptotic and clonogenic cell kill. Prostate Cancer Prostatic Dis 2003;6: 73–85.
- Tome WA, Fowler JF. On cold spots in tumor subvolumes. Med Phys 2002;29:1590–8.
- Zhang Y, Zhou J, Baldwin J, Held KD, Prise KM, Redmond RW, et al. Ionizing radiation-induced bystander mutagenesis and adaptation: quantitative and temporal aspects. Mutat Res 2009;671:20–5.
- Ryan LA, Seymour CB, Joiner MC, Mothersill CE. Radiation-induced adaptive response is not seen in cell lines showing a bystander effect but is seen in lines showing HRS/IRR response. Int J Radiat Biol 2009;85:87–95.
- Hamada N. Evidence and significance of non-targeted effects of ionizing radiation. Curr Mol Pharmacol 2011;4:78.
- Butterworth KT, McGarry CK, O’Sullivan JM, Hounsell AR, Prise KM. A study of the biological effects of modulated 6 MV radiation fields. Phys Med Biol 2010;55:1607–18.
- Butterworth KT, McGarry CK, Trainor C, O’Sullivan JM, Hounsell AR, Prise KM. Out-of-field cell survival following exposure to intensity-modulated radiation fields. Int J Radiat Oncol Biol Phys 2011;79:1516–22.
- Puck TT, Marcus PI. Action of x-rays on mammalian cells. J Exp Med 1956;103:653–66.
- Mothersill C, Smith R, Singh H, Seymour C. Serum serotonin levels determine the magnitude and type of bystander effects in medium transfer experiments. Radiat Res 2010; 174:119–23.
- Mothersill C, Seymour C. Medium from irradiated human epithelial cells but not human fibroblasts reduces the clonogenic survival of unirradiated cells. Int J Radiat Biol 1997; 71:421–7.
- IAEA. Absorbed dose determination in external beam radiotherapy: An international code of practice based on the standards of absorbed dose to water. Technical Report Series 398. Vienna: IAEA; 2000.
- Klipp E, Liebermeister W. Mathematical modeling of intracellular signaling pathways. BMC Neurosci 2006;7 (Suppl 1):S10.
- Nagar S, Smith LE, Morgan WF. Mechanisms of cell death associated with death-inducing factors from genomically unstable cell lines. Mutagenesis 2003;18:549–60.
- Schaue D, Kachikwu E, McBride W. Cytokines in radiobiological responses: A review. Radiat Res 2012;178:505–23.
- Muller K, Meineke V. Radiation-induced alterations in cytokine production by skin cells. Exp Hematol 2007;35(4 Suppl 1):96–104.
- Bentzen SM. Preventing or reducing late side effects of radiation therapy: Radiobiology meets molecular pathology. Nat Rev Cancer 2006;6:702–13.
- Burr KL, Robinson JI, Rastogi S, Boylan MT, Coates PJ, Lorimore SA, et al. Radiation-induced delayed bystander-type effects mediated by hemopoietic cells. Radiat Res 2010;173:760–8.
- Hei TK, Zhou H, Ivanov VN, Hong M, Lieberman HB, Brenner DJ, et al. Mechanism of radiation-induced bystander effects: A unifying model. J Pharm Pharmacol 2008;60: 943–50.
- Hei TK, Zhou H, Chai Y, Ponnaiya B, Ivanov VN. Radiation induced non-targeted response: Mechanism and potential clinical implications. Curr Mol Pharmacol 2011;4:96–105.
- Zhou H, Ivanov VN, Gillespie J, Geard CR, Amundson SA, Brenner DJ, et al. Mechanism of radiation-induced bystander effect: Role of the cyclooxygenase-2 signaling pathway. Proc Natl Acad Sci U S A 2005;102:14641–6.
- Mackonis EC, Suchowerska N, Zhang M, Ebert M, McKenzie DR, Jackson M. Cellular response to modulated radiation fields. Phys Med Biol 2007; 52:5469–82.
- Mariotti L, Facoetti A, Alloni D, Bertolotti A, Ranza E, Ottolenghi A. Effects of ionizing radiation on cell-to-cell communication. Radiat Res 2010;174:280–9.
- Bromley R, Davey R, Oliver L, Harvie R, Baldock C. A preliminary investigation of cell growth after irradiation using a modulated x-ray intensity pattern. Phys Med Biol 2006;51: 3639–51.
- Bromley R, Oliver L, Davey R, Harvie R, Baldock C. Predicting the clonogenic survival of A549 cells after modulated x-ray irradiation using the linear quadratic model. Phys Med Biol 2009;54:187–206.
- Butterworth KT, McGarry CK, O’Sullivan JM, Hounsell AR, Prise KM. A study of the biological effects of modulated 6 MV radiation fields. Phys Med Biol 2010;55:1607–18.
- Trainor C, Butterworth K, Mc Garry C. DNA damage responses following exposure to modulated radiation fields. PLoS One 2012;7:e43326.
- Suchowerska N, Ebert MA, Zhang M, Jackson M. In vitro response of tumour cells to non-uniform irradiation. Phys Med Biol 2005;50:3041–51.
- Lisbona A, Averbeck D, Supiot S, Delpon G, Ali D, Vinas F, et al. IMRT combined to IGRT: increase of the irradiated volume. Consequences? Cancer Radiother 2010;14:563–70.