Abstract
Background Image-guided adaptive proton therapy (IGAPT) can potentially be applied to take into account interfraction motion while limiting organ at risk (OAR) dose in cervical cancer radiation therapy (RT). In this study, the potential dosimetric advantages of IGAPT compared with photon-based image-guided adaptive RT (IGART) were investigated.
Material and methods For 13 cervical cancer patients, full and empty bladder planning computed tomography (CT) images and weekly CTs were acquired. Based on both primary clinical target volumes (pCTVs) [i.e. gross tumor volume (GTV), cervix, corpus-uterus and upper part of the vagina] on planning CTs, the pretreatment observed full range primary internal target volume (pITV) was interpolated to derive pITV subranges. Given corresponding ITVs (i.e. pITVs including lymph nodes), patient-specific photon and proton plan libraries were generated. Using all weekly CTs, IGART and IGAPT treatments were simulated by selecting library plans and recalculating the dose. For each recalculated IGART and IGAPT fraction, CTV (i.e. pCTV including lymph nodes) coverage was assessed and differences in fractionated substitutes of dose-volume histogram (DVH) parameters (V15Gy, V30Gy, V45Gy, Dmean, D2cc) for bladder, bowel and rectum were tested for significance (Wilcoxon signed-rank test). Also, differences in toxicity-related DVH parameters (rectum V30Gy, bowel V45Gy) were approximated based on accumulated dose distributions.
Results In 92% (96%) of all recalculated IGAPT (IGART) fractions adequate CTV coverage (V95% >98%) was obtained. All dose parameters for bladder, bowel and rectum, except the fractionated substitute for rectum V45Gy, were improved using IGAPT. Also, IGAPT reduced the mean dose to bowel, bladder and rectum significantly (p < 0.01). In addition, an average decrease of rectum V30Gy and bowel V45Gy indicated reductions in toxicity probabilities when using IGAPT.
Conclusion This study demonstrates the feasibility of IGAPT in cervical cancer using a plan-library based plan-of-the-day approach. Compared to photon-based IGART, IGAPT maintains target coverage while significant dose reductions for the bladder, bowel and rectum can be achieved.
Radiation therapy (RT) for patients with locally advanced cervical cancer usually consists of external beam RT (EBRT) with concomitant chemotherapy or hyperthermia and subsequently brachytherapy [Citation1]. Compared to conformal EBRT, intensity-modulated RT (IMRT) allows highly conformal dose distributions resulting in decreased organ at risk (OAR) dose [Citation2]. To completely benefit from this potential advantage, adequate patient set-up based on daily image guidance is essential. However, despite drinking instructions, large bladder volume variations may induce interfraction target motion which increases the risk of target under-dosing and limits OAR sparing [Citation3].
Pre-fraction cone-beam computed tomography (CBCT) allows adapting the radiation delivery during the course of treatment. This is known as adaptive RT (ART) and several ART strategies have been investigated [Citation4,Citation5]. Similar to our current clinical practice, a practical approach frequently applied for pelvic EBRT is the plan-library based plan-of-the-day strategy [Citation6,Citation7]. Based on the pretreatment acquired target motion range, treatment plans corresponding to different target shapes and positions are created and using pre-fraction CBCT the plan best fitting the target shape is selected. Also in cervical cancer, this online adaptive strategy has the potential to correct for most interfraction target motion to ensure daily target coverage. However, OARs still receive substantial dose during photon-based image-guided ART (IGART) [Citation6,Citation7].
Compared to photons, protons have certain distinct advantages due to their finite range and hold the promise of limited OAR toxicity [Citation8,Citation9]. Intensity-modulated proton therapy (IMPT) enables a highly conformal dose delivery to complex-shaped target volumes including steep dose fall-offs around the target. However, due to its sensitivity to range and position uncertainties, IMPT dose delivery requires appropriate online image guidance [Citation10]. Moreover, anatomical changes can largely influence dose delivery and therefore IMPT can benefit from treatment adaptation based on pre-fraction imaging.
Previously, only a limited number of studies investigated the potential benefit of proton-based RT compared to photon-based RT in cervical cancer [Citation8,Citation11–13]. Even though the planning target volume (PTV) concept is less suitable for IMPT [Citation10], those studies used the PTV concept to anticipate on uncertainties. Moreover, none of them recalculated planned dose distributions using additional imaging data in order to evaluate differences between photon-based and proton-based RT in terms of delivered dose.
To investigate the actual benefit of proton therapy in cervical cancer, differences in delivered dose between photon-based and proton-based RT treatments using an adaptive strategy need to be determined. Therefore, our aim was to investigate the dosimetric advantages of image-guided adaptive proton therapy (IGAPT) in cervical cancer compared to photon-based IGART. Using a plan-library based plan-of-the-day adaptive strategy, both IGAPT and IGART treatments were simulated by recalculating selected plans on weekly CTs. Differences were evaluated in terms of dose-volume histogram (DVH) parameters and normal tissue complication probability (NTCP).
Material and methods
Patients and imaging
Thirteen cervical cancer patients treated with photon-based RT between January 2014 and August 2015 were included after obtaining written informed consent for additional CT imaging. These additional CTs were acquired solely for a pilot study to explore the potential benefit of ART and were approved by the local medical ethical committee. One patient was excluded because of image artifacts induced by a metal hip prosthesis. Patients were treated in prone position using a belly board device and in supine position when selected for para-aortic irradiation or when the prone position was not possible. In order to treat with a full bladder, patients were instructed to empty their bladder, to drink 0.5 l of water, and to refrain from voiding 1.5 hours prior to irradiation. Supplementary Table A1 (available online at http://www.informahealthcare.com) presents patient characteristics and clinical treatment details.
Besides the full bladder planning CT, an additional empty bladder planning CT prior to treatment and weekly CTs directly after RT delivery were acquired. All CT images with a slice thickness of 2.5 mm were obtained in RT treatment position. For two patients, the empty bladder planning CT was not acquired and the weekly CT with the smallest bladder volume was used as surrogate and excluded for treatment simulations. Three patients received four instead of five weekly CTs due to logistical issues and one patient received an additional sixth CT due to clinical issues regarding tumor response. The radiation oncologist delineated on all CTs the gross tumor volume (GTV), corpus-uterus, cervix, upper part of the vagina and elective lymph nodes. Also, the body, bowel cavity, as a surrogate for small bowel, rectum, kidneys and bladder were delineated using RTOG guidelines [Citation14]. The primary CTV (pCTV) encompassed the GTV, cervix, corpus-uterus and upper part of the vagina. The CTV included the pCTV and elective lymph nodes.
Library of structures
After bony registration of both planning CTs, the pCTV on the full bladder planning CT was registered to the pCTV on the empty bladder planning CT using a structure-based deformable image registration algorithm [Citation15]. The obtained deformation vectors after registration represented the connection between corresponding points on both structures. By linear scaling of the deformation vectors, the patient-specific full range primary internal target volume (pITV) was divided into three, one or two pITV subranges when the corpus-uterus tip displacement was above 20 mm, below 10 mm or in between, respectively (Supplementary Table A1 and Supplementary Figure A1, available online at http://www.informahealthcare.com). Corresponding to each pITV subrange an ITV was constructed by including elective lymph nodes.
Treatment planning
All treatment plans were generated with RayStation (version 4.4, RaySearch Laboratories AB, Stockholm, Sweden). Contrast agent induced high-density values on planning CTs used for vagina or bowel and bladder visualization were corrected to muscle (1.05 g/cm3) and water (1.0 g/cm3) density, respectively. Patient-specific plan libraries for both photons and protons were created based on a prescribed physical ITV dose of 46 Gy (23 × 2 Gy) for pelvic irradiation or 50.4 Gy (28 × 1.8 Gy) for para-aortic irradiation. Plans were robustly optimized on a uniform 3 mm dose grid with the beam isocenter set to the ITV center of mass using the full bladder planning CT [Citation16]. Plan optimizations were started with the initial set of planning objectives for each plan (Supplementary Table A2, available online at http://www.informahealthcare.com) and objective values were individually optimized to minimize OAR dose while maintaining ICRU-based ITV coverage (D98% >95%, D2% <107%). Since the prescribed target dose is below OAR dose limits, only target coverage robustness was evaluated by applying errors and recalculating the dose. For all perturbed dose distributions adequate ITV coverage was required.
Photon plans were generated using a 10-MV dual-arc volumetric modulated arc therapy (VMAT) technique (356° per arc, fixed 20° collimator angle). Besides the nominal isocenter position, six isocenter position shifts in each main direction (left, right, inferior, superior, posterior, anterior) were included to consider in total seven scenarios for robust optimization. Position shifts of 8 mm were applied, which equals the clinically used ITV-to-PTV margin in cervical cancer RT. For robustness evaluation, next to the six error scenarios used for optimization, eight additional error scenarios with 8 mm isocenter shifts along the diagonal of each octant in three-dimensional space were added. In total, 14 error scenarios were considered for ITV coverage robustness evaluation.
IMPT plans were generated based on pencil beam scanning [spot size in air: σ = 2.5–7.0 mm (226.7–70.0 MeV)] using four fixed posterior beams [30°, 90°, 270°, 330° (prone); 90°, 150°, 210°, 270° (supine)] [Citation17]. Assuming a proton relative biological effectiveness of 1.1 [Citation18], IMPT plans were created with a prescribed ITV dose of 46 Gy-equivalent or 50.4 Gy-equivalent. Besides the nominal isocenter position and the six isocenter position shifts of 8 mm in the main directions also three range errors (3%, 0%, -3%) were included to consider in total 21 scenarios for robust optimization. ITV coverage robustness was evaluated using 28 error scenarios, consisting of 14 position errors (8 mm) and two range errors (-3%, 3%). Similar to the VMAT plans, these position errors included isocenter position shifts in the six main directions and the eight diagonal directions of each octant in three-dimensional space.
Planned dose distributions
To explore differences between VMAT and IMPT plans, dose distributions for bladder, rectum and bowel cavity were evaluated. Planned dose parameters for the volumes receiving 15 Gy (V15Gy), 30 Gy (V30Gy) and 45 Gy (V45Gy) were extracted [Citation4,Citation19]. Because we aim to treat with a full bladder to minimize bowel irradiation, only planned dose parameters of library plans representing the full bladder planning CT anatomy were evaluated.
Recalculated fraction doses
Using all weekly CTs, IGART and IGAPT treatments were simulated by selecting plans from the created plan libraries and recalculating fraction dose distributions based on our clinical applied strategy. First, image-guided patient alignment was simulated by registering CTs to the full bladder planning CT based on bony anatomy using only translations. Patient-specific ITV structures were projected on the registered CT and the ITV encompassing the pCTV was selected. Both VMAT and IMPT library plans corresponding to the selected ITV were recalculated on the registered CTs to obtain fraction dose distributions.
IGART and IGAPT fraction dose distributions were verified on target coverage and compared for OAR doses. Target coverage was assessed by calculating the CTV receiving at least 95% of the prescribed fraction dose (V95%). Differences in bladder, bowel cavity and rectum sparing were verified by calculating the mean (Dmean) and maximum (D2cc) fraction dose and extracting fractionated substitutes of V15Gy, V30Gy and V45Gy (i.e. V15Gy-fx, V30Gy-fx and V45Gy-fx). A fractionated substitute represents the volume receiving the corresponding fraction dose (i.e. the dose level divided by the number of fractions). Patient-specific DVHs were plotted and dose differences between IGART and IGAPT fractions were tested pairwise for significance using a non-parametric statistical test (Wilcoxon signed-rank test).
OAR toxicity
In addition to the analysis based on recalculated fraction dose distributions, differences in toxicity probabilities for bowel and rectum were estimated. Differences between the DVH parameter associated with overall rectum toxicity (V30Gy) were derived [Citation19]. For bowel, NTCP differences associated with grade 2 acute gastrointestinal toxicity were quantified using
where V45Gy represents the volume (cm3) receiving 45 Gy, V50=410 cm3 and k = 3.2 [Citation20].
The toxicity-related DVH parameters (rectum V30Gy, bowel cavity V45Gy) were estimated by scaling patient-specific averages of rectum V30Gy-fx and bowel cavity V45Gy-fx with the prescribed number of fractions. Alternatively, CTs were registered deformably to the full bladder planning CT using the hybrid deformable registration algorithm implemented in RayStation [Citation21]. Based on these registrations, fraction dose distributions were warped and weighted accumulated to derive total delivered dose distributions. Toxicity-related DVH parameter values were extracted and differences were tested pairwise using a non-parametric statistical test (Wilcoxon signed rank test). The explanation and validation of the algorithm can be found in Supplementary Appendix C (available online at http://www.informahealthcare.com).
Results
Per patient, plan libraries consisting of clinically acceptable VMAT and IMPT plans were created (Supplementary Figure A2, available online at http://www.informahealthcare.com). Adequate ITV coverage (D98% ≥95%; D2% ≤107%) was found for all perturbed dose distributions after robustness evaluation and resulted in a total number of 58 robust library plans. Planned dose distributions indicated improvements in OAR sparing using IGAPT. The average planned V15Gy, V30Gy and V45Gy decreased with 0.1%, 13.0% and 26.0%, 19.0%, 27.0% and 15.5%, and 43.2%, 26.9% and 10.7% for rectum, bladder and bowel cavity, respectively. Supplementary Figures B1–B12 (available online at http://www.informahealthcare.com) show patient-specific DVHs of planned dose distributions.
After dose recalculation on the weekly CTs, six simulated fractions (10.7%) from four different patients showed inadequate CTV coverage for both the IGART and IGAPT strategy. Due to substantial deviating anatomy compared to the pretreatment derived full range pITV, library plans were inappropriate and these fractions were excluded from further analysis. For the remaining fractions, adequate target coverage (V95% >98%) was obtained in 92% (96%) of the recalculated IGAPT (IGART) plans and results on planned and recalculated target dose (D98%, D2%) are presented (). Although two (four) fractions resulted in CTV V95% <98% for the IGART (IGAPT) strategy while the IGAPT (IGART) strategy resulted in CTV V95% >98%, the average CTV coverage per patient was adequate for both strategies.
Figure 1. For the planned dose distributions based on the ITV (left) and the recalculated dose distribution based on the CTV (right), boxplots of target dose parameters over all patients are shown as percentage of the presribed dose for both the IGART and the IGAPT strategy. Boxes represent upper and lower quartiles (IQR), the band inside the box the median value and the whiskers the highest (lowest) value within 1.5 IQR of the upper (lower) quartile. Horizontal dashed lines indicate 95% and 107% of the prescribed target dose.
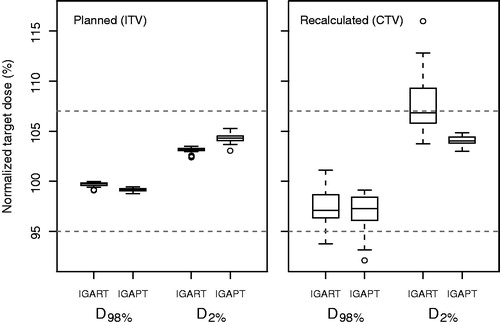
The simulated fractions indicated OAR dose reductions for V15Gy-fx, V30Gy-fx and V45Gy-fx, similar to the OAR dose reductions represented by the planned dose distributions. As an example, shows DVHs of recalculated fraction dose distributions for two typical patients. Supplementary Figures B1–B12 (available online at http://www.informahealthcare.com) show fraction DVHs for each patient. All DVH parameters, except rectum V45Gy-fx, showed a relative improvement in OAR sparing when using IGAPT. Also, both Dmean and V30Gy-fx for bladder, rectum and bowel cavity decreased significantly (). presents the mean dosimetric parameter values for the OAR evaluations and the absolute and relative differences between IGART and IGAPT.
Figure 2. For Patient 1 (a–d) and Patient 5 (e–h), DVHs of recalculated fraction dose distributions are shown for target and OARs based on VMAT plans for the IGART strategy (solid lines) and IMPT plans for the IGAPT strategy (dotted lines). The intersection of the two solid black lines indicates V95%=98%. For one fraction of Patient 1 (b), IGAPT resulted in inadequate CTV coverage while CTV coverage using IGART was adequate.
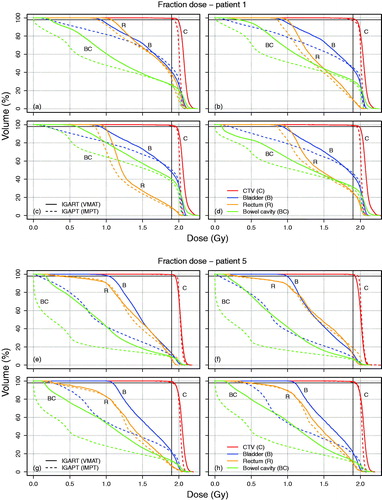
Figure 3. For bladder (upper), rectum (middle) and bowel cavity (lower), boxplots of fraction DVH parameters over all analyzed fractions of all patients are shown. Boxes represent upper and lower quartiles (IQR), the band inside the box the median value and the whiskers the highest (lowest) value within 1.5 IQR of the upper (lower) quartile. Horizontal lines including an asterisk indicate statistical significant difference (p < 0.01) based on a paired non-parametric statistical test.
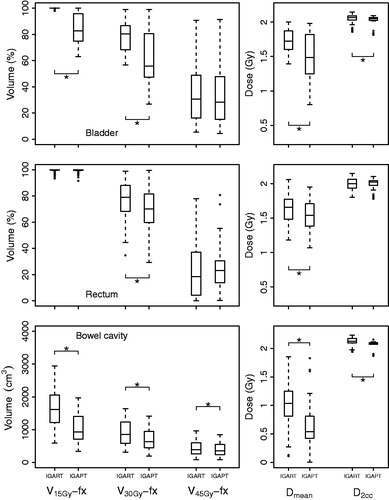
Table 1. Comparison of the mean dosimetric parameters for the recalculated fractions of all patients.
The scaled averages of DVH parameters indicated a reduction in rectum V30Gy of 8% for IGAPT (average, 69%) compared to IGART (average, 77%). Also, a decrease in NTCP for bowel of 0.07 was estimated for IGAPT (average, 0.43) compared to IGART (average, 0.50). Dose accumulation based on deformable registration resulted in an average rectum V30Gy difference of 7% between IGART (average, 81%) and IGAPT (average, 74%). For bowel, the NTCP decreased on average 0.15 when using IGAPT (average, 0.18) instead of IGART (average, 0.33). Moreover, IGAPT resulted in significant improvements (p < 0.01) for rectum V30Gy and bowel NTCP. However, two (one) patients showed a limited increase in rectum V30Gy (bowel NTCP) for IGAPT compared to IGART.
Discussion
In this study, we investigated the feasibility and potential dosimetric advantages of adaptive proton therapy compared to adaptive photon therapy in cervical cancer. Using a plan-library based plan-of-the-day image-guided adaptive strategy to compensate for anatomical interfraction changes, IGART and IGAPT treatments were simulated using weekly CTs and compared for target coverage and OAR dose. Our study is the first to demonstrate the potential benefit of IGAPT in cervical cancer including a dosimetric analysis based on weekly CTs. Compared to photon-based IGART, IGAPT maintains adequate target coverage while significant dose reductions can be achieved for bladder, bowel cavity and rectum, which results in decreased toxicity.
Unlike previously reported studies on proton therapy in cervical cancer [Citation8,Citation11–13], our analysis included treatment simulations by recalculating dose distributions using additional imaging obtained during treatment. To anticipate on anatomical changes during the treatment course and prevent incorrect dose delivery, we combined proton therapy with a state-of-the-art adaptive strategy. Consequently, our recalculated dose distributions are more representative in terms of target coverage and OAR sparing compared to the use of only planned proton dose distributions.
Besides the recalculation of dose distributions, the highly recommended robust optimization for proton therapy planning was applied using reported uncertainty values instead of using the photon-based PTV concept based on population-based margins [Citation10,Citation17]. However, uncertainty values reflecting the optimal trade-off between target coverage robustness and OAR sparing need to be derived. To compare IGART and IGAPT fairly, also VMAT plans were robustly optimized using reported uncertainty values thereby eliminating the influence of different optimization techniques. Consequently, IGART results derived in this study do not directly represent results of our clinically applied PTV-based IGART treatments. To complete the comparison between IGART and IGAPT, additional research is needed to investigate the dosimetric advantage of IGAPT compared to our clinical IGART results.
Except for the robust optimization, the applied adaptive strategy in this study is similar to our clinically implemented adaptive strategy for cervical cancer RT. Prior to treatment, the full range pITV was derived based on two planning CTs with extreme bladder volumes and interpolated to obtain pITV subranges. The number of pITVs generated is based on our clinical experience and the pretreatment predicted full range pITV was sufficient for almost all included patients. However, 10.7% of all fractions showed substantial deviation from the pretreatment derived full range pITV which resulted in inappropriate library plans. Since additional shifts after bony registration are not permitted to ensure lymph node coverage, treatment fractions will be postponed in clinical practice and justified the exclusion of this limited amount of fractions from our analysis. Even when this percentage increases, the use of a motion-robust backup plan (i.e. single field uniform dose plan to the full range ITV, including robust optimization using larger uncertainty values) can be considered.
Next to interfraction motion, intrafraction organ motion during cervical cancer RT can be considerable and might affect dose delivery [Citation22]. Besides potentially prolonged delivery times, IGAPT is more sensitive to uncertainties compared to IGART and intrafraction motion should be taken into account. In this study, we anticipated on possible intrafraction motion by robust optimization using appropriate uncertainty values. Moreover, the use of ITVs in our proposed adaptive strategy already compensated for possible intrafraction target motion induced by intrafraction bladder filling. Hence, the combination of ITVs with robust optimization is assumed to limit dosimetric consequences of intrafraction motion.
Similar to our current clinical workflow, all library plans were optimized using the full bladder planning CT. Preferably, plan optimization would be performed using the CT representing the corresponding anatomy. However, since multiple library plans were created corresponding to targets obtained by interpolation, available planning CTs do not necessarily represent associated anatomy for all target volumes.
Similar to the plan selection procedure applied clinically, the library plan best fitting the target shape and position as seen on pre-fraction imaging was selected visually after bony registration. In our study, plan selection was relatively easy since only CTs were used. CBCT-based plan selection is more challenging due to limited soft-tissue contrast, resulting in an additional observer variation. To minimize the effect of this variation, plan selection based on automatic organ segmentation could be applied [Citation23]. However, disturbed CBCT quality could hinder automatic organ segmentation and still requires manual selection. Alternatively, dose-guided plan selection could be beneficial and can be applied when online recalculation of library plans based on pre-fraction imaging will become available [Citation24].
Although cervical cancer irradiation is generally delivered in 23 or 28 fractions, we only used a limited number of weekly CTs per patient to simulate adaptive treatments. Consequently, the complete range of anatomical deformations occurring during the course of treatment is not necessarily included. Even though the included anatomical changes can influence observed dose differences due to their weighted contribution, this influence is assumed to be limited due to the substantial number of included patients. To completely eliminate these possible overestimations or underestimations of dosimetric differences, daily anatomical variations preferably based on an increased number of patients need to be included. However, daily CBCT images are not directly suitable for dose calculations.
Our dosimetric analysis is mainly based on DVHs of recalculated fraction dose distributions, from which parameter values were extracted. DVH parameter differences between recalculated IGART and IGAPT fractions were analyzed and statistical tests were applied pairwise to allow different fractionation schemes. In addition to the analysis based on fraction DVH parameters, reductions in toxicity probabilities for rectum and bowel were estimated based on DVH parameters representing the complete treatment course. Because no spatial information is present in fraction DVHs, the scaled average fraction DVH parameter approximation not necessarily represents reliably accumulated OAR parameter values. Therefore, DVH parameters were also derived based on dose accumulation after deformable image registration. For rectum V30Gy, both methods resulted in similar parameter values while an improvement for bowel V45Gy was observed when using the dose accumulation method.
Although the deformable registration algorithm was validated previously for pelvic registrations [Citation21], we validated our registrations by visual inspection and OAR similarity quantification to ensure plausible dose warping. However, an extensive validation of the complete anatomical correctness and the influence of possible incorrectness on dose accumulation is still an important challenge in RT [Citation25] and beyond the scope of this study.
According to QUANTEC recommendations, differences in NTCP for grade 2 acute gastrointestinal toxicity were quantified using the associated DVH parameter (bowel V45Gy) [Citation20]. However, the recommended lowest toxicity thresholds for rectum (V50Gy <50%) and bladder (V65Gy <50%) were in our study never reached. To differentiate between IGAPT and IGART, the reported DVH parameter associated with overall rectum toxicity (V30Gy) was used [Citation19].
Even though the application of IGAPT in cervical cancer has been demonstrated, additional steps will have to be taken before implementing this technique clinically. Daily pre-fraction imaging, preferably using magnetic resonance imaging or CT, will be required for plan selection. In addition, clinical logistics including appropriate protocols regarding plan selection need to be developed.
In conclusion, our study demonstrates the feasibility of adaptive proton therapy in cervical cancer. Compared to photon-based IGART, simulated IGAPT treatments result in adequate target coverage while the OAR dose decreases significantly. In addition, our results indicate toxicity probability reductions, but further analysis is needed to determine clinical outcome in terms of toxicity.
SupplementaryMaterial_AJAJvandeSchoot__revised_.pdf
Download PDF (5 MB)Acknowledgments
The authors would like to gratefully acknowledge RaySearch Laboratories AB, Stockholm, Sweden for providing access to the RayStation treatment planning system for this study. The authors would also like to thank Dr. M. Hoogeman (Erasmus MC, Rotterdam, The Netherlands) for making the Erasmus RTStudio, an application of the Erasmus MatterhornRT Software Development Platform, available.
Disclosure statement
The authors report no conflict of interest. The authors alone are responsible for the content and writing of the paper. Dr Bel does consultancy work for Elekta, is project leader of several Elekta-sponsored projects and reports non-financial support from RaySearch, outside the submitted work. Elekta and RaySearch had no involvement in study design, data collection and analysis, and writing of the manuscript.
References
- Eifel PJ, Winter K, Morris M, Levenback C, Grigsby PW, Cooper J, et al. Pelvic irradiation with concurrent chemotherapy versus pelvic and para-aortic irradiation for high-risk cervical cancer: an update of radiation therapy oncology group trial (RTOG) 90-01. J Clin Oncol 2004;22:872–80.
- Portelance L, Chao KS, Grigsby PW, Bennet H, Low D. Intensity-modulated radiation therapy (IMRT) reduces small bowel, rectum, and bladder doses in patients with cervical cancer receiving pelvic and para-aortic irradiation. Int. J Radiat Oncol Biol Phys 2001;51:261–6.
- Jadon R, Pembroke CA, Hanna CL, Palaniappan N, Evans M, Cleves AE, et al. A systematic review of organ motion and image-guided strategies in external beam radiotherapy for cervical cancer. Clin Oncol 2014;26:185–96.
- Stewart J, Lim K, Kelly V, Xie J, Brock KK, Moseley J, et al. Automated weekly replanning for intensity-modulated radiotherapy of cervix cancer. Int J Radiat Oncol Biol Phys 2010;78:350–8.
- Vestergaard A, Søndergaard J, Petersen JB, Høyer M, Muren LP. A comparison of three different adaptive strategies in image-guided radiotherapy of bladder cancer. Acta Oncol 2010;49:1069–76.
- Lutkenhaus LJ, Visser J, de Jong R, Hulshof MCCM, Bel A. Evaluation of delivered dose for a clinical daily adaptive plan selection strategy for bladder cancer radiotherapy. Radiother Oncol 2015;116:51–6.
- Heijkoop ST, Langerak TR, Quint S, Bondar L, Mens JWM, Heijmen BJM, et al. Clinical implementation of an online adaptive plan-of-the-day protocol for nonrigid motion management in locally advanced cervical cancer IMRT. Int J Radiat Oncol Biol Phys 2014;90:673–9.
- Marnitz S, Wlodarczyk W, Neumann O, Koehler C, Weihrauch M, Budach V, et al. Which technique for radiation is most beneficial for patients with locally advanced cervical cancer? Intensity modulated proton therapy versus intensity modulated photon treatment, helical tomotherapy and volumetric arc therapy for primary radiation – an individual comparison. Radiat Oncol 2015;10:91.
- Johansson B, Ridderheim M, Glimelius B. The potential of proton beam radiation therapy in prostate cancer, other urological cancers and gynaecological cancers. Acta Oncol 2005;44:890–5.
- Engelsman M, Schwarz M, Dong L. Physics controversies in proton therapy. Semin Radiat Oncol 2013;23:88–96.
- Song WY, Huh SN, Liang Y, White G, Nichols RC, Watkins T, et al. Dosimetric comparison study between intensity modulated radiation therapy and threedimensional conformal proton therapy for pelvic bone marrow sparing in the treatment of cervical cancer. J Appl Clin Med Phys 2014;11:1–8.
- Georg D, Kirisits C, Hillbrand M, Dimopoulos J, Pötter R. Image-guided radiotherapy for cervix cancer: high-tech external beam therapy versus high-tech brachytherapy. Int J Radiat Oncol Biol Phys 2008;71:1272–8.
- Milby AB, Both S, Ingram M, Lin LL. Dosimetric comparison of combined intensity-modulated radiotherapy (IMRT) and proton therapy versus IMRT alone for pelvic and para-aortic radiotherapy in gynecologic malignancies. Int J Radiat Oncol Biol Phys 2012;82:e477–84.
- Gay HA, Barthold HJ, O’Meara E, Bosch WR, El Naqa I, Al-Lozi R, et al. Pelvic normal tissue contouring guidelines for radiation therapy: a Radiation Therapy Oncology Group consensus panel atlas. Int J Radiat Oncol Biol Phys 2012;83:e353–62.
- Bondar L, Hoogeman MS, Vásquez Osorio EM, Heijmen BJM. A symmetric nonrigid registration method to handle large organ deformations in cervical cancer patients. Med Phys 2010;37:3760–72.
- Fredriksson A, Forsgren A, Hårdemark B. Minimax optimization for handling range and setup uncertainties in proton therapy. Med Phys 2011;38:1672–84.
- van de Schoot AJAJ, Visser J, van Kesteren Z, Janssen TM, Rasch CRN, Bel A. Beam configuration selection for robust intensity-modulated proton therapy in cervical cancer using Pareto front comparison. Phys Med Biol 2016; 61:1780-1794
- Paganetti H, Niemierko A, Ancukiewicz M, Gerweck LE, Goitein M, Loeffler JS, et al. Relative biological effectiveness (RBE) values for proton beam therapy. Int J Radiat Oncol Biol Phys 2002;53:407–21.
- Stenmark MH, Conlon ASC, Johnson S, Daignault S, Litzenberg D, Marsh R, et al. Dose to the inferior rectum is strongly associated with patient reported bowel quality of life after radiation therapy for prostate cancer. Radiother Oncol 2014;110:291–7.
- Roeske JC, Bonta D, Mell LK, Lujan AE, Mundt AJ. A dosimetric analysis of acute gastrointestinal toxicity in women receiving intensity-modulated whole-pelvic radiation therapy. Radiother Oncol 2003;69:201–7.
- Weistrand O, Svensson S. The ANACONDA algorithm for deformable image registration in radiotherapy. Med Phys 2015;42:40–53.
- Heijkoop ST, Langerak TR, Quint S, Mens JWM, Zolnay AG, Heijmen BJM, et al. Quantification of intra-fraction changes during radiotherapy of cervical cancer assessed with pre- and post-fraction Cone Beam CT scans. Radiother Oncol 2015;117:536–41.
- van de Schoot AJAJ, Schooneveldt G, Wognum S, Hoogeman MS, Chai X, Stalpers LJA, et al. Generic method for automatic bladder segmentation on cone beam CT using a patient-specific bladder shape model. Med Phys 2014;41:031707.
- van Rooijen DC, van Wieringen N, Stippel G, Crezee J, Koning CCE, Bel A. Dose-guided radiotherapy: potential benefit of online dose recalculation for stereotactic lung irradiation in patients with non-small-cell lung cancer. Int J Radiat Oncol Biol Phys 2012;83:e557–62.
- Wognum S, Heethuis SE, Rosario T, Hoogeman MS, Bel A. Validation of deformable image registration algorithms on CT images of ex vivo porcine bladders with fiducial markers. Med Phys 2014;41:071916.