Abstract
The aim of this experimental study was to investigate whether, and how then, docosahexaenoic acid (DHA) could alleviate the cyclosporine A (CsA)-induced nephrotoxicity. Three main groups of Sprague–Dawley rats were treated orally with CsA (25 mg/kg), DHA (100 mg/kg), and CsA along with DHA. A corresponding control group was also used. DHA administration significantly reduced CsA-induced nephrotoxicity and associated hyperlipidemia and proteinuria as assessed by estimating serum triacylglycerol, serum total cholesterol, serum total protein, serum urea, and creatinine clearance. Furthermore, urinary excretions of protein and N-acetyl-β-d-glucosaminidase were significantly inhibited following DHA administration. DHA supplementation slightly attenuated the oxidative damage in kidney tissues as evaluated by the levels of thiobarbituric acid-reacting substances and protein carbonyl content in the kidney homogenate, although there were no significant differences between CsA-intoxicated and DHA-treated animals. Moreover, DHA treatment significantly restored total nitric oxide (NO) levels in both renal tissues and urine. This study demonstrates the ability of DHA to ameliorate CsA-induced renal dysfunction, which might be beneficial to enhance the therapeutic index of CsA. The data suggest that the protective potential of DHA in the prevention of CsA nephrotoxicity in rats was mainly associated with the increase of total NO bioavailability in renal tissues. Nevertheless, the exact independent mechanism in which DHA exerts its beneficial effect is yet to be fully elucidated.
INTRODUCTION
Cyclosporine A (CsA) is a highly insoluble polypeptide consisting of 11 amino acids. It is an immunosuppressive drug that has provided a crucial support in transplantation medicine and has improved survival rates after organ transplantation, when compared with conventional immunosuppressant therapy. However, the renal damage consequent to CsA administration has limited its clinical usage.Citation1–3
CsA nephrotoxicity is distinguished by inducing a strong vasoconstriction in the afferent preglomerular arteriole that induces a decrease in renal plasma flow and glomerular filtration rate leading to renal function impairment. Renal vasoconstriction is attributed to a disturbance in the complex dynamic interaction of predominating vasoconstrictors with counteracting vasoactive substances.Citation2,Citation4–8 Moreover, the oxidative damage in the body induced by reactive oxygen species and lipid peroxidation products is frequently reported in the CsA nephrotoxicity.Citation9–11 The involvement of free radical species in CsA nephrotoxicity was supported by the fact that many antioxidants and free radical scavengers provide marked functional protection.Citation12–15
Docosahexaenoic acid (22:6, n–3; DHA) is an essential polyunsaturated fatty acid, which is predominant in fish and marine oils.Citation16 Polyunsaturated fatty acids are known to have potential cardiovascular benefits that include lowering blood pressure and improving vascular tone, whereas their impact on lipid peroxidation has been controversial.Citation17–21 In view of this, we investigated the ability of DHA to restore the deteriorated renal functions induced by CsA in a rat model. Furthermore, renal tissue nitric oxide (NO) level, lipid peroxidation, protein oxidation, and urinary level of NO were investigated in an attempt to understand the mechanistic basis of the observed DHA activities.
MATERIALS AND METHODS
Chemicals
CsA [(Novartis Pharma AG, Basel, Switzerland)] was dissolved in olive oil to give a final concentration of 10 mg/mL. cis-4,7,10,13,16,19-DHA was purchased from Sigma-Aldrich Chemical (St. Louis, MO, USA). All other chemicals used were of the highest available commercial grade.
Animals
Forty-eight male Sprague–Dawley rats, weighing 160–180 g, were obtained from our animal facility (Al-Azhar University, Cairo, Egypt). The rats were kept in wire-floored cages under standard laboratory conditions of 12 h/12 h light/dark, 25 ± 2°C with free access to food and water.
Experimental Design
The rats were randomized and divided into 4 groups of 12 animals each. Two groups were treated by gavage with CsA at a dose of 25 mg/kg of body weight for 21 days. One of the two groups was fed orally with DHA at a dose of 100 mg/kg of body weight for 4 days before and for 21 days concomitant with CsA.Citation16,Citation11 The third group received DHA alone in the same dose and by the same route of administration for 25 consecutive days. The last group of animals served as control and received by gavage an equivalent volume of vehicle for the same number of days. In this experiment, 1 mL of olive oil for each animal in all groups was used as vehicle.
On the last day of treatment, rats in all groups were in individual metabolic cages housed for 24 h of urine collection. During urine collection animals had water ad libitum, whereas food was allowed for the first 12 h only. The volume of collected urine was measured to ascertain urine parameters [creatinine, total protein, N‐acetyl-β-d-glucosaminidase (NAG), and NO]. After urine collection, and at 24 h after the last injection, blood samples from starved animals were taken from abdominal aorta under light ether anesthesia, and used for determination of serum triacylglycerol (TAG), serum total cholesterol (TC), serum total protein, serum urea, and creatinine levels. All animals were then killed by cervical dislocation and both kidneys from each rat were quickly removed, rinsed in ice-cold physiological saline, blotted dry on filter paper, weighed, and then 10% (w/v) homogenate of the kidney was made in ice-cold 0.15 M KCl solution using a Potter–Elvehjem homogenizer. Aliquots of the homogenates were used for determination of tissue contents of thiobarbituric acid-reacting substances (TBARS) as index of lipid peroxidation, protein carbonyl content (PCC) as index of protein oxidation, and NO. Total protein content of homogenates was also determined to ascertain tissue parameters.
Biochemical Analysis
Serum TAG, TC, and urea were assayed according to the methods of Fossati and PrencipeCitation22; Roeschlau et al.,Citation23 and Hallet and Cook,Citation24 respectively. Creatinine clearance (Ccr) was calculated after estimation of serum and urinary creatinine levels using the alkaline picrate method.Citation25 NAG activity of the collected urine samples was measured using the methods of Moore and Morris.Citation26 Kidney homogenate was used for the determination of TBARS and PCC according to the methods of Mihara and UchiyamaCitation27 and Levine et al.,Citation28 respectively. Urine and tissue concentrations of NO were measured as its stable metabolites, nitrate and nitrite. Nitrate was first reduced by nitrate reductase to nitrite and then nitrite was determined spectrophotometrically by the Griess reaction.Citation29 The protein content of the serum, urine, and homogenate samples was measured by the method of Lowry et al.Citation30
Statistical Analysis
Data are expressed as mean ± SD for the groups. Data analysis was evaluated by one-way analysis of variance (ANOVA) followed by Tukey–Kramer test for multiple comparisons. A 0.05 level of probability was used as the criterion for significance.
RESULTS
The consequences of animal treatment with CsA aggravated an observed renal dysfunction, as evidenced by significant reduction in body weight gain (16%) and serum total protein (31%), whereas serum urea was significantly increased (169%) when compared with control animals. Furthermore, apparent hyperlipidemia was observed through the increased levels of serum TAG (47%) and serum TC (46%) as compared with the control values in vehicle-receiving rats. The administration of DHA significantly restored those modified values. Besides, an obvious reduction (79%) in Ccr was observed in the CsA-treated group, and it was highly improved upon DHA administration. Moreover, the nephrotic (CsA-treated) rats showed high urinary excretion of both protein (∼14-fold) and NAG (112%) compared with vehicle-treated non-nephrotic rats. A significant protection was observed upon DHA administration through decrease of the high urinary protein and NAG excretion ().
Table 1. Effect of DHA administration on body weight, serum total protein, serum urea, serum TAG, serum TC, urinary protein, urinary NAG, and Ccr modified by CsA treatment in rats
In nephrotic kidneys, CsA treatment significantly increased TBARS and PCC levels, which were noticeably alleviated upon administration of DHA ( and ).
Figure 1. Effect of DHA on the level of TBARs (index of lipid peroxidation) in the kidney of control, DHA, and CsA nephrotoxicity groups. Data are presented as mean ± SD, n = 12. Multiple comparisons were achieved using one-way ANOVA followed by Tukey–Kramer as post-ANOVA test.
Note: a and b indicate significant change from control, DHA, and CsA nephrotoxicity groups, respectively, at p < 0.05.
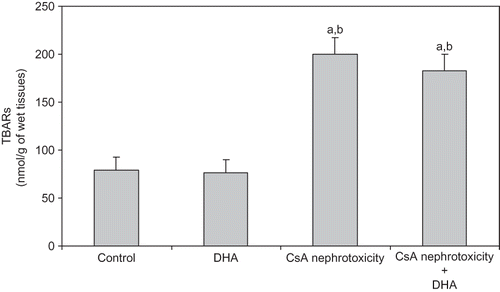
Figure 2. Effect of DHA on the level of PCC (index of protein oxidation) in the kidney of control, DHA, and CsA nephrotoxicity groups. Data are presented as mean ± SD, n = 12. Multiple comparisons were achieved using one-way ANOVA followed by Tukey–Kramer as post-ANOVA test.
Note: a and b indicate significant change from control, DHA, and CsA nephrotoxicity groups, respectively, at p < 0.05.
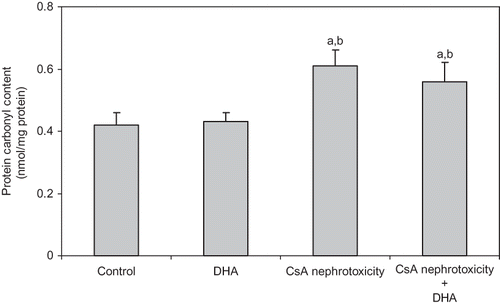
indicates that CsA treatment significantly decreased both nitrite concentration of kidney homogenate () and urinary excretion of nitrite () when compared with the control levels. Administration of DHA significantly increased the declined values of urinary and tissue nitrite almost to values obtained from the control group.
Figure 3. Effect of DHA administration on the renal tissue nitrite (A), and urinary excretion of nitrite (B) modified by CsA treatment in rats. Data are presented as mean ± SD, n = 12. Multiple comparisons were achieved using one-way ANOVA followed by Tukey–Kramer as post-ANOVA test.
Note: a, b, and c indicate significant change from control, DHA, and CsA nephrotoxicity groups, respectively, at p < 0.05.
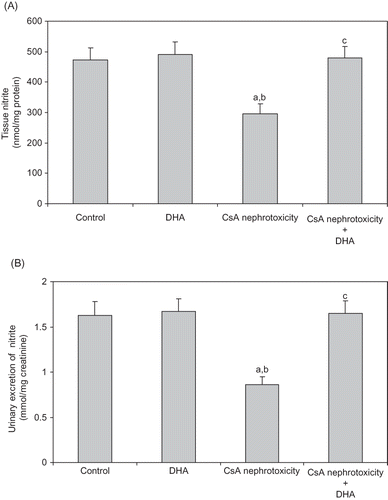
The solitary administration of DHA has insignificant changes on the levels of all parameters measured compared with the control animals.
DISCUSSION
The results of this study showed that CsA-treated rats (25 mg/kg for 21 days) revealed a severe nephropathy as reflected by body weight loss with substantial hypoproteinemia, high level of serum urea, hyperlipidemia, and proteinuria. This nephrotic toxicity was also associated with obvious decrease of Ccr and apparent renal tubular injury that is indicated by the increased urinary excretion of NAG (). This distinctive figure of CsA-induced nephrotoxicity is comparable with those reported by other research groups.Citation11,Citation13,Citation31,Citation32
CsA nephrotoxicity is parallel to its immunosuppressive property and cannot be totally avoided. The precise mechanism by which CsA causes renal injury remains obscure and is still not completely elucidated, but several studies suggest that transforming growth factor-β1 expression,Citation33 intracellular calcium handling,Citation34 oxidative stress,Citation35,Citation36 vasoconstriction, and altered renal hemodynamicsCitation37–39 are involved. However, participation of NO, an important renal vasodilator, is highly suggested to participate in CsA-induced nephrotoxicity. Gossmann et al.Citation5 reported that acetylcholine-induced vasodilatation is impaired in vascular beds of CsA-treated animals, which may indicate deficiency of endothelial NO synthesis. However, this conclusion can also be referred to the enhanced generation of free radicals in CsA-induced nephrotoxicity which is able to inactivate NO.Citation40
DHA administration in a dose of 100 mg/kg body weight significantly normalized all the measured parameters affected by CsA toxicity. DHA significantly decreased body weight loss, serum urea, serum TAG, serum TC, and urinary excretion of protein and NAG. It also increased Ccr and serum total protein when compared with the untreated CsA-intoxicated animals (). This is consistent with previous findings of Sabry et al.,Citation39 Kim and Chung, Citation41 and El-Mesery et al.,Citation42 who have demonstrated the protective renal effect of omega-3 fatty acids.
The results of this study also revealed a significant increase in TBARS generation and the level of PCC in the kidneys of CsA-treated animals ( and ). The ability of CsA-induced nephrotoxicity to modulate kidney oxidative status proves the role of increased oxidant stress. The role of free radicals formation and the consequent oxidative stress in the induction of CsA-induced nephrotoxicity are widely accepted throughout different studies that support this notionCitation9–11,Citation32. However, the contribution of DHA in prevention of renal oxidative stress induced by CsA was not that beneficial, although both lipid and protein oxidative parameters were slightly attenuated following DHA administration ( and ). In contrast to our results, Kubo et al.Citation43 reported that DHA is thought to be closely associated with the tissue lipid peroxide formation. More recently, Bouzidi et al.Citation44 reported that omega-3 supplementation improves oxidative stress in patients with chronic renal failure. Nevertheless, Calviello et al.Citation45 stated that DHA-induced lipid peroxidation is only dose dependant. Later, Sunada et al.,Citation46 Taccone-Gallucci et al.,Citation47 and Kim and ChungCitation41 reported that DHA neither affects susceptibility to oxidative stress nor promotes lipid peroxidation in different rat tissues even under high oxidative stress.
Moreover, we made an attempt to define the relationship between NO levels and CsA nephrotoxicity. The tissue and urine NO levels of rats intoxicated with CsA were significantly reduced ( and ), which indicates that abnormal NO bioavailability plays an important role in the pathogenesis of CsA-induced nephrotoxicity. Physiological levels of NO play an important role in the conservation of renal hemodynamics due to its vasodilator and antithrombogenic properties. It has been reported that decrease in renal NO generation is linked to the pathogenesis of renal disease.Citation48–51 The decreased bioavailability of NO in the kidney as a consequence of increased oxidative stress was also reported.Citation52,Citation53
Our study revealed that DHA significantly increased NO levels in both renal tissues and urine of CsA-treated rats ( and 3B). DHA administration was proved to increase the release of endothelium-derived relaxing factor, presumably NO, which facilitates arterial relaxation, decreases blood pressure, improves vascularity, and consequently reduces the kidney function impairment.Citation17,Citation54 Similar results were reported by Robinson and Stone,Citation20 who observed that DHA enhanced the formation of NO, which induces its hypotensive effect.
Under our experimental conditions, DHA single treatment of rats did not significantly affect any of the studied parameters compared with the control animals.
In conclusion, this study provided further evidence to support the involvement of NO in pathogenesis of nephrotoxicity caused by CsA. These findings also suggested that administration of DHA exerts a renoprotective effect in CsA-induced nephrotoxicity. It seems that the protective effect induced by DHA is most probably due to the regulation of NO bioavailability in the kidney. However, the detailed mechanism by which DHA decreased the high levels of NO in both kidney and urine of CsA-treated rats is not well defined. Therefore, further investigation is required to elucidate the detailed mechanism.
Declaration of interest:
The authors report no conflicts of interest. The authors alone are responsible for the content and writing of the paper.
REFERENCES
- Calne R, White D, Thiru S, Cyclosporin A in patients receiving renal allografts from cadaver donors. Lancet. 1978;2(8104–8105):1323–1327.
- Busauschina A, Schnuelle P, Vander Woude F. Cyclosporine nephrotoxicity. Transplant Proc. 2004;36(2):229S–233S.
- Li C, Lim S, Sun B, Yang C. Chronic cyclosporine nephrotoxicity: New insights and preventive strategies. Yonsei Med J. 2004;45(6):1004–1016.
- English J, Evan A, Houghton D, Bennett W. Cyclosporin-induced acute renal dysfunction in the rat. Evidence of arteriolar vasoconstriction with preservation of tubular function. Transplantation. 1987;44(1):135–141.
- Gossmann J, Radounikli A, Bernemann A, Pathophysiology of cyclosporine-induced nephrotoxicity in humans: A role for nitric oxide? Kidney Blood Press Res. 2001;24(2):111–115.
- Kon V, Sugiura M, Inagami T, Harvie B, Ichikawa I, Hoover R. Role of endothelin in cyclosporine-induced glomerular dysfunction. Kidney Int. 1990;37:1487–1491.
- Shihab F, Hong Y, Bennett W, Andoh T. Effect of nitric oxide modulation on TGF-beta1 and matrix proteins in chronic cyclosporine nephrotoxicity. Kidney Int. 2000;58(3):1174–1185.
- Vaziri N, Ni Z, Zhang Y, Ruzics E, Maleki P, Ding Y. Depressed renal and vascular nitric oxide synthase expression in cyclosporine-induced hypertension. Kidney Int. 1998;54: 482–491.
- Burdmann E, Andoh T, Yu L, Bennett W. Cyclosporine nephrotoxicity. Semin Nephrol. 2003;23(5):465–476.
- Parra Cid T, Conejo Garcia J, Carballo Alvarez F, de Arriba G. Antioxidant nutrients protect against cyclosporine A nephrotoxicity. Toxicology. 2003;189(1–2):99–111.
- Wongmekiat O, Leelarugrayub N, Thamprasert K. Beneficial effect of shallot (Allium ascalonicum L.) extract on cyclosporine nephrotoxicity in rats. Food Chem Toxicol. 2008;46(5): 1844–1850.
- Ghaznavi R, Zahmatkesh M, Kadkhodaee M, Mahdavi-Mazdeh M. Cyclosporine effects on the antioxidant capacity of rat renal tissues. Transplant Proc. 2007;39(4):866–867.
- Gökçe A, Oktar S, Yönden Z, Protective effect of caffeic acid phenethyl ester on cyclosporine A-induced nephrotoxicity in rats. Ren Fail. 2009;31(9):843–847.
- Pari L, Sivasankari R. Effect of ellagic acid on cyclosporine A-induced oxidative damage in the liver of rats. Fundam Clin Pharmacol. 2008;22(4):395–401.
- Wongmekiat O, Thamprasert K. Investigating the protective effects of aged garlic extract on cyclosporine-induced nephrotoxicity in rats. Fundam Clin Pharmacol. 2005;19(5):555–562.
- Hirunpanich V, Murakoso K, Sato H. Inhibitory effect of docosahexaenoic acid (DHA) on the intestinal metabolism of midazolam: In vitro and in vivo studies in rats. Int J Pharm. 2008;351(1–2):133–143.
- Harris W, Rambjor G, Windsor S, Diederich D. n-3 fatty acids and urinary excretion of nitric oxide metabolites in humans. Am J Clin Nutr. 1997;65:459–464.
- Nenseter M, Drevon C. Dietary polyunsaturates and peroxidation of low density lipoprotein. Curr Opin Lipidol. 1996; 7(1):8–13.
- Pan H, Kao T, Ou Y, Protective effect of docosahexaenoic acid against brain injury in ischemic rats. J Nutr Biochem. 2009;20(9):715–725.
- Robinson J, Stone N. Antiatherosclerotic and antithrombotic effects of omega-3 fatty acids. Am J Cardiol. 2006;98(4A):39i–49i.
- Song J, Fujimoto K, Miyazawal T. Polyunsaturated (n-3) Fatty acids susceptible to peroxidation are increased in plasma and tissue lipids of rats fed docosahexaenoic acid–containing oils. J Nutr. 2000;130(12):3028–3033.
- Fossati P, Prencipe L. Serum triglycerides determined colorimetrically with an enzyme that produces hydrogen peroxide. Clin Chem. 1982;28(10):2077–2080.
- Roeschlau P, Bernt E, Gruber W. Enzymatic determination of total cholesterol in serum. Z Klin Chem Klin Biochem. 1974; 12(5):226–230.
- Hallet G, Cook J. Reduced nicotinamide adenine dinucleotide for emergency blood urea estimation. Clin Chim Acta. 1971;35(1):33–37.
- Bonsnes R, Taussky H. On the colorimetric determination of creatinine by the Jaff reaction. J Biol Chem. 1945;158:581–591.
- Moore J, Morris J. A simple automated colorimetric method for determination of N-acetyl-β-D-glucosaminidase. Ann Clin Biochem. 1982;19(3):157–159.
- Mihara M, Uchiyama M. Determination of malonaldehyde precursor in tissues by thiobarbituric acid test. Anal Biochem. 1978;86(1):271–278.
- Levine R, Garland D, Oliver C, Determination of carbonyl content in oxidatively modified proteins. Methods Enzymol. 1990;186:464–478.
- Green L, Wagner D, Glogowski J, Skipper P, Wishnok J, Tannenbaum S. Analysis of nitrate, nitrite and [15N] nitrate in biological fluids. Anal Biochem. 1982;126(1):131–138.
- Lowry O, Rosebrough N, Farr A, Randall R. Protein measurement with the Folin phenol reagent. J Biol Chem. 1951;193(1): 265–275.
- Atessahin A, Ceribasi A, Yilmaz S. Lycopene a carotenoid, attenuates cyclosporine-induced renal dysfunction and oxidative stress in rats. Basic Clin Pharmacol Toxicol. 2007;100(6): 372–376.
- Mohamadin A, El-Beshbishy H, El-Mahdy M. Green tea extract attenuates cyclosporine A-induced oxidative stress in rats. Pharmacol Res. 2005;51(1):51–57.
- Shi S, Zheng S, Jia C, Zhu Y, Xie H. Inhibitory effect of tea polyphenals on transforming growth factor-beta1 expression in rat with cyclosporine A-induced chronic nephrotoxicity. Acta Pharmacol Sin. 2004;25(1):98–103.
- Cheng C, Hsieh C, Shu K, Chen Y, Chen H. Effect of calcium channel antagonist diltiazem and calcium ionophore A23187 on cyclosporine A induced apoptosis of renal tubular cells. FEBS Lett. 2002;516(1–3):191–196.
- Satyanarayana P, Chopra K. Oxidative stress-mediated renal dysfunction by cyclosporine A in rats: Attenuation by trimetazidine. Ren Fail. 2002;24(3):259–274.
- Tirkey N, Kaur G, Vij G, Chopra K. Curcumin a diferuloylmethane, attenuates cyclosporine-induced renal dysfunction and oxidative stress in rat kidneys. BMC Pharmacol. 2005;5: 15–24.
- Chander V, Chopra K. Effect of molsidomine and l-arginine in cyclosporine nephrotoxicity: Role of nitric oxide. Toxicology. 2005;207(3):463–474.
- De Nicola L, Thomson S, Wead L, Brown M, Gabbai F. Arginine feeding modifies cyclosporine nephrotoxicity in rats. J Clin Invest. 1993;92(4):1859–1865.
- Sabry A, El-Husseini A, Sheashaa H, Colchicine vs. omega-3 fatty acids for prevention of chronic cyclosporine nephrotoxicity in Sprague Dawley rats: An experimental animal model. Arch Med Res. 2006;37(8):933–940.
- Diederich D, Skopec J, Diederich A, Dai F. Cyclosporine produces endothelial dysfunction by increased production of superoxide. Hypertension. 1994;23(6 Pt 2):957–961.
- Kim Y, Chung H. Antioxidative and anti-inflammatory actions of docosahexaenoic acid and eicosapentaenoic acid in renal epithelial cells and macrophages. J Med Food. 2007; 10(2):225–231.
- El-Mesery M, Al-Gayyar M, Salem H, Darweish M, E‐Mowafy A. Chemopreventive and renal protective effects for docosahexaenoic acid (DHA): Implications of CRP and lipid peroxides. Cell Div. 2009;(4):6–22.
- Kubo K, Saito M, Tadokoro T, Maekawa A. Preferential incorporation of docosahexaenoic acid into nonphosphorus lipids and phosphatidylethanolamine protects rats from dietary DHA-stimulated lipid peroxidation. J Nutr. 2000;130(7): 1749–1759.
- Bouzidi N, Mekki K, Boukaddoum A, Dida N, Kaddous A, Bouchenak M. Effects of omega-3 polyunsaturated fatty acid supplementation on redox status in chronic renal failure patients with dyslipidemia. J Ren Nutr. 2010;20(5):321–328.
- Calviello G, Palozza P, Franceschelli P, Bartoli G. Low-dose eicosapentaenoic or docosahexaenoic acid administration modifies fatty acid composition and does not affect susceptibility to oxidative stress in rat erythrocytes and tissues. Lipids. 1997;32(10):1075–1083.
- Sunada S, Kiyose C, Kubo K, Takebayashi J, Sanada H, Saito M. Effect of docosahexaenoic acid intake on lipid peroxidation in diabetic rat retina under oxidative stress. Free Radic Res. 2006;40(8):837–846.
- Taccone-Gallucci M, Manca-di-Villahermosa S, Battistini L, Stuffler R, Tedesco M, Maccarrone M. N-3 PUFAs reduce oxidative stress in ESRD patients on maintenance HD by inhibiting 5-lipoxygenase activity. Kidney Int. 2006;69(8):1450–1454.
- Cattel V. Nitric oxide-potential mediator in glomerulonephritis. Nephrol Dial Transplant. 1995;10:759–774.
- Mumtaz F, Dashwood M, Khan M, Thompson C, Mikhailidis D, Morgan R. Down-regulation of nitric oxide synthase in the diabetic rabbit kidney: Potential relevance to the early pathogenesis of diabetic nephropathy. Curr Med Res Opin. 2004;20(1):1–6.
- Prabhakar S. Pathogenic role of nitric oxide alterations in diabetic nephropathy. Curr Diab Rep. 2005;5:449–454.
- Raij L, Baylis C. Glomerular action of nitric oxide. Kidney Int. 1995;48(1):20–32.
- Pacher P, Obrosova I, Mabley J, Szabo C. Role of nitrosative stress and peroxynitrite in the pathogenesis of diabetic complications: Emerging new therapeutical strategies. Curr Med Chem. 2005;12:267–275.
- Prabhakar S, Starnes J, Shi S, Lonis B, Tran R. Diabetic nephropathy is associated with oxidative stress and decreased renal nitric oxide production. J Am Soc Nephrol. 2007;18:2945–2952.
- Shimokawa H, Vanhoutte P. Dietary cod-liver oil improves endothelium dependent responses in hypercholesterolemic and atherosclenotic porcine coronary arteries. Circulation. 1988;78(6):1421–1430.